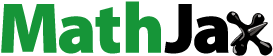
ABSTRACT
The application of mung bean protein in foods is limited due to its poor functionality. Acylation with succinic anhydride could be used to improve the functionalities of protein. In this study, the influence of the weight ratio of succinic anhydride to protein (0–0.10) on the degree of N-acylation, physicochemical properties and protein functionalities including emulsifying properties of mung bean protein isolates (MPI) were investigated. The extent of N-acylation of MPI increased as the succinic anhydride:protein ratio increased, while the ζ-potential at neutral pH decreased. In addition, succinylation had an impact on the average particle size of MPI depending on the succinic anhydride:protein ratio. Succinylation had no impact on the total and exposed free sulfhydryl groups. Moreover, the isoelectric point (pI) of the succinylated proteins shifted from pH 5 to lower pH. Succinylation increased the emulsifying activity index (EAI), while the impact on the emulsion stability index depended on the succinic anhydride:protein ratio. Thus, succinylation at low succinic anhydride:protein ratio can alter mung bean protein charge and affect physicochemical and functional properties especially emulsifying properties that could be used to increase its utilization.
Introduction
There are many factors that affect the functional properties[Citation1] of proteins used for food applications such as emulsification, gel formation, thickening, foaming, water holding capacity, and fat binding. The physicochemical and conformational properties of proteins, their molecular structure, the composition of the solution surrounding the proteins, and their thermal-mechanical history will impact their ability to provide specific and desirable characteristics in food applications.[Citation2–Citation4] For example, it has been reported that the surface charge of protein governs several physicochemical and functional properties of proteins such as solubility; emulsifying, foaming, and gelling properties.[Citation5]
The most common chemical modification used for altering the physicochemical and functional properties of proteins is acylation. Acylation with acid anhydride has been used to improve the functional properties of various proteins.[Citation6–Citation8] The ε-NH2 at the protein surface easily reacts with acid anhydride such as acetic anhydride, propionic anhydride, maleic anhydride and succinic anhydride. Acylation reduces the positive charges of the ε-NH2 affected amino acids and dominates the negative charges of the proteins.[Citation9]
Succinylation, acylation with succinic anhydride, is used to alter functionality in a range of proteins. It has been reported that succinylation decreases the surface charge or ζ-potential of patatin protein,[Citation10] soybean protein,[Citation11] and kidney bean protein isolate.[Citation8] The average particle size or Z-average of milk proteins are decreased by succinylation.[Citation12,Citation13] Succinylation increases the molecular weight of patatin protein[Citation10] and shifts the isoelectric point (pI) of legume proteins to a more acidic pH.[Citation8,Citation14] The water solubility of bambara groundnut protein concentrate,[Citation15] oat protein isolate,[Citation16] and milk protein concentrate[Citation12] is also enhanced by succinylation. Succinylation reduces the foaming capacity, but increases the water and oil binding capacity of oat protein isolate.[Citation16] Lablab bean protein concentrate and lentil globulin treated with succinylation have been shown to exhibit higher emulsion activity and stability.[Citation6,Citation14] Succinylation also alters the physicochemical properties of protein. Recently succinylation was used to produce a superabsorbent polymer material from soy protein with high water uptake.[Citation17] Mung bean protein isolate (MPI) is not commercially available. It is a by-product from mung bean vermicelli production, and has high protein content and high nutritional value. MPI could be considered as a value-added product and reduced waste in the vermicelli production. However, the use of mung bean protein in food products is limited due to its beany flavor. Moreover, mung bean protein exhibits poor functionalities in the area of protein solubility, foaming, and emulsifying. The succinylation of MPI has been done at the concentration of 0.2 to 1.0 g succinic anhydride/g of protein.[Citation18] The high level of protein modification could induce molecular conformational changes of protein via high electrostatic repulsion force at the protein surface.
We hypothesized that succinylation at lower succinic anhydride:protein ratio than reported previously would alter the protein charge at the surface and affect the physicochemical and functional properties of MPI. The objective of this study was to determine the influence of the weight ratio of succinic anhydride to protein (0–0.10) on the degree of N-acylation, physicochemical properties and protein functionalities of mung bean protein isolates (MPI), with particular focus on its emulsifying properties. The knowledge from this work could be used as a guideline for the targeted alteration of protein functionality in other proteins.
Materials and methods
Materials
Mung bean protein isolate (MPI; 91.99% protein) dry basis was obtained from the laboratory of a local mung bean vermicelli factory (Pathum Thani, Thailand). Sodium dihydrogenphosphate and trisodium phosphate were purchased from Wako Pure Chemical Industries, Ltd. (Osaka, Japan). Succinic anhydride, TNBS (2,4,6-trinitrobenzene sulfonic acid), ANS (1,8-anilinonaphthalenesulfonate), and DTNB (5,5ʹ-dithiobis (2-nitrobenzoic acid)) were purchased from Sigma Aldrich (St. Louis, MO). Analytical grade hydrochloric acid and sodium hydroxide were purchased from Carlo Erba Reagents (Carlo Erba, Milan, Italy). All chemicals were used directly from the container without further purification. Distilled and de-ionized water was used for the preparation of all buffer solutions. The pH of all buffer solutions used in this study was measured using a pH meter (SevenEasyTM pH Meter S20, Mettler-Toledo, Schwerzenbach, Switzerland).
