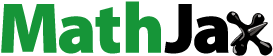
ABSTRACT
The use of antioxidant-rich medicinal plants having the potential to reduce oxidative stress and postprandial hyperglycemic pressure is one of the most promising option for the management of diabetes. This study presents information on metabolite profiling and in vitro anti-diabetic effects of leaf extracts of Ficus benjamina. The DPPH (2, 2-diphenyl-1-picrylhydrazyl radicals) assay was performed to determine the in vitro antioxidant potential of the plant extracts. The anti-diabetic effects were investigated by evaluating inhibitory properties of F. benjamina leaf extracts towards carbohydrate hydrolyzing enzymes, i.e., α-glucosidase and α-amylase, whereas 1H NMR and UHPLC-QTOF-MS/MS analytical methods were employed for metabolite profiling of F. benjamina leaf extracts. Among 40, 60, 80, and 100% ethanolic leaf extracts of F. benjamina, 80% ethanolic extract exhibited the highest antioxidant activity based upon its DPPH radical scavenging ability (IC50 value: 63.71 ± 2.66 µg/mL). The 80% ethanolic leaf extract of F. benjamina also proved to be the most efficient α-glucosidase and α-amylase inhibitor with IC50 values of 9.65 ± 1.04 µg/mL and 13.08 ± 1.06 µg/mL, respectively; these values were even better than acarbose with α-glucosidase inhibition activity (IC50 = 116.01 ± 3.83 µg/mL) and α-amylase inhibition activity (IC50 = 152.66 ± 7.32 µg/mL). Moreover, a total of 31 metabolites were identified in F. benjamina leaf extract, which may have the potential to contribute to its antioxidant and inhibitory properties against carbohydrate hydrolyzing enzymes. The findings of this study depict F. benjamina leaf extracts as a promising α-glucosidase and α-amylase inhibitor, and therefore, can be utilized for the development of anti-diabetic functional diets/nutra-pharmaceuticals.
Introduction
Diabetes mellitus is a common and diversified world-wide metabolic disorder, and is usually characterized by irregularities in blood glucose levels.[Citation1–Citation3] Diabetes pathogenesis results in adverse health complications, such as neuropathy, retinopathy, and cardiovascular disorders, etc.[Citation4–Citation6] In some cases, it also leads to death.[Citation1,Citation2] Numerous pharmacological agents having a diverse mode of action are being used for the cure and management of diabetes mellitus. Although, hormone therapy is effective for the treatment of diabetes mellitus, its use is restricted because of its limited actions and adverse side effects, such as hypoglycemia, weight loss, abdominal pain, damage to liver, diarrhea, lactic acidosis, and loss of appetite.[Citation5–Citation7] The other strategy to manage diabetes mellitus is based on reduction of glucose absorption through interference in the activity of α-glucosidase and α-amylase (carbohydrate-hydrolysing enzymes).[Citation8–Citation10] Alpha-amylase hydrolyzes polysaccharides to oligosaccharides, whereas α-glucosidase catalyzes the last carbohydrate hydrolysis step resulting in the generation of absorbable monosaccharides.[Citation11] Use of the inhibitors with ability to inhibit hydrolyzing enzymes could be a workable and emphatic approach to delay the uptake of dietary carbohydrates, leading to the suppression of postprandial hyperglycemia.[Citation8,Citation11,Citation12] To date, only a limited number of α-glucosidase and α-amylase inhibitors are available commercially; perhaps because their synthesis is very complex and also serious gastrointestinal complications have been reported to be linked with their usage.[Citation13]
Alternatively, medicinal herbs having promising therapeutic potential and minimal side effects are now getting much attention and recognition for the management of diabetes mellitus. For example, numerous medicinal plants have been explored and are reported to exhibit potent α-amylase and α-glucosidase inhibition properties.[Citation14–Citation17] Many of them are even more effective than the synthetic drugs.[Citation1,Citation18–Citation20] The search for novel medicinal plants that have potent anti-diabetic activity based on their inhibitory role toward α-amylase and α-glucosidase is of significant importance.
Ficus benjamina is a widely distributed tree common in Asia, America, Australia and in some European countries as well. Traditionally, it is used in folk medicines for therapeutic management of numerous diseases, such as respiratory tract infections, influenza, malaria and dysentery. It has also been reported to exhibit antitumor, antibacterial, antiviral and analgestic properties.[Citation21–Citation23] In the present study, F. benjamina leaf extract was investigated for its anti-enzymatic activity against α-amylase and α-glucosidase. Additionally, metabolite profiling of the extract was also carried out using analytical techniques like 1H NMR and UHPLC-QTOF-MS/MS.
Materials and methods
Chemicals and reagents
All the chemicals and reagents utilized during the current study were of analytical grade. Alpha-glucosidase and α-amylase enzymes, 1,1-diphenyl-2-picrylhydrazyl (DPPH), methanol, p-nitrophenyl-α-d-glucopyranoside, potassium dihydrogen phosphate (KH2PO4), glycine, dinitrosalicylic acid, and the reference standards including acarbose and butylated hydroxyanisole (BHA) were procured from Sigma–Aldrich (St. Louis, USA). Methanol-d4 (CD3OD, 99.8%), sodium deuterium oxide (NaOD) deuterium oxide (D2O, 99.9%) and trimethyllsilyl propionic acid-d4 sodium salt (TSP) were obtained from Merck (Darmstadt, Germany). UHPLC grade acetonitrile and ethanol were purchased from Merck (Darm-stadt, Germany). Liquid nitrogen was procured from MOX Company (Petaling Jaya, Malaysia).
