ABSTRACT
In this study, the antioxidant, carbohydrate metabolism, cholinergic, monoaminergic, and purinergic enzyme activities of ethyl acetate leaf fraction of Cnidoscolus aconitifolius were evaluated with the high-performance liquid chromatography (HPLC) analysis. The ethyl acetate fraction of the plant leaf was tested for antioxidant properties, key carbohydrate metabolism, cholinergic, monoaminergic, and purinergic enzyme activities using standard procedures, while the chemical composition of the fraction was evaluated using HPLC. The results revealed that the fraction has higher phenolic compounds than flavonoid and exhibited the ability to scavenge iron chelation and ABTS. The fraction also inhibited the activities of α-amylase and α-glucosidase, acetylcholinesterase, butyrylcholinesterase, monoamine oxidase, tyrosinase, arginase, Ecto-5’-nucleotidase, phosphodieterase-5, angiotensin-I-converting enzyme and encouraged the activity of Na+/K+-ATPase. The HPLC analysis revealed that the ethyl acetate fraction contained coumaric acid, amentoflavone, hesperidin, protocatechuic acid, kaempferol, dihydromyricetin, quercetin,and rutin. The obtained results in this study suggest that the ethyl acetate fraction obtained from aqueous extracts of Cnidoscolus aconitifolius leaf possessed outstanding antioxidative potentials. Thus, excellent enzyme inhibitory activities probably due to bioactive compounds are also observed in the leaf.
Introduction
Diabetes mellitus, especially type II is a lingering disease with increasing fasting blood glucose level (hyperglycaemia).[Citation1] Presently, more than 422 million people globally are suffering from diabetes mellitus, particularly type II diabetes mellitus, and it is anticipated to reach 642 million by 2040 if necessary actions are not taken.[Citation2,Citation3] Added to this, diabetes mellitus is rated as the sixth leading cause of death.[Citation4] One of the features of type II diabetes mellitus is non-insulin-dependent hyperglycaemia.[Citation5] Fujisawa et al.[Citation6] have documented that persistent hyperglycaemia might induce production of free radical mainly as reactive oxygen species (ROS). This may trigger the destruction of pancreatic beta cell, an insulin-secreting organ, and couple with the stimulation of all key pathways essential for diverse mechanisms of chronic vascular diabetes mellitus complications like glycation and sorbitol pathways amongst others.[Citation7,Citation8] Furthermore, persistent hyperglycaemia may trigger neurodegenerative diseases, erectile dysfunction, hypertension, etc. Moreover, several studies have suggested that there is a negative correlation between the consumption of the plant products and the incidence of some chronic diseases such as atherosclerosis, Alzheimer’s diseases, and diabetes mellitus.[Citation9]
Adefegha et al.[Citation10] described neurodegenerative diseases as a multifactorial disease, associated with loss in memory, poor learning habit, cognitive dysfunction, neuronal death, and brain damage. The pathogenesis of neurodegenerative diseases are linked to continuous hyperglycaemia which may promote oxidative stress, defeat in neuronal function, reduction of neurotransmitters, alterations in protein expression, and protein aggregation amongst others as reported by Uttara et al.[Citation11] In another vein, erection of penile is known as neurovascular event, depending on neural integrity, functional ability of vascular system, and healthy cavernosal tissue.[Citation12] This process required relaxation of the corpus cavernosum smooth muscles and vasodilation of the arterioles in the penis as reported by Kandeel et al.[Citation13] This encourages increase in pressure of blood flow and expansion of the sinusoidal spaces of the tissue with enlargement of penis. Persistent hyperglycaemia, which induces oxidative stress, could trigger alterations in the penile vasculature may trigger the impairment of the erection process which leads to the development of erectile dysfunction.[Citation14] This is one of the secondary problem associated with men suffering from diabetes mellitus globally, especially at chronic stage.
Several bioactive compounds have been documented and isolated with medicinal properties from herbal products. For instance, phenolic compounds are documented as responsible for the antioxidant activities of plants. These phytochemicals have been reported to be beneficial in the management of a plethora of neurodegenerative diseases, diabetes mellitus, and cardiovascular diseases.[Citation15,Citation16] In addition to these, food additive such as saffron is also known in natural medicine for improving memory and learning abilities and for the treatment of sickness such as spasm, asthma, bronchitis, fever, colds, and cardiovascular diseases; furthermore, it also possesses anticancer, antioxidant, hypolipemic, and anticonvulsant activities.[Citation17,Citation18]
Hence, numerous pharmacological methods have been used in the management and/or treatment of these diseases but are characterized with series of side effects. Moreover, these are expensive and not even available in the rural areas. Therefore, one of the main focus of the scientist globally is to develop alternative means of treatments/management of these diseases with no or little side effects. This includes application of functional foods. Africa, especially in Nigeria, is a country endowed with series of functional foods. Example of such plant is Cnidoscolus aconitifolius (family Euphorbiaceae), usually called Chaya. This plant is also called spinach tree and is consumed as vegetable soups and salads across Nigeria. Cnidoscolus aconitifolius is a plant with medicinal benefits for different diseases such as diabetes mellitus, atherosclerosis, hypertension, etc., as reported by Kuti and Torres.[Citation19] The plant is a rich source of protein, vitamins, calcium, iron, and in vitro antioxidants.[Citation20] The aqueous leaf extract of Cnidoscolus aconitifolius has been documented by Obichi et al.[Citation21] on some antioxidant enzymes and haematological parameters of high-fat diet and streptozotocin-induced diabetic Wistar albino rats. Hence, there is scanty information on ethyl acetate fraction of the plant leaf. Therefore, the present study was designed to focus on inhibitory activities of key enzymes on carbohydrate metabolism, cholinergic, monoaminergic, purinergic, and chemical fingerprinting of ethyl acetate fraction of Cnidoscolus aconitifolius leaves.
Materials and methods
Plant source and authentication
Cnidoscolus aconitifolius leaf was acquired from Basiri, Ado-Ekiti, Ekiti State, Nigeria on February 20, 2018. This was then identified and authenticated by a Senior taxonomist at Forestry Research Institute of Nigeria, Ibadan, Nigeria.
Chemicals and plant material
All chemicals and reagents were procured from Sigma-Aldrich, Inc., (St Louis, MO, USA). The obtained Cnidoscolus aconitifolius leaves were washed using distilled water and air-dried for 20 days to a constant weight. Then, the air-dried leaves were pounded to a fine powder with the aid of a kitchen blender and stored in an airtight container for subsequent analyses. Thereafter, 100 g of the powdered sample was soaked in 1000 mL of distilled water for 24 h with intermittent shaking. It was filtered and dried using a water bath at 50°C. The obtained yield was 41.14 g. This was stored inside the fridge using a universal bottle.[Citation22]
Preparation of ethyl acetate fraction
Five grams of the obtained yield was suspended in 500 mL of distilled water and successively partitioned with n-hexane (3 x 200 mL), ethyl acetate (3 x 200 mL), and n-butanol (3 x 200 mL). Rotary evaporator was used to dry the fractions at 40°C. The fractions were weighed separately, transferred into universal bottles, and stored at 4°C for subsequent analysis.[Citation23] However, ethyl acetate fraction was the most potent from the preliminary assays carried out by the authors.
