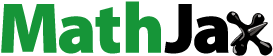
ABSTRACT
The objective of this study was to investigate the effects of explosion puffing on the nutritional composition, physical properties, and digestibility of grains including millet, barley, black rice, rice, glutinous rice, and wheat. Explosion puffing of grains resulted in the nutritional composition with higher total sugar content and lower moisture, starch and fat contents. Although the protein content scarcely changed comparing with the untreated grains, the solubility declined significantly. Moreover, explosion puffing could significantly improve the physical properties including the water absorption index, water solubility index and gelatinization degree. The in vitro digestion experiment was employed to investigate the influences of explosion puffing on the starch hydrolysis rate and free amino acid content, and it was indicated that the digestibility of the starch and protein in grains was highly improved. The results demonstrated that explosion puffing is a suitable technique to improve the physical properties and digestibility of grains, which provided the theoretic foundation for the use of explosion puffing technology in coarse cereal processing.
Introduction
Explosion puffing technology is widely used in snack food processing industry in the world, especially in East Asia. It mainly utilizes the principle of phase-change and the thermal pressure effect of the gas. The water in the raw materials is heated and instantaneously vaporized with the sudden decompression, that results in a volume increase of raw materials and the phenomenon of “blowing-up”, meanwhile a porous structure of the materials is formed. Compared with extrusion puffing, explosion puffing does not require smash, blender, and extrusion of raw materials, therefore it can maintain the original appearance, color, aroma, and taste of raw materials more closely than extrusion puffing. In recent years, there are many researches concerning extrusion puffing, and accumulating evidence has suggested that extrusion puffing can cause significant changes in the physicochemical properties and the content of some nutrients of raw materials. Ai Y. et al.[Citation1] suggested that extrusion puffing led to the gelatinization of soybean starch and denaturation of soybean protein and it could change the gelatinization characteristics and solubility of soybean meal. Honců et al.[Citation2] analyzed the changes in β-glucan content in barley after extrusion and demonstrated that extrusion effectively increased the extraction rate of β-glucan, which contributed to an increase in the nutritional value of the barley products. Alyssa Hidalgo et al.[Citation3] revealed that puffing induced significant changes in technological characteristics (1000 kernel weight, water uptake, water absorption and solubility index, pasting properties, surface area, color) and composition (protein, starch, tocol content, and carotenoid) of puffed bread wheat and einkorn kernels, the puffed einkorn kernels were richer in proteins and bioactive compounds like tocols and carotenoids. Stefano Cattaneo et al.[Citation4] studied the effect of processing conditions on heat damage, starch digestibility, release of advanced glycation end products (AGEs) and antioxidant capacity of puffed wheat kernels and observed that puffing influences starch in vitro digestibility, being most of the starch hydrolyzed to maltotriose, maltose, and glucose whereas only limited amounts of AGEs were released. Mariotti et al.[Citation5] investigated that the effect of puffing treatment on ultrastructure and physical properties of grains was strongly influenced by their botanical origin and hence by the morphology and composition of the kernel. And the significant changes induced by puffing on starch structure and its physical properties modified the water holding capacity of grains. Nevertheless, compared with extrusion puffing, limited literature is available about comparative studies of the physical and chemical properties and digestibility of different grains before and after explosion puffing systematically. Therefore, we investigated systematically the effects of explosion puffing on the nutritional composition, physical properties, and digestibility of grains including millet, barley, black rice, rice, glutinous rice, and wheat, which laid a theoretical foundation for the development of explosion puffing in cereal processing.
Materials and methods
Materials
Millet, barley, wheat, rice, black rice, and glutinous rice were purchased from a local market in Chengdu, the capital of Sichuan province in China. Porcine pancreatic α-amylase (A3403-500KU, type VI-B from porcine pancreas, 500 U/mg), trypsin (T8003-500MG, trypsin from porcine pancreas Type IX-S, lyophilized powder, 10000 BAEE U/mg protein), pepsin (P700-25G, pepsin from gastric mucosa, 250 U/mg) and amyloglucosidase (10115-1G-F, 70 U/mg) were purchased from Sigma-Aldrich, Inc. (St. Louis, MO, USA). All other chemicals and reagents used for this study were of AR grade.
