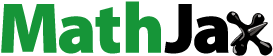
ABSTRACT
1,3-Dioleoyl-2-palmitoylglycerol (OPO) is used widely as a food additive. However, the structured lipid is sensitive to oxygen and light in the production process. This results in the loss of its original nutrition and production of harmful substances. In this study, the OPO was microencapsulated using whey protein isolate and maltodextrin as the wall material and monostearin as an emulsifier by spray-drying technology added to the infant formula milk, in order to improve its oxidative stability. The OPO microcapsules were released in vitro through the simulated gastrointestinal tract; sensory evaluation of microencapsulated OPO in infant formula powder and nutrition absorption of microencapsulated OPO in infant formula power by animal experiments were investigated. The results showed that OPO microcapsules have a slow-release effect; after 2 h of simulated gastric fluid digestion, only 16.1 ± 3.2% of the oil was released from microencapsulated OPO, and after another 2 h of simulated intestinal fluid digestion, there was 92.3 ± 2.8% of oil released from the microencapsulated OPO. The infant formula with microencapsulated OPO has a uniform colour and no odour. The quality of infant formula with microencapsulated OPO was obviously better than infant formula with OPO by storage test. Everted mice gut sac experiments confirmed that microencapsulation did not affect absorption of mice to OPO in infant formula and prevented the loss of calcium. The study confirmed that addition of microencapsulated OPO makes infant formula more efficient for product quality and nutrition absorption.
Introduction
Breast milk is the ideal choice of food for infants in their early life. The fat contained in milk, as much as 2–6%, provides 50–60% of the total energy needed by infants, and triacylglycerols (TAGs) in the total fat are approximately 98%.[Citation1] In breast milk, the main component of milk TAGs is 1,3-dioleoyl-2-palmitoylglycerol (OPO). Sixty-eight per cent of palmitic acid and 44.8% of fatty acids are located at Sn-2 position; however, only 10.3% of palmitic acid content is located at Sn-1,3 positions besides 62.6% unsaturated fatty acids and 30.7% saturated fatty acids.[Citation2]
In traditional infant formula, certain formulations from vegetable oils or dairy products have similar fat content to fatty acids in breast milk but differ in lipid structure, and palmitic acid of the TAG from the vegetable oil is located at Sn-1,3 positions.[Citation1] Palmitic acid at Sn-1,3 position will be hydrolysed by the enzyme lipase in the intestine into free fatty acids. These free fatty acids would bind calcium in the intestinal tract and changed to insoluble “calcium soaps”, which would cause the loss of calcium, resulting in bone mineralization and constipation that can affect the health of infants.[Citation3] However, palmitic acid at Sn-2 position in the TAG can reduce the formation of insoluble calcium soaps. Therefore, the position of palmitic acids in the TAG is important for the process of absorption of fat and calcium.[Citation4]
Microencapsulation is widely applied in retaining solid, liquid, or gaseous materials within an edible membrane, and the microcapsules can release their contents sealed at controlled rates under specific conditions.[Citation5,Citation6] Microencapsulation has been found to be an effective method to improve the quality of oil products. Bakry et al.[Citation7] researched the microencapsulation process prepared by whey protein isolate (WPI) and carboxymethyl cellulose (CMC) or pullulan and evaluated physicochemical characteristics and oxidative stability of microencapsulated peppermint oil and tuna oil. Anwar and Kunz[Citation8] investigated the stability of microencapsulated fish oil prepared by spray granulation, spray drying, and freeze drying, and the oil produced from the spray granulation process provided maximum protection for the oil droplets.
At present, the study of microencapsulated OPO is less reported. In this study, the OPO was microencapsulated using WPI and maltodextrin as the wall material and monostearin as an emulsifier by spray-drying technology added to the infant formulas milk, in order to improve its oxidative stability. The main subjects of the study were shown as follows: (1) investigating the structured lipid microcapsules in vitro released through the gastrointestinal simulation experiment, (2) determining the sensory indicators of the structured lipid microcapsules in infant formula power, and (3) examining the fat and calcium absorption of structured lipid microcapsules in infant formula power in vivo.
Materials and methods
Chemicals and enzymes
OPO used in this work was purchased from Yitong Food Industry Co., Ltd. (Xuzhou, China). Whey protein was supplied from Marquez Manufacturing Group (USA). Pancreatic lipase, pepsin, and trypsin were obtained from Gibco Co. (Baii Tech. Co., Shanghai, China).