Plant materials and extraction
Fresh leaves of the sample plant were obtained from Agriculture Park, University Putra Malaysia (UPM) and voucher specimen (H025) was submitted at Faculty of Forestry, UPM for identification. The plant specie was identified as Ficus benjamina. The fresh leaves were washed, initially dried with tissue paper, treated immediately with liquid nitrogen for instant quenching of secondary metabolites and then freeze-dried. The freeze-dried F. benjamina leaves were ground to powder and extraction was carried out by dissolving 10 grams of powdered F. benjamina leaves in each of 40, 60, 80, and 100% ethanol. The resultant mixtures were vortexed at ambient temperature and ultra-sonicated for 1 hr. Filtration was done using filter paper (Whatman, no. 1) and excess solvent was removed on rotary evaporator at 40°C under vacuum till the recovery of the extract. The crude F. benjamina extracts were finally freeze-dried and stored at −80°C till further use.
DPPH radical scavenging activity
DPPH (2,2-diphenyl-1-picrylhydrazyl radicals) radical scavenging activity of ethanolic F. benjamina leaf extracts was tested to assess their antioxidant potential.[Citation24,Citation25] Briefly, 0.25 mL of various concentrations (10–100 μg/mL) prepared from F. benjamina extracts and standard antioxidant (BHA) were mixed with 1.75 mL of DPPH reagent in a microplate. The plate containing reaction mixtures was incubated for 30 min at room temperature in a dark room. The absorbance was then measured at 515 nm using Elisa plate reader (Biotek, EL 800) and % inhibition was calculated using the following formula:-
where A (Sample) is the absorbance of the test sample, while A (Blank) is absorbance of the blank/control consisting of all the reagents except sample. Moreover, IC50 for the extract was calculated by plotting extract concentration against % inhibition.
1H-NMR spectroscopic analysis of Ficus benjamina leaf extract
For 1H-NMR analysis briefly, 25 mg of the F. benjamina leaf extract was taken in a 2.0 mL micro tube followed by the addition of 0.7 mL of methanol-d4 and KH2PO4 buffer in 1:1 (pH, 6.0 units) along with 0.1% TSP (Trimethylsilypropionic acid sodium salt). Each micro tube was then vortexed at room temperature for 1 min followed by ultrasonication (15 min) and centrifugation at 13000 rpm for 10 min. The supernatant was separated and transferred (0.6 mL) to NMR tubes for 1H-NMR analysis which was executed with a 500 MHz spectrometer (Varian INOVA model Inc., California, USA). The operation frequency of instrument was 499.887 MHz and each spectrum was recorded at 26°C. Every spectrum was constituted of 64 scans having 20 ppm width with acquisition time of 3.53 min. Phasing and baseline correction of the spectra were carried out by using Chenomx software (v. 5.1. Alberta, Canada). Moreover, chenomx library, literature study along with two-dimensional 1H–1H J-resolved was used for the identification of compounds.
Ultrahigh performance liquid chromatography- quadropole/time of flight- tandem mass spectrometry (UHPLC–QTOF-MS/MS)
UHPLC–QTOF-MS/MS analysis was executed using Agilent 1290 UHPLC coupled with Sciex High Resolution MS/MS with Accurate mass QTOF. The system was fitted with Phenomenex Synergy RP C18 column (100 mm x 2.0 mm x 3 uM). The mobile phase composed of water (A) and acetonitrile (B) having 0.1% formic acid and 5 mM ammonium formate was used for gradient elution programming: 5% B to 95% B from 0.01 min to 20 min, hold for 5 min and back to 5% B in 0.1 min and re-equilibrated for 5 min. Each plant extract (2 mg) was dissolved in 1 mL methanol (80%) and filtered through 0.45 um nylon filter and a sample volume of 10 uL was injected into LC-MS/MS. Mass spectrometric data were acquired based on tandem mass spectrometer using ESI source in Negative ionization mode and accurate mass QTOF. Full scan MS/MS data were collected at a resolving power 70000 FWHM with scanning range of m/z 50–1000 amu. The UHPLC-QTOF-MS/MS system was equipped with mass spectral data processing software (Analyst 1.5.2) and also with an ACD/Labs spectral library for structure elucidation (ACD/Labs, Inc., Toronto, ON, Canada). The information inferred from molecular ion peak data and possible patterns of mass fragmentation were utilized to identify the peaks resolved in chromatogram. Spectral data were evaluated and confirmed by comparing with ACD/Labs library and previously reported information.
Inhibition of α-glucosidase activity assay
Alpha-glucosidase inhibition activity of F. benjamina leaf extract was performed using the method described by Jabeen et al.[Citation26] with some modification in 96 well micro-plate. Briefly, 10 µL of the each extract was mixed separately with 70 µL of 100 mM potassium phosphate buffer having pH of 6.8 units. After preparing appropriate dilutions, 10 µL α-glucosidase (1 unit/mL) was added and the mixture was incubated at 37°C for 10 min. The reaction was initiated by adding 10 µL of substrate (5 mM p-nitrophenyl glucopyranoside). After having 30 min incubation at 37°C, the reaction was stopped with the addition of 50 µL glycine (adjusted to pH 10 units). Finally, the absorbance was measured for released p-nitrophenol at 405 nm using Elisa 96-well micro-plate reader (Biotek, EL 800) and percent α-glucosidase inhibition was calculated. Acarbose and quercetin were used as reference inhibitors/positive control. All the experiments were carried out in triplicate. Moreover, IC50 for the extract was calculated by plotting extract concentration against % inhibition.
Inhibition of α-amylase activity assay
Alpha-amylase inhibition activity of F. benjamina leaf extract was executed using a modified procedure described by Kazeem et al.[Citation16] The mixture was consisted of 200 μL of F. benjamina leaf extract (5.0–30 µ/mL), 200 μL of 0.02 M sodium phosphate buffer (pH 6.9 units) and 0.5 mg/mL α-amylase solution. The mixture was preincubated for 10 min at 25∘C, following the addition of 200 μL 1% starch solution (prepared in 0.02 M sodium phosphate buffer (pH 6.9 units) and was incubated further at 25∘C for 10 min. 400 μL of DNS (dinitrosalicylic acid) reagent was then added to terminate the reaction. Finally, absorbance was taken at 540 nm. Parallel to this, a control (without extract) was also run under same set of conditions.