Sample treatment
The moisture contents of the grains were adjusted to 13–14%. Then, the grains were heated in an expansion chamber under pressure of 0.8–1.5 MPa for 70–80 s. At the end of the puffing process, the chamber was opened, resulting in a sudden drop in pressure which caused the instantaneous evaporation of the water in the grains. The puffed grains were cooled to room temperature, ground using a grinder and passed through a 250-μm sieve. The flour from the grains was sealed in polyethylene bags and stored in a desiccator until measurements were taken for further analysis.
Analysis of nutritional composition
The moisture content, protein content, fat content, and total sugar were determined according to the methods of AOAC (No. 925.09, No. 920.87, No. 920.85, No. 991.43).[Citation6] Starch was determined using the amyloglucosidase enzyme method of AOAC.[Citation7] The determination of protein content was based on the method of Haikerwal and Mathieson[Citation8] with some modifications: the samples were extracted with distilled water, 5 kg/m3 NaCl solution, 75 kg/m3 ethanol solution, 0.2 kg/m3 NaOH solution, and 1 kg/m3 SDS solution to separate albumin, salt-soluble protein, gliadin, glutenin, and SDS-soluble protein, respectively. The protein content of each composition was determined using an automatic Kjeldahl apparatus. All measurements were performed in triplicate.
Analysis of physical properties
Gelatinization degree
The gelatinization degree of the grains was determined according to the method used by Birch and Priestley[Citation9] with several modifications. A 2.0 g sample was added to 100 ml of mixed solution, which consisted of 98 ml of distilled water and 2 ml of 10 mol/L KOH solution, and stirred gently for 5 min. The mixture was centrifuged at 3500 g for 10 min, a 1 ml aliquot of the supernatant was treated with 0.8 ml of a 0.5 mol/L HCl solution, and the volume was maintained at 20 ml with distilled water. Then, 0.1 ml of an iodine reagent was added, and the absorbance of the mixed solution was measured at 600 nm. In addition, 95 ml of distilled water and 5 ml of a 10 mol/L KOH solution, which was neutralized with 2 ml of a 0.5 mol/L HCl solution, were used to repeat the estimation. The gelatinization degree (%) is proportional to the ratio of the two light densities obtained from each sample.
Water absorption index (WAI) and water solubility index (WSI)
The water absorption index and water solubility index were determined using the method of Ding et al.[Citation10] with some modifications. The ground samples were suspended in distilled water at 30°C for 30 min with gentle stirring. The samples were then centrifuged at 2000 g for 10 min. The supernatant was poured into an evaporating dish of known weight. The WSI is the weight of the dry solid in the supernatant, which is expressed as the percentage of the original weight of the sample. The WAI was calculated as the weight of gel per gram of dry sample. Determinations were made in triplicate. The WAI and WSI were calculated based on the following equations:
In vitro digestibility of grains
In vitro starch digestibility
An in vitro simulated starch digestion experiment was carried out according to the method of Goñi I.et al.[Citation11] with some modifications. First, 1.0 g of the defatted sample was transferred into a 50 ml flask containing 10 ml of phosphate buffer (pH 6.9) to maintain constant acidity. After grinding evenly, the volume was kept constant at 50 ml and the sample was incubated at 100°C for 30 min. Then, 3.5 ml of trypsin (1 kg/m3) and 3.5 ml of glucoamylase (1 kg/m3) were added to the sample. The mixture was kept for 0 min, 10 min, 20 min, 30 min, 60 min, 90 min, 120 min, and 150 min. At the end of the incubation period, the hydrolytic samples were boiled for 5 min to stop the enzymatic reactions. The hydrolysate was centrifuged at 6000 g for 10 min, and the supernatant was diluted 10 times. Then, 2 ml of 3, 5-dinitrosalicylic acid reagent was added, and the mixture was boiled for 5 min. After cooling, the absorbance of the filtered solution was measured at 540 nm, using glucose as a standard. The in vitro starch digestibility was calculated based on the following equation:
In vitro protein digestibility
An in vitro protein digestion test, reported by Chavan, Mckenzie et al.[Citation12] was used as an experimental model of pepsin-trypsin enzymatic hydrolysis, with some modifications. A 2.5 g dry and defatted sample was diluted with 25 ml of 0.01 mol/L HCl solution and incubated in a 50-ml colorimetric tube at 37°C for 5 min. Next, 10 mg of pepsin was added to the samples and incubated at 37°C for 2 h. The pepsin hydrolysate was neutralized with a 1.0 mol/L NaOH solution. Then, 10 mg of trypsin was added, and the solution was incubated at 37°C for 2 h. The hydrolysates were collected at different digestion times (0 min, 10 min, 30 min, 60 min, 90 min, 120 min, 150 min, 180 min, 210 min, and 240 min) to be analyzed after boiling for 5 min to stop the enzymatic reactions. Then, the free amino acid content of the hydrolysates was determined using the method of Paul et al.[Citation13] The hydrolysates were centrifuged at 6000 g for 10 min. Next, 1 ml of ninhydrin and 1 ml of phosphate buffer (pH 8.04) were added to 0.5 ml of supernatant and incubated at 100°C for 15 min. After cooling, the volume was maintained at 10 ml with distilled water and the solution was shaken gently. After 15 min, the absorbance of the filtered solution was measured at 570 nm, with a blank group used as the standard. The content of free amino acids in 1 g samples was calculated according to the standard curve of amino acids.