Preparation of microencapsulated OPO
The microencapsulated process of OPO
In this study, whey protein and maltodextrin were used as the wall material and monoglyceride was used as an emulsifier; microencapsulated OPO was prepared by spray drying, and the schematic process flow diagram of the microencapsulation is shown in .
The whey protein and maltodextrin (in a ratio of 2:1, w:w) were dissolved in the distilled water at 50–60 °C. The mixture was kept at 60°C for 20 min with constant stirring. OPO (core-wall, 1:2, w:w) and 0.3% of monoglyceride were added to the mixture of whey protein and maltodextrin and constantly stirred for 10 min. The sample with 25% of solid content was treated by a homogenizing process with 25 Mpa homogeneous pressure. The sample was dried by spray drying (Buchi Mini Spray Dryer B-290, BUCHI, Sweden) with inlet air temperature at 184°C and outlet air temperature at 89°C.
In-vitro gastric and intestinal digestions of microencapsulated OPO
Simulated gastric juice was comprised of 9 g/L NaCl and 5 g/L pepsin and adjusted the pH to 2.5 with HCl[Citation9] with slight modification. Simulated intestinal juice was prepared using bile saltsintestinal solution (3.58 g/L Na2HPO4 · 12H2O, 1.58 g/L NaH2PO4 · 2H2O, and 10 g/L trypsin) at pH 7.0 to final concentrations of 2% (w/v). One hundred milligram of microencapsulated OPO was added to 100 ml simulated gastric juice and incubated at 37°C, 100 rpm (simulated gastrointestinal motility) for 2 h,[Citation10] adjusted the pH to 7.0 with 0.1 mol/L NaOH, and then mixed with 100 ml simulated intestinal juice for 2 h digestion. During this period, NaOH was continuously added to maintain the pH of the system at 7.0, and samples were taken periodically to record the amount of NaOH consumed at different times.[Citation11,Citation12]
Preparation of infant formula powder
A base formulation was prepared according to the nutrient specifications for an infant.[Citation13] Fat in milk powder was replaced with soybean oil, OPO, and microencapsulated OPO, respectively. After being mixed, samples were heated to 70–75°C and homogenized at 180 bar. The concentrates were then heated to 95°C by a heater, cooled to 70°C, and spray-dried.
Sensory evaluation of milk powder with microencapsulated OPO was followed as per the recommendations of the International Dairy Federation (IDF)[Citation14] with some modifications.[Citation15,Citation16] Thirty assessors were used for sensory evaluation of milk powder samples. A 0-to 10-point scale was used for four sensory attributes (overall impression, frankincense, colour, and reconstituability) with 0 being unacceptable and 10 being excellent; ≥5 indicated an acceptable sample. A 5-point scale was used for fishy and defined 0 as extremely fishy, 2 as acceptable, and 5 as no fishy. Milk powder with microencapsulated OPO, milk powder with OPO, and milk powder with soybean oil were analysed. The assessors evaluated the milk powder placed in coded dishes in independent space by consumer acceptance test based on these sensory attributes after milk powder kept at 60°C for 30-day storage.
Fatty acid analysis
Hydrolysis of triglycerides
OPO sample of 0.1 g was placed in a 10-ml centrifuge tube. Twenty milligrams of lipase and 2 ml of Tris-base buffer solution (pH 7.6) were added and shaken carefully. Then, 0.5 ml of sodium cholate solution (1 g/L) and 0.2 ml of calcium chloride solution (220 g/L) were added to the mixture. After shaking carefully, the tube was placed in the water bath set at 40°C, maintaining manual shaking for 60 ± 2 s. The tube was removed from the water bath and shaken vigorously at 40°C for 120 ± 2 s using the shaker. Each 1 ml of HCl and diethyl ether was added immediately to the tube and shaken vigorously using the shaker. The mixture in the tube was centrifuged at 4000 rpm for 4 min, and the organic phase was transferred to another tube; 200 μl of the extract was collected by blowing nitrogen for analysis.[Citation17]
Isolation of fatty acid at the Sn-2
Isolation of fatty acid at the Sn-2 was carried out by the International Organization for Standardization (ISO) 6800 standard.[Citation17] The extract was transferred onto a prepared plate as a continuous band of fine droplets 15 mm from one of the edges using a microsyringe. Stand the plate in the developing tank, which has previously been saturated with the developing solvent (light petroleum-–diethyl ether–formic acid, 70:30:1, v:v:v). Develop the plate at a temperature of 20°C. Dry the plate in air at 20°C and spray it with the indicator solution (1% of 2,7-dichlorofluorescein) using the apparatus. Mark the band of monoglyceride (Rf = 0.02), 1,2-diacylglycerol (Rf = 0.22), 1,3-diacylglycerol (Rf = 0.31), fatty acid (Rf = 0.02), and triglyceride (Rf = 0.69) under ultraviolet light and scrape off using a microspatula. The collected silica was transferred into diethyl ether solution and shaken vigorously. Then, evaporate the solvent in a stream of nitrogen. The methyl esters of the fatty acids for the monoglycerides extracted from the silica prior to gas chromatography analysis were prepared.