Results and discussion
Percent extraction yield
Determination of quantitative yield of a plant extract is first imperative step during the evaluation of biological activity of medicinal plants. Extraction from F. benjamina leaves was made by using 40, 60, 80, and 100% aqueous ethanol as an extraction solvent. The effect of extraction solvent, ethanol, used at variable concentrations on yield of F. benjamina leaves is shown as % extraction in . The highest yield of F. benjamina leaves extract (17.73 ± 0.32%) was obtained by using 60% ethanol, while the lowest level of extract yield (12.07 ± 0.78%) was procured with 100% ethanol. The yield of F. benjamina leaves extract using 40% and 80% ethanol amounted to be 14.60 ± 0.95% and 15.20 ± 0.53%, respectively. The highest yield achieved by using 60% ethanol differed significantly (P < 0.05) from that obtained by using 40, 80, and 100% ethanol. These results regarding % extract yield were comparable with those of Al‐Zuaidy et al. and Zhao et al.,[Citation24,Citation27] who reported that during the course of extraction from Melicope Lunu‐ankenda and Epimedium brevicornum by using aqueous ethanol in various proportions as extracting solvent, the % extract yield increased initially, reaching a maximum value with 60% ethanol and then declined with increase in ethanol concentration. In a previous study, Abdel-Hameed et al.[Citation28] had reported 18.12% extract yield from a Ficus sp. leaves, while Imran et al.[Citation22] had reported 23.34% yield of F. benjamina leaves extract by using methanol as extraction solvent. Slight variations in the percent extract yield may be attributed to difference in the extraction solvent and its composition along with other factors, including medicinal plant maturity, season, and agro-climatic conditions[Citation22]
DPPH scavenging activity
Free radicals are well recognized as major contributors towards numerous chronic diseases including diabetes mellitus, renal failures, and cancer along with many other degenerative complications because of their role in disruption of natural defense systems/mechanisms[Citation22] Antioxidants exhibit protective activity against oxidative damage and thus they play an imperative role in diminishing the risk of chronic diseases[Citation17,Citation29] Antioxidant-rich medicinal plants hold promise to potentiate antioxidant defense system with least side effects[Citation22]
Due to DPPH stability and its handling ease, DPPH radical scavenging assay has been widely employed by researchers for investigating antioxidant activity of natural antioxidants and medicinal plant extracts.[Citation17,Citation30] In the present study, DPPH assay was performed to determine the antiradical potential of extracts of F. benjamina leaves. The percentage DPPH scavenging activity, and IC50 of ethanolic F. benjamina leaf extracts (EFBE) and butylated hydroxyanisole (BHA) at different concentrations are shown in and , respectively.
Figure 2. % DPPH scavenging by ethanolic Ficus benjamina leaf extracts (EFBE) and Butylated hydroxyanisole (BHA) at different concentrations.
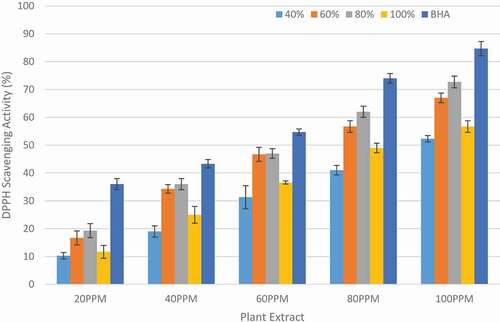
Figure 3. IC50 (μg/mL) for DPPH scavenging by ethanolic Ficus benjamina leaf extracts (EFBE) and Butylated hydroxyanisole (BHA). Different letters describe significance difference (p < 0.05) between different groups.
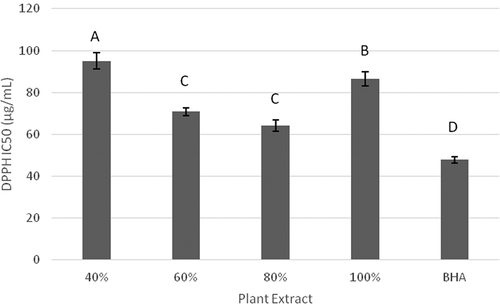
Among the different extracts studied, 80% ethanolic extract was found to exhibit highest antioxidant activity based upon its DPPH radical scavenging ability with IC50 value of 63.71 ± 2.66 µg/mL. It was found that the antioxidant activity of 80% ethanolic extract was comparable and was non-significantly different (P > 0.05) from 60% ethanolic extract with IC50 value of 69.32 ± 1.75 µg/mL; however, it differed significantly (P < 0.05) from 40% and 100% and BHA with IC50 values 96.33 ± 3.99 µg/mL, 85.52 ± 3.29 µg/mL and 46.24 ± 1.54 µg/mL, respectively. Previously, Imran et al.[Citation22] also performed DPPH radical scavenging assay of methanolic, n-butanol, chloroform, ethyl acetate and hexane extracts of F. benjamina leaves and reported IC50 values of 49.86 ± 3.39, 147.46 ± 3.85, 103.96 ± 2.06, 223.00 ± 2.68, and 436.21 ± 7.27 µg/mL, respectively. The slight variation in the DPPH radical scavenging activity might be due to the different extracting solvents used. The results demonstrated that extracts of F. benjamina leaves exhibited potent DPPH radical scavenging activity and thus making them a good natural source of antioxidants.