Statistical analysis
The IBM SPSS Statistics software (version 22) was used for data analysis. Duncan’s multiple range test and T-test at the 5% level was used to identify the significant differences of each treatment. Diagrams were plotted using Origin software (version 9.0).
Results and discussion
Effects of explosion puffing on nutritional composition
The effects of explosion puffing on the protein content, moisture content, starch content, total sugar content, and fat content of the grains are presented in . The results showed a highly significant (P < 0.05) impact of explosion puffing on the starch content, the total sugar content, the fat content, and the moisture content. And the difference between the species is also significant (P < 0.05). The moisture content of the puffed grains decreased significantly due to the high pressure and temperatures during the explosion, with the water being changed into vapor and escaping from grains.[Citation14] The starch content decreased, while the total sugar content increased specifically. Starch is a major component of grains; the hydrogen bonds and glycoside bonds between starch molecules would have been destroyed and that would promote the gelatinization of starch during the explosion. A mixture consisting of melted starch, gelatinized starch, and degraded starch would exist in a system after the explosion. Meanwhile, starch could be degraded into many small molecules such as glucose, maltose, etc., which leads to the increase of total sugar content and the decrease of starch content.[Citation15–Citation17]
Table 1. The nutritional composition of grains before and after explosion puffing.
The fat content of the grains decreased significantly after puffing (p<0.05). Kaur K.et al.[Citation18] indicated that the decreased fat content was attributed to the appearance of an amylose-fat complex that formed with the combination of free fat and amylose. In addition, the fat was decomposed into fatty acids and monoglycerides under high temperature and pressure, which was also responsible for the decreased fat content.
Even though the explosion puffing had no significant effect on the total protein content, there was a significant decrease in the solubility of the protein. As shown in , the contents of albumin, globulin, gliadin, and glutenin significantly decreased (p<0.05). However, the content of SDS protein increased, which was similar to the results of the study on extruded millet by Ummadi et al.[Citation19] The protein solubility decreased due to protein aggregation that occurred during extrusion. Mohammed Z. H. et al.[Citation20] proposed that heating and shearing during the expansion process could denature protein and change its structure. This would expose the non-polar groups buried inside protein and increase the surface hydrophobicity and the formation of protein aggregates, resulting in a decline in protein solubility. The SDS solution could disrupt the hydrophobic interactions between protein molecules due to its amphiphilicity, leading to increased protein extraction by the SDS solution. It also proved that the decrease in solubility was indeed related to its surface hydrophobicity.
Table 2. The percentages of protein factions before and after explosion puffing (%, db).
Effects of explosion puffing on physical properties
Water absorption index (WAI)
The water absorption index (WAI) is used to measure the ability of materials to bound water after swelling in excess water and uses the level of starch gelatinization as an indicator.[Citation21] The effects of explosion puffing on the WAI of grains are shown in . For millet, black rice, rice, glutinous rice, and wheat, the WAI almost doubled after puffing, and the WAI of the puffed barley was 1.5 times higher than before puffing. Rampersad R. et al.[Citation22] found that higher WAI might be attributed to the higher amount of damaged polymer chains in starch formed at a higher temperature and pressure. Zapotoczny et al.[Citation23] studied the effect of temperature on physical functional and mechanical characteristics of hot-air puffed amaranth seeds and investigated that the increase in WAI of puffed amaranth seeds depended strongly on the basis of starch-water-protein interactions which affected the solid phase structure of the processed material. WAI has been attributed to the dispersion of starch in excess water which was increased by the degree of starch damage due to gelatinization and process-induced fragmentation. And the type of proteins, degree of their denaturation and amount of fiber present in processed cereal material also were factors affecting WAI of grains.