Total fatty acid composition analyses
The lipids were extracted with chloroform/methanol/water as previously described.[Citation18] The developed method of fatty acid methyl esters (FAME) was carried out. The lipid extracts were incubated in boron trifluoride/methanol at 70°C for 10 min. One millilitre of hexane was used to extract the samples. Undecanoic acid methyl ester (Sigma Aldrich) is as an internal standard. After being decanted for 5 min, the upper phase was separated to analyse.
Gas chromatography (GC) system was used to perform the analysis of FAME in hexane (Agilent Technologies 6890, Hewlett-Packard, Avondale, PA) equipped with a split/splitless injector. The flow rate of nitrogen as the carrier gas was 128 ml/min. One microlitre of samples was injected, and it was done in a split ratio of 1:50. The temperature was 150°C and held for 5 min and increased at a rate of 4°C/min to 220°C and held for 30 min. Temperature of injection was 250°C. Temperature of detection was 280°C.
Bioavailability studies in mice
Experimental groups
Animal experiments referred to the published paper with minor modification.[Citation19] Four-week-old Sprague-Dawley (SD) mice (90 ± 10 g) were purchased from the Harbin Veterinary Research Institute (Harbin, China). The animals were adapted to the surroundings for one week before the experiments. The temperature was 25 ± 2°C and relative humidity was 55 ± 5% alongside a 12/12 h light/dark cycle. All animal experiments complied with the rules of the Care and Use of Laboratory Animals of Northeast Agricultural University. Mice were randomly divided into three groups; experimental groups designed are shown in . The blood, faeces, and small intestine were subsequently collected for nutrition absorption analysis in mice.
Table 1. Experimental groups of mice.
Nutrition absorption analysis
The mice were housed and fed in individual plastic cages for 2 weeks. Body weight and food consumption were recorded every day. The mice’s faeces were collected in the last three days of the feeding experiment and frozen at −20°C until further analysis. At the end of the experiment, mice were fasted for 12 h and then anaesthetized with diethyl ether. All mice were decapitated and the blood was collected in sodium heparin-coated plastic tubes and centrifuged (4000 rpm for 15 min at 4°C). All blood samples were stored at −80°C until further analysis.
Faeces were dried by freeze dryer (CHRIST, Alpha 1–2/LD plus, Osterode, Germany) for 24 h. Faeces were then milled by food mill FW100 (TAISITE Instrument Co., Ltd, Tianjin, China) for 30 s. Faeces lipid was extracted from the faecal powder by the Bligh–Dyer method[Citation20] with acid hydrolysis using 3 mol/L HCl for 2 h at 80°C. Blood lipid was extracted by exchanging the NaCl solution with 1 mol/L HCl and methylated with BF3.[Citation21] Methylated fatty acids were extracted, separated, and quantified by GC. Calcium and magnesium in the faeces were determined by flame atomic absorption spectrometry. Absorption rate was calculated approximatively on the base of the ratio of fat metabolism and excretion of mice.