Alpha-glucosidase and alpha-amylase inhibition activity
The use of antioxidant-rich medicinal plants that can reduce oxidative stress and postprandial hyperglycemia may be considered as one of the most promising approach for the management of diabetics.[Citation17,Citation31] The carbohydrates hydrolyzing enzymes (α-glucosidase and α-amylase) have been documented as therapeutic targets towards the modulation of postprandial hyperglycemia.[Citation8,Citation32,Citation33] Alpha-glucosidase and α-amylase normally hydrolyze dietary carbohydrates which are the main source of blood glucose. Inhibition of these carbohydrates hydrolyzing enzymes therefore can be an important treatment option for diabetes mellitus.[Citation34] The medicinal plant extracts with efficient inhibitory properties towards α-glucosidase and α-amylase enzymes could therefore be highly useful for the treatment of diabetes by retarding uptake of dietary carbohydrates, delaying glucose absorption and suppression of postprandial hyperglycemia.[Citation8,Citation35]
In the present study, inhibitory effect of different ethanolic extracts of F. benjamina towards α-glucosidase and α-amylase enzymes was investigated. The α-glucosidase and α-amylase inhibition activities of ethanolic F. benjamina extracts are shown in and . The results revealed that extracts of F. benjamina leaves exhibit potent α-glucosidase and α-amylase inhibition activity. The IC50 values of different ethanolic F. benjamina extracts (EFBE) towards α-glucosidase and α-amylase inhibitions are presented in . The 80% ethanolic extract of F. benjamina leaves was depicted to be the most efficient α-glucosidase inhibitor with IC50 value of 9.65 ± 1.04 µg/mL. The lowest α-glucosidase inhibition activity was shown by 40% ethanolic F. benjamina extract with IC50 value of 22.02 ± 0.89 µg/mL, whereas the IC50 values for 60% and 100% ethanolic extracts were 13.19 ± 1.14 µg/mL and 18.21 ± 1.35 µg/mL, respectively. The comparative descending order of α-glucosidase inhibition of the extracts were revealed to be: EFBE 80% > EFBE 60% > EFBE 100% > EFBE 40%.
Table 1. IC50 (µg/mL) values of different ethanolic Ficus benjamina leaf extracts (EFBE) towards α-glucosidase and α-amylase inhibitions.
Figure 4. (A) Percent α-glucosidase inhibition activity (B) Percent α-amylase inhibition activity of ethanolic Ficus benjamina leaf extracts (EFBE) at different concentrations.
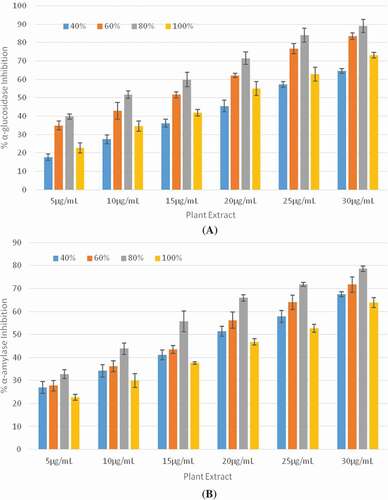
Interestingly, the ethanolic extracts F. benjamina leaves demonstrated higher α-glucosidase inhibition activity than the standard α-glucosidase inhibitors, i.e., acarbose (IC50 = 116.01 ± 3.83 µg/mL). The 80% ethanolic extract of F. benjamina leaves also showed highest inhibition activity toward α-amylase enzyme with IC50 value of 13.08 ± 1.06 μg/mL; however, the IC50 values for 40, 60, and 100% ethanolic extracts amounted to be 19.62 ± 1.31 µg/mL, 15.85 ± 1.49 µg/mL, and 25.15 ± 4.35 µg/mL, respectively. The descending order of α-amylase inhibition activity of the extracts was EFBE 80% > EFBE 60% > EFBE 40% > EFBE 100%. The α-amylase inhibition activity demonstrated by extracts of F. benjamina leaves were also higher than those demonstrated by acarbose (IC50 = 152.66 ± 7.32 µg/mL).
Previously, numerous medicinal plants have been reported by researchers having higher α-glucosidase and α-amylase inhibition activity compared to the standard synthetic inhibitors/drugs. Zhang et al.[Citation36] investigated α-glucosidase inhibitory potential of various ethanolic extracts of propolis (EEP) and reported higher α-glucosidase inhibition activity of 25% EEP (IC50 = 11.32 ± 2.23 µg/mL), 50% EEP (IC50 = 12.90 ± 1.14 µg/mL), 75% EEP (IC50 = 7.24 ± 1.16 µg/mL), 95% EEP (IC50 = 16.26 ± 0.43 µg/mL) and 100% EEP (IC50 = 20.01 ± 1.54 µg/mL) compared to the α-glucosidase inhibition activity of acarbose (IC50 = 177.47 ± 6.28 µg/mL). Poongunran et al.[Citation37] evaluated inhibitory effect of Ficus racemosa stem bark and Pterocarpus marsupium latex towards α-glucosidase and α-amylase enzymes and discovered that α-glucosidase inhibition potential of F. racemosa stem bark (IC50 = 19.88 µg/mL) and Pterocarpus marsupium latex (IC50 = 0.54 µg/mL) was better than acarbose (IC50 = 208.53 µg/mL); also, the α-amylase inhibition activity of F. racemosa stem bark (IC50 = 19.73 µg/mL) and Pterocarpus marsupium latex (IC50 = 2.97 µg/mL) were higher as compared to the acarbose (IC50 = 262.54 µg/mL). Elya et al.[Citation38] also reported higher α-glucosidase inhibition activity of Syzygium polyanthum (IC50 = 19.06 µg/mL) than that of acarbose, whereas Jyothi et al. [Citation39] reported higher α-amylase inhibition activity of Rosa damascene (IC50 = 70.33 + 0.14 μg/mL) as compared to the acarbose. In another study, Gao et al.[Citation40] investigated inhibitory properties of green tea extract and green tea polyphenols against α-glucosidase and α-amylase enzymes and reported higher α-glucosidase activity of green tea (IC50 = 4.421 ± 0.018 µg/mL) and green tea polyphenols (IC50 = 10.019 ± 0.017 µg/mL) when compared with that of acarbose (IC50 = 4822.783 ± 2 6.04 µg/mL). Similarly, green tea polyphenols showed higher inhibitory effect against α-amylase enzyme (IC50 = 1,370.812 ± 59.081 µg/mL) compared to the α-amylase inhibition activity of acarbose (IC50 = 2715.654 ± 24.709 µg/mL).
