ABSTRACT
β-Glucan is an important chemical found in cereals, tremendously beneficial to human health. In this study, β-glucan contents in wild and cultivated barley from representative regions worldwide were investigated. Results exhibited that coefficient of variation of β-glucan content of wild and cultivated barley was 24.18% and 13.99%, respectively. The β-glucan contents of studied wild barley accessions were ranged from 3.26% to 7.67% while cultivated barley varieties were ranged from 2.68% to 4.74%. A significant difference of β-glucan content (p ≤ 0.001) was found between wild and cultivated barely. Wild barley showed a higher β-glucan content and variation than cultivated barley. This study gives an idea about elite germplasms for genetic improvement and shed light to trace barley domestication in relation to grain metabolite view.
Introduction
Barley (Hordeum vulgare L.) is the fourth largest cereal crop produced worldwide, and it is utilized in food, feed, and malt production.[Citation1] Also, being the most extensively adapted cereal grain species, which can be cultivated from fertile to desert lands, and it is the staple food source for some nations in Himalayan, Morocco, and Ethiopia.[Citation2] Wild barley (Hordeum spontaneum) is the progenitor of cultivated barley, which offers considerable potential as a genetic resource for barley improvement. Naturally occurring wild barley populations are distributed primarily across the Fertile Crescent, Central Asia, and Tibet.[Citation3–Citation6]
When utilizing barley for human food, appearance and flavour are the limiting factors. However, recent researches proved that the interest in barley is surging in the last decades, mainly due to its high content of soluble dietary fiber, especially (1,3;1,4)-β-D-glucan (hereafter referred as β-glucan).[Citation7,Citation8] β-Glucan is a linear molecule of partially water-soluble polysaccharides, consisting of glucose[Citation9] linked by both β-(1→3) and β-(1→4)-linkages,[Citation10] and it is a major component of endosperm cell walls and sub-aleurone layer of Gramineae.[Citation11,Citation12] Compared with other cereals, barley and oats contain relatively higher β-glucan contents.[Citation9] However, higher β-glucan content in barley can be either advantageous or disadvantageous, depending on the industry which barley used. Low β-glucan content is required for malting and brewing industries, while high level is desirable for applications in human health and nutrition.[Citation13]
A positive relationship between cereal consumption with the recommended β-glucan content and reduction of the risk of chronic health problems had been proved in a number of health studies. β-Glucan reduces hypertension and diabetes due to regulation of blood cholesterol and glucose levels.[Citation14] It reduces the chance of coronary heart disease and ischemic heart disease.[Citation15] Also, β-glucan has immune modulating properties.[Citation16] The main feature of β-glucan which renders it benefits is that it can increase intraluminal viscosity by forming highly viscous solutions.[Citation17] Other positive roles of β-glucan include increasing mineral and vitamin bioavailability, and play an important role in gut physiology and reducing the risk of colon cancer.[Citation18–Citation20]
β-Glucan plays an important role as a fat substitute in processed foods, particularly in the production of cheese and ice-cream. As a gel-forming (non-caloric thickening and stabilizing) component, β-glucans can be used for the elaboration of products with high dietary fiber.[Citation21] Food and supplement industries pay increasing attention on this bioactive grain component, and the trend is to incorporate β-glucan as an ingredient in commercial-level product formulations.[Citation22]
Although a high β-glucan content is important in the food industry, it may create problems such as reduced rate of wort filtration and haze formation in beer, when used for brewing, and adversely affect the recovery of malt extract.[Citation23,Citation24] The high viscosity of aqueous solutions of barley β-glucan has also been implicated with problems encountered in the stock feed industry. Therefore, it is considered as an anti-nutritional factor, mainly in poultry feed, which may give sticky droppings and affect nutritional intake, growth rate, and feed conversion efficiency.[Citation25,Citation26]
There are several methods to determine β-glucan content in barley. The three main methods are enzymatic method, calcofluor method, and colorimetric method. The most widely used method in research laboratories is the enzymatic method,[Citation27] which is also called as Approved Method 32–23 by AACC International 2000.[Citation28] Enzymatic method is the mixed-linkage method, where linear β-glucan polymers are extracted from grain flour, hydrolyzed into monosaccharides by sequential enzymatic reactions using endo-(1→3,1→4)-β-D-glucan 4-glucanohydrolase (lichenase), followed by β-glucosidase, and finally coupled to generate color with glucose oxidase and a chromogenic substrate.[Citation28]
The impact of genetic and environmental variations, for the β-glucan content in barley, has been studied, and the genetic factors have been found to be the most important determinant of β-glucan content in barley.[Citation29–Citation31] The variation is not only caused by genetics and environment but can be a result of the evaluation methodology. The earlier analytical approaches used to measure β-glucan gave excessive values, while the enzymatic method,[Citation24] which use a specific assay kit, has proved to be a simple procedure and gives good repeatability.[Citation31]
It is clear that the knowledge of β-glucan levels in barley is a valuable attribute for consumers, and it will create an opportunity for scientists and breeders to develop new cultivars, such as nutraceutical-enriched barley. The objectives of this study were to determine and compare the β-glucan contents of wild and cultivated barley, from different locations of the world, for the purpose of evaluation of its difference and variation. It is expected that these findings will help to identify the suitable barley genotypes and their locations to be used in breeding programs, to obtain barley with required β-glucan contents, required for the specific purpose, such as human food, livestock feed, malt, and brewing industries.