Everted mice gut sac experimental operation referred to the described method.[Citation22] Mice were sacrificed, then the abdominal area was shaved, and subsequently, the abdominal cavity was cut open with a midline incision about 2–3 cm. Carefully separate the small intestine and place quickly in code vessel with ice-cold Krebs-Ringer’s solution (7.8 g NaCl, 0.35 KCl, 1.37 g NaHCO3, 0.02 g MgCl2, 0.32 g NaH2PO4 and 1.48 g glucose, and 1000 ml distilled water). Rinsed the small intestines immediately with ice-cold Krebs-Ringer’s solution to clean intestinal contents and get rid of the underlying mesenterium. Ten centimetre length of the small intestine was tied at one end, then evert with a smooth glass rod, and removed any mucus present. Then, the serosa compartment was injected with 1 ml of Krebs-Ringer’s solution. At time equal to zero, the everted intestine sac was suspenin 25 ml of sample solution (containing OPO, microencapsulated OPO, and soybean oil). The sample solution was continually treated with the rate of CO2 and O2 (5:95, %) and kept at 37°C in a water bath. After being kept for 30 min, aliquots of 1 ml serosal solution were taken for fatty acid analysis according to the total fatty acid composition method in this study.
Statistical analysis
The SPSS software 19.0 was used to perform all statistical analyses. All data were expressed as the mean ± SD. P < 0.05 was used to indicate the significant differences.
Results and discussion
Composition of fatty acid in OPO
The total fatty acid composition and fatty acid distribution at the Sn-2 position of OPO in this study and breast milk are presented in . For fatty acids of breast milk,[Citation2] most C16:0 was located at Sn-2 position (44.8% of total fatty acids and 68% of total C16:0); however, the C16:0 content at the Sn-1,3 position was low (10.3%). At Sn-1,3 position, 62.6% were unsaturated fatty acids (such as C18:1 and C18:2), and only 30.7% were saturated fatty acids (such as C12:0, C14:0, C16:0, and C18:0). For OPO sample in this study, 72% of total C16:0 and 50.05% of total fatty acids at this position were located at Sn-2 position, and only 9.62% of C16:0 content were located at the Sn-1,3 position; 75.47% of fatty acids were unsaturated fatty acids at Sn-1,3 position and 16.49% are saturated fatty acids. Thus, the key dominant section of OPO sample in this study was OPO.
Table 2. Fatty acid composition of OPO and human milk fat (%).
There are lots of evidence that indicate that the absorbed and transported ability of OPO is more effective than other oils, such as vegetable and milchig oils which were commonly used in infant products, containing palmitic acid located predominantly at the Sn-1,3 position.[Citation23–Citation25] Therefore, the application of OPO with most unsaturated fatty acids at the Sn-1,3 position meets the specific purpose of infant nutrition.
In-vitro gastric and intestinal digestions of microencapsulated OPO
The encapsulating technology is commonly used to stabilize, solubilize, and release active ingredients that are sensitive to circumstance factors. The microencapsulation can minimize volume or weight and improve storage and transportation conditions compared with the form of liquid or gel.[Citation26] Whey proteins are widely used for the microencapsulation processes due to their natures, such as better encapsulants, low cost, and availability.[Citation27,Citation28] In this work, the microencapsulated OPO was prepared using WPI and maltodextrin as wall material and monostearin as emulsifier by spray-drying technology. Bakry et al.[Citation7] also successfully microencapsulated tuna oil using WPI as emulsifier and CMC or amylopectin as stabilizers with better oxidative stability.
In-vitro digestion was performed to assess the potential for delivery by oral administration of food to targeted delivery of biologically active compounds to intestinal microencapsulated oil.[Citation11] The digestive lipids and their uptake mainly occur in the small intestine.[Citation29] Hence, it is crucial to control the release of microencapsulated oil to prevent any degradation before the oil enters the small intestine.
The results indicated that only 16.1 ± 3.2% of the oil was released from microencapsulated OPO after 2 h of simulated gastric digestion. The results showed that microcapsules coated by WPI and maltodextrin have greater resistance to the stomach environment and the protected bioactive components when passed through stomach environment. Subsequently, there was 92.3 ± 2.8% of oil released from microencapsulated OPO after another 2 h of intestinal digestion. The amount of microencapsulated oil released from simulated intestinal juice was significantly (P < 0.05) higher than that in the simulated gastric juice, indicating that the wall materials used effectively improved the bioaccessibility of OPO. Lim and Nyam[Citation30] also found the similar results when they researched microencapsulated kenaf seed oil with wall materials, such as soy lecithin, carboxymethyl cellulose, and maltodextrin during digestion.