There is sufficient available literature which shows that the medicinal plant extracts demonstrate better α-glucosidase and α-amylase inhibition activities comparative to the standard drugs/inhibitors. In the present study, ethanolic extract of F. benjamina leaves has also been ascertained to exhibit potent α-glucosidase and α-amylase inhibition activities, hence can decrease blood glucose level and suppress postprandial hyperglycemia. These excellent inhibitory properties of extracts of F. benjamina leaves may be attributed to the presence of biologically functional molecules.
1H-NMR and uhplc-qtof-ms/ms-based metabolite profiling
The bioactive constituents in the ethanolic F. benjamina extract were identified by using 1H-NMR in combination with J-resolved spectra and UHPLC-QTOF-MS/MS. Primary metabolites were identified by comparing their 1H-NMR peaks (chemical shifts) with those described in Chenomx database. A typical 1H-NMR spectrum of F. benjamina leaf extract is shown in . The signals of metabolites in the aliphatic region (δ 0.5–3.0) belong to amino acids and organic acids. Whereas, sugars appeared in carbohydrates region ranging from δ 3.0–5.5 in spectrum. The region (δ 5.5–9.0) was characterized as aromatic corresponds to secondary metabolites including phenolics and flavonoids.
In case of UHPLC-QTOF-MS/MS, the metabolites were identified on the basis of accurate masses, molecular ion peaks, and fragmentation patterns in addition to comparison with ACD/Labs library and previously reported relevant data in the literature. UHPLC-QTOF-MS/MS chromatogram of F. benjamina extract is shown in .
Numerous constituents as shown in were detected in F. benjamina extract by using either 1H NMR or UHPLC-QTOF-MS/MS including quercetin, quercetin 3-O-α-rhamnoside, quercetin 3-O-β-glucoside, quercetin-rahmnosylgalactoside, rutin, catechin, catechin trimer, epicatechin, (epi)catechin-(epi)catechin-(epi)-catechin, (epi)afzelechin-(epi)catechin, kampferol, morin, naringenin, apigenin-6,8-di-C-β-D-glucopyranoside (Vicenin II), 6-C-glucosyl-8-C-arabinosyl apigenin, apigenin 6-C-glucoside, gallic acid, caffic acid, chlorogenic acid, quinic acid, 3-O-Caffeoylquinic acid, dicaffeoquinic acid, procyanidin B1, 2ʹ’-O-Rhamnose-C-Hexoside, α-glucose, β-glucose, sucrose, choline, leucine, alanine and pyruvate. Characteristic chemical shift values along with J resolved and molecular ion peaks along with mass fragmentation patterns of identified metabolites were obtained by 1H NMR, and UHPLC-QTOF-MS/MS analysis, respectively, and are presented in .
Table 2. Metabolites identified in 80% ethanolic leaf extract of Ficus benjamina.
Quercetin is a major dietary flavonol present in medicinal plants. Within the flavonoid family, it exhibits potent anti-oxidant activity and is known to impart positive impact on glucose metabolism. It has the ability to reduce intestinal absorption of glucose[Citation41,Citation42] Quercetin from Calendula officinalis L. leaves significantly inhibited the activity of α-amylase.[Citation43] Rutin is also a well-known flavonoid to exhibit anti-diabetic effects. Flavonoids from Morus atropurpurea leaves have been investigated for their ability to inhibit carbohydrate hydrolyzing enzyme by Hong et al.,[Citation44] and it was revealed that out of all the tested flavonoids, such as rutin, kaempferol-3-O-rutinoside, isoquercetin, and astragalin, rutin showed highest α-glucosidase inhibitory potential (IC50 = 13.19 ± 1.10 µM). Catechins isolated from grape seeds have also been reported to be good inhibitors of carbohydrate hydrolyzing enzymes. [Citation45] Similarly, epicatechin and its isomers isolated from Rhodiola crenulata roots have been reported to exhibit significant α-glucosidase inhibitory activity.[Citation46]
Habtemariam et al.[Citation47] reported kampferol to be a potent inhibitor of carbohydrates hydrolyzing enzyme. In another study, morin had shown to exert significant inhibitory effect on α-glucosidase (IC50 = 4.48 ± 0.04 μM)..[Citation48] Potent inhibitory activity of naringenin (flavanone) against α-glucosidase enzyme has been reported by Priscilla et al.[Citation49] Apigenin and apigenin-glucopyranoside isolated from Chrozophora plicata have also been reported to impart significant inhibitory effect on α-glucosidase activity.[Citation50] Gallic acid is an important constituent of numerous medicinal plants and has shown strong inhibitory properties against carbohydrates hydrolyzing enzymes. In a previous study, better inhibitory activity of α-glucosidase of gallic acid (IC50 = 24.3 μM) has been reported compared to the acarbose (IC50 = 59.5 μmol/L).[Citation51,Citation52] Potent α-glucosidase and α-amylase inhibition activities of caffeic acid and chlorogenic acid have been reported by Oboh et al.[Citation12] Dose-dependent inhibition of α-amylase and α-glucosidase was revealed by both of these phenolic acids. However, caffeic acid showed significantly higher inhibitory activity towards α-glucosidase (IC50 = 4.98 μg/mL) and α-amylase (IC50 = 3.68 μg/mL) as compared to chlorogenic acid (α-glucosidase IC50 = 9.24 μg/mL and α-amylase (IC50 = 9.10 μg/mL). Furthermore, quinic acid and its derivatives from Pluchea indica leaves were also found to be potent inhibitors of carbohydrate hydrolyzing enzymes.[Citation53]
Significant carbohydrates hydrolyzing enzymes inhibition properties of all the above discussed metabolites prove them to be promising therapeutic candidates for the management of type 2 diabetes. The present study revealed that extract of F. benjamina leaves is enriched with a wide range of natural bioactive constituents, and therefore the underlying potent antioxidant and α-glucosidase and α-amylase inhibitory properties of extract of F. benjamina leaves might be attributed to the presence of these metabolites and their synergistic effects.