Materials and methods
Plant materials
Sixty barley seed samples including 32 wild barley accessions from Fertile Crescent (Mediterranean), Central Asian, and Tibet regions, and 28 cultivated barley varieties from different locations of the world were used for the study. All the seed samples were stored in seed stores of State Key Laboratory, Northwest Agriculture and Forest University, China, until processing for β-glucan content.
Sample preparation
Barley grains were ground in a centrifugal mill and passed through a 0.5 mm sieve to obtain a consistent fine powder, after drying in an oven for 24 h. The mill was cleaned between samples.
Chemical analysis
The β-glucan content was determined enzymatically by using the commercially available kit (Megazyme International Ireland Ltd., Bray, Ireland), according to the Megazyme β-glucan assay.[Citation24,Citation27] This method has been accepted by the AOAC international (Method 995.16) and the AACC international (Method 32-23).[Citation32,Citation33] Then, the β-glucan contents were calculated precisely according to the guidelines of the Megazyme commercial kit. For each sample, two biological replicates were performed, and means were used for downstream analysis.
In brief, complying with the streamlined procedure of Megazyme, 80–120 mg of milled flour was mixed with 200 µl of 50% (v/v) ethanol and 4 ml sodium phosphate buffer (20 mM, pH 6.5). This mixture was incubated with lichenase enzyme (200 µl, 50 U/ml). Then the enzymatic reaction was stopped with 5 ml sodium acetate buffer (200 mM, pH 4.0). After centrifugation, 100 µl aliquot was reacted to completion with 100 µl β-glucosidase enzyme and the amount of glucose was detected with 3 ml glucose oxidase/peroxidase developing reagent by measuring absorbance at 510 nm. The values of the β-glucan levels were obtained for each sample as the mean ± standard deviation (SD). Then, the β-glucan content was calculated as a percentage.
Statistical analysis
above experimental data were used to perform the statistical analysis and determine the difference and variation of β-glucan content in wild and cultivated barley genotypes. Under the statistical analysis Shapiro–Wilk test, coefficient of variation (CV), analysis of variance (ANOVA), and construction of dendrogram were performed using SPSS 23.0 software, IBM, USA.
Results
β-Glucan content of selected accessions
In this study, wild barley samples were selected from Fertile Crescent, Central Asia, and Tibet, and cultivated barley samples from representative regions worldwide to determine β-glucan contents of wild and cultivated barley and their variations. illustrates selected wild and cultivated barley accessions, their location, average β-glucan content, and SD.
Table 1. β-Glucan content of wild and cultivated barley accessions.