Protein solution preparation
Powdered MPI was dissolved in 5 mM phosphate buffer (pH 8.5 ± 0.1) to obtain 10 wt% MPI. Protein solutions were stirred for 1 h to ensure complete dissolution. The pH of protein solutions was adjusted to 8.5 using 1.0 N NaOH or 1.0 N HCl prior to succinylation.
Succinylation
Succinylation was performed based on the method of Yin and others.[Citation8] All protein solutions were incubated in an ice bath for five minutes before succinylation. Succinylation was performed for 30 min by gradual addition of powdered succinic anhydride (0–10 wt% of protein) into the protein solutions with slow stirring. The pH of the protein solutions during succinylation was maintained at 8.5–9.0 using 2.0 N HCl or 2.0 N NaOH. The reaction was terminated by adjusting the pH of the protein solutions to 7.0 at 0°C. Then, the protein solutions were transferred into a dialysis bag (1 kDa cut-off) for dialysis against distilled water at 4°C for 48 h to remove succinic acid and salts. The modified protein solution:distilled water volume ratio during dialysis was fixed at 1:10. Distilled water used for dialysis was changed every 6 h. The contents were then freeze-dried (Dura-Dry MP, FTS SystemTM, USA) at −85°C, 500 mTor. The experiments were done in triplicate.
Degree of N-succinylation determination
The influence of succinylation on the degree of succinylation or N-acylation of the proteins was determined following the method of Yin and others.[Citation8] Briefly, 1.0 wt% protein suspensions were prepared by dissolving the powdered proteins in 50 mM phosphate buffer (pH 8.5) containing 5 mM NaCl. One milliliter of protein solution was mixed with 1 ml of 0.1% (w/v) TNBS solution. The mixed solution was incubated at 60°C for 2 h then cooled to room temperature and 1 ml of 10% SDS (sodium dodecyl sulfate) and 0.5 ml of 1.0 N HCl were added. The absorbance (Abs) of the final solution and the reagent blank was determined at 335 nm using a UV-Vis spectrophotometer (Helios Alpha, Thermo Electron Corporation, England). Since TNBS reacts with the free NH2 group of primary amine, the degree of N-succinylation can be determined based on the amount of the free NH2 groups available for the reaction. The degree of N-acylation (DA) was calculated as follows:
Zeta (ζ) potential measurement
The effect of succinylation on the Zeta (ζ) potential of the protein solutions was determined using a method described by Chanasattru, Decker, and McClements.[Citation19] Briefly, 1.0 wt% protein suspensions were prepared by dissolving the powdered proteins in distilled water under moderate stirring for 1 h. The pH of the protein suspensions was adjusted to 7.0 prior to analysis. The ζ-potential of the protein particles was determined using a particle electrophoresis instrument (Zetasizer Nano ZS, Malvern Instruments Ltd., UK). Measurements were carried out on three sample solutions prepared at different times from the same materials (replicates).
Particle size measurement
The influence of succinylation on the mean particle size of the protein suspensions was determined using a commercial dynamic light scattering instrument (Zetasizer Nano ZS, Malvern Instruments Ltd., UK) as described by Shilpashree and others.[Citation12] Briefly, 1.0 wt% protein solutions were prepared by dissolving the powdered protein in distilled water under moderate stirring for 1 h. The pH of the protein suspensions was adjusted to 7.0 prior to analysis. The mean particle size was reported as a “Z-average”, calculated from the signal intensity versus particle size data normalized to size increments using Mie Theory. Measurements were carried out on three sample solutions prepared at different times from the same materials (replicates).
Molecular weight of proteins
Molecular weight of protein samples was determined using sodium dodecyl sulfate-polyacrylamide gel electrophoresis (SDS-PAGE) as described by Laemmli.[Citation20] Briefly, SDS-PAGE was performed on a discontinuous buffer system using 12.5% separating gel and 4% stacking gel. Protein samples in loading buffer (containing 0.0625 M Tris-HCl, 10% glycerine, 2% SDS, and 0.0025% bromophenol blue) were loaded onto the gel (10 µg of protein/well). Gel electrophoresis was performed at 20 mA for 90 min. Gel was stained with Coomassie Blue G250 and destained in a destaining reagent (30% methanol, 10% acetic acid and 60% distilled water) prior to gel imaging.
Exposed and total free sulfhydryl groups
The influence of succinylation on the exposed and total free sulfhydryl (SH) groups of the proteins was studied using Ellman’s reagent [5,5ʹ-dithiobis (2-nitrobenzoic acid); DTNB] as described by Du and others.[Citation21] Protein solutions (0.05 wt%) were prepared by dissolving the powdered proteins in a buffer solution pH 8.0 (86 mM TRIS, 90 mM glycine and 4 mM EDTA). One milliliter of protein solution was mixed with 10 μl of Ellman’s reagent (4 mg of DTNB/ml of standard buffer) then allowed to incubate at room temperature for 1 h. The mixture was centrifuged at 12,000xg, 4°C for 10 min. The supernatant was collected for exposed free sulfhydryl group content measurement. Absorbance was recorded at 412 nm using a UV-Vis spectrophotometer (Helios Alpha, Thermo Electron Corporation, England). The total free sulfhydryl group content was also determined following the same technique but using the standard buffer containing denaturing agent (8 M urea and 0.5% (w/v) SDS). The standard buffer was used as blank. Free sulfhydryl groups (μmole SH/g protein) of each sample were calculated using a molar extinction coefficient of 1.36 x 104 M−1. Measurements were carried out on three sample solutions prepared at different times from the same materials (replicates).