Determination of total phenolic content
An aliquot (1.0 mL) of appropriately diluted ethyl acetate fraction of Cnidoscolus aconitifolius was mixed with 0.5 mL 0.5 N Folin–Ciocalteu reagent, and the reaction was neutralized with saturated sodium carbonate (75 g/L). After incubation for 2 h at 25°C, the absorbance of the resulting mixture was read using a spectrophotometer. Total phenolic content of the fraction was expressed as milligrams of gallic acid equivalent (mg GAE) per 100 g of dry weight.[Citation24]
Determination of total flavonoid
The ethyl acetate fraction of Cnidoscolus aconitifolius (0.5 mg/mL), methanol (500 µL), 50 µL of 10% aluminum chloride (AlCl3), 50 µL of 1 M potassium acetate, and 1.4 mL of distilled water was incubated at 25°C for 30 min. Thereafter, the absorbance of the reaction mixture was read at 415 nm and the total flavonoid content calculated as quercetin equivalent (mg GAE) per 100 g of dry weight.[Citation25]
Determination of metal ion chelating radical scavenging activity
The freshly prepared 500 μM FeSO4 (150 μL) was added to a reaction mixture containing 168 μL 0.1 M Tris-HCl (pH 7.4), 218 μL saline, and 25 μL of different concentrations of the fraction. The reaction mixture was incubated for 5 min, before the addition of 13 μL 0.25% 1,10-phenanthroline (w/v). Also, ethylenediaminetetraacetic acid (EDTA) was used as a standard. The absorbance was subsequently read at 510 nm with the aid of the visible spectrophotometer.[Citation26]
Determination of 2,2-azino-bis3-ethylbenthiazoline-6-sulphonic acid (ABTS) radical scavenging activity
ABTS cation radical was fashioned by the reaction between 7 mM ABTS in water and 2.45 mM potassium persulphate (1:1), which was stored in the dark place at room temperature for 12–16 h before use. ABTS+ solution was then diluted with methanol to get an absorbance of 0.700 at 734 nm. After the addition of 15 μL of ethyl acetate fraction of Cnidoscolus aconitifolius to 3.995 mL of diluted ABTS solution, the absorbance was read at 30 min after the initial mixing. The percentage inhibition of ABTS by the ethyl acetate fraction was calculated and compared with gallic acid as described by Re et al.[Citation27]
Determination of α-amylase inhibitory activity
α-Amylase inhibitory activity of ethyl acetate fraction of Cnidoscolus aconitifolius was determined according to the method described by Shai et al.[Citation28] with slight modifications. A 250 µL of the fraction or acarbose at different concentrations was incubated with 500 µL of porcine pancreatic amylase (2 U/mL) in 100 mM phosphate buffer (pH 6.8) at 37°C for 20 min. Thereafter, 250 µL of 1% starch dissolved in 100 mM phosphate buffer (pH 6.8) was then added to the reaction mixture and incubated for 1 h at 37°C. Then, 1 mL of dinitrosalicylic acid (DNS) was added and boiled for 10 min. The absorbance of the resulting mixture was measured at 540 nm.
Determination of α-glucosidase inhibitory activity
α-Glucosidase inhibitory activity of ethyl acetate fraction of Cnidoscolus aconitifolius was determined according to the method of Ademiluyi and Oboh[Citation29] with slight modifications. Briefly, 250 µL solution of ethyl acetate fraction of Cnidoscolus aconitifolius or acarbose at different concentrations was incubated with 500 µL of 1.0 U/mL α -glucosidase solution in 100 mM phosphate buffer (pH 6.8) at 37°C for 15 min. Thereafter, a 250 µL of pNPG solution (5 mM) in 100 mM phosphate buffer (pH 6.8) was added. The reaction mixture was incubated further for 20 min at 37°C. The absorbance of the released p-nitrophenol was measured at 405 nm.
Handling of experimental animals
Ten 10–14-weeks-old male adult Wistar albino rats weighing between 250 and 300 g were obtained from animal house of Afe Babalola University, Ado-Ekiti, and were handled in accordance with the Guide for the Care and Use of Laboratory Animals formulated by ABUAD Animal Ethical Committee with approval number ABUAD/SCI/ BCH/150. The rats were allowed to acclimatize for 2 weeks and maintained at room temperature under laboratory conditions of 12-h light/dark cycle with access to standard animal feed and water ad libitum. The handling of the animals was in accordance with ABUAD Ethical Animal Committee.
Preparation of tissues homogenate
The male albino rats were decapitated using halothane as an anesthesia and rapidly dissected. The brain and penile tissues were removed and placed on ice and weighed. These tissues were homogenized subsequently in cold saline (1/10 w/v) with mortar and pestle. Thereafter, each homogenate was centrifuged for 10 min at 3000 ×g to yield a pellet that was discarded and a low-speed supernatant (S1) that was kept for assay.[Citation30]
Determination of acetylcholinesterase and butyrylcholinesterase inhibitory activities
The inhibitory activities of ethyl acetate fraction of Cnidoscolus aconitifolius on acetylcholinesterase (AChE) and butyrylcholinesterase (BChE) activities were determined using the Ellman’s method described by Perry et al.[Citation31] A mixture containing 0.1 M phosphate buffer with pH 8.0, 200 μL of brain tissue homogenate as the source of AChE in 100 μL of a solution of 5,5-dithio-bis (2-nitrobenzoic) acid (DTNB 3.3mM), ethyl acetate fraction of Cnidoscolus aconitifolius at different concentrations, and 500 μL of phosphate buffer with pH 8.0 was incubated at 25°C for 20 min,and 100 μL of 0.05 mM solution of the substrate (acetylthiocholine iodide) was added. AChE activity was determined with a UV spectrophotometer from the changes in absorbance at 412 nm for 3 min at room temperature. This same procedure was used to determine BChE activity using butyrylthiocholine iodide as substrate.
Determination of monoamine oxidase inhibitory activity
The mixture for the reaction contained 0.025 M phosphate buffer of pH 7, 12.5 mM semicarbazide, 10 mM benzylamine (pH adjusted to 7), and 75 μL of enzyme (monoamine oxidase, MOA) and different concentrations of ethyl acetate fraction of Cnidoscolus aconitifolius dissolved in water. Thirty minutes thereafter, 250 μL of acetic acid was added and boiled for 3 min in a water bath followed by centrifugation. The resulting supernatant (1 mL) was mixed with an equal volume of 0.05% of 2,4-dinitrophenylhydrazine (DNPH), and 1.25 mL of benzene was added after 10 min and incubated at room temperature. The benzene layer was separated and mixed with the same volume of sodium hydroxide (1 N). The alkaline layer was decanted and heated for 10 min at 80°C. The orange–yellow colour formed was measured at 450 nm with the aid of a UV-spectrophotometer.[Citation32]
Determination of tyrosinase inhibitory activity
The assay was performed according to the protocol described by Macrini et al.[Citation33] with some modifications. Aliquot of 10 µL of the solution composed of 125 U/mL of mushroom tyrosinase was added to 96-well microplates. Then 70 µL of pH 6.8 phosphate buffer solution, 60 µL of ethyl acetate fraction of Cnidoscolus aconitifolius leaves, and 350 µg/mL (in 25% dimethyl sulphoxide) were also added. To the mixture, 70 µL of L-tyrosine was added at a concentration of 0.3 mg/mL in distilled water (with a final volume of 210 µL). The absorbance of the microplate wells was thereafter read using a microplate spectrophotometer at 510 nm (T0). Then, the microplate was incubated at 30°C for 60 min and the absorbance was measured again (T1). An additional incubation period of 60 min at 30°C was done, and after this period, a new spectrophotometric reading was taken (T2).