Descriptive statistics and frequency distribution
The assumption of normality was tested for both populations for β-glucan using Shapiro–Wilk test for normality, and skewness and kurtosis statistics suggested that the β-glucan content is reasonably normally distributed in wild barley, but not in cultivated barley population, with skewness of 0.468 (standard error [SE] = 0.414) and kurtosis of −0.648 (SE = 0.809) in wild barley and skewness of 1.350 (SE = 0.441) and kurtosis of 3.854 (SE = 0.858) in cultivated barley. This is due to one exceptional variety in cultivated barley population called Sumire Mochi form Japan (box plot was used to identify this variety). After removing it from the cultivated population, using Shapiro–Wilk test for normality, and skewness and kurtosis statistics suggested that the β-glucan content is normally distributed in both populations, where with same skewness and kurtosis in wild barley and skewness of −0.009 (SE = 0.448) and kurtosis of −0.550 (SE = 0.872) in cultivated barley. Therefore, further statistical analysis was done by removing that accession, except in the dendrogram. The used acceptable range for skewness and kurtosis is from +1.96 to – 1.96 and the significant value for the Shapiro–Wilk test is 0.05. The mean β-glucan contents of wild and cultivated accessions were 5.17% (SD = 1.25, SE = 0.22) and 3.72% (SD = 0.52, SE = 0.10), respectively ().
Table 2. Descriptive statistics of β-glucan content of wild and cultivated barley accessions.
The β-glucan contents of 32 wild barley accessions were ranged from 3.26% for JOR_KMH_2 to 7.67% for TUR_IG39839 and approximately 60% of accessions belong to the range of 4.0–6.0%. β-Glucan content of 28 cultivated barley varieties from different locations of the world were ranged from 2.68% for BMC, a commercial cultivar from Israel, to 4.74% for Mitsukiko, a commercial cultivar from Japan, and approximately 90% of cultivars belong to the range of 3.0–5.0% (). The Japanese variety Sumire Mochi, which was considered as the exceptional accession of cultivated barley population, showed the highest value of 6.15% for the cultivated group. The wild and cultivated barley accessions differed widely in the CV %, where CV % of wild and cultivated barley are 24.18% and 13.99%, respectively, and wild barley showed higher CV % compared to cultivated barley (). Also, the box plot () graphically illustrates the variation of β-glucan content between wild and cultivated barley accessions.
A great variation of β-glucan contents in wild and cultivated barley was detected. According to the frequency distribution of β-glucan content in wild and cultivated barley accessions, majority of wild barley accessions were between the range of 4–5%, which represents 34.4% of the population, while majority of cultivated barley accessions were between the range of 3–4%, which represents 57.1% of the population. In cultivated barley, several accessions were categorized into <3% of β-glucan category, while in wild barley, no accession was found in that category. In contrast, several accessions of wild barley were categorized into >7% of β-glucan category, while in cultivated barley, no accession belongs to that category.
ANOVA of barley populations
ANOVA of β-glucan content of wild and cultivated barley, within the population, exhibited that the effects of varieties or accessions were highly significant (p ≤ 0.001). According to the mean β-glucan content of wild and cultivated barley, several clusters were identified (). Each cluster was considered as a group, and the accessions within the cluster were considered as a replicate of that group to perform ANOVA within the wild and cultivated populations. ANOVA of β-glucan content between wild and cultivated barley populations confirmed a higher significant difference (p ≤ 0.001), between two populations. Results of the F-test also indicated that there was a significant difference of β-glucan content between wild and cultivated barley, F > F critical, p < 0.001.
Cluster analysis
According to the dendrogram obtained from statistical analysis (), wild barley population was divided into five clusters and cultivated barley population was divided into four clusters, depending on the β-glucan contents. Based on the clusters of β-glucan, all wild and cultivated barley were divided into three main categories, as low, medium, and high. The low category comprises β-glucan content less than 3% and 3–4%, medium 4–5% and 5–6 %, and high 6–7% and above 7%. In wild barley accessions, 15.5% were grouped into low, 62.5% into medium, and 22.0% into high β-glucan categories, whereas in cultivated barley, 64.0% were grouped into low, 32.0% into medium, and 4.0% into high β-glucan categories. It demonstrated the variation in β-glucan content in wild and cultivated barley.
Wild barley accessions AFG_IG38660, TJK_IG131790, TUR_IG116112, and TUR_IG39839 were categorized into a single cluster, which showed the highest β-glucan content (above 7%). However, in cultivated barley, only one accession, named Sumire Mochi from Japan contained the highest β-glucan content (6–7%) and it was clustered separately. This Sumire Mochi variety is used as human food, and no accession was found to be above 7% cluster. In wild barley, the lowest β-glucan contained cluster was 3–4% cluster, which consisted of JOR_KFH_1, JOR_KFH_2, JOR_KMH_2, ISR_TBS_1, and ISR_TBS_2 accessions. Accordingly, in cultivated barley, the lowest cluster was <3% cluster – Golden Promise® from the United Kingdom and BMC from Israel – which are used for the malting purpose, whereas no <3% cluster was found in wild barley population.