Sensory evaluation
The OPO, microencapsulated OPO, and soybean oil were added to milk powder. As shown in , the overall impression, frankincense, fishy, colour, and reconstituability did differ among the three products at 60°C for 30-day storage. Sensory state of milk powder with soybean oil and OPO was obviously lower than that with microencapsulated OPO at 60°C for 10-day storage, while microencapsulated OPO can keep a better state of the sensory at 60°C for 30-day storage. The change of five sensory indexes in milk powder with microencapsulated OPO was below 5% at 60°C for 30-day storage, while the change of sensory indexes in milk powder with soybean and OPO was within the range of 4–14% at 60°C for 10-day storage and reach 11–28% at 60°C for 30-day storage.
Table 3. Sensory evaluation of milk powder with different fats at 60°C for 30day storage (mean ± SD).
Effect of different milk powder on weight of mice
During the last three days of the feeding period, dietary intake and weight gain were shown in . Dietary intake of mice feeding control group (9.96 ± 0.43 g/3 days) was significantly higher than that in the other two groups (P < 0.05), and dietary intake of mice feeding SO group (5.27 ± 0.83 g/3 days) was lower than that in SMO group (6.53 ± 0.62 g/3 days). It may be due to more unsaturated fatty acids exist in the OPO that affected the flavour of milk powder. The increased fishy of SO group results in the lower dietary intake. The increased weight (1.78 ± 0.17 g/3 days) of SMO group was the highest and significantly higher than the other two groups (P < 0.05). Thus, digestion utilization of SMO group was significantly higher than the other group (P < 0.05). At the same time, the analysis of the unit dietary weight gain (see ) also confirmed this conclusion; unit dietary weight value of SMO group was 0.31 ± 0.16 g/g, of control group was 0.15 ± 0.12 g/g, and of SO group was 0.27 ± 0.09 g/g.
Table 4. Feed intake/body weight gain of mice (g/g).
Fatty acid composition of blood lipids and mice faeces
Blood lipid samples were treated by methyl esterification and determined by gas chromatography; the determination results are shown in . From the results, the content of C16:0 in control group (15.66 ± 2.53%) was significantly lower than the other two groups (P < 0.05), while the difference was not significant (P > 0.05) between SO group and SMO group. This indicated that the C16:0 of both SO group and SMO group got the same higher absorption efficiency; the microencapsulated method did not affect the absorption of fat. The total amount of C18:1, C18:2, and C18:3 in blood lipid from mice feeding SMO group was up to 44.30% and higher than the other groups.
Table 5. Fatty acid composition of blood serum lipids (%).
Faeces samples were treated by methyl esterification and determined by GC; the determination results are shown in . The C16:0 content (0.14 ± 0.12%) in milk powder with soybean oil was about two times as the other two groups. The C18:1 content in SMO group was only 1.51 ± 0.31%, and the C18:1 content in SO group was 1.26 ± 1.57%, which were significantly lower than the control group (19.27 ± 1.12%) (P < 0.05). From the results, C18:1 in SO group and SMO group were also received the higher absorption. For short, medium-chain fatty acid (C8:0, C10:0, and C12:0) content was extremely low in the three groups. It showed that their digestion and absorption speed faster, with less faeces discharge.
Table 6. Fatty acid composition of faecal fats (%).
Most infants do not breastfeed from their mothers but instead take in milk containing a formula that comes from vegetable oils or dairy products. OPO contains fatty acid composition similar to those in breast milk which is important in toddler nutrition.[Citation1] Microencapsulation process can protect bioactive components against spoilage, volatile losses, and other property damage. Therefore, this physical barrier will be formed that can extend shelf life and control release of encapsulated under adverse environmental condition.[Citation31] From the results in , microencapsulation step did not affect nutrition absorption in mice to OPO.
Calcium and magnesium analysis in faeces
The magnesium content among the three groups did not differ significantly. The excretion rate of calcium in faeces was a significant difference. The excretion rate of calcium in SO group and SMO group was lower than the control group. This could be caused by that palmitic acid at Sn-1 and Sn-3 can bind calcium to form calcium soap, and calcium soap keeps a lower solubility in the bile; thus, fat and calcium in the soap is difficult to be absorbed and excreted with faeces.[Citation1] It would cause an increase in the rate of calcium excretion in the faeces. “Calcium soap” formation would cause a loss of energy in the stool and just as with the loss of calcium that can affect bone mineralization. In addition to the stretcher, the high formation of “calcium soap” in solution can cause constipation, a condition that is not good for the baby.[Citation32] From the results, SMO group reduced the excretion of calcium and improved the absorption of calcium.