Conclusion
Results of the present study ascertained the potent antioxidant and inhibitory potential of leaf extracts of F. benjamina against α-glucosidase and α-amylase enzymes. The 80% ethanolic leaf extract of F. benjamina proved as the best inhibitor of carbohydrate hydrolyzing enzyme. Presence of numerous valuable bioactive metabolites in 80% ethanolic extract further supported the findings and these metabolites may also have some synergistic interaction which could also be responsible for the observed potent anti-diabetic effect of extract. These results, therefore, provide significant scientific evidence concerning the prodigious use of F. benjamina leaves towards the development of medicinal preparations, functional foods or nutraceuticals for the management of diabetes and related medical conditions.
References
- Petchi, R.; Vijaya, C.; Parasuraman, S. Antidiabetic Activity of Polyherbal Formulation in Streptozotocin–Nicotinamide Induced Diabetic Wistar Rats. Journal of Traditional and Complementary Medicine. 2014, 4, 108–117. C-1: Check all??
- Parasuraman, S.; Kumar, E.; Kumar, A.; Emerson, S. Free Radical Scavenging Property and Diuretic Effect of Triglize, a Polyherbal Formulation in Experimental Models. Journal of Pharmacology & Pharmacotherapeutics 2010, 1(1), 38–41. DOI: 10.4103/0976-500X.64535.
- Adeghate, E.; Schattner, P.; Dunn, E. An Update on the Etiology and Epidemiology of Diabetes Mellitus. Annals of the New York Academy of Sciences 2006, 1084, 1–29. DOI: 10.1196/annals.1372.029.
- Olokoba, A.; Obateru, O. A.; Olokoba, L. B. Type 2 Diabetes Mellitus: A Review of Current Trends. Oman Medical Journal 2012, 27, 269. DOI: 10.5001/omj.2012.03.
- Jacobsen, I.; Henriksen, J. E.; Beck‐Nielsen, H. The Effect of Metformin in Overweight Patients with Type 1 Diabetes and Poor Metabolic Control. Basic & Clinical Pharmacology & Toxicology 2009, 105, 145–149. DOI: 10.1111/j.1742-7843.2009.00380.x.
- Asche, C.; McAdam‐Marx, C.; Shane‐McWhorter, L.; Sheng, X.; Plauschinat, C. Association between Oral Antidiabetic Use, Adverse Events and Outcomes in Patients with Type 2 Diabetes. Diabetes, Obesity and Metabolism 2008, 10, 638–645. DOI: 10.1111/dom.2008.10.issue-8.
- Mohammed, A.; Kumar, D.; Rizvi, S. I. Antidiabetic Potential of Some Less Commonly Used Plants in Traditional Medicinal Systems of India and Nigeria. Journal of Intercultural Ethnopharmacology 2015, 4, 78. DOI: 10.5455/jice.20141128032845.
- Thilagam, E.; Parimaladevi, B.; Kumarappan, C.; Mandal, S. C. α-Glucosidase and α-amylase Inhibitory Activity of Senna Surattensis. Journal of Acupuncture and Meridian Studies 2013, 6, 24–30. DOI: 10.1016/j.jams.2012.10.005.
- Wang, H.; Du, Y.-J.; Song, H.-C. α-Glucosidase and α-amylase Inhibitory Activities of Guava Leaves. Food Chemistry 2010, 123, 6–13. DOI: 10.1016/j.foodchem.2010.03.088.
- Bhandari, M.; Jong-Anurakkun, N.; Hong, G.; Kawabata, J. α-Glucosidase and α-amylase Inhibitory Activities of Nepalese Medicinal Herb Pakhanbhed (Bergenia Ciliata, Haw.). Food Chemistry 2008, 106, 247–252. DOI: 10.1016/j.foodchem.2007.05.077.
- Kumar, D.; Gupta, N.; Ghosh, R.; Gaonkar, R. H.; Pal, B. C. α-Glucosidase and α-amylase Inhibitory Constituent of Carex Baccans: Bio-Assay Guided Isolation and Quantification by Validated RP-HPLC–DAD. Journal of Functional Foods 2013, 5, 211–218. DOI: 10.1016/j.jff.2012.10.007.
- Oboh, G.; Agunloye, O. M.; Adefegha, S. A.; Akinyemi, A. J.; Ademiluyi, A. O. Caffeic and Chlorogenic Acids Inhibit Key Enzymes Linked to Type 2 Diabetes (In Vitro): A Comparative Study. Journal of Basic and Clinical Physiology and Pharmacology 2015, 26, 165–170. DOI: 10.1515/jbcpp-2013-0141.
- Yin, Z.; Zhang, W.; Feng, F.; Zhang, Y.; Kang, W. α-Glucosidase Inhibitors Isolated from Medicinal Plants. Food Science and Human Wellness 2014, 3, 136–174. DOI: 10.1016/j.fshw.2014.11.003.
- Telagari, M.; Hullatti, K. In-Vitro α-amylase and α-glucosidase Inhibitory Activity of Adiantum Caudatum Linn. And Celosia Argentea Linn. Extracts and Fractions. Indian Journal of Pharmacology 2015, 47, 425. DOI: 10.4103/0253-7613.161270.
- Kim, K.; Rioux, L. E.; Turgeon, S. L. Alpha-Amylase and Alpha-Glucosidase Inhibition Is Differentially Modulated by Fucoidan Obtained from Fucus Vesiculosus and Ascophyllum Nodosum. Phytochemistry 2014, 98, 27–33. DOI: 10.1016/j.phytochem.2013.12.003.