Discussion
The improvement of grain quality of barley depends on the collection of desirable genetic variation. Wild barley is the undisputed progenitor of cultivated barley and offers considerable potential as a genetic resource for barley improvement. Naturally occurring populations are distributed primarily across the Fertile Crescent, Central Asia, and Tibet. Therefore, the potential use of the wild barley germplasm is to be exploited largely, especially from the places where barley originated. These regions are recognized as the original centers of cultivated barley, where higher genetic diversity was reported and wild progenitors are still colonizing in these regions.[Citation4–Citation6] The countries with significant territory within the Fertile Crescent include Iraq, Syria, Lebanon, Jordan, Israel, Palestine, Egypt, Turkey, and Iran, and Central Asia include Kazakhstan, Kyrgyzstan, Tajikistan, Turkmenistan, Uzbekistan, and Afghanistan. Therefore, wild barley samples for this study were selected from these regions to determine β-glucan content and to evaluate the variation of β-glucan content between wild and cultivated barley.
Genotypic and environmental variations in β-glucan content of cultivated barley have been studied extensively for several decades, while studies on wild barley are comparatively very low. This study presents a direct analysis and comparison of β-glucan content in wild and cultivated barley. The β-glucan content was determined by the mixed-linkage β-glucan assay procedure, by using the commercially available kit, which is the most widely used technique in research laboratories. In the majority of the research conducted for the β-glucan analysis, the researchers plant original seeds in their own fields and then analyze the content of β-glucan from the harvest due to lack of original seeds for the analysis. In this study, β-glucan content was analyzed from the original seeds directly collected from the particular location. Therefore, there is a minimum chance for the change of β-glucan content due to environmental and other factors in local fields; thus, it is able to obtain actual values of β-glucan content of wild and cultivated barley.
In the present study, great variations were observed in β-glucan content in wild barley, showing its diversity, and β-glucan content in wild barley was significantly higher than cultivated barley. In support of this research, a study conducted in 1986[Citation29] reported a range of grain β-glucan contents of 3.4–5.7% in a series of cultivated barley, while values of up to 13% have been reported for wild barley. [Citation34] Also, in 1987, 21 wild barley accessions collected from Israel[Citation35] and, in 1985, 13 cultivated barley varieties collected from Australia[Citation36] have been investigated for the β-glucan content. The average β-glucan contents of wild and cultivated barley were 8.69% and 4.69%, respectively, and the ranges were 6.37–12.66% and 4.03–5.26%, respectively. In our study, average values of wild and cultivated barley were 5.17% and 3.80%, respectively, and the ranges were 3.26–7.67% and 2.68–4.74%, respectively. Compared to the current study, the average values were higher in both wild and cultivated barley in previous studies. However, the range of the β-glucan content in cultivated barley was higher and the β-glucan range was lower in wild barley in the current study compared to the previous studies. This is graphically illustrated in . The ANOVA of β-glucan content between both wild and both cultivated barley in the present and previous studies was highly significant (p < 0.001).
Figure 3. Comparison of β-glucan content of wild and cultivated barley in current and previous studies.