Determination of arginase inhibitory activity
The arginase activity was estimated by the measurement of urea produced by the reaction of Ehrlich’s reagent. The solution contained the following concentration: 1.0 mM Tris-HCl buffer (pH 9.5), 1.0 mM MnCl, 0.1 M arginine solution, appropriate diluted ethyl acetate fraction of Cnidoscolus aconitifolius, and 50 mM of the penile homogenate. The solution was made to a final volume of 1.0 mL. The mixture was thereafter incubated for 10 min at 37°C. The reaction was ended by adding 2.5 mL of Ehrlich's reagent (2.0 g of p-dimethyl aminobenzaldehyde in 20 mL of concentrated hydrochloric acid and made up to 100 mL with distilled water). Hence, the absorbance was read at 450 nm after 20 min of incubation at room temperature. The control experiment was performed without the test sample, and the arginase inhibitory activity was expressed as percentage inhibition.[Citation34]
Determination of purinergic enzymes
Na+/K+ATPase activity
The Na+/K+ ATPase activity was performed according to the modified method described by Wyse et al.[Citation35] The assay mixture consisted of 100 mL of Na+/K+ ATPase substrate buffer at pH of 7.4 (containing 30 Mm Tris–HCl, 0.1 mM EDTA, 50 mM NaCl, 5 mM KCl, 6 mM MgCl2), 50 µL of ethyl acetate fraction of Cnidoscolus aconitifolius, and 50 µL of brain homogenates in the presence or absence of 50 µL of ouabain (1 mM), with a final volume of 200 µL. The reaction was initiated by the addition of 50 µL of 12 mM adenosine triphosphate (ATP) after incubating for 30 min at 37°C. The reaction was terminated by the addition of 70 µL of 50% (w/v) trichloroacetic acid. The amount of inorganic phosphate (Pi) released was quantified and Na+/K+ ATPase activity was reported in nmol Pi released/min/mg protein as documented by.[Citation36]
Determination of Ecto-5΄-nucleotidase inhibitory activity
Twenty microliters of the brain homogenate were incubated with ethyl acetate fraction of Cnidoscolus aconitifolius (12.5–50 µg/mL) dissolved in water. The solution was added to a mixture of 10 mM MgSO4 and 100 mM Tris–HCl buffer at pH 7.5. The solution was incubated for 10 min at 37°C. Adenosine monophosphate (AMP) was added to the mixture to reach a final concentration of 2.0 mM. After 20 min, 200 µL of tricholoroacetic (10%) acid was added to the mixture to stop the reaction. The tubes containing the solution were placed on ice for 10 min.[Citation37] Fiske and Subbarow's method [Citation36] was used to measure the Pi that was released. Percentage inhibition was used to express enzyme inhibitory activity.
Determination of phosphodiesterase-5 inhibitory
The reaction mixture containing 5 mM of the substrate (p-nitrophenyl phenyl phosphonate), penile homogenate, 20 mM Tris buffer (pH 8.0), and ethyl acetate fraction of Cnidoscolus aconitifolius was incubated for 10 min at 37°C. The intensity of p-nitrophenol created was measured as a change in absorbance at 400 nm after 5 min. The control experiment was performed without the extract.[Citation30]
Determination of angiotensin-I-converting enzyme inhibitory activity
The inhibition of the angiotensin-I-converting enzyme (ACE) activity of the extract was determined according to the protocol described by Cushman and Cheung.[Citation38] Different concentrations of ethyl acetate fraction of Cnidoscolus aconitifolius and 50 µL of penile homogenate as a source of ACE (4 mU/mL) were pre-incubated at 37°C for 15 min. Thereafter, the enzymatic reaction was started by adding 150 µL of 8.33 mM ACE substrate [hippuryl-l-histidyl-l-leucine (HHL)] in 125 mM of Tris-HCl buffer (pH 8.3) to the solution and incubated at 37°C for 30 min. The reaction was stopped by adding 250 µL of 1M HCl. The hippuric acid (Bz-Gly) produced by the reaction was extracted with 1.5 mL of ethyl acetate. The mixture was then centrifuged to separate the ethyl acetate layer, after which the 1 mL of the ethyl acetate layer was moved to a clean test tube and evaporated to dryness. The residue was redissolved in distilled water, and its absorbance was read at 228 nm. The control experiment was performed without the test sample.
Identification of compounds by high-performance liquid chromatography coupled with diode array detector
Reversed-phase chromatographic analyses were performed under gradient conditions apex octadecyl 104 using C18 column (4.6 mm × 250 mm) packed with 5-μm diameter particles. The mobile phase with a flow rate of 0.8 mL/min consisted of a mixture of solvents: A (water/formic acid, 98:2% v/v) and B (methanol/water/formic acid, 70:28:2% v/v). The following gradient elution program was used: 0–3 min: 0% B; 3–25 min: 30% B; 25–43 min: 50% B; 43–55 min: 60% B; 55–60 min: 80% B; 60–65 min: 50% B; and 65– 69 min: 0% B. Appropriate detection wavelengths were used for detection of different compounds in the extract. Ethyl acetate fraction of Cnidoscolus aconitifolius (12 mg/mL) and mobile phase were filtered through a 0.45-mm membrane filter (Millipore) and then degassed by ultrasonic bath prior to use. Stock solutions of standards were prepared in the high-performance liquid chromatography mobile phase (Shimadzu, Kyoto, Japan) in the concentration range of 0.030–0.500 mg/mL. Chromatographic peaks were assigned by comparing their retention times with those of reference standards and by comparison of DAD spectra (200–600 nm). All chromatographic runs were performed at ambient temperature and in triplicate.[Citation39]
Data analysis
The results of triplicate readings were pooled and expressed as mean ± standard error of the mean (SEM). One-way analysis of variance was used to analyze the mean. Post hoc treatment was performed using Duncan multiple test. Significance was accepted at p ≤ 0. 05. Also, IC50 (extract concentration causing 50% enzyme/antioxidant activity) was determined using non-linear regression analysis [Citation40].
Results
The total phenol and flavonoid contents of ethyl acetate fraction of Cnidoscolus aconitifolius are 28.18 mg GAE/100g and 20.49 mg QUE/ 100 g, respectively, as indicated in . The phenolic profile of ethyl acetate fraction of Cnidoscolus aconitifolius as shown in and revealed the following compounds: coumaric acid, amentoflavone, hesperidin, protocatechuic acid, kaempferol, dihydromyricetin, quercetin, and rutin. The most abundant compound is quercetin with 6.943 mg/g, while rutin is the least with 0.233 mg/g.
Table 1. Total phenolic and total flavonoid content of ethyl acetate fraction of Cnidoscolus aconitifolius leaves.
Table 2. Phenolic constituents of ethyl acetate fraction of Cnidoscolus aconitifolius leaves.
Figure 1. Representative high-performance liquid chromatography profile of ethyl acetate fraction of Cnidoscolus aconitifolius leaves: coumaric acid (peak 1), amentoflavone (peak 2), hesperidin (peak 3), protocatechuic acid (peak 4), kaempferol (peak 5), dihydromyricetin (peak 6), quercitrin (peak 7), and rutin (peak 8).
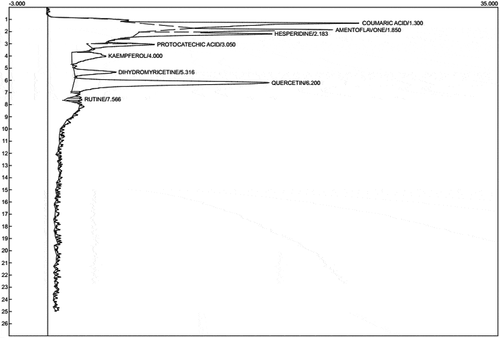
As the concentration increased, the metal ion chelating radical scavenging activity of ethyl acetate fraction of Cnidoscolus aconitifolius also increased with IC50 of 23.11 µg/mL. EDTA)was used as a standard () with IC50 of 17.51 µg/mL. The same trend was also observed in ABTS radical scavenging activity of ethyl acetate fraction of Cnidoscolus aconitifolius with IC50 of 14.14 µg/mL. Gallic acid was used as a standard with IC50 of 19.50 µg/mL ().
Figure 2. Metal ion chelating radical scavenging ability of ethyl acetate fraction of Cnidoscolus aconitifolius leaves. Values are represented as mean ± standard error of the mean (SEM) of triplicate experiment.
Legend: EAFCA: ethyl acetate fraction of Cnidoscolus aconitifolius leaves; EDTA: ethylenediaminetetraaceticacid.