- Kazeem, M.; Adamson, J.; Ogunwande, I. Modes of Inhibition of α-amylase and α-glucosidase by Aqueous Extract of Morinda Lucida Benth Leaf. BioMed Research International. 2013, 1–6. DOI: 10.1155/2013/527570.
- Yao, X.; Zhu, L.; Chen, Y.; Tian, J.; Wang, Y. In Vivo and in Vitro Antioxidant Activity and α-glucosidase, α-amylase Inhibitory Effects of Flavonoids from Cichorium Glandulosum Seeds. Food Chemistry 2013, 139, 59–66. DOI: 10.1016/j.foodchem.2012.12.045.
- Na, D.; Ji, H. Y.; Park, E. J.; Kim, M. S.; Liu, K.-H.; Lee, H. S. Evaluation of Metabolism-Mediated Herb-Drug Interactions. Archives of Pharmacal Research 2011, 34, 1829–1842. DOI: 10.1007/s12272-011-1105-0.
- Osemene, K.; Elujoba, A.; Ilori, M. An Overview of Herbal Medicine Research and Development in Nigeria. Research Journal of Medical Sciences 2011, 5, 228–232. DOI: 10.3923/rjmsci.2011.228.232.
- Patwardhan, B.; Vaidya, A. D. Natural Products Drug Discovery: Accelerating the Clinical Candidate Development Using Reverse Pharmacology Approaches. Indian Journal of Experimental Biology 2010, 48, 220–227.
- Abdelkader, A.; Aldughaish, A. M. Physiological and Chemical Characteristics of Age-Differed Ficus Benjamina L. Trees Cultivated in El-Ahassa, Saudi Arabia. Journal of Plant Sciences 2016, 4, 63–67.
- Imran, M.; Rasool, N.; Rizwan, K.; Zubair, M.; Riaz, M.; Zia-Ul-Haq, M.; Rana, U. A.; Nafady, A.; Jaafar, H. Z. Chemical Composition and Biological Studies of Ficus Benjamina. Chemistry Central Journal 2014, 8, 12. DOI: 10.1186/1752-153X-8-19.
- Sirisha, N.; Sreenivasulu, M.; Sangeeta, K.; Chetty, C. M. Antioxidant Properties of Ficus Species–A Review. International Journal of PharmTech Research 2010, 2, 2174–2182.
- AL‐Zuaidy, M.; Hamid, A. A.; Ismail, A.; Mohamed, S.; Razis, A.; Faizal, A.; Mumtaz, M. W.; Salleh, S. Z. Potent Antidiabetic Activity and Metabolite Profiling of Melicope Lunu‐Ankenda Leaves. Journal of Food Science 2016, 81, 1080–1090. DOI: 10.1111/1750-3841.13293.
- Brand-Williams, W.; Cuvelier, M. E.; Berset, C. Use of a Free Radical Method to Evaluate Antioxidant Activity. LWT-Food Science and Technology 1995, 28, 25–30. DOI: 10.1016/S0023-6438(95)80008-5.
- Jabeen, B.; Riaz, N.; Saleem, M.; Naveed, M. A.; Ashraf, M.; Alam, U.; Rafiq, H. M.; Tareen, R. B.; Jabbar, A. Isolation of Natural Compounds from Phlomis Stewartii Showing α-glucosidase Inhibitory Activity. Phytochemistry 2013, 96, 443–448. DOI: 10.1016/j.phytochem.2013.09.015.
- Zhao, Y.; Hou, Y.; Tang, G.; Cai, E.; Liu, S.; Yang, H.; Zhang, L.; Wang, S. Optimization of Ultrasonic Extraction of Phenolic Compounds from Epimedium Brevicornum Maxim Using Response Surface Methodology and Evaluation of Its Antioxidant Activities in Vitro. Journal of Analytical Methods in Chemistry 2014, 2014, 1–7.
- Abdel-Hameed, E.;. Total Phenolic Contents and Free Radical Scavenging Activity of Certain Egyptian Ficus Species Leaf Samples. Food Chemistry 2009, 114, 1271–1277. DOI: 10.1016/j.foodchem.2008.11.005.
- Liu, R.; Adom, K. K. Antioxidant Activity of Grains. Journal of Agricultural and Food Chemistry 2002, 50, 6182–6187.
- Danino, O.; Gottlieb, H. E.; Grossman, S.; Bergman, M. Antioxidant Activity of 1, 3-Dicaffeoylquinic Acid Isolated from Inula Viscosa. Food Research International 2009, 42, 1273–1280. DOI: 10.1016/j.foodres.2009.03.023.
- James, O.; David, S. H. Postprandial Hyperglycemia/Hyperlipidemia (Postprandial Dysmetabolism) Is a Cardiovascular Risk Factor. American Journal of Cardiology 2007, 100, 899–904. DOI: 10.1016/j.amjcard.2007.03.107.
- Kim, M.; Jeong, Y. K.; Wang, M. H.; Lee, W. Y.; Rhee, H. I. Inhibitory Effect of Pine Extract on α-glucosidase Activity and Postprandial Hyperglycemia. Nutrition 2005, 21, 756–761. DOI: 10.1016/j.nut.2004.10.014.
- Leroux-Stewart, J.; Rabasa-Lhoret, R.; Chiasson, J. L. Alpha-Glucosidase Inhibitors. In International Textbook of Diabetes Mellitus, 4th ed.; Defronzo, R. A., Ferrannini, E., Keen, H., Zimmet, P., Alberti, G., Eds.; Wiley-Blackwell: Chichester, 2004; PP 673-85.
- Krentz, A.; Bailey, C. Oral Diabetic Agents Current Role in Type 2 Diabetes Melitus. Riview Article 2005, 65, 394.
- Watanabe, J.; Kawabata, J.; Kurihara, H.; Niki, R. Isolation and identification of α-glucosidase inhibitors from tochu-cha (Eucommia ulmoides). Bioscience, Biotechnology, and Biochemistry 1997, 61, 177–178.