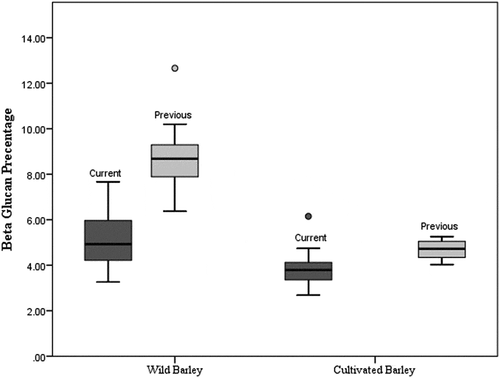
This variability of β-glucan content is due to a wide genetic variability and the variation in the environment.Citation[29–Citation31] Another explanation for this variability could be that the synthesis of β-glucan in diploid species is promoted by the function of many genes.[Citation13] β-Glucan is synthesized by individuals of the CslF and CslH gene families.[Citation37,Citation38] The CslF gene family consists of 10 associates[Citation39] and is a part of the cellulose synthase gene superfamily, which is responsible for the production of several plant cell wall polysaccharides.[Citation40] Variants among individuals of the CslF and CslH gene families regulate directly or indirectly the relative abundance and specific structure of β-glucans in both grain and the rest of the plant.[Citation41] According to the studies, the CslJ gene family is also believed to be involved in β-glucan synthesis.[Citation42,Citation43] Furthermore, it has been reported in a recent study that the interaction of a barley gene, HvTLP8 (thaumatin-like protein 8) with β-glucan and indicated that expression of HvTLP8 relates with the amount of β-glucan in the barley grain. The interaction has been found to be redox-dependent.[Citation44] Since barley is mostly used in the brewing industry, during the breeding and genetic improvement process, the effect of genes which are coding for β-glucan synthesis could be automatically depleted. Due to low genetic influence for β-glucan synthesis, cultivated varieties may record a lower amount of β-glucan than wild barley accessions. Other than malting and brewing industries, the importance, effects, and advantages of β-glucan in food processing industries have been investigated and documented in several studies.[Citation45–Citation48]
These results confirmed the findings of earlier studies of β-glucan; barleys with low β-glucan content are used in the malting and brewing industries, whereas, barleys with high levels of β-glucan content are used in applications for human health and nutrition.[Citation13] It was suggested that wild barley accessions with high β-glucan content (AFG_IG38660, TJK_IG131790, TUR_IG116112, TUR_IG39839, JOR_ISH_2, PSE_IG39630, and ISR_MHL_2) can be used for breeding and genetic improvements programs, as donors of health beneficial high β-glucan content genes to improve barley varieties particularly in human food industry, as well as potential raw material for preparation of functional foods, while wild barley accessions with low β-glucan content (JOR_KFH_2, JOR_KMH_2, ISR_TBS_1, ISR_TBS_2, and JOR_KFH_1) can be used for breeding and genetic improvement programs of low β-glucan content varieties used in livestock feed, malting, and brewing industries. Also, this research highlighted the need of the similar kind of further research with more different accessions, to obtain the most suitable accessions or cultivars in breeding programs. In addition to chemical analysis, marker-assisted selection is also useful to obtain better results in breeding and genetic improvement programs.
Conclusion
In this study, wild barley samples were selected from Fertile Crescent, Central Asia, and Tibet, and cultivated barley samples representing all over the world, to determine β-glucan content and evaluate the difference and variation of β-glucan content between wild and cultivated barley. A great difference and variation of β-glucan content in wild and cultivated barley were found and β-glucan content in wild barley was higher than that of cultivated barley. It provides guidelines to select suitable wild barley accessions for breeding and genetic improvement programs, to obtain expected β-glucan content in cultivated barley for the required purpose.
Acknowledgments
This research was funded by the National Natural Science Foundation of China (Grant No: 31571647), and partially supported by the National Natural Science Foundation of Shaanxi Province, China (Grant No: 2015JQ3074). The authors declare that they have no conflict of interest.
Additional information
Funding
References
- Arngren, M.; Hansen, P. W.; Eriksen, B.; Larsen, J.; Larsen, R. Analysis of Pregerminated Barley Using Hyperspectral Image Analysis. Journal of Agricultural and Food Chemistry 2011, 59, 11385–11394. DOI: 10.1021/jf202122y.
- Newman, C.; Newman, R.; Brief, A. History of Barley Foods. Cereal Foods World 2006, 51, 4–7.
- Harlan, J. R.; Zohary, D. Distribution of Wild Wheats and Barley. Science 1966, 153, 1074–1080. DOI: 10.1126/science.153.3740.1074.
- Zohary, D.; Hopf, M. Domestication of Plants in the Old World. The Origin and Spread of Cultivated Plants in West Asia, Europe and the Nile Valley; Clarendon Press: Oxford, England, 1993
- Wei, K.; Xue, D.; Jin, X.; Wu, F.; Zhang, G. Genotypic and Environmental Variation of β-amylase Activity, β- Glucan and Protein Fraction Contents in Tibetan Wild Barley. Journal of Zhejiang University 2009, 35, 639–644.
- Nevo, E. Origin, Evolution, Population Genetics and Resources for Breeding of Wild Barley, Hordeum Spontaneum, in the Fertile Crescent. In Barley: Genetics, Biochemistry, Molecular Biology and Biotechnology; Shewry, P. R., Eds.; C.A.B. International: The Alden Press: Oxford, 1992. pp 19–43.
- Bilgi, B.; Celik, S. Solubility and Emulsifying Properties of Barley Protein Concentrate. European Food Research and Technology 2004, 218, 437–441. DOI: 10.1007/s00217-004-0895-4.
- Bhatty, R. S. β-Glucan and Flour Yield of Hull-Less Barley. Cereal Chemistry Journal 1999, 76, 314–315. DOI: 10.1094/CCHEM.1999.76.2.314.
- Johansson, L.; Tuomainen, P.; Ylinen, M.; Ekholm, P.; Virkki, L. Structural Analysis of Water-Soluble and -Insoluble β-glucans of Whole-Grain Oats and Barley. Carbohydrate Polymers 2004, 58, 267–274. DOI: 10.1016/j.carbpol.2004.06.041.
- Rimsten, L.; Stenberg, T.; Andersson, R.; Andersson, A.; Åman, P. Determination of β-Glucan Molecular Weight Using SEC with Calcofluor Detection in Cereal Extracts. Cereal Chemistry Journal 2003, 80, 485–490. DOI: 10.1094/CCHEM.2003.80.4.485.
- Carpita, N. C. Structure and Biogenesis of the Cell Walls of Grasses. Annual Review of Plant Biology 2003, 47, 445–476. DOI: 10.1146/annurev.arplant.47.1.445.
- Wood, P. J.; Fulcher, R. G.; Stone, B. A. Studies on the Specificity of Interaction of Cereal Cell Wall Components with Congo Red and Calcofluor. Specific Detection and Histochemistry of (1→3),(1→4),-β-D-glucan. Journal of Cereal Science 1983, 1, 95–110. DOI: 10.1016/S0733-5210(83)80027-7.
- Houston, K.; Russell, J.; Schreiber, M.; Halpin, C.; Oakey, H.; Washington, J.; Booth, A.; Shirley, N.; Burton, R.; Fincher, G. A Genome Wide Association Scan for (1,3;1,4)-β-glucan Content in the Grain of Contemporary 2-Row Spring and Winter Barleys. BMC Genomics 2014, 15, 1471–2164. DOI: 10.1186/1471-2164-15-907.
- Li, J.; Kaneko, T.; Qin, L.; Wang, J.; Wang, Y. Effects of Barley Intake on Glucose Tolerance, Lipid Metabolism, and Bowel Function in Women. Nutrition 2003, 19, 926–929.
- Keogh, G. F.; Cooper, G. J.; Mulvey, T. B.; McArdle, B. H.; Coles, G. D.; Monro, J. A.; Poppitt, S. D. Randomized Controlled Crossover Study of the Effect of a Highly β-glucan–Enriched Barley on Cardiovascular Disease Risk Factors in Mildly Hypercholesterolemic Men. The American Journal of Clinical Nutrition 2003, 78, 711–718. DOI: 10.1093/ajcn/78.4.711.
- Murphy, E.; Davis, J.; Brown, A. S.; Carmichael, M. D.; Mayer, E. P.; Ghaffar, A. Effects of Moderate Exercise and Oat β-glucan on Lung Tumor Metastases and Macrophage Antitumor Cytotoxicity. Journal of Applied Physiology 2004, 97, 955–959. DOI: 10.1152/japplphysiol.00252.2004.
- Jenkins, D. J.; Wolever, T. M. S.; Leeds, A. R.; Gassull, M. A.; Haisman, P.; Dilawari, J. B.; Goff, D. V.; Metz, G. L.; Alberti, K. G. Dietary Fibres, Fibre Analogues, and Glucose Tolerance: Importance of Viscosity. British Medical Journal 1978, 1, 1392–1394.
- Klopfenstein, C. F. The Role of Cereal Beta-Glucans in Nutrition and Health. Cereal Foods World 1988, 33, 865–869.
- Martinez, V. M.; Newman, R. K.; Newman, C. W. Barley Diets with Different Fat Sources Have Hypocholesterolemic Effects in Chicks. Journal of Nutrition 1992, 122, 1070–1076. DOI: 10.1093/jn/122.5.1070.