Absorption test across everted gut sacs
The everted intestinal sac model in vitro was used to obtain valuable information on OPO absorption through the gut.[Citation33] The everted gut sac model has an apparent advantage because of its fast absorption of nutrients.[Citation34] The small intestine absorption experiment was carried out through the everted gut sacs; the results are shown in . From the results in , the C8:0 and C10:0 content of OPO was 11.51 ± 1.28% and 5.16 ± 1.89%, respectively. The C8:0 and C10:0 content of microencapsulated OPO was 13.89 ± 1.49% and 5.57 ± 0.09%, respectively. The C8:0 and C10:0 content of both SO group and SMO group was higher than the control group. The SMO group was slightly higher than SO group. This indicated that the short-chain fatty acids in SO group and SMO group had a better adsorption rate. The C16:0 content of SO group and SMO group were 20.85 ± 0.43% and 19.84 ± 2.44% and significantly higher than the control group. The results showed that palmitic acid from SO group and SMO group got better absorption.
Table 7. The content of the calcium and magnesium in the mice faeces (%).
Table 8. Fatty acid composition of the intestinal absorption test (%).
Conclusion
In this study, the addition of microencapsulated OPO in infant formula can significantly improve the oxidative stability of infant formula and extend the shelf life of the product. Infant formula with microencapsulated OPO possess better sense state and provide a broader space for its industrial production. The microencapsulated OPO can also provide the necessary theoretical and practical foundation for the large-scale industrial production of the structured lipid microcapsules.
Additional information
Funding
References
- Djajasoepena, S.; Suprijana, O.; Diana, R.S.; Pratomo, U.; Puspitasari, S., Production of Human Milk Fat Replacement Rich of 1,3-Dioleoyl-2-Palmitoilglycerol from Enzymatic Interesterification Tripalmitin, Ethyl Oleate and Mixture of Vco, Soybean Oil and Fish Oil. Procedia Chemistry 2015, 16, 384–391. DOI: 10.1016/j.proche.2015.12.068.
- Lien, E.L.; Boyle, F.G.; Yuhas, R.; Tomarelli, R.M.; Quinlan, P., The Effect of Triglyceride Positional Distribution on Fatty Acid Absorption in Rats. Journal of Pediatric Gastroenterology and Nutrition 1997, 25, 167–174. DOI: 10.1097/00005176-199708000-00007.
- López-López, A.; Castellote-Bargalló, A.I.; Campoy-Folgoso, C.M.; Rivero-Urgel, R.; Infante-Pina, D., The Influence of Dietary Palmitic Acid Triacylglyceride Position on the Fatty Acid, Calcium and Magnesium Contents of at Term Newborn Faeces. Early Human Development 2001, 65, 83–94. DOI: 10.1016/s0378-3782(01)00210-9.
- Koletzko, B.; Rodriguez-Palmero, M.; Demmelmair, H.; Fidler, N.; Jensen, R.; Sauerwald, T., Physiological Aspects of Human Milk Lipids. Early Human Development 2001, 65, 3–18. DOI: 10.1016/s0378-3782(01)00204-3.
- Anal, A.K.; Stevens, W.F., Chitosan-Alginate Multilayer Beads for Controlled Release of Ampicillin. International Journal of Pharmaceutics 2005, 290, 45–54. DOI: 10.1016/j.ijpharm.2004.11.015.
- Mohammed, N.K.; Hussin, A.S.M.; Tan, C.P.; Manap, M.Y.A.; Alhelli, A.M., Quality Changes of Microencapsulated Nigella Sativa Oil upon Accelerated Storage. International Journal of Food Properties 2018, 3, 1–14. DOI: 10.1080/10942912.2017.1371189.
- Bakry, A.M.; Fang, Z.; Ni, Y.; Cheng, H.; Chen, Y.Q.; Liang, L., Stability of Tuna Oil and Tuna Oil/Peppermint Oil Blend Microencapsulated Using Whey Protein Isolate in Combination with Carboxymethyl Cellulose or Pullulan. Food Hydrocolloid 2016, 60, 559–571. DOI: 10.1016/j.foodhyd.2016.04.026.