- Zhang, H.; Wang, G.; Dong, J. Inhibitory Properties of Aqueous Ethanol Extracts of Propolis on Alpha-Glucosidase. Evidence-Based Complementary and Alternative Medicine 2015, 1–7.
- Poongunran, J.; Perera, H.; Fernando, W.; Jayasinghe, L.; Sivakanesan, R. alpha-Glucosidase and alpha-Amylase Inhibitory Activities of Nine Sri Lankan Antidiabetic Plants. British Journal of Pharmaceutical Research 2015, 7, 365–374. DOI: 10.9734/BJPR/2015/18645.
- Elya, B.; Handayani, R.; Sauriasari, R.; Hasyyati, U. S.; Permana, I. T.; Permatasari, Y. I. Antidiabetic Activity and Phytochemical Screening of Extracts from Indonesian Plants by Inhibition of Alpha Amylase, Alpha Glucosidase and Dipeptidyl Peptidase IV. Pakistan Journal of Biological Sciences 2015, 18, 279. DOI: 10.3923/pjbs.2015.279.284.
- Jyothi, K.; Hemalatha, P.; Avanthi, A.; Challa, S. A Comparative Analysis on the Alpha Amylase Inhibitory Potential of Six Ornamental Medicinal Plants. Journal of Natural Product and Plant Resources 2013, 3, 1–6.
- Gao, J.; Xu, P.; Wang, Y.; Wang, Y.; Hochstetter, D. Combined Effects of Green Tea Extracts, Green Tea Polyphenols or Epigallocatechin Gallate with Acarbose on Inhibition against α-amylase and α-glucosidase in Vitro. Molecules 2013, 18, 11614–11623. DOI: 10.3390/molecules180911614.
- Eid, H.; Nachar, A.; Thong, F.; Sweeney, G.; Haddad, P. S. The Molecular Basis of the Antidiabetic Action of Quercetin in Cultured Skeletal Muscle Cells and Hepatocytes. Pharmacognosy Magazine 2015, 11, 74. DOI: 10.4103/0973-1296.168985.
- Manzano, S.; Williamson, G. Polyphenols and Phenolic Acids from Strawberry and Apple Decrease Glucose Uptake and Transport by Human Intestinal Caco‐2 Cells. Molecular Nutrition & Food Research 2010, 54, 1773–1780. DOI: 10.1002/mnfr.201000019.
- Olennikov, D.; Kashchenko, N. I. Componential Profile and Amylase Inhibiting Activity of Phenolic Compounds from Calendula Officinalis L. Leaves. The Scientific World Journal 2014, 654193.
- Hong, C.; Li, S. L.; Zhang, X. Q.; Ye, W. C.; Zhang, Q. W. Flavonoids with α-glucosidase Inhibitory Activities and Their Contents in the Leaves of Morus Atropurpurea. Chinese Medicine 2013, 8, 19. DOI: 10.1186/1749-8546-8-19.
- Yilmazer-Musa, M.; Griffith, A. M.; Michels, A. J.; Schneider, E.; Frei, B. Grape Seed and Tea Extracts and Catechin 3-Gallates are Potent Inhibitors of α-amylase and α-glucosidase Activity. Journal of Agricultural and Food Chemistry 2012, 60, 8924–8929. DOI: 10.1021/jf301147n.
- Chu, H.; Wu, S. H.; Hsieh, J. F. Isolation and characterization of α-glucosidase inhibitory constituents from Rhodiola crenulata. Food Research International 2014, 57, 8–14. DOI: 10.1016/j.foodres.2014.01.029.
- Habtemariam, S.;. A-Glucosidase Inhibitory Activity of kaempferol-3-O-rutinoside. Natural Product Communications 2011, 6, 201–203.
- Zeng, L.; Zhang, G.; Liao, Y.; Gong, D. Inhibitory Mechanism of Morin on α-glucosidase and Its Anti-Glycation Properties. Food & Function 2016, 7, 3953–3963. DOI: 10.1039/C6FO00680A.
- Priscilla, D.; Roy, D.; Suresh, A.; Kumar, V.; Thirumurugan, K. Naringenin Inhibits α-glucosidase Activity: A Promising Strategy for the Regulation of Postprandial Hyperglycemia in High Fat Diet Fed Streptozotocin Induced Diabetic Rats. Chemico-Biological Interactions 2014, 210, 77–85. DOI: 10.1016/j.cbi.2013.12.014.
- Tabussum, A.; Riaz, N.; Saleem, M.; Ashraf, M.; Ahmad, M.; Alam, U.; Jabeen, B.; Malik, A.; Jabbar, A. α-Glucosidase inhibitory constituents from Chrozophora plicata. Phytochemistry Letters 2013, 6, 614–619. DOI: 10.1016/j.phytol.2013.08.005.
- Zhao, J.; Zhou, X. W.; Chen, X. B.; Wang, Q. X. α-Glucosidase inhibitory constituents from Toona sinensis. Chemistry of Natural Compounds 2009, 45, 244–246. DOI: 10.1007/s10600-009-9289-y.
- Wansi, J.; Lallemand, M.-C.; Chiozem, D. D.; Toze, F. A. A.; Mbaze, L. M. A.; Naharkhan, S.; Iqbal, M. C.; Tillequin, F.; Wandji, J.; Fomum, Z. T. α-Glucosidase Inhibitory Constituents from Stem Bark of Terminalia Superba (Combretaceae). Phytochemistry 2007, 68, 2096–2100. DOI: 10.1016/j.phytochem.2007.02.020.
- Arsiningtyas, I.; Gunawan-Puteri, M. D.; Kato, E.; Kawabata, J. Identification of α-glucosidase Inhibitors from the Leaves of Pluchea Indica (L.) Less., A Traditional Indonesian Herb: Promotion of Natural Product Use. Natural Product Research 2014, 28, 1350–1353. DOI: 10.1080/14786419.2014.904306.