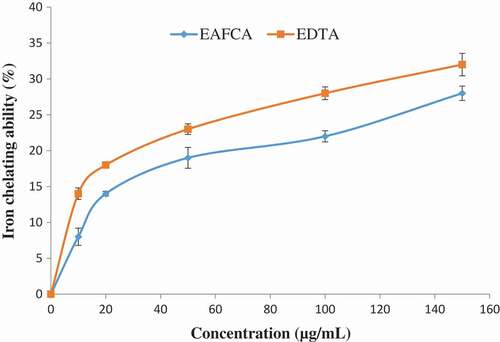
Figure 3. 2,2-Azino-bis3-ethylbenthiazoline-6-sulphonic acid (ABTS) radical scavenging ability of ethyl acetate fraction of Cnidoscolus aconitifolius leaves. Values are represented as mean ± standard error of the mean (SEM) of triplicate experiment.
Legend: EAFCA: ethyl acetate fraction of Cnidoscolus aconitifolius leaves.
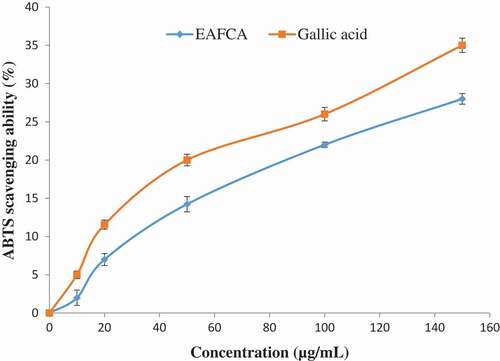
and show the percentage inhibitions of α-amylase and α-glucosidase by ethyl acetate fraction of Cnidoscolus aconitifolius and acarbose (standard drug used). As the concentration increased, there was a significant increase (p < 0.05) in both the fraction and acarbose inhibitory activity of α-amylase and α-glucosidase. The ethyl acetate fraction of Cnidoscolus aconitifolius had higher inhibitory activity against α-amylase and α-glucosidase with IC50 of 13.85 and 18.98 µg/mL compared to the acarbose with IC50 of 17.52 and 24.51 µg/mL, respectively.
Figure 4. α-Amylase inhibitory potential of ethyl acetate fraction of Cnidoscolus aconitifolius leaves. Values are represented as mean ± standard error of the mean (SEM) of triplicate experiment.
Legend: EAFCA: ethyl acetate fraction of Cnidoscolus aconitifolius leaves.
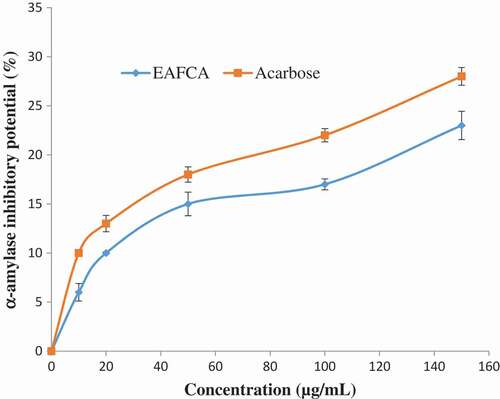
Figure 5. α-Glucosidase inhibitory potential of ethyl acetate fraction of Cnidoscolus aconitifolius leaves. Values are represented as mean ± standard error of the mean (SEM) of triplicate experiment.
Legend: EAFCA: ethyl acetate fraction of Cnidoscolus aconitifolius leaves.
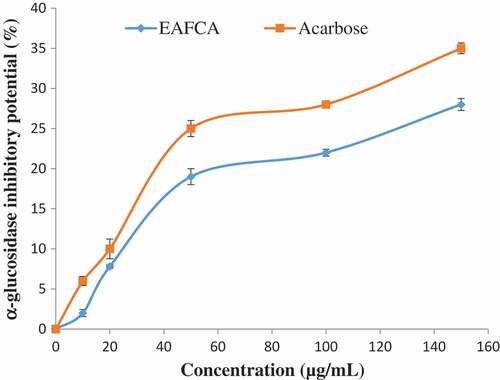
The effect of ethyl acetate fraction of Cnidoscolus aconitifolius on AChE and BChE activities in vitro was investigated and the results are presented in and respectively. Ethyl acetate fraction of Cnidoscolus aconitifolius exhibited higher BChE inhibition with IC50 of 56.25 µg/mL than AChE inhibition with IC50 of 61.13 µg/ mL. The inhibition of MAO activity displayed by ethyl acetate fraction of Cnidoscolus aconitifolius as presented in . The activity increased with increasing in extract concentration with IC50 of 2.56 µg/mL.
Figure 6. Acetylcholinesterase (AChE) inhibitory potential of ethyl acetate fraction of Cnidoscolus aconitifolius leaves. Values are represented as mean ± standard error of the mean (SEM) of triplicate experiment.
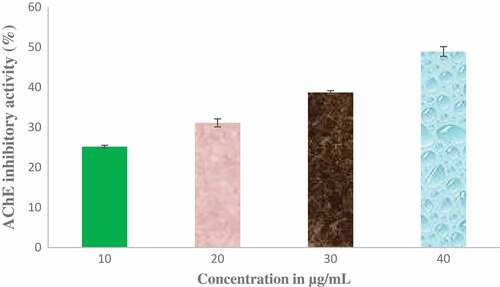
Figure 7. Butyrylcholinesterase (BChE) inhibitory potential of ethyl acetate fraction of Cnidoscolus aconitifolius leaves. Values are represented as mean ± standard error of the mean (SEM) of triplicate experiment.
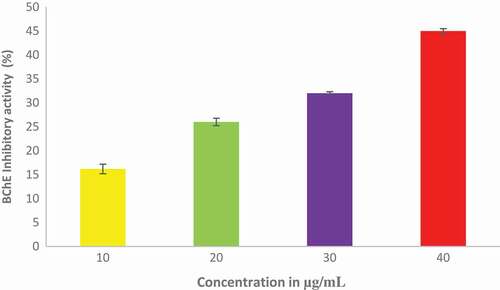
Figure 8. Inhibitory effect of ethyl acetate fraction of Cnidoscolus aconitifolius leaves on monoamine oxidase (MAO) activity. Values are represented as mean ± standard error of the mean (SEM) of triplicate experiment.
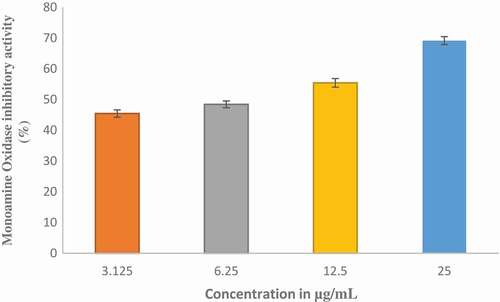
The ethyl acetate fraction of Cnidoscolus aconitifolius leaves also exhibited tyrosinase inhibitory activity (). shows the effect of ethyl acetate fraction of Cnidoscolus aconitifolius leaves on arginase activity. At high concentration, the inhibitory effect on arginase activity was also high. However, N-(omega)-hydroxyl-1-arginine (L-NOHA) (standard drug used) showed more inhibitory ability (IC50 of 24.22 µg/mL) than the fraction (IC50 of 27.56 µg/mL).
Figure 9. Inhibitory effect of ethyl acetate fraction of Cnidoscolus aconitifolius leaves on tyrosinase activity. Values are represented as mean ± standard error of the mean (SEM) of triplicate experiment.
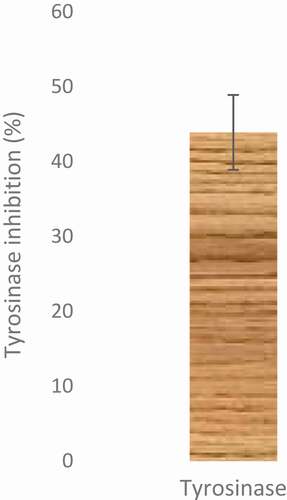
Figure 10. Inhibitory effect of ethyl acetate fraction of Cnidoscolus aconitifolius leaves on arginase activity. Values are represented as mean ± standard error of the mean (SEM) of triplicate experiment.