- Anwar, S.H.; Kunz, B., The Influence of Drying Methods on the Stabilization of Fish Oil Microcapsules: Comparison of Spray Granulation, Spray Drying, and Freeze Drying. Journal of Food Engineering 2011, 105, 367–378. DOI: 10.1016/j.jfoodeng.2011.02.047.
- Chávarri, M.; Marañón, I.; Ares, R.; Ibáñez, F.C.; Marzo, F.; Villarán, M.C., Microencapsulation of a Probiotic and Prebiotic in Alginate-Chitosan Capsules Improves Survival in Simulated Gastro-Intestinal Conditions. International Journal of Food Microbiology 2010, 142, 185–189. DOI: 10.1016/j.ijfoodmicro.2010.06.022.
- Shani-Levi, C.; Levi-Tal, S.; Lesmes, U., Comparative Performance of Milk Proteins and Their Emulsions under Dynamic in Vitro Adult and Infant Gastric Digestion. Food Hydrocolloids 2013, 32, 349–357. DOI: 10.1016/j.foodhyd.2013.01.017.
- Chew, S.C.; Tan, C.P.; Long, K.; Nyam, K.L., In-Vitro, Evaluation of Kenaf Seed Oil in Chitosan Coated-High Methoxyl Pectin-Alginate Microcapsules. Industrial Crops & Products 2015, 76, 230–236. DOI: 10.1016/j.indcrop.2015.06.055.
- Wan, P.J.; Pakarinen, D.R.; Wakelyn, P.J., Concerns for the Determination of Free Fatty Acid in Cottonseed. Journal of the American Oil Chemists Society 1998, 75, 1321–1324. DOI: 10.1007/s11746-998-0178-5.
- Sims, B.; Trussville, A.L. Method of making infant formula and related devices. U. S. Patent 9345360 B2, May 24, 2016.
- IDF. Sensory Evaluation of Dairy Products by Scoring. Standard 99C; International Dairy Federation; Brussels, Belgium, 1997.
- Drake, M.A.; Karagul-Yuceer, Y.; Cadwallader, K.R.; Civille, G.V.; Tong, P.S., Determination of the Sensory Attributes of Dried Milk Powders and Dairy Ingredients. Journal of Sensory Studies 2003, 18, 199–216. DOI: 10.1111/j.1745-459x.2003.tb00385.x.
- Kamath, A.; Ravi, R.; Rajalakshmi, D., Sensory Profiling and Positioning of Commercial Samples of Milk Powder. Journal of Sensory Studies 2010, 14, 303–319. DOI: 10.1111/j.1745-459x.1999.tb00119.x.
- ISO. Animal and Vegetable Fats and Oils- Determination of the Composition of Fatty Acids in the 2-Position of the Triglyceride Molecules, Second; International Organization for Standardization, Switzerland, ISO 6800: 1997.
- Li, C.; Zhao, J.L.; Wang, Y.T.; Han, X.; Liu, N., Synthesis of Cyclopropane Fatty Acid and Its Effect on Freeze-Drying Survival of Lactobacillus Bulgaricus L2 at Different Growth Conditions. World Journal of Microbiology & Biotechnology 2009, 25, 1659–1665. DOI: 10.1007/s11274-009-0060-0.
- Zhang, Y.; Li, A.L.; Sun, Y.Q.; Du, P.; Liu, L.B.; Li, S., Lactobacillus Acidophilus, Regulates STAT3 and STAT5 Signaling in Bovine β-lg-sensitized Mice Model. Dairy Science & Technology 2016, 96, 501–512. DOI: 10.1007/s13594-016-0284-3.
- Tamura, M.; Ohnishi, Y.; Kotani, T.; Gato, N., Effects of New Dietary Fiber from Japanese Apricot (Prunus Mume Sieb. Et Zucc.) On Gut Function and Intestinal Microflora in Adult Mice. International Journal of Molecular Sciences 2011, 12, 2088–2099. DOI: 10.3390/ijms12042088.
- Kristensen, J.B.; Jorgensen, H.; Mu, H., Diacylglycerol Oil Does Not Affect Portal Vein Transport of Nonesterified Fatty Acids but Decreases the Postprandial Plasma Lipid Response in Catheterized Pigs. Journal of Nutrition 2006, 136, 1800–1805. DOI: 10.1093/jn/136.7.1800.