- Thorburn, A.; Muir, J. G.; Proietto, J. Carbohydrate Fermentation Decreases Hepatic Glucose Output in Healthy Subjects. Metabolism-Clinical and Experimental 1993, 42, 780–785.
- Reed, G.; Nagodawithana, T. W. Yeast-Derived Products. Yeast Technology; Springer: Dordrecht, 1991; pp 369–412. DOI: 10.1007/978-94-011-9771-7_9.
- Vasanthan, T.; Temelli, F. Grain Fractionation Technologies for Cereal Beta-Glucan Concentration. Food Research International 2008, 41, 876–881. DOI: 10.1016/j.foodres.2008.07.022.
- Barrett, J.; Clapperton, J. F.; Divers, D. M.; Rennie, H. Factors Affecting Wort Separation. Journal of the Institute of Brewing 1973, 79, 407–413. DOI: 10.1002/jib.1973.79.issue-5.
- McCleary, B. V.; Glennieholmes, M. Enzymic Quantification of (1-3)(1-4)-β-D-glucan in Barley and Malt. Journal of the Institute of Brewing 1985, 91, 285–295. DOI: 10.1002/j.2050-0416.1985.tb04345.x.
- Hesselman, K.; Elwinger, K.; Thomke, S. Influence of Increasing Levels of β-glucanase on the Productive Value of Barley Diets for Broiler Chickens. Animal Feed Science and Technology 1982, 7, 351–358. DOI: 10.1016/0377-8401(82)90004-9.
- Wang, L.; Newman, R. K.; Newman, C. W.; Hofer, P. J. Barley Beta-Glucans Alter Intestinal Viscosity and Reduce Plasma Cholesterol Concentrations in Chicks. Journal of Nutrition 1992, 122, 2292–2297. DOI: 10.1093/jn/122.11.2292.
- McCleary, B. V.; Codd, R. Measurement of (1->3),(1->4)‐β‐D‐glucan in Barley and Oats: A Streamlined Enzymic Procedure. Journal of the Science of Food and Agriculture 1991, 55, 303–312. DOI: 10.1002/jsfa.2740550215.
- Schmitt, M. R.; Wise, M. L. Barley and Oat β-Glucan Content Measured by Calcofluor Fluorescence in a Microplate Assay. Cereal Chemistry 2009, 86, 187–190. DOI: 10.1094/CCHEM-86-2-0187.
- Henry, R. J. Genetic and Environmental Variation in the Pentosan and β-glucan Contents of Barley, and Their Relation to Malting Quality. Journal of Cereal Science 1986, 4, 269–277. DOI: 10.1016/S0733-5210(86)80029-7.
- Molinacano, J. L.; Francesch, M.; Perezvendrell, A. M.; Ramo, T.; Voltas, J.; Brufau, J. Genetic and Environmental Variation in Malting and Feed Quality of Barley. Journal of Cereal Science 1997, 25, 37–47. DOI: 10.1006/jcrs.1996.0067.
- Zhang, G.; Junmei, W.; Jinxin, C. Analysis of β-glucan Content in Barley Cultivars from Different Locations of China. Food Chemistry 2002, 79, 251–254. DOI: 10.1016/S0308-8146(02)00127-9.
- BBG01/05. Mixed-Linkage β-glucan Assay Procedure (Mccleary Method); Megazyme International Ireland Limited, Bray, Ireland, 2004.
- Johansson, L.; Virkki, L.; Anttila, H. S. I.; Esselstrom, H.; Tuomainen, P.; Sontagstrohm, T. Hydrolysis of β-glucan. Food Chemistry 2006, 97, 71–79. DOI: 10.1016/j.foodchem.2005.03.031.
- Kellogg, E. A.;. The Grasses: A Case Study in Macroevolution. Annual Review of Ecology, Evolution, and Systematics 2000, 31, 217–238. DOI: 10.1146/annurev.ecolsys.31.1.217.
- Henry, R.; Brown, A. Variation in the Carbohydrate Composition of Wild Barley (Hordeum Spontaneum) Grain. Plant Breeding 1987, 98, 97–103. DOI: 10.1111/pbr.1987.98.issue-2.
- Henry, R. A Comparative Study of the Total β-glucan Contents of Some Australian Barleys. Australian Journal of Experimental Agriculture 1985, 25, 424–427. DOI: 10.1071/EA9850424.
- Burton, R. A.; Wilson, S. J.; Hrmova, M.; Harvey, A. J.; Shirley, N. J.; Medhurst, A.; Stone, B. A.; Newbigin, E.; Bacic, A.; Fincher, G. B. Cellulose Synthase-Like CslF Genes Mediate the Synthesis of Cell Wall (1,3;1,4)-β-d-Glucans. Science 2006, 311, 1940–1942. DOI: 10.1126/science.1122975.
- Doblin, M. S.; Pettolino, F. A.; Wilson, S. M.; Campbell, R.; Burton, R. A.; Fincher, G. B.; Newbigin, E.; Bacic, A. A Barley Cellulose Synthase-Like CSLH Gene Mediates (1,3;1,4)-Beta-D-Glucan Synthesis in Transgenic Arabidopsis. Proceedings of the National Academy of Sciences of the United States of America 2009, 106, 5996–6001. DOI: 10.1073/pnas.0902019106.
- Schreiber, M.; Wright, F. L.; Mackenzie, K.; Hedley, P. E.; Schwerdt, J.; Little, A.; Burton, R. A.; Fincher, G. B.; Marshall, D. F.; Waugh, R. The Barley Genome Sequence Assembly Reveals Three Additional Members of the CslF (1,3;1,4)-β-glucan Synthase Gene Family. PLOS One 2014, 9, e90888. DOI: 10.1371/journal.pone.0090888.
- Richmond, T.; Somerville, C. The Cellulose Synthase Superfamily. Plant Physiology 2000, 124, 495–498.
- Burton, R. A.; Collins, H. M.; Kibble, N. A.; Smith, J. A.; Shirley, N. J.; Jobling, S. A.; Henderson, M.; Singh, R. R.; Pettolino, F.; Wilson, S. M. Over-Expression of Specific HvCslF Cellulose Synthase-Like Genes in Transgenic Barley Increases the Levels of Cell Wall (1,3;1,4)-Beta-D-Glucans and Alters Their Fine Structure. Plant Biotechnology Journal 2011, 9, 117–135. DOI: 10.1111/j.1467-7652.2010.00532.x.
- Farrokhi, N.; Burton, R. A.; Brownfield, L.; Hrmova, M.; Wilson, S. M.; Bacic, A.; Fincher, G. B. Plant Cell Wall Biosynthesis: Genetic, Biochemical and Functional Genomics Approaches to the Identification of Key Genes. Plant Biotechnology Journal 2006, 4, 145–167. DOI: 10.1111/j.1467-7652.2005.00169.x.
- Fincher, G. B.;. Revolutionary Times in Our Understanding of Cell Wall Biosynthesis and Remodeling in the Grasses. Plant Physiology 2009, 149, 27–37. DOI: 10.1104/pp.108.130096.
- Singh, S.; Tripathi, R. K.; Lemaux, P. G.; Buchanan, B. B.; Singh, J. Redox-Dependent Interaction between Thaumatin-Like Protein and β-glucan Influences Malting Quality of Barley. Proceedings of the National Academy of Sciences 2017, 114, 7725–7730. DOI: 10.1073/pnas.1701824114.
- Rezaei, R.; Khomeiri, M.; Kashaninejad, M.; Aalami, M.; Mazaheri-Tehrani, M. Steady and Dynamic Rheological Behaviour of Frozen Soy Yogurt Mix Affected by Resistant Starch and β-glucan. International Journal of Food Properties 2018, 20, 2688–2695. DOI: 10.1080/10942912.2017.1397692.
- Ahmed, J.; Thomas, L. Effect of β-glucan Concentrate on the Water Uptake, Rheological and Textural Properties of Wheat Flour Dough. International Journal of Food Properties 2015, 18, 1801–1816. DOI: 10.1080/10942912.2014.971183.
- Brennan, C.; Samyue, E. Evaluation of Starch Degradation and Textural Characteristics of Dietary Fiber Enriched Biscuits. International Journal of Food Properties 2004, 7, 647–657. DOI: 10.1081/JFP-200033070.
- Gupta, M.; Bawa, A. S.; Semwal, A. D. Effect of Barley Flour Incorporation on the Instrumental Texture of Sponge Cake. International Journal of Food Properties 2009, 12, 243–251. DOI: 10.1080/10942910802312082.