Surface hydrophobicity of protein determination
The surface hydrophobicity (S0) of the succinylated protein was determined using 1, 8-anilinonapthalenesulfonate (ANS−) as described by Haskard and Li-Chan.[Citation22] ANS− stock solution (8 mM) was prepared by dissolving ANS− in 10 mM of phosphate buffer (pH 7.0). The protein content of MPI sample was determined by Lowry’s method using bovine serum albumin (BSA) as a standard.[Citation23] The protein suspensions of 0.20–0.50 mg protein/ml were prepared in 10 mM of phosphate buffer pH 7.0. Four milliliters of diluted protein suspension were mixed with 20 µl of ANS− stock solution and vortexed for 5 s. The fluorescence intensity (FI) of the mixtures was measured at an excitation wavelength at 370 nm and emission wavelength at 470 nm using a microplate reader (Infinite M200 PRO, TECAN Austria GmbH, Austria). The FI of each sample was calculated by subtracting the FI of the buffer. The S0 was the initial slope of the subtracted FI versus the protein concentration calculated by linear regression analysis. Measurements were carried out on three sample solutions prepared at different times from the same materials (replicates).
Protein solubility profile analysis
The influence of succinylation on the solubility profile of the proteins was studied using a method described by Tsumura and others.[Citation24] Briefly, 1.0 wt% protein solutions were prepared by dissolving the powdered protein in distilled water under slow stirring at room temperature for 1 h. The pH of the protein suspensions was adjusted and varied from 3 to 9. The solutions were continually stirred for 1 h. Protein suspensions were centrifuged at 8,000xg, 4°C for 20 min. The supernatants were collected. The protein content in the supernatants was determined using Lowry’s method with BSA (Bovine serum albumin) as a standard protein. It was assumed that all soluble proteins dissolved in 0.5 N NaOH. The protein solubility was calculated as follows:
Measurements were carried out on two or three sample solutions prepared at different times from the same materials (replicates).
Emulsifying properties
The influence of succinylation on the emulsifying properties of the proteins was elucidated using a method adapted from Harkouss and others.[Citation25] Emulsifying properties of protein samples were determined. Briefly, 1.0 wt% protein solutions were prepared by dissolving powdered protein in 5mM phosphate buffer pH 7.0 at the level of 1.0 wt%. The final pH of protein solution was adjusted to 7.0 ± 0.1 using 1.0 N NaOH or 1.0 N HCl. For emulsion preparation, 10 g of soybean oil and 30 g of the protein solutions were used. The mixtures were homogenized (ULTRA TURRAX® T25 basic, IKA® WERKE, Germany) at a speed of 13,500 rpm for 1 min. After that, a 50 µl aliquot of the emulsion was collected at 0 and 10 min. Collected emulsions were mixed with 5 ml of 0.10% SDS solution using a vortex mixer. The absorbance of mixture was measured at 500 nm using a spectrophotometer (Helios Alpha, Thermo Electron Corporation, England) and the absorbance at 0 (A0) and 10 min (A10) after emulsion formation was used to calculate the emulsifying activity index (EAI) and emulsion stability index (ESI) as follows:
where DF is dilution factor, L is the path length of cuvette (cm), ϕ is oil volume fraction of emulsion, C is weight of protein/unit volume (g/ml) of aqueous phase before emulsion formation.
Statistical analysis
Experiments were performed triplicate using freshly prepared samples. Data were analyzed by analysis of variance (ANOVA) using SPSS. Duncan’s multiple range tests were used to compare the mean at a 95% confidence level.
Results and discussion
Influence of succinic concentration on the degree of N-acylation of protein
MPI was used to study the influence of the succinic anhydride concentration on the degree of N-acylation of proteins. The treatment without succinic anhydride was used as the control. The influence of the succinic anhydride:protein weight ratios on the degree of N-acylation of the proteins is shown in . The results showed that the increase in succinic anhydride:protein weight ratio significantly increased (p < 0.05) the degree of N-acylation in a linear manner. This could be due to the fact that both the ε-amino and α-amino groups of the MPI molecules could form N-acylation with the carboxylic groups of succinic anhydride. The increase of succinic anhydride:protein weight ratios from 0 to 0.10 resulted in an overall 40% increase of the degree of N-acylation (). It had been reported that the degree of N-acylation increased from 0% to 78% for mung bean protein isolated treated with succinic anhydride:protein weight ratio of 1.0.[Citation18] The degree of N-acylation of MPI exhibited a linear relationship with the succinic anhydride:protein weight ratio (Degree of N-acylation = 383.5 x (succinic anhydride:protein ratio) +3.073, R2 = 0.980). These results suggest that the higher reaction rate observed with increasing succinic anhydride:protein weight ratios was due to a higher collision frequency between the carboxylic groups of succinic anhydride and the amino groups of protein. Our results agreed with the phenomena found previously in mung bean protein isolates,[Citation18] kidney bean protein isolates,[Citation8] and lablab bean protein concentrate[Citation6] and confirmed that the degree of succinylation is governed by the succinic anhydride:protein weight ratios.
Table 1. Influence of succinic anhydride:protein ratio on the degree of N-acylation, ζ-potential, and surface hydrophobicity (S0) of mung bean protein isolate (MPI). Means within a row with different superscripts are significantly different at p < 0.05.
Influence of succinylation on the zeta (ζ) potential of protein
The purpose of this experiment was to examine the effect of succinylation on the ζ-potential of MPI. The effect of the succinic anhydride:protein weight ratios on the ζ-potential of proteins is shown in . The result showed that the ζ-potential of succinylated MPI significantly decreased (p < 0.05) with increasing succinic anhydride:protein weight ratio. This result generally agrees with research in which succinylated proteins with decreasing ζ-potential at pH higher than their pI were reported in kidney bean protein isolates,[Citation8] soy protein isolates,[Citation11] and patatin.[Citation10] The ζ-potential of MPI after succinylation at 0–0.10 succinic anhydride:protein weight ratios decreased from −19.20 ± 0.70 to −32.62 ± 0.86 mV (). The ζ-potential of MPI had a linear relationship with the succinic anhydride:protein weight ratio (ζ-potential = −149.7 x (succinic anhydride:protein ratio) – 19.96, R2 = 0.941).
Succinylation of proteins is a reaction between the carboxylic groups of succinic anhydride and both ε-amino and α-amino groups of proteins. The net charge of the protein molecules depends upon a balance between the negative and positive charges at the protein surface.[Citation9] The reduction in the ζ-potential could be attributed to the reduction of the positive charges at the protein surface resulting in a dominant negative charge. The decrease in ζ-potential of MPI with the increase of the succinic anhydride:protein weight ratios indicates that succinic anhydride likely reacts with the ε-amino or α-amino groups at the protein surface, creating fewer positive charges at the protein surface.
Influence of succinylation on the particle size of protein
We hypothesize that a small degree of succinylation could change the charge of the protein without interfering with the unfolded state of the protein. Thus, 0 to 0.10 succinic anhydride:protein weight ratio was selected to minimize any changes to the folded state of the protein. The influence of the succinylation on the average particle size (Z-average) of MPI is shown in . These results show that succinylation at 0–0.10 succinic anhydride:protein weight ratios significantly impacted on the Z-average (p < 0.05) of proteins. The Z-average of MPI without succinic anhydride treatment was 959.7 ± 77.0 nm () and the Z-average-succinic anhydride:protein weight ratio curve of MPI thereafter can be characterized into three regions (). The first region, characterized by succinic anhydride:protein weight ratios from 0 to 0.03, exhibited an increase in Z-average with the increasing succinic anhydride concentrations, from 959.7 ± 77.0 to 2675.3 ± 507.2 nm. The increase of MPI particle size in this region could be attributed to the increase of its molecule size caused by the succinyl group reacting with the amino groups of MPI. The second region, characterized by succinic anhydride:protein weight ratios from 0.03 to 0.07, exhibited constant Z-average in the range of 2516.0 ± 400.2 to 2675.3 ± 507.2 nm. The third region, characterized by succinic anhydride:protein weight ratios from 0.07 to 0.10, exhibited decreasing Z-average with increased succinic anhydride concentration. This could be due to succinylation causing MPI aggregate to break down due to increased electrostatic repulsive force between succinylated MPI molecules, as evidenced in milk protein concentrate[Citation12] and sodium caseinate.[Citation13]
Influence of succinylation on the molecular weight of protein
The effect of succinylation at 0–0.10 succinic anhydride:protein weight ratios on the molecular weight of MPI is shown in . The results showed that the succinic anhydride:protein weight ratios increased the molecular weight of the major protein band. Untreated MPI exhibited a major band at 50 kDa, which shifted to a higher molecular weight with the increasing succinic anhydride:protein weight ratios, likely due to the addition of succinyl groups at both the ε-amino and α-amino groups of protein as mentioned in the previous section. These results correspond with the degree of the N-acylation and the ζ-potential measurements () described previously. The molecular weight of the succinylated protein increased with increased succinic anhydride:protein weight ratios in oat protein isolates,[Citation16] red kidney bean protein isolate[Citation26] and hemp protein isolate.[Citation27]
Influence of succinylation on the free sulfhydryl groups of protein
The influence of the succinylation on the exposed and total free sulfhydryl group contents of proteins was studied. Powder of the succinylated MPI treated with various succinic anhydride:protein weight ratios (0–0.10) was used in this experiment. Proteins treated with succinic anhydride:protein weight ratio of 0 were used as control. The influence of the succinic anhydride:protein weight ratios on the total and exposed free sulfhydryl group contents is shown in . The results showed that succinylation had no impact on the free sulfhydryl group contents of all treatment ratios. In addition, these results suggested that some sulfhydryl group had been buried inside of the protein molecule. The total free sulfhydryl group content of proteins was 1.90 ± 0.15 µmol/g protein for control MPI (). Succinylation also had no effect on the exposed free sulfhydryl group contents of MPI. The exposed free sulfhydryl group contents of the control MPI was 0.89 ± 0.02 µmol/g protein.
Influence of succinylation on the surface hydrophobicity of protein
The effect of succinylation at the succinic anhydride:protein weight ratios of 0–0.10 on the hydrophobicity (S0) of MPI was investigated. Proteins treated with succinic anhydride:protein weight ratio of 0 were used as control. The influence of the succinic anhydride:protein weight ratios on the S0 of proteins is shown in . The result showed that succinylation had an impact (p < 0.05) on the S0 of all treatment ratios. The S0 of the proteins decreased with the increasing of the succinic anhydride:protein weight ratios. Increase the succinic anhydride:protein weight ratios from 0 to 0.10 resulted in a decrease of S0 from 37.26 ± 0.35 x 104 to 29.85 ± 0.36 x 104 (). The decreased S0 in the succinylated proteins could be due to the presence of a higher net negative charge at the surface of protein molecules, affecting the ability of the ANS− probe to bind to the polar sites because of high electrostatic repulsion. Similar trends were found in soy protein hydrolysate,[Citation28] kidney bean protein isolate,[Citation8] sodium caseinate,[Citation13] milk protein concentrate,[Citation12] yak casein micelles[Citation29] and whey protein concentrate.[Citation30] Other studies suggested that the decreasing of S0 of succinylated proteins could be attributed to the polarity of the succinylated proteins where a higher amount of polar charged groups or a relatively lower amount of non-polar groups at the protein surface resulting in the increasing of the net charge.[Citation12,Citation28,Citation29] In addition, stearic hindrance could also cause the reduction of the S0 of succinylated proteins, attributed to the structural modification of proteins upon succinylation.[Citation12,Citation29]
Influence of succinylation on the protein solubility
The influence of the succinic anhydride:protein weight ratios on MPI solubility is shown in . The results show that the succinic concentrations as succinic anhydride:protein weight ratios had a significant effect on the protein solubility (p < 0.05). Our data revealed that the control MPI had lowest protein solubility at pH 5.0, which is close to its isoelectric point (pI). The lowest solubility of control MPI was 3.16 ± 0.13% (). The solubility increased as the pH was adjusted away from the pI. Proteins have higher negative charges at pH higher than that of their pI and higher positive charge at the pH lower than that of their pI. The results showed that the pI of MPI shifted to a lower pH with the increase of succinic anhydride:protein weight ratios (). Similar results were observed in lablab protein concentrate,[Citation6] jack bean protein concentrate,[Citation31] bambara groundnut protein concentrate,[Citation15] soy protein isolate[Citation11] and milk protein concentrate.[Citation12] These results could be attributed to the capability of succinic anhydride to bind with free ε-amino and α-amino groups of proteins resulting in less free ε-amino and α-amino groups remaining. At neutral pH, free ε-amino and α-amino groups expressed their positive changes, while carboxylic groups express their negative charges. Succinylation altered the free ε -amino and α-amino groups with carboxylic groups. Thus, less positive charges of free ε-amino and α-amino groups on the surface of proteins resulted in the increasing of the net negative charges. The solubility at pH 5.0 of succinylated MPI increased with the increasing of succinic anhydride:protein weight ratios. The pHs that succinylated MPI showed the lowest protein solubility were in the range of 4–5 (). Similar trends were found in lentil globulin,[Citation14] rapeseed protein isolate,[Citation32] lablab protein concentrate,[Citation6] jack bean protein concentrate,[Citation31] bambara groundnut protein concentrate,[Citation15] soy protein isolate[Citation11] and kidney bean protein isolate.[Citation8]
Influence of succinylation on the emulsifying properties of protein
The effect of the succinic anhydride:protein weight ratios of 0 to 0.10 on the emulsifying activity index (EAI) and emulsion stability index (ESI) are shown in . The results showed that the succinic anhydride:protein weight ratios had an impact on the EAI depending on the succinic anhydride:protein weight ratios. The EAI of control MPI was 24.62 ± 1.92 (m2/g protein). The EAI of MPI treated with succinic anhydride:protein weight ratio of 0.07 exhibited higher EAI than that of the control, and significantly increased with increased succinic anhydride:protein weight ratio of 0.10, as shown in . These results agree with previous research in MPI.[Citation18] Proteins that also show this trend include α-globulin from sesame seed,[Citation33] lentil globulin,[Citation14] oat protein isolate,[Citation16] red kidney bean protein isolate,[Citation26] hemp protein isolate,[Citation27] milk protein concentrate,[Citation12] sodium caseinate,[Citation13] lablab bean protein concentrate[Citation6] and whey protein concentrate.[Citation30] However, succinylation decreased the emulsion activity of jack bean protein concentrate[Citation31] and bambara groundnut protein concentrates.[Citation15] Emulsion activity depends on the interfacial interaction of aqueous-oil interfaces.[Citation2,Citation34] It has been suggested that the emulsifying activity of protein is governed by (1) the capability of protein molecules to adsorb at the water-oil interface, (2) the strength of the protective membrane surrounding the oil droplets, (3) the ability of protein molecules to partially unfold at the oil-water interface, and (4) the ability of protein molecules to arrange the hydrophobic and hydrophilic parts at the oil-water interface.[Citation2,Citation34] The increase in the emulsifying activity of succinylated protein could be attributed to the structural flexibility of succinylated protein, which could facilitate the diffusion of proteins at the oil-water interface and form a film surrounding the emulsion droplets.[Citation12,Citation16,Citation30] Delahaije and others[Citation10] suggested that the improvement of the emulsifying properties of succinylated proteins is not only attributed to the net charge of proteins but also the partial unfolding of proteins upon succinylation, which increases the adsorption rate of the protein to the aqueous-oil interface. Succinylation could enhance the exposure of buried hydrophilic and hydrophobic amino groups within protein molecules, facilitating the adsorption rate of proteins at the aqueous-oil interface resulting in the observed increase of the emulsifying activity of proteins.[Citation6,Citation15,Citation31]
Figure 5. Influence of succinylation on the emulsifying activity index (EAI) and emulsion stability index (ESI) of mung bean protein isolate (MPI) at various succinic anhydride:protein ratios.
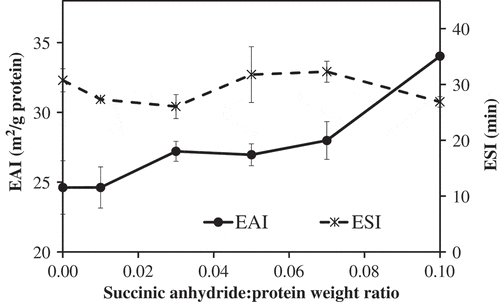
There was no difference in the ESI of MPI treated with succinic anhydride:protein weight ratios of 0–0.10 (). This result is counter to ESI of succinylated MPI protein,[Citation18] and also α-globulin from sesame seed,[Citation33] lentil globulin,[Citation14] lablab bean protein concentrate,[Citation6] red kidney bean protein isolate,[Citation26] milk protein concentrate,[Citation12] sodium caseinate[Citation13] and whey protein concentrate.[Citation30] In contrast, succinylation decreased the emulsion stability of jack bean protein concentrate,[Citation31] bambara groundnut protein concentrate[Citation15] hemp protein isolates[Citation27] and oat protein isolates.[Citation16] Normally, the emulsion stability is governed by the strength of the protective membrane and is facilitated by the protein emulsifier coating on the oil droplet.[Citation2] The stability of emulsions stabilized by the control protein could relate to the magnitude of the electrostatic repulsive force created by charges at the protein surface. Our ζ-potential measurement suggested that all succinylated proteins should have a stronger protective membrane due to their higher ζ-potential. The stability of the emulsions stabilized by succinylated MPI had a constant trend with the increasing of the succinic anhydride:protein weight ratios. These results suggested that the stability of the emulsions stabilized by succinylated protein was not governed solely by the electrostatic repulsive force generated by the negative charges of the carboxylic groups of proteins at the protein surface but also governed by the other factors. We hypothesize that the net negative charge, surface hydrophobicity, conformation, and flexibility of proteins could produce a strong protective membrane surrounding the oil droplet. The increase in the emulsion stability could be attributed to the increase of the negative charges of succinylated proteins at the membrane of emulsion droplets, increasing the electrostatic repulsion between emulsion droplets and retarding the droplets flocculation.[Citation30] Shilpashree and others[Citation13] suggested that the addition of succinyl groups at the protein molecules by succinylation could increase the electrical potential of the ionized layer of the interfacial film around oil droplets, which enhancing the emulsion stability. Lawal, Adebowale, and Adebowale[Citation15] suggested that succinylation could increase the emulsion stability of proteins by retarding the droplets coalescence due to an increasing the active binding sites by unfolding of protein and also the formation of charged film and/or hydrated layer around oil droplets, which caused droplets repulsion. On the other hand, succinylation could decrease the emulsion stability of proteins due to decrease in the protein–protein interactions, which lower elasticity of protein film around emulsion droplets.[Citation16] Moreover, it had been reported that the emulsion properties of succinylated proteins were influenced by many factors such as degree of succinylation,[Citation18] pH[Citation15,Citation31] and ionic strength.[Citation6,Citation15,Citation31]
Conclusion
We have shown that succinylation has an impact on the physicochemical and emulsifying properties of MPI. The degree of N-acylation of MPI increased with the increasing of succinic anhydride:protein weight ratios. Succinylation had an impact on the Z-average of MPI depending on the succinic anhydride:protein ratio. Succinylation decreased the ζ-potentials of MPI. The molecular weight of MPI increased after succinylation. Succinylation had no impact on the exposed free sulfhydryl groups of MPI. The S0 of MPI decreased with the increasing of succinic anhydride:protein weight ratios. Succinylation had an impact on the protein solubility profile of MPI, which shift the pH that the protein had the lowest solubility (pI) to more acidic pH. The EAI of MPI increased after succinylation, but succinylation had no impact on the ESI of MPI. This suggests that the physicochemical and emulsifying properties of proteins can be altered by succinylation at low succinic anhydride:protein weight ratios. This could be used as a guideline to improve the solubility and emulsifying properties of other protein. Thus, improving the physicochemical and emulsifying properties of MPI by this method could be used to expand its applications. However, the modification method employed in this study used a chemical reagent, so the safety of the product should be studied before applying this process to food and cosmetics.
Acknowledgments
The authors gratefully acknowledge the financial support partially provided by Thammasat University under the TU Research Scholar.
References
- Damodaran, S.;. Amino Acids, Peptides and Proteins. In Food Chemistry, 3rd ed.; Fennema, O. R., Ed.; Marcel Dekker: New York, 1996, pp 321–429.
- McClements, D. J.;. Modulation of Globular Protein Functionality by Weakly Interacting Cosolvents. Critical Reviews in Food Science and Nutrition 2002, 45(2), 417–471. DOI: 10.1080/20024091054210.
- Schmidt, R. H.;. Gelation and Coagulation. In Protein Functionality in Foods, Cherry, J. P., Ed.; ACS Symposium Series. American Chemical Society: Washington, DC. 1981; Vol. 147, pp 131–147.
- Ziegler, G. R.; Foegeding, E. A. The Gelation of Proteins. Advances in Food and Nutrition Research 1990, 34, 203–298.
- Zayas, J. F.; Functionality of Proteins in Food; Springer-Verlag: New York, 1997
- Lawal, O. S.;. Functionality of Native and Succinylated Lablab Bean (Lablab Purpureus) Protein Concentrate. Food Hydrocolloids 2005, 19, 63–72. DOI: 10.1016/j.foodhyd.2004.04.015.
- Miedzianka, J.; Peksa, A.; Aniolowska, M. Properties of Acetylated Potato Protein Preparations. Food Chemistry 2012, 133, 1283–1291. DOI: 10.1016/j.foodchem.2011.08.080.
- Yin, S. W.; Tang, C. H.; Wen, Q. B.; Yang, X. Q.; Yuan, D. B. The Relationships between Physicochemical Properties and Conformational Features of Succinylated and Acetylated Kidney Bean (Phaseo Vulgaris L.). Food Research International 2010, 43, 730–738. DOI: 10.1016/j.foodres.2009.11.007.
- Damodaran, S.;. Amino Acids, Peptides and Proteins. In Fennema’s Food Chemistry, 4th ed.; Damodaran, S., Parkin, K. L., Fennema, O. R., Eds.; CRC Press: Boca Raton, 2007, pp 217–330.
- Delahaije, R. J. B. M.; Wierenga, P. A.; Giuseppin, M. L. F.; Gruppen, H. Improved Emulsion Stability by Succinylation of Patatin Caused by Partial Unfolding Rather than Charge Effect. Journal of Colloid and Interface Science 2014, 430, 69–77. DOI: 10.1016/j.jcis.2014.05.019.
- Caillard, R.; Petit, A.; Subirade, M. Design and Evaluation of Succinylated Soy Protein Tablet as Delayed Drug Delivery Systems. International Journal of Biological Macromolecules 2009, 45, 414–420. DOI: 10.1016/j.ijbiomac.2009.06.013.
- Shilpashree, B. G.; Arora, S.; Chawla, P.; Tomar, S. K. Effect of Succinylation on Physicochemical and Functional Properties of Milk Protein Concentrate. Food Research International 2015, 72, 223–230. DOI: 10.1016/j.foodres.2015.04.008.
- Shilpashree, B. G.; Arora, S.; Chawla, P.; Vakkalagadda, R.; Sharma, A. Succinylation of Sodium Caseinate and Its Effect on Physicochemical and Functional Properties of Protein. LWT-Food Science and Technology 2015, 64, 1270–1277. DOI: 10.1016/j.lwt.2015.07.008.
- Bora, P. S.;. Functional Properties of Native and Succinylated Lentil (Lens Culinaris) Globulins. Food Chemistry 2002, 77, 171–176. DOI: 10.1016/S0308-8146(01)00332-6.
- Lawal, O. S.; Adebowale, K. O.; Adebowale, Y. A. Functional Properties of Native and Chemically Modified Protein Concentrates from Bambara Groundnut. Food Research International 2007, 40, 1003–1011. DOI: 10.1016/j.foodres.2007.05.011.
- Mirmoghtadaie, L.; Kadivar, M.; Shahedi, M. Effects of Succinylation and Deamidation on Functional Properties of Oat Protein Isolate. Food Chemistry 2009, 114, 127–131. DOI: 10.1016/j.foodchem.2008.09.025.
- Cuadri, A. A.; Romero, A.; Bengoechea, C.; Guerrero, A. Natural Superabsorbent Plastic Materials Based on a Functionalized Soy Protein. Polymer Testing 2017, 58, 126–134. DOI: 10.1016/j.polymertesting.2016.12.024.
- El-Adawy, T. A.;. Functional Properties and Nutritional Quality of Acetylated and Succinylated Mung Bean Protein Isolate. Food Chemistry 2000, 70, 83–91. DOI: 10.1016/S0308-8146(00)00079-0.
- Chanasattru, W.; Decker, E. A.; McClements, D. J. Influence of Glycerol and Sorbitol on Thermally Induced Droplet Aggregation in Oil-In-Water Emulsions Stabilized by β-lactoglobulin. Food Hydrocolloids 2009, 23, 253–261. DOI: 10.1016/j.foodhyd.2008.02.004.
- Laemmli, U. K.;. Cleavage of Structural Proteins during the Assembly of the Head of Bacteriophage T4. Nature 1970, 227, 680–685.
- Du, Y.; Jiang, Y.; Zhu, X.; Xiong, H.; Shi, S.; Hu, J.; Peng, H.; Zhou, Q.; Sun, W. Physicochemical and Functional Properties of the Protein Isolate and Major Fractions Prepared from Akebia Trifoliata Var. Australis Seed. Food Chemistry 2012, 133, 923–929. DOI: 10.1016/j.foodchem.2012.02.005.
- Haskard, C. A.; Li-Chan, E. C. Y. Hydrophobicity of Bovine Serum Albumin and Ovalbumin Determined Using Uncharged (PRODAN) and Anionic (ANS-) Fluorescent Probes. Journal of Agricultural and Food Chemistry 1998, 46, 2671–2677. DOI: 10.1021/jf970876y.
- Lowry, O. H.; Rosebrough, N. A.; Farr, A. L.; Randall, R. J. Protein Measurement with Folin Phenol Reagent. Journal of Biological Chemistry 1951, 193, 265–275.
- Tsumura, K.; Saito, T.; Kugimiya, W.; Inouye, K. Selective Proteolysis of the Glycinin and β-conglycinin Fractions in a Soy Protein Isolate by Pepsin and Papain with Controlled pH and Temperature. Journal of Food Science 2004, 69, 363–367. DOI: 10.1111/j.1365-2621.2004.tb10698.x.
- Harkouss, R.; Astruc, T.; Lebert, A.; Gatellier, P.; Loison, O.; Safa, H.; Portanguen, S.; Parafita, E.; Mirade, P. Quantitative Study of the Relationships among Proteolysis, Lipid Oxidation, Structure and Texture Throughout the Dry-Cured Ham Process. Food Chemistry 2015, 166, 522–530. DOI: 10.1016/j.foodchem.2014.06.013.
- Yin, S. W.; Tang, C. H.; Wen, Q. B.; Yang, X. Q. Effects of Acylation on the Functional Properties an in Vitro Trypsin Digestibility of Red Kidney Bean (Phaseolus Vulgaris L.) Protein Isolate. Journal of Food Science 2009, 74, 488–492. DOI: 10.1111/j.1750-3841.2009.01349.x.
- Yin, S. W.; Tang, C. H.; Wen, Q. B.; Yang, X. Q. Functional and Structural Properties and in Vitro Digestibility of Acylated Hemp (Cannabis Sativa L.) Protein Isolates. International Journal of Food Science and Technology 2009, 44, 2653–2661. DOI: 10.1111/j.1365-2621.2009.02098.x.
- Achouri, A.; Zhang, W. Effect of Succinylation on the Physicochemical Properties of Soy Protein Hydrolysate. Food Research International 2001, 34, 507–514. DOI: 10.1016/S0963-9969(01)00063-1.
- Yang, M.; Yang, J.; Zhang, Y.; Zhang, W. Influence of Succinylation on Physicochemical Property of Yak Casein Micelles. Food Chemistry 2016, 190, 836–842. DOI: 10.1016/j.foodchem.2015.06.030.
- Shilpashree, B. G.; Arora, S.; Sharma, V.; Chawla, P.; Vakkalagadda, R. Changes in Physicochemical and Functional Properties of Whey Protein Concentrate upon Succinylation. International Journal of Dairy Technology 2016, 70, 137–145. DOI: 10.1111/1471-0307.12321.
- Lawal, O. S.; Adebowale, K. O. The Acylated Protein Derivatives of Canavalia Ensiformis (Jack Bean): A Study of Functional Characteristics. LWT-Food Science and Technology 2006, 39, 918–929. DOI: 10.1016/j.lwt.2005.06.016.
- Krause, J. P.;. Comparison of the Effect of Acylation and Phosphorylation on Surface Pressure, Surface Potential and Foaming Properties of Protein Isolates from Rapeseed (Brassica Napus). International Crops and Products 2002, 15, 221–228. DOI: 10.1016/S0926-6690(01)00117-0.
- Zaghloul, M.; Prakash, V. Effect of Succinylation on the Functional and Physicochemical Properties of α-globulin, the Major Protein Fraction from Sesamum Indicum L. Nahrung/Food 2002, 46, 364–369. DOI: 10.1002/1521-3803(20020901)46:5<364::AID-FOOD364>3.0.CO;2-3.
- Damodaran, S.; Paraf, A. Food Proteins and Their Applications; CRC Press: New York, 1997