Water solubility index (WSI)
The water solubility index (WSI) indicates the degree of degradation of macromolecules. It also measures the amount of soluble polysaccharides released from the starch macromolecules after puffing. The effects of explosion puffing on the WSI of grains are shown in . It was observed that the WSI increased significantly after puffing (P < 0.05), which of the puffed grains were 3 times higher than raw materials or more. The phenomenon was mainly attributed to the partial depolymerization of the macromolecules present in grains including starch and protein. During the explosion, the starch in grains would be degraded into several kinds of micromolecules, such as dextrin and soluble polysaccharides.[Citation24] In addition, the increase was also due to the destruction of the tertiary and quaternary structure of the protein in grains. Because of the destruction, the molecular weight of polypeptide chain was reduced while the number of the polar groups was increased, that reduced the hydrophobic interaction between proteins molecules, while the reaction of protein and water was enhanced.[Citation25]
Gelatinization degree
Gelatinization is derived from changes in the morphology of starch. As shown in , there was a significant increase in the gelatinization degree of puffed grains. The reasons for the increase were mainly due to the gelatinization, degradation, and structural changes of starch. During the explosion, starch reacted with water at high temperature and pressure, the hydrogen bond and glycosidic bond between the starch molecules were broken, then the starch granules were partially disintegrated to form a network structure, which caused the gelatinization. Expansion resulted in the degradation of starch and the polymer compound was decomposed into small molecules.[Citation26–Citation28] The higher the gelatinization degree, the easier it is for the starch to be hydrolyzed, which is beneficial for digestion and absorption in the human body.[Citation29] As shown in , the gelatinization degree of the puffed grains was above 80%, and glutinous rice reached 95.59%. Ma Yong-xuan et al.[Citation30] studied the effects of extrusion on the gelatinization of rice and brown rice, it showed that the gelatinization degrees was increased to above 80% of rice and brown rice after extrusion.
Effect of explosion puffing on in vitro digestibility of grains
In vitro protein digestibility
The free amino acid content of grains is shown in . At the beginning of in vitro digestion, the free amino acid content of the grains increased gently under the action of pepsin. When trypsin was added after 120 min of digestion, the free amino acid content increased more rapidly than before. The differences were mainly caused by the different sites of action of pepsin and trypsin. The sites of action of pepsin are the tyrosine, tryptophan, and the peptide bond formed by the residues of phenylalanine, while the sites of action of trypsin are lysine and the peptide bond formed by the residues of arginine. After adding the trypsin, the protein and polypeptide which can be hydrolyzed by trypsin but cannot by pepsin are further hydrolyzed.[Citation31] As a result, the free amino acid content of grains increased more rapidly under the action of trypsin. As shown in , the free amino acid content of puffed grains increased significantly (p < 0.05), which mainly related to the changes of conformation, hydrolysis of protein in grains during the explosion. In addition, heat treatment to the food materials would lead to alteration in the specific structure of the protein molecule. In many instances, increase in digestibility of the protein occurs, perhaps by the opening of the complex structure which serves as the site for the digestive enzyme.[Citation32] Fischer T.[Citation33] found that the force maintaining the stability of the tertiary and quaternary structures of protein was reduced when wheat protein was extruded. Irreversible denaturation occurred in the protein molecule and exposed more enzymatic hydrolysis sites, which increased the opportunity for enzymes to contact the protein and improved the protein digestibility.
In vitro starch digestibility
As shown in , explosion puffing has a significant influence on the in vitro starch digestibility and the difference caused by the different species of puffed grains was also significant (P < 0.05). Compared with the untreated grains, the puffed grains exhibited a significantly higher starch hydrolysis rate, which might be caused by the gelatinization of starch, the changes of starch fractions and structure. It was found that high temperature and pressure during explosion resulted in higher gelatinization. And the higher gelatinization degree indicated that it could provide more opportunities for amylases to make contact with starch, which promotes the hydrolysis of starch.[Citation34,Citation35] The result was in concordance with the studies of Dust et al.[Citation36] and Lankhorst, C. et al.[Citation37] in which the quality of the starches could be modified by thermal and mechanical treatment through gelatinization. Furthermore, Roopa et al.[Citation38] studied the effect of puffing on the starch fractions and investigated that rapidly digestible starch increased with the decrease of slowly digestible starch and resistant starch, which highlighted an enhancing effect of puffing on starch digestibility. In addition, the structure of grains after puffing became loose and porous and the steric hindrance was reduced, which could provide more area for enzymes to hydrolyze the starch of grains. Thereby, explosion puffing could be an effective technique to promote the digestibility of starch and improve the digestion and absorption rate of grains in the human body.
Conclusion
Explosion puffing significantly affected the nutritional composition, physical properties and digestive properties of millet, barley, black rice, rice, glutinous rice, and wheat. After undergoing explosion puffing process, the starch, moisture, and fat contents decreased significantly and the total sugar increased. Although there were no significant changes in protein content, the protein solubility was greatly decreased. At the same time, the physical properties of the grains, involving gelatinization degree, water absorption index, and water solubility index, were increased. In addition, the free amino acid content and starch hydrolysis rate of six kinds of grain were increased during in vitro digestion. The results demonstrated the utilization of explosion puffing technique on various available raw grains can provide great versatility for the development of low cost and high nutritive cereal products.
Acknowledgments
The authors would like to thank Sichuan Huiji Food Co., LTD for their technical support.
References
- Ai, Y.; Cichy, K.A.; Harte, J.B.; Kelly, J.D.; Ng, P.K.W., Effects of Extrusion Cooking on the Chemical Composition and Functional Properties of Dry Common Bean Powders. Food Chemistry 2016, 211, 538–545. DOI: 10.1016/j.foodchem.2016.05.095.
- Honců, I.; Sluková, M.; Vaculová, K.; Sedláčková, I.; Wiege, B.; Fehling, E., The Effects of Extrusion on the Content and Properties of Dietary Fiber Components in Various Barley Cultivars. Journal of Cereal Science 2016, 68, 132–139. DOI: 10.1016/j.jcs.2016.01.012.
- Hidalgo, A.; Scuppa, S.; Brandolini, A., Technological Quality and Chemical Composition of Puffed Grains from Einkorn (Triticum Monococcum, L. Subsp. Monococcum) and Bread Wheat (Triticum Aestivum, L. Subsp. Aestivum). LWT - Food Science and Technology 2016, 68, 541–548. DOI: 10.1016/j.lwt.2015.12.068.
- Cattaneo, S.; Hidalgo, A.; Masotti, F.; Stuknytė, M.; Brandolini, A.; De Noni, I., Heat Damage and in Vitro, Starch Digestibility of Puffed Wheat Kernels. Food Chemistry 2015, 188, 286–293. DOI: 10.1016/j.foodchem.2015.05.019.
- Mariotti, M.; Alamprese, C.; Pagani, M.A.; Lucisano, M., Effect of Puffing on Ultrastructure and Physical Characteristics of Cereal Grains and Flours. Journal of Cereal Science 2006, 43, 47–56. DOI: 10.1016/j.jcs.2005.06.007.
- Association of Official Analytical Chemists (AOAC). Official Methods of AOAC, International, 18th ed.; Association of Official Chemists: Gaithersburg, MD, USA, 2006.
- Association of Official Analytical Chemists (AOAC). Official Methods of AOAC; Association of Official Chemists: Washington, 1980; Vol. II.
- Haikerwal, M.; Mathieson, A.R. Extraction and Fractionation of Proteins of Sorghum Kernels. Journal of the Science of Food & Agriculture 2010, 22(3), 142–145. DOI: 10.1002/jsfa.2740220311.
- Birch, G.G.; Priestley, R.J. Degree of Gelatinization of Cooked Rice. Starch - Stã¤Rke 1973, 25(3), 98–100. DOI: 10.1002/star.19730250308.
- Ding, Q.; Ainsworth, P.; Tucker, G.; Marson, H. The Effect of Extrusion Conditions on the Physicochemical Properties and Sensory Characteristics of Rice-Based Expanded Snacks. Journal of Food Engineering 2005, 66(3), 283–289. DOI: 10.1016/j.jfoodeng.2004.03.019.
- Goñi, I.; Garcia-Alonso, A.; Saura-Calixto, F. A Starch Hydrolysis Procedure to Estimate Glycemic Index. Nutrition Research 1997, 17(3), 427–437. DOI: 10.1016/S0271-5317(97)00010-9.
- Chavan, U.D.; McKenzie, D.B.; Shahidi, F. Functional Properties of Protein Isolates from Beach Pea (Lathyrus Maritimus L.). Food Chemistry 2001, 74(2), 177–187. DOI: 10.1016/S0308-8146(01)00123-6.
- Hurst, P.L.; Sinclair, B.K.; Eason, J.R. Amino Acids Interfere with the Ninhydrin Assay for Asparagine. Food Chemistry 1995, 53(4), 467–469. DOI: 10.1016/0308-8146(95)99843-O.
- Burgos, V.E.; Armada, M. Characterization and Nutritional Value of Precooked Products of Kiwicha Grains (Amaranthus Caudatus). Food Science & Technology 2015, 35(ahead). DOI: 10.1590/1678-457X.6767.
- Ratnayake, W.S.; Hoover, R.; Shahidi, F.; Perera, C.; Jane, J. Composition, Molecular Structure, and Physicochemical Properties of Starches from Four Field Pea (Pisum Sativum L.) Cultivars. Food Chemistry 2001, 74(2), 189–202. DOI: 10.1016/S0144-8617(00)00260-5.
- Jyothi, A.N.; Sheriff, J.T.; Sajeev, M.S. Physical and Functional Properties of Arrowroot Starch Extrudates. Journal of Food Science 2009, 74(2), 97–104. DOI: 10.1111/j.1750-3841.2008.01038.
- Guha, M.; Ali, S.Z.; Bhattacharya, S. Twin-Screw Extrusion of Rice Flour without a Die: Effect of Barrel Temperature and Screw Speed on Extrusion and Extrudate Characteristics. Journal of Food Engineering 1997, 32(3), 251–267. DOI: 10.1016/S0260-8774(97)00028-9.
- Kaur, K.; Singh, N. Amylose-Lipid Complex Formation during Cooking of Rice Flour. Food Chemistry 2000, 71(4), 511–517. DOI: 10.1016/S0308-8146(00)00202-8.
- Ummadi, P.; Chenoweth, W.L.; Pkw, N. Changes in Solubility and Distribution of Semolina Proteins Due to Extrusion Processing. Cereal Chemistry 1995, 72(6), 564–567.
- Mohammed, Z.H.; Hill, S.E.; Mitchell, J.R. Covalent Crosslinking in Heated Protein Systems. Journal of Food Science 2010, 65(2), 221–226. DOI: 10.1111/j.1365-2621.2000.tb15983.x.
- Van, D.E.R.M.; Van, D.G.A.J.; Boom, R.M. Understanding Molecular Weight Reduction of Starch during Heating-Shearing Process. Journal of Food Science 2010, 68(8), 2396–2404. DOI: 10.1111/j.1365-2621.2003.tb07036.x.
- Rampersad, R.; Badrie, N.; Comissiong, E. Physico-Chemical and Sensory Characteristics of Flavored Snacks from Extruded Cassava/Pigeonpea Flour. Journal of Food Science 2003, 68(1), 363–367. DOI: 10.1111/j.1365-2621.2003.tb14166.x.
- Zapotoczny, P.; Markowski, M.; Majewska, K.; Ratajski, A.; Konopko, H. Effect of Temperature on the Physical, Functional, and Mechanical Characteristics of Hot-Air-Puffed Amaranth Seeds. Journal of Food Engineering 2006, 76(4), 469–476. DOI: 10.1016/j.jfoodeng.2005.05.045.
- Pelembe, L.A.M.; Erasmus, C.; Taylor, J.R.N. Development of a Protein-Rich Composite Sorghum–Cowpea Instant Porridge by Extrusion Cooking Process. LWT - Food Science and Technology 2002, 35(2), 120–127. DOI: 10.1006/fstl.2001.0812.
- Guerrero, P.; Beatty, E.; Kerry, J.P.; De La Caba, K. Extrusion of Soy Protein with Gelatin and Sugars at Low Moisture Content. Journal of Food Engineering 2012, 110(1), 53–59. DOI: 10.1016/j.jfoodeng.2011.12.009.
- Karim, A.A.; Norziah, M.H.; Seow, C.C. Methods for the Study of Starch Retrogradation. Food Chemistry 2000, 71(1), 9–36. DOI: 10.1016/S0308-8146(00)00130-8.
- Moad, G.;. Chemical Modification of Starch by Reactive Extrusion. Progress in Polymer Science 2011, 36(2), 218–237. DOI: 10.1016/j.progpolymsci.2010.11.002.
- Alrabadi, G.J.; Torley, P.J.; Williams, B.A.; Bryden, W.L.; Gidley, M.J. Effect of Extrusion Temperature and Pre-Extrusion Particle Size on Starch Digestion Kinetics in Barley and Sorghum Grain Extrudates. Animal Feed Science & Technology 2011, 168(3–4), 267–279. DOI: 10.1016/j.anifeedsci.2011.04.097.
- Tang, H.; Mitsunaga, T.; Kawamura, Y. Molecular Arrangement in Blocklets and Starch Granule Architecture. Carbohydrate Polymers 2006, 63(4), 555–560. DOI: 10.1016/j.carbpol.2005.10.016.
- Ma, Y.–.X.; Zhang, M.–.W.; Zhen, C.H.; Zhang, Y.; Zhang, R.–.F.; Deng, Y.–.Y.; Liu, L.; Tang, X.–.J.; Xiao, J.; Huang, F.; Dong, L.-H. Effects of Extrusion on Physiochemical and Nutritional Property of Rice and Brown Rice. Food Research and Development. 2017, 38(12), 9–12. ( (In Chinese)). DOI: 10.3969/j.issn.1005-6521.2017.12.003.
- Su, Y.-T.; Yin, T.; Zhao, S.-M.; Li, J.-T. Effects of Cooking Methods and Rice Varieties on Protein Digestion Properties of Cooked Rice. Food Science. 2014, 35(3), 100–105. (In Chinese). DOI: 10.7506/spkx1002-6630-201403021.
- Rathod, R.P.; Annapure, U.S., Physicochemical Properties, Protein and Starch Digestibility of Lentil Based Noodle Prepared by Using Extrusion Processing. LWT-Food Science and Technology 2017, 80, 121–130. DOI: 10.1016/j.lwt.2017.02.001.
- Fischer, T.;. Effect of Extrusion Cooking on Protein Modification in Wheat Flour. European Food Research & Technology 2004, 218(2), 128–132. DOI: 10.1007/s00217-003-0810-4.
- Van, H.P.; Chau, H.T.; Phi, N.T., In Vitro Digestibility and in Vivo Glucose Response of Native and Physically Modified Rice Starches Varying Amylose Contents. Food Chemistry 2016, 191, 74–80. DOI: 10.1016/j.foodchem.2015.02.118.
- Mishra, G.; Joshi, D.C.; Panda, B.K. Popping and Puffing of Cereal Grains: A Review. Journal of Grain Processing and Storage 2014, 1, 34–46.
- Dust, J.M.; Gajda, A.M.; Flickinger, E.A.; Burkhalter, T.M.; Merchen, N.R.; Fahey, G.C. Extrusion Conditions Affect Chemical Composition and in Vitro Digestion of Select Food Ingredients. Journal of Agricultural & Food Chemistry 2004, 52(10), 2989–2996. DOI: 10.1021/jf049883u.
- Lankhorst, C.; Tran, Q.D.; Havenaar, R.; Hendriks, W.H.; van der Poel, A.F.B. The Effect of Extrusion on the Nutritional Value of Canine Diets as Assessed by in Vitro Indicators. Animal Feed Science and Technology 2007, 138(3), 285–297. DOI: 10.1016/j.anifeedsci.2006.11.015.
- Roopa, S.; Premavalli, K.S. Effect of Processing on Starch Fractions in Different Varieties of Finger Millet. Food Chemistry 2008, 106(3), 875–882. DOI: 10.1016/j.foodchem.2006.08.035.