ABSTRACT
The main goal of the present investigation was to the synthesis of bioactive TiO2 nanoparticle-incorporated poly(methylmethacrylate)/polyetheretherketone nanocomposite for enhanced antibacterial and biocompatibility characteristics with suitable properties for manufacturing 3D printed stereolitographic dental prosthesis. The carboxylic acid-functionalized TiO2 nanoparticles have been dispersed into the copolymer matrixes through emulsion polymerization method, and the structural and morphological properties were investigated by using Fourier transform infrared spectroscopy (FTIR), X-ray diffraction, field emission scanning electron microscopy (FE-SEM), transmission electron microscopy, and energy dispersive X-ray (EDX) analytical techniques. The FTIR spectrum of synthesized nanocomposites that shows high intense peaks at 2,970–2,850 cm−1 is related to the symmetric and asymmetric stretching C-H vibration of methyl and methylene groups of the polymer matrix. The microscopic results of nanocomposite exhibited that functionalized nanoparticles were effectively and uniformly dispersed into the polymer matrix in the absence of particle agglomerations, and it has been given excellent killing efficiency of 99% under dark conditions against bacterial pathogens Escherichia coli and Staphylococcus aureus and also exhibited favorable cell compatibility with dental pulp cells. This facile synthesized nanocomposite was efficaciously employed for denture manufacturing with stereolitographic technique.
Introduction
The composited dental resin has been extensively applied for dental caries restoration and other tooth defects instead of conventional blend alloys, owing to their excellent and effective advantages such as high esthetic quality, favorable mechanical properties, excellent biocompatibility, and easy handling.[Citation1–Citation5] Dental composite resins are generally designed of resin polymer matrixes with inorganic nano-fillers and initiating agents.[Citation6] In the recent materials, researchers have increased large attentions on nano-inorganic materials assisted with organic hybrid composites because of their prospective applications in different areas such as sensors (bio- and chemical-), electronics, energy, plastic reinforcement, and particularly biomaterials for bone and dental regeneration applications.[Citation7–Citation11] The composites synthesized for dental restoration used by a combination of different shape and sized suitable inorganic nanoparticles encapsulated with the cold-cure acrylic polymeric matrix. These types of composited resins frequently consist of methacrylates, specifically poly(methyl methacrylate) (PMMA) and also poly ethyl methacrylate. The PMMA polymer matrixes have extensively been applied in dentistry restorations for widespread denture preparations due to their luxury processing for dental repair, cost-effective, tasteless, odorless nature, transparency, great suitability and biocompatibility to the patients, and also outstanding esthetic characteristics.[Citation6,Citation12–Citation14] Polyethereketone (PEEK) is a semicrystalline polymer (crystallinity = 30–35%), which has been applied as a potential material for the dental and bone implant due to its favorable glass transition temperature (143°C) and excellent elastic modulus for the ease of molding to 3D printed materials.[Citation15,Citation16]The dispersion of effective inorganic nanoparticles into the polymeric composite resins can be progressed and greatly influenced by some potential resin restoration properties of nanoparticles such as suitable modulus, active flexural strength, wear resistance, and gloss retention.[Citation17–Citation19] The development of dental implants with titanium dioxide (TiO2)-based nanomaterials has become a topic of growing interest since the recent years. The nanostructures of TiO2 have been employed in different efficient areas involving energy and biomedical applications due to their dielectric properties, high photo-catalytic behavior, high stability, excellent corrosion resistance, mechanical strength, and favorable biocompatibility in biological applications.[Citation20,Citation21] A biologically active TiO2 nanoparticle-incorporated material is always employed as a reinforced material for dental and bone implant regeneration coatings due to its excellent antimicrobial and biological activity.[Citation22–Citation26] Improving the antimicrobial properties of the synthesized composite resins was most helpful in monitoring secondary caries adjacent to the dental filling, which was investigated and reported as the main reason for many restoration failures. The biological characteristics of TiO2 have been efficiently enhanced the antibacterial protection and osteoblast cell adhesion after the dental implant and also aggressively improved the self-healing characteristics by attaining a direct chemical bonding to the surrounding dental tissues.[Citation27–Citation31] In the present investigation, the morphologically improved TiO2 nanoparticles have been introducing into the PMMA/PEEK copolymer composite resins for improving the antibacterial activity and biocompatibility. The homogeneous dispersions of TiO2 nanoparticles in the composite resins and structural modifications were analyzed by microscopic and spectroscopic analyses. Afterward, evaluations for ion release, cytotoxicity, and antibacterial activity of the synthesized nanocomposites were also carried out. The favorable results and hypothesis of the current study are that the TiO2 nanoparticle-incorporated nanomaterials are highly able to serve as effective reinforcement agents for dental composite resin with high antibacterial activity.