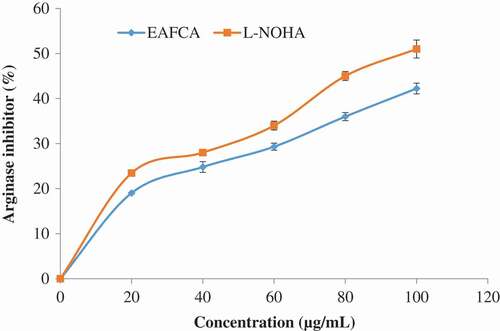
reveals the modulatory effect of ethyl acetate fraction of Cnidoscolus aconitifolius leaves on Na+/K+-ATPase activity. The result shows that ethyl acetate fraction of Cnidoscolus aconitifolius leaves increased Na+/K+-ATPase activity with an increase in fraction concentration, therefore, causing 50% stimulatory effect of 3.05 µg/mL. Furthermore, – show the inhibitory potential of ethyl acetate fraction of Cnidoscolus aconitifolius leaves on Ecto-5-nucleotidase (E-NTDase), phosphodiesterase-5 (PDE-5), and ACE activities. There was a significant increase in these enzymes activity as the ethyl acetate fraction of Cnidoscolus aconitifolius leaves' concentrations increased with IC50 of 1.57, 22.51, and 56.33 µg/mL, respectively.
Discussion
According to Oboh et al.,[Citation30] phenolic compounds have been described with several pharmacological properties, which includes antidiabetic, anti-erectile dysfunction and neuroprotective amongst others. They are useful as neuroprotective compounds because polyphenols are proficient in crossing the blood–brain barrier and improve neurotransmission, inhibit protein aggregation, prevent neuronal death, etc., as reported by Perry et al.[Citation31] Ojo et al.[Citation41] also reported that phenolic compounds are useful agents in the development of new nutraceuticals or pharmaceuticals products. Hence, the high phenolic content of the fraction () may be useful in the management of diabetes mellitus, Alzheimer’s disease, hypertension, and erectile dysfunction, etc.. Flavonoids are also major classes of phenolics, which are important in ameliorating or controlling diseases.[Citation42] The observed compounds in ethyl acetate fraction of Cnidoscolus aconitifolius leaves are coumaric acid, amentoflavone, hesperidin, protocatechuic, kaempferol, dihydromyricetin, quercitrin, and rutin ( and ). A previous study has demonstrated the roles of some of these compounds in different diseases.[Citation43]
Sarkar et al.[Citation44] reported that iron increases the production of ROS, which induces the initiation of lipid peroxidation. In the Fenton reaction, iron II (Fe2+) reacts with H2O2 to produce highly reactive hydroxyl radical. This can cause damage to proteins, lipids, and nucleic acids. In this study, ethyl acetate fraction of Cnidoscolus aconitifolius leaves was able to chelate Fe2+ (), although in a concentration-dependent manner. This might be due to the phenolic nature of the fraction (). These phenolic compounds can produce a complex with iron, so making it unavailable for stimulating the formation of reactive radicals. Therefore, Fe2+ chelating ability of ethyl acetate fraction of Cnidoscolus aconitifolius leaves could be helpful in the management/prevention of diseases such as erectile dysfunction, Alzheimer’s disease, and diabetes mellitus amongst others. This concurs with the earlier report by Oboh et al.[Citation30] Also, ABTS scavenging property of the fraction in dose-dependent manner () could be due to antioxidant nature of phenolic compounds in the plant ().
Furthermore, the main therapeutic approach for managing hyperglycaemia in diabetes mellitus patients is by delaying glucose absorption through inhibition of two key carbohydrate metabolism enzymes (α-amylase and α-glucosidase).[Citation45,Citation46] These enzymes are present in the mouth and small intestine.[Citation47] In this study, ethyl acetate fraction of Cnidoscolus aconitifolius leaves was able to inhibit α-amylase and α-glucosidase activities in a dose-dependent manner ( and ). The inhibition of carbohydrate-metabolizing enzymes by the ethyl acetate fraction of Cnidoscolus aconitifolius leaves suggests its efficacy as antidiabetic agent by prolonging the absorption time of glucose, thereby reducing the level of ingested glucose, hence retard postprandial hyperglycaemia. This finding is in agreement with the report of Ojo et al.[Citation23]
Ethyl acetate fraction of Cnidoscolus aconitifolius leaves demonstrated inhibitory effects on AChE and BChE activities ( to ). Oboh et al.[Citation48] documented that cholinesterases are significant in regulating neurotransmission through hydrolysis of acetylcholine (ACh), an important neurotransmitter in cholinergic neurons. The brain of Alzheimer's disease patient’s is characterized by an increased in the activity of cholinesterases. This reduces the accessibility of ACh, causing cholinergic deficit and memory impairment.[Citation41,Citation48] The inhibition of AChE and BChE exhibited by ethyl acetate fraction of Cnidoscolus aconitifolius leaves could attenuate cholinergic dysfunction, which could be attributed to phenolic nature of the fraction such as coumaric acid, amentoflavone, hesperidin, protocatechuic, kaempferol, dihydromyricetin, quercitrin, and rutin. These compounds can compete with cholinesterases substrate and blocking the enzymes active site as reported by Nordberg et al.[Citation49] Therefore, ethyl acetate fraction of Cnidoscolus aconitifolius leaves may be useful in the management of Alzheimer's disease.
The inhibition of MOA is an important biomarker for the management of Alzheimer’s diseases, and the ability of ethyl acetate fraction of Cnidoscolus aconitifolius leaves to inhibit this enzyme () is an indication of therapeutic likely in the management of Alzheimer’s diseases. This decrease in MOA activity by the fraction may trigger an increase in the level of amine neurotransmitters like dopamine and serotonin and prevent degradation of amine by ROS.[Citation50] This inhibition could be linked to the phenolic compounds in the plant fraction, due to their structural similarities with synthetic MOA inhibitors as reported by Benamar et al.[Citation51], which corroborate the therapeutic activity of the plant.
Neurodegenerative diseases in humans are partly caused by dopamine deficiency as reported by Meiser et al.[Citation52] Tyrosinase catalyses the conversion of L-tyrosine to L-DOPA, then converted to dopamine by DOPA decarboxylase in the nervous system. The ability of the fraction to inhibit the activity of tyrosinase () could be linked to antioxidant nature of the phenolic compounds present in the plant (), with the tendency to compete with the substrate when binding to the active center of the enzyme. Tyrosinase is one of the crucial enzymes for production of dopamine, and ethyl acetate fraction of Cnidoscolus aconitifolius leaves might be a likely drug that can be used for the treatment/management of neurodegenerative diseases (e.g., Alzheimer’s disease).
According to Bivalacqua et al.[Citation12], increased arginase activity is a characteristic of diabetic penile corpus cavernosum which signifies impairment in erectile response. Conversely, decreased arginase activity in patients with erectile dysfunction has been associated with vaso-relaxation of corpus cavernosum smooth muscle by Cox et al.[Citation53] Thus, inhibition of arginase activity could be a useful approach in the treatment/management of patients with erectile dysfunction globally. This finding observed that ethyl acetate fraction of Cnidoscolus aconitifolius leaves inhibited arginase activity in a dose-dependent manner (). This could be linked to phenolic compounds identified in the fraction. This is in agreement with the report by Akomolafe et al.[Citation54]
The obtained results also reveal that ethyl acetate fraction of Cnidoscolus aconitifolius leaves increased Na+/K+-ATPase activity in brain homogenates in a dose-dependent manner (). Oboh et al.[Citation55] reported that decreased activity of Na+/K+-ATPase might impair learning and memory, which induces neuronal hyperexcitability and neuronal death. Na+/K+-ATPase plays an important role in the regulation of cell membrane potential and neuronal excitability by pumping Na+ and K+ against the electrochemical gradient across the cell membrane as reported by Wyse et al.[Citation56] Hence, the ability of the ethyl acetate fraction of Cnidoscolus aconitifolius leaves to increase the activity of Na+/K+-ATPase might improve learning and memory and prevent neuronal dysfunction. This implies that ethyl acetate fraction of Cnidoscolus aconitifolius leaves may serve as a useful means of managing/or treating Alzheimer’s disease, which may be attributed to the phenolic compounds present in the fraction.
Neurodegenerative diseases have been characterized by abnormal increase in E-NTDase linked with neuro-inflammation and neuro-immune reactions as documented by Burnstock.[Citation57] This enzyme is involved in purinergic transmission and nucleotide-mediated signaling by regulating hydrolysis of AMP to adenosine and Pi. Hence, alteration of E-NTDase activity can trigger an increase in the level of adenosine in the synaptic cleft that may interrupt the release of other neurotransmitters like ACh, dopamine, glutamate, epinephrine, and noradrenaline.[Citation30] In this study, ethyl acetate fraction of Cnidoscolus aconitifolius leaves demonstrated the ability to inhibit the activity of E-NTDase in a dose-dependent manner (), which encourages the availability of adenosine for synaptic cleft, since adenosine is useful in protecting the brain against neuronal dysfunction. The inhibitory effects exhibited by the fraction could be associated with the phenolic constituents present in the leaves.
Boswell-Smith et al.[Citation58] reported that inhibition of PDE-5 is essential for penile erectile process. It catalyzes the breakdown of the cyclic guanosine monophosphate (cGMP) and also reduces the levels of nitric oxide (NO) in the endothelial cells, so decreasing signalling.[Citation58] PDE-5 inhibitors (sidenafil, tadalafil, and verdanafil) usually increase the level of cGMP, stimulates activation of cGMP as well as increases NO bioavailability for the relaxation of penile tissue for penile erection, but they are characterized with series of side effects. The result revealed that PDE-5 activity was inhibited by the ethyl acetate fraction of Cnidoscolus aconitifolius leaves concentration in dose-dependent manner (). This finding supports the previous report by Shin et al.[Citation59] The inhibitory ability of the fraction could be due to the phenolic composition of the plant leaves (), which implies that ethyl acetate fraction of Cnidoscolus aconitifolius leaves could be used as the therapeutic agent in the management and treatment of erectile dysfunction in humans.
Figure 13. Inhibitory effect of ethyl acetate fraction of Cnidoscolus aconitifolius leaves on PDE-5 activity. Values are represented as mean ± standard error of the mean (SEM) of triplicate experiment.
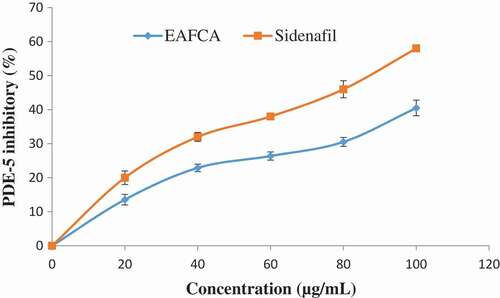
According to Akomolafe et al.,[Citation60] continuous increase in blood pressure (hypertension) has been described as one of the causes of erectile dysfunction coupled with angiotensin-II. Thus, inhibition of angiotensin-II secretion by ACE inhibition could be a source of therapeutic target in the management/treatment of hypertension and hypertensive-induced erectile dysfunction. Also, the inhibition of ACE activates the release of NO as well as bradykinin (active compound in erectile function process) as reported by Akomolafe et al.[Citation60] Thus, the inhibition of ACE activity by ethyl acetate fraction of Cnidoscolus aconitifolius leaves in the present study () could be linked to interaction between the phenolics compounds present in the plant with disulphide bridge of the enzyme in the active site of the enzyme as reported by Ademiluyi et al.[Citation61] This is in line with earlier report by Akomolafe et al.[Citation60]
Conclusion
Ethyl acetate fraction of Cnidoscolus aconitifolius leaves demonstrates the ability to scavenge metal ion chelation and ABTS radical scavenging. The fraction also inhibits the activities of α-amylase and α-glucosidase, cholinesterases, MOA, tyrosinase, arginase, sodium/potassium ATPase, E-NTDase, PDE-5, and ACE in a dose-dependent manner. These may be attributed to the phenolic compounds found in the fraction. This study suggests that ethyl acetate fraction of Cnidoscolus aconitifolius leaves shows encouraging potential for the management/or treatment of diabetes mellitus, neurodegenerative diseases, and erectile dysfunction.
Conflict of interest
The authors declare no conflict of interest.
Additional information
Funding
References
- Ajiboye, B. O.; Ojo, O. A.; Adeyonu, O.; Imiere, O.; Oyinloye, B. E.; Ogunmodede, O. Ameliorative Activity of Ethanolic Extract of Artocarpus Heterophyllus Stem Bark on Alloxan-Induced Diabetic Rats. Advancement Pharmaceutical Bulletin 2018, 8(1), 141–147. DOI: 10.15171/apb.2018.017.
- Srinivasan, K.; Viswanad, B.; Lydia, A.; Kaul, C. L.; Ramarao, P. Combination of High- Fat Diet-Fed and Low-Dose Streptozotocin Treated Rat: A Model for Type 2 Diabetes and Pharmacological Screening. Pharmacology Researcher 2005, 52, 313–320.
- Quilliot, D.; Walter, E.; Bonte, J. P.; Fruchart, J. C.; Duriez, P.; Ziegler, O. Diabetes Mellitus Worsens Antioxidant Status in Patients with Chronic Pancreatitis. The American Journal of Clinical Nutrition 2005, 81, 1111–1117.
- Wiernsperger, N. F.;. Oxidative Stress as a Therapeutic Target in Diabetes: Revisiting the Controversy. Diabetes & Metabolism 2003, 29, 579–585.
- Neuser, D.; Benson, A.; Bruckner, R.; Goldberg, B.; Hoogwerf, J.; Petzinna, D. Safety and Tolerability of Acarbose in the Treatment of Type 1 and Type 2 Diabetes Mellitus. Clinical Drug Invest 2005, 25, 579−587.
- Fujisawa, T.; Ikegami, H.; Inoue, K.; Kawabata, Y.; Ogihara, T. Effect of Two α-glucosidase Inhibitors, Voglibose and Acarbose, on Postprandial Hyperglycemia Correlates with Subjective Abdominal Symptoms. Metabolism 2005, 54, 387−390.
- Ibrahim, M. A.; Koorbanally, N. A.; Islam, M. S. Antioxidative Activity and Inhibition of Key Enzymes Linked to Type-2 Diabetes (α-glucosidase and α-amylase) by Khaya Senegalensis. Acta Pharmaceutica (Zagreb, Croatia) 2014, 64, 311–324.
- Ajiboye, B. O.; Ojo, O. A.; Adeyonu, O.; Imiere, O.; Fadaka, A.; Osukoya, A. Ameliorative Activity of Ethanolic Extract of Artocarpus Heterophyllus Stem Bark on Pancreatic Beta-Cell Dysfunction in Alloxan-Induced Diabetic Rats. Journal of Evidence-Based Complementary and Alternative Medicine 2017, 22, 4, 538–543.
- Uysal, A.; Zengin, G.; Mollica, A.; Gunes, E.; Locatelli, M.; Yilmaz, T.; Aktumsek, A. Chemical and Biological Insights on Cotoneaster Integerrimus: A New (-)- Epicatechin Source for Food and Medicinal Applications. Phytomedicine 2016. DOI: 10.1016/j.phymed.2016.06.011.
- Adefegha, S. A.; Oboh, G.; Olasehinde, T. A. Alkaloid Extracts from Shea Butter and Breadfruit as Potential Inhibitors of Monoamine Oxidase, Cholinesterases, and Lipid Peroxidation in Rats’ Brain Homogenates: A Comparative Study. Comparative Clinical Pathology 2016, 25, 1213–1219.
- Uttara, B.; Singh, A. V.; Zamboni, P.; Mahajan, R. T. Oxidative Stress and Neurodegenerative Diseases: A Review of Upstream and Downstream Antioxidant Therapeutic Options. Current Neuropharm 2009, 7, 65–74.
- Bivalacqua, T. J.; Usta, M. F.; Champion, H. C.; Kadowitz, P. J.; Hellstrom, W. J. Endothelial Dysfunction in Erectile Dysfunction: Role of the Endothelium in Erectile Physiology and Disease. Journal of Andrology 2003, 24, 17–37.
- Kandeel, F. R.; Koussa, V. K.; Swerdloff, R. S. Male Sexual Function and Its Disorders: Physiology, Pathophysiology, Clinical Investigation and Treatment. Endocrine Reviews 2001, 22, 342–388.
- Andersson, K. E.;. Erectile Physiological and Pathophysiological Pathways Involved in Erectile Dysfunction. The Journal of Urology 2003, 170, 6–14.
- Gonzalez, R.; Ballester, I.; Lopez-Posadas, R.; Suarez, M.; Zarzuelo, A.; Martinez-Augustin, O.; Medina, F. S. D. Effects of Flavonoids and Other Polyphenols on Inflammation. Critical Reviews in Food Science and Nutrition 2011, 51, 4, 331–362.
- Zengin, G.; Uysalb, A.; Aktumseka, A.; Mocan, A.; Mollicad, A.; Locatellid, M.; Custodioe, L.; Nengf, R.; Nogueiraf, J. M. F.; Aumeeruddy-Elalfig, Z.; et al. Euphorbia Denticulata Lam.: A Promising Source of Phyto-Pharmaceuticals for the Development of Novel Functional Formulations. Biomedicine and Pharmacotherapy 2017, 87, 27–36.
- Bathaie, S. Z.; Mousavi, S. Z. New Applications and Mechanisms of Action of Saffron and Its Important Ingredients. Critical Reviews in Food Science and Nutrition 2010, 50, 761–786.
- Monte, C. D.; Bizzarri, B.; Gidaro, M. C.; Carradori, S.; Mollica, A.; Luisi, G.; Granese, A.; Alcaro, S.; Costa, G.; Basilico, N.; et al. Bioactive Compounds of Crocus sativus L. and Their Semi-Synthetic Derivatives as Promising Anti-Helicobacter pylori, Anti-Malarial and Anti-Leishmanial Agents. Journal of Enzyme Inhibition and Medicinal Chemistry 2015, 30(6), 1027-1033.
- Kuti, J. O.; Torres, E. S. Potential Nutritional and Health Benefits of Tree Spinach. In Progress in New Crops; Janick, J., Ed.; ASHS Press: Arlington, VA, 1996. pp 516–520.
- Kuti, J. O.; Konuru, H. B. Antioxidant Capacity and Phenolic Content in Leaf Extracts of Tree Spinach (Cnidoscolus Spp.). Journal Agricultural Food Chemical 2004, 52, 1, 117–121.
- Obichi, E. A.; Monago, C. C.; Belonwu, D. C. Effect of Cnidoscolus Aconitifolius (Family Euphorbiaceae) Aqueous Leaf Extract on Some Antioxidant Enzymes and Haematological Susceptibility of Tetranychus Urticae Koch to an Ethanol Extract of Cnidoscolus Aconitifolius Leaves under Laboratory Conditions Parameters of High Fat Diet and Streptozotocin Induced Diabetic Wistar Albino Rats. Journal Applications Sciences Environment Managed 2015, 19, 2, 201–209.
- Ojo, O. A.; Ajiboye, B. O.; Debbie, I. O.; Adeyonu, O.; Olayide, I.; Fadaka, A. O. Antioxidative Properties of Blighia Sapida K.D. Koenig Stem Bark Extract and Inhibitory Effects on Carbohydrate Hydrolyzing Enzymes Associated with Non-Insulin Dependent Diabetes Mellitus. Pharmacognosy Journal 2018a, 10, 376–383.
- Ojo, O. A.; Ojo, A. B.; Ajiboye, B. O.; Olaiya, O.; Akawa, A.; Olaoye, O.; Anifowose, O. O.; Idowu, O.; Olasehinde, O.; Obafemi, T.; et al. Inhibitory Effect of Bryophyllum Pinnatum (Lam.) Oken Leaf Extract and Their Fractions on α-amylase, α-glucosidase and Cholinesterase Enzyme. Pharmacognosy Journal 2018b, 10, 3, 497–506.
- Kim, D. O.; Jeong, S. W.; Lee, C. Y. Antioxidant Capacity of Phenolic Phytochemicals from Various Cultivars of Plums. Food Chemistry 2003, 81, 321–326.
- Meda, A.; Lamien, C. E.; Romito, M.; Millogo, J.; Nacoulma, O. G. Determination of the Total Phenolic, Flavonoid and Proline Contents in Burkina Fasan Honey, as Well as Their Radical Scavenging Activity. Food Chemistry 2005, 91, 571–577.
- Minotti, G.; Aust, S. D. An Investigation into the Mechanism of citrate-Fe2þ-dependent Lipid Peroxidation. Free Rad Biologic Medica 1987, 3, 379–387.
- Re, R.; Pellegrini, N.; Proteggente, A.; Pannala, A.; Yang, M.; Rice-Evans, C. Antioxidant Activity Applying an Improved ABTS Radical Cation Decolorization Assay. Free Radical Biologic Medica 1999, 26, 1231–1237.
- Shai, L. J.; Masoko, P.; Mokgotho, M. P.; Magano, S. R.; Mogale, A. M.; Boaduo, N.; Eloff, J. N. Yeast Alpha Glucosidase Inhibitory and Antioxidant Activities of Six Medicinal Plants Collected in Phalaborwa, South Africa. Southern African Journal Botanic 2010, 76, 465–470.
- Ademiluyi, A. O.; Oboh, G. Health Benefits of Traditional Corn, Beans, and Pumpkin: In Vitro Studies for Hyperglycemia and Hypertension Management. Journal of Medicinal Food 2013, 16, 88–93.
- Oboh, G.; Adewuni, T. M.; Ademiluyi, A. O.; Olasehinde, T. A.; Ademosun, A. O. Phenolic Constituents and Inhibitory Effects of Hibiscus Sabdariffa L. (Sorrel) Calyx on Cholinergic, Monoaminergic, and Purinergic Enzyme Activities. Journal of Dietary Supplements 2017. DOI: 10.1080/19390211.2017.1406426.
- Perry, N. S.; Houghton, P. J.; Theobald, A.; Jenner, P.; Perry, E. K. In-Vitro Inhibition of Human Erythrocyte Acetylcholinesterase by Salvia Lavandula Efolia Essential Oil and Constituent Terpenes. Journal Pharmaceutical 2000, 52, 895–902.
- Green, A. L.; Haughton, T. M. A. Colorimetric Method for the Estimation of Monoamine Oxidase. Biochemical Journal 1961, 78, 172–176.
- Macrini, D. J.; Suffredini, I. V.; Varella, A. D.; Ohara, M. T. Extract from Amazonian Plants Have Inhibitory Activity against Tyroxinase: An Invitro Evaluation. Brazilian Journal of Pharmaceutical Science 2009, 45, 4.
- Adefegha, S. A.; Oboh, G.; Ejakpovi, I. I.; Oyeleye, S. I. Antioxidant and Antidiabetic Effects of Gallic and Protocatechuic Acids: A Structure–Function Perspective. Comparative Clinical Pathologists 2015, 24, 1579–1585.
- Wyse, A. T.; Streck, E. L.; Worm, P.; Wajner, A.; Ritter, F.; Netto, C. A. Preconditioning Prevents the Inhibition of Na+/K+ -Atpase Activity after Brain Ischemia. Neurochem Researcher 2000, 25, 969–973.
- Fiske, C. H.; Subbarow, Y. The Colorimetric Determination of Phosphorous. Journal of Biological Chemistry 1925, 66, 375–400.
- Heymann, D.; Reddington, M.; Kreutzberg, G. W. Subcellular Localization of 5- Nucleotidase in Rat Brain. Journal Neurochem 1984, 43, 971–978.
- Cushman, D. W.; Cheung, H. S. Spectrophotometric Assay Band Properties of the Angiotensin-1-Converting Enzyme of Rabbit Lung. Biochemical Pharmacology 1971, 20, 1637–1684.
- Ojo, O. A.; Ojo, A. B.; Ajiboye, B. O.; Oyinloye, B. E.; Akinyemi, A. J.; Okesola, M. A.; Boligon, A. A.; De Campos, M. M. Chromatographic Fingerprint Analysis, Antioxidant Properties, and Inhibition of Cholinergic Enzymes (Acetylcholinesterase and Butyrylcholinesterase) of Phenolic Extracts from Irvingia Gabonensis (Aubry-Lecomte Ex O’Rorke) Baill Bark. Journal of Basic and Clinical Physiology and Pharmacology 2018c, 29, 2, 217–224.
- Zar, J. H. Biostatistical analysis. Prentice-Hall, Upper Saddle River,NJ; 1984, pp.620.
- Ojo, O. A.; Ojo, A. B.; Ajiboye, B. O.; Olaiya, O.; Okesola, M. A.; Boligon, A. A.; De Campos, M. M. A.; Oyinloye, B. E.; Kappo, A. P. HPLC-DAD Fingerprinting Analysis, Antioxidant Activities of Tithonia Diversifolia (Hemsl.) A. Gray Leaves and Its Inhibition of Key Enzymes Linked to Alzheimer’s Disease. Toxicology Reports 2018d, 5, 585–592.
- Ojo, O. A.; Ajiboye, B. O.; Ojo, A. B.; Olayide, I. I.; Akinyemi, A. J.; Fadaka, A. O.; Adedeji, E. A.; Boligon, A. A.; De Campos, M. M. HPLC-DAD Fingerprinting Analysis, Antioxidant Activity of Phenolic Extracts from Blighia Sapida Bark and Its Inhibition of Cholinergic Enzymes Linked to Alzheimer’s Disease. Jordan Journal of Biological Sciences 2017, 10, 4, 257–264.
- Olasehinde, T. A.; Olaniran, A. O.; Okoh, A. I. Therapeutic Potentials of Microalgae in the Treatment of Alzheimer’s Disease. Molecules 2017, 22, 3, 1–18.
- Sarkar, R.; Hazra, B.; Mandal, N. Hepatoprotective Potential of Caesalpinia Crista against Iron-Overload-Induced Liver Toxicity in Mice. Evidence-Based Compl Altitude Medica 2012, 2012, Article ID896341. 9.
- Ajiboye, B. O.; Ojo, O. A.; Adeyonu, O.; Imiere, O.; Olayide, I.; Fadaka, A.; Oyinloye, B. E. Inhibitory Effect of Key Enzymes Relevant to Acute Type-2-Diabetes and Antioxidative Activity of Ethanolic Extract of Artocarpus Heterophyllus Stem Bark. Journal Acute Diseases 2016, 5, 423–429.
- Ojo, O. A.; Afon, A. A.; Ojo, A. B.; Ajiboye, B. O.; Oyinloye, B. E.; Kappo, A. P. Inhibitory Effects of Solvent-Partitioned Fractions of Two Nigerian Herbs (Spondias Mombin Linn. And Mangifera Indica L.) On α-Amylase and α-Glucosidase. Antioxidants 2018e, 7, 6, 73.
- Mohamed, E. L. H.; Siddiqui, M. J. A.; Ang, L. F.; Sadikun, A.; Chan, S. H.; Tan, S. C.; Asmawi, M. Z.; Yam, M. F. Potent α-glucosidase and α-amylase Inhibitory Activities of Standardized 50% Ethanolic Extracts and Sinensetin from Orthosiphonstamineus Benth as Anti-Diabetic Mechanism. BMC Complementary Alternative Medica 2012, 12, 176.
- Oboh, G.; Olasehinde, T. A.; Ademosun, A. O. Essential Oil from Lemon Peels Inhibit Key Enzymes Linked to Neurodegenerative Conditions and Pro-Oxidant Induced Lipid Peroxidation. Journal Oleo Sciences 2014, 63, 373–381.
- Nordberg, A.; Ballard, C.; Bullock, R.; Darreh-Shori, T.; Somogyi, M. A. Review of Butyrylcholinesterase as a Therapeutic Target in the Treatment of Alzheimer’s Disease. The Pry Care Companion for CNS Disorder 2013, 15, 12r01412.
- Thomas, T.;. Monoamine oxidase-B Inhibitors in the Treatment of Alzheimers Disease. Neurobiology of Aging 2000, 21, 343–348.
- Benamar, H.; Rached, W.; Derdour, A.; Marouf, A. Screening of Algerian Medicinal Plants for Acetylcholinesterase Inhibitory Activity. The Journal of Biological Sciences 2010, 10, 1–9.
- Meiser, J.; Weindl, D.; Hiller, K. Complexity of Dopamine Metabolism. Cell Communication and Signalling 2013, 11, 34.
- Cox, J. D.; Kim, N. N.; Traish, A. M.; Christianson, D. W. Arginaseboronic Acid Complex Highlights a Physiological Role in Erectile Function. Nature Structural & Molecular Biology 1999, 6, 1043–1047.
- Akomolafe, S.; Oboh, G.; Olasehinde, T.; Ogunsuyi, O.; Oyeleye, S. Modulatory Effects of Aqueous Extract from Tetracarpidium Conophorum Leaves on Key Enzymes Linked to Erectile Dysfunction and Oxidative Stress-Induced Lipid Peroxidation in Penile and Testicular Tissues. Journal App Pharmaceutical Sciences 2017, 7, 1, 051–056.
- Oboh, G.; Adewuni, T. M.; Ademosun, A. O.; Olasehinde, T. A. Sorghum Stem Extract Modulates Na+/K+-ATPase, Ecto-5-Nucleotidase, and Acetylcholinesterase Activities. Comparative Clinical Path 2016, 25, 749–756.
- Wyse, A. T.; Bavaresco, C. S.; Reis, E. A.; Zugno, A. I.; Tagliari, B.; Calcagnotto, T.; Netto, C. A. Training in Inhibitory Avoidance Causes a Reduction of Na+/K+-ATPase Activity in Rat Hippocampus. Physics Behavior 2004, 80, 475–479.
- Burnstock, G.;. Purinergic Signalling and Disorders of the Central Nervous System. Nature Review in Drug Discov 2008, 7, 575–590.
- Boswell-Smith, V.; Spina, D.; Page, C. P. Phosphodiesterase Inhibitors. British Journal of Pharmacology 2006, 147, 252–257.
- Shin, Y. S.; Zhao, C.; Zhang, L. T.; Park, J. K. Current Status and Clinical Studies of Oriental Herbs in Sexual Medicine in Korea. The World Journal of Men’s Health 2015, 33, 62–72.
- Akomolafe, S. F.; Oboh, G.; Oyeleye, S. I.; Boligon, A. A. Aqueous Extract from Ficus Capensis Leaves Inhibits Key Enzymes Linked to Erectile Dysfunction and Prevent Oxidative Stress in Rats’ Penile Tissue. NFS Journal 2016, 4, 15–21.
- Ademiluyi, A. O.; Oyeleye, S. I.; Oboh, G. Biological Activities, Antioxidant Properties and Phytoconstituents of Essential Oil from Sweet Basil (Ocimum basilicum L.) Leaves. Comparative Clinical Pathologists 2015, 25, 169–1768.