- Sharma, P.; Chawla, H.P.; Panchagnula, R., LC Determination of Cephalosporins in In Vitro Rat Intestinal Sac Absorption Model. Journal of Pharmaceutical and Biomedical Analysis 2002, 27, 39–50. DOI: 10.1016/s0731-7085(01)00506-4.
- Ramírez, M.; Amate, L.; Gil, A., Absorption and Distribution of Dietary Fatty Acids from Different Sources. Early Human Development 2001, 65, 95–101. DOI: 10.1016/s0378-3782(01)00211-0.
- Koo, W.W.; Hockman, E.M.; Dow, M., Palm Olein in the Fat Blend of Infant Formulas, Effect on the Intestinal Absorption of Calcium and Fat, and Bone Mineralization. Journal of the American College of Nutrition 2006, 25, 117–122. DOI: 10.1080/07315724.2006.10719521.
- Esteban, L.; Jiménez, M.J.; Hita, E.; González, P.A.; Martín, L.; Robles, A., Production of Structured Triacylglycerols Rich in Palmitic Acid at Sn −2 Position and Oleic Acid at Sn −1,3 Positions as Human Milk Fat Substitutes by Enzymatic Acidolysis. Biochemical Engineering Journal 2011, 54, 62–69. DOI: 10.1016/j.bej.2011.01.009.
- Keshani, S.; Wan, R.; Nourouzi, W.D.; Ghasemi, M.M.; Namvar, F., Spray Drying, an Overview on Wall Deposition, Process and Modeling. Journal of Food Engineering 2015, 146, 152–162. DOI: 10.1016/j.jfoodeng.2014.09.004.
- Carneiro, H.C.F.; Tonon, R.V.; Grosso, C.R.F.; Hubinger, M.D., Encapsulation Efficiency and Oxidative Stability of Flaxseed Oil Microencapsulated by Spray Drying Using Different Combinations of Wall Materials. Journal of Food Engineering 2013, 115, 443–451. DOI: 10.1016/j.jfoodeng.2012.03.033.
- Costa, J.M.G.D.; Silva, E.K.; Hijo, A.A.C.T.; Azevedo, V.M.; Malta, M.R.; Alves, J.G.L.F., Microencapsulation of Swiss Cheese Bioaroma by Spray-Drying, Process Optimization and Characterization of Particles. Powder Technology 2015, 274, 296–304. DOI: 10.1016/j.powtec.2015.01.037.
- Chatterjea, M.; Shinde, R. Textbook of Medical Biochemistry. Jaypee Brothers Medical Publications 2012, 391–393. DOI: 10.5005/jp/books/11486.
- Lim, W.T.; Nyam, K.L., Characteristics and Controlled Release Behavior of Microencapsulated Kenaf Seed Oil during In-Vitro Digestion. Journal of Food Engineering 2016, 182, 26–32. DOI: 10.1016/j.jfoodeng.2016.02.022.
- Akhavan, M.S.; Jafari, S.M.; Assadpoor, E.; Dehnad, D., Microencapsulation Optimization of Natural Anthocyanins with Maltodextrin, Gum Arabic and Gelatin. International Journal of Biological Macromolecules 2016, 85, 379–385. DOI: 10.1016/j.ijbiomac.2016.01.011.
- Li, Y.; Mu, H.; Andersen, J.E.T.; Xu, X.; Meyer, O.; Ørngreen, A., New Human Milk Fat Substitutes from Butterfat to Improve Fat Absorption. Food Research International 2010, 43, 739–744. DOI: 10.1016/j.foodres.2009.11.006.
- Wang, L.; Jiang, X.; Xu, W.; Li, C., Complexation of Tanshinone IIa with 2-Hydroxypropyl-Beta-Cyclodextrin, Effect on Aqueous Solubility, Dissolution Rate, and Intestinal Absorption Behavior in Rats. International Journal of Pharmaceutics 2007, 341, 58–67. DOI: 10.1016/j.ijpharm.2007.03.046.
- Balimane, P.V.; Chong, S.; Morrison, R.A., Current Methodologies Used for Evaluation of Intestinal Permeability and Absorption. Journal of Pharmacological and Toxicological 2000, 44, 301–312. DOI: 10.1016/j.proche.2015.12.068.