Materials and methods
Functionalization of TIO2 nanoparticles
The nano-TiO2 particles were functionalized with carboxylic acid (acrylic acid) according to the following method: First, the purchased 0.5 g of nano-TiO2 particles were mixed to the 14.4 g of acrylic acid and 16 g hexane with small quantity of water to placed for sonication under ultrasonic processor instrument for 20 min at 0°C. Then, the above-prepared mixture was agitated at room temperature for 2 days. In the completion of the reaction, the milk-like mixture was formed, and it was separated by centrifugation at 12,000 rpm for 1 h. The precipitated material was repeatedly redistributed in ethyl alcohol (30 ml) and centrifuged again at 12,000 rpm for another 1 h to remove the acrylic acid residues adsorbed on the surface of TiO2 nanoparticles. Finally, the resulting functionalized TiO2 nanoparticles were dried under vacuum oven.[Citation32]
Synthesis of nano-TiO2-incorporated PMMA/PEEK nanocomposites
The carboxylic acid-functionalized TiO2 powder was added into PEEK (1 wt%) solution, and the mixture was homogeneously dispersed under sonication for 1 h according to the previous literature described.[Citation33] The TiO2/PEEK dispersion was added into methyl methacrylate (MMA) monomer solution in the jacketed reactor for the PMMA polymerization under equipped with stirred, reflux condenser under N2 inlet atmosphere under emulsion polymerization process.[Citation12] Then, the polymerization reaction started by increasing the temperature to 70°C with potassium persulphate initiator, and the reaction process was continued for 3 h for the PMMA polymerization on the TiO2/PEEK nanomaterials. The prepared nanocomposites were recuperated by precipitation and washed with alcoholic solvents to remove the excess of surfactant and other impurities. Finally, the resulting products were dried at 90°C for 12 h in an air oven.
Physical characterization details
X-ray diffraction (XRD; PANalytical’s X’Pert PRO MRD diffractometer) investigations have been executed in CuKα radiation in the 2θ = 20−70° range. Fourier transform infrared spectroscopy (FTIR; SHIMADZU) analysis was observed using KBr disks in the region 400–4,000 cm−1. Morphological surface and structural characterization of nano-TiO2-coated PEEK/PMMA was performed by scanning electron microscopy (SEM; Carl Zeiss AURIGA) combined with EDX and transmission electron microscopy (TEM; Hitachi H8100) operated at 200 kV. For TEM analysis, a drop of samples sonicated and deposited onto 200-mesh copper grids and permissible to dry before taking microscopic images.
Antibacterial analysis
The bacterial pathogen cultures (Escherichia coli and Staphylococcus aureus) were used for these antibacterial investigations. The microorganisms were cultured in sterilized nutrient broth and then incubated aerobically overnight at 37°C under shaking incubator. The antibacterial efficacy of nano-TiO2-incorporated PMMA/PEEK nanocomposites and their interactions of bacterial cell surface are the dynamic role that analyzes the antibacterial activity. In the current investigation, interactions between nano-TiO2 nanoparticles and bacterial cell surface were studied by two different techniques (agar diffusion method and solution suspension). The decrease of viable bacteria was observed by calculating total viable counts at different intermissions of time. And the destruction of pathogen cell wall and death of the bacterial cells were displayed by using confocal laser scanning microscopic (CLSM) technique. Fluorescein isothiocyanate (FITC) stain was used for recognizing living cells on bacterial after nanoparticle addition. The FITC green fluorescent dye penetrates only undamaged (living) cell walls giving increase to fluorescence emission. The respective stain (0.5 µl) was added to bacterial cultured samples and then incubated for 10 min under appropriate conditions. The residual fluorescent stain was removed by using distilled water, and then, samples observed under CLSM (Nikon A1R, Nikon, Japan).
In vitro cell culture and cytocompatibility analysis
The culture of human dental pulp stem cells (hDPSCs) was carried out following the procedure method. The pulp tissues were pulverized and processed into the collagenase type I (3 mg/ml) solution and dispase (4 mg/ml) for 45–60 min at 37°C. The pulp cell suspension was attained through a 70-μm cell strainer, and it was pelleted and then incubated with Dulbecco's modified Eagle's medium (DMEM) (with fetal calf serum, penicillin, and streptomycin) growth medium in a moistened atmosphere (95% air and 5% CO2). Non-attached cells were removed 2 days after the plating, and culture medium was changed every 3 days. For each experiment, a set of triplicate nanocomposite scaffolds was placed in 96-well culture plates. The hDPSCs were then planted, and the plates were then incubated at 37°C in a humidified atmosphere. After 24 h of incubation, the cell viability was observed using 3-(4, 5-dimethylthiazol-2-yl)-diphenyltetrazolium bromide (MTT) assay under Cell Proliferation Kit I. Ten microliters of MTT labeling reagent were added to each well and incubated for 3 h, and then, 100 µl of the solubilizing solution was added and kept for 12 h. The absorbance value was noted using a microplate reader for the cell viability diagram.
Results and discussion
FTIR and XRD analysis
In this work, a surface-functionalized nano-TiO2-coated PMMA/PEEK composite was synthesized and characterized by different spectroscopic (FTIR and XRD) and microscopic (SEM and TEM) techniques for analyzing structural changes and morphological changes with the assistance of nano-fillers. shows the FTIR spectra of the pure TiO2 encapsulated with the PMMA/PEEK polymer matrixes after the polymerization process. The high intense peak at 1,732 cm−1 (stretching vibrations), which corresponds to ester carbonyl groups of PMMA polymer, and an absorption peak at 2,970–2,850 cm−1 are related to the symmetric and asymmetric stretching C-H vibration of methyl and methylene groups of PMMA matrix (). The high intensity peak of 1,732 cm−1 has been slightly shifted to 1,760 cm−1 and increased the peak intensity in the spectrum of TiO2–PEEK/PMMA, which confirms the functionalization of TiO2 by the carboxylic group.[Citation34] The presence of polymer C-H (bending vibrations) groups was also confirmed by the peaks in the range of 1,440 and 1,385 cm−1. The broad and intense bands perceived in the range 3,110 cm−1 O-H stretching vibrations and 1,615 cm−1 (O-H bending vibrations) are ascribed to hydroxyl groups of absorbed water molecules.
The results from the XRD investigation of the pure nano-TiO2 and nanoparticle-incorporated PEEK/PMMA nanocomposites are presented in . The diffraction signals of the synthesized samples are exhibited from 20° to 80° and product entailed of the characteristic diffraction patterns of anatase (JCPDS 21–1272) nano-crystalline TiO2 structure. The XRD pattern analysis of nanocomposites () displays typical diffraction peaks of anatase TiO2 nanoparticles, which confirmed that the crystalline TiO2 nanoparticles are homogeneously dispersed into the PMMA/PEEK copolymer matrixes by emulsion polymerization. These results concluded that the crystalline structure of TiO2 has not affected by the incorporation with polymer matrixes during polymerization.
SEM and TEM analyses
The surface morphology and dispersion of the synthesized TiO2 nanoparticles onto PEEK/PMMA nanocomposite matrix were analyzed and displayed by the FE-SEM and TEM microscopic techniques. Typical differently magnified FE-SEM images of raw TiO2 and anatase nano-TiO2-incorporated PEEK/PMMA composites are shown in . The SEM image of TiO2–PEEK/PMMA (a and b) exhibited that functionalized nanoparticles were effectively and uniformly dispersed into the polymer matrix in the absence of particle agglomeration. The EDX spectrum (c) of the composites contains the chief element peaks of Ti, which confirms the successful incorporation of TiO2 nanoparticles onto the polymer matrixes, and the peaks of carbon (C) and oxygen (O) were confirmed the formation of polymers under polymerization process. The TEM micrograph () of both the functionalized TiO2 and incorporated TiO2 nanoparticles indicated that the average particle sizes probably are about 30–40 nm. PEEK/PMMA polymeric matrixes show spherical TiO2 particles which appear as small dark particles over the light sheet-like background of polymer matrixes. The particles were monodispersed with a limited degree of aggregation. The TEM images clearly displayed the synthesized samples with the assistance of PEEK/PMMA polymers, which was monodispersed in nature with a very limited degree of agglomeration, and most of the nanoparticles are smooth spherical in nature.
Antibacterial activities and cytocompatibility
It is projected that the nano-TiO2-incorporated copolymeric matrixes have been effectively enhanced antibacterial activities compared to the pure anatase TiO2 nanoparticles against two different bacterial pathogens such as S. aureus and E. coli. exhibits that the inactivation of bacterial cells on the functionalized TiO2-encapsulated polymer matrix surfaces visualized by CLSM images (). The survival percentage of bacterial growth can be calculated by % survival = B/A × 100. The surface-functionalized nanp-TiO2– PEEK/PMMA polymer nanocomposites have been given the excellent killing efficiency of 99% under dark conditions in the short time periods. Especially, the TiO2 nanoparticles with PMMA polymeric group with the assistance of PEEK displayed excellent antibacterial efficacy because the selection of polymer groups in the nanocomposites provided support for the extra antimicrobial activity to the TiO2 composites. The cytocompatibility of the synthesized nanocomposites has been demonstrated through the percentages of dental pulp cells survival after treated with different concentrations of synthesized samples. The TiO2 nanoparticle-incorporated PEEK/PMMA nanocomposite has great cell survival percentages comparable to the PEEK/PMMA copolymeric composite in the absence of TiO2 (). These results of cell viability with this nanocomposite have been suggested that TiO2 Nanoparticles (NPs) are highly supported and promoted cell adhesion and spreading of dental pulp cells and it exhibited greater activity and promising for cell compatibility comparable to nanocomposites without NPs.
Figure 5. CLSM images of bacterial cell death and plot of % survival versus on the TiO2 and TiO2loaded PEEK/PMMA nanocomposite against E. coli and S. aureus.
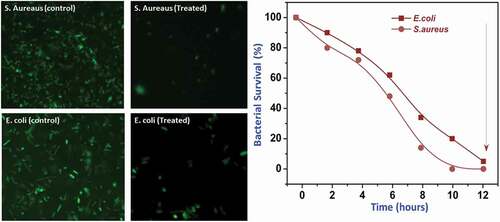
Figure 6. Quantified cell viability (a) of the PEEK/PMMA with and without TiO2 nanoparticles at 1, 3, and 5 days of culture using dental pulp cells and (b) 3D printed prosthesis by using TiO2-incorporated PEEK/PMMA nanocomposite.
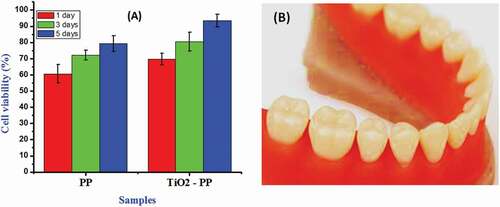
Computer aided design-computer aided manufacturing substractive method was used for denture manufacturing in clinical practice with numerous disadvantages regarding more cost, central manufacturing, and importantly need for strong bonding between tooth and denture base with a high risk of bacterial pathogen adulteration. In recent researches, 3D printing technology habitually applied in dentistry model for engineering surgical patterns for dental implant supplement, provisional crowns, and resin replicas, and also, it has some more limitations due to the properties of the usage of photo-catalytic materials. To overcome these problems in the dental material manufacturing, a nanocomposite prepared with effective and favorable TiO2 nanoparticles loaded with PEEK/PMMA copolymer matrixes with improved antibacterial properties was projected and successfully exploited for preparing a stereolithographic dental prosthesis.
Conclusion
The bioactive copolymeric nanocomposite with carboxylic acid-functionalized nano-TiO2 was successfully prepared through an emulsion polymerization process, and it has been employed to manufacturing a complete stereolithographic dental prosthesis. The spectral analyses are clearly established the successful functionalization of TiO2 and also exhibited the monodispersed nature of particle structure. The microscopic investigations of nanocomposites visualized that particles are formed to spherically shaped with less degree of agglomeration under PEEK/PMMA copolymer matrixes. The bioactive functionalized TiO2 nanoparticles are highly dispersed in the prepared dental composite materials, which has been excellently influenced bactericidal activity on the gram-positive and gram-negative bacterial pathogens and also altered the cytocompatibility properties with dental pulp cells. This facile synthesized nanocomposite was effectively employed for complete denture manufacturing with stereolitographic technique.
Additional information
Funding
References
- Liu, F.; Wang, R.; Cheng, Y.; Jiang, X.; Zhang, Q.; Zhu, M. Polymer Grafted Hydroxyapatite Whisker as a Filler for Dental Composite Resin with Enhanced Physical and Mechanical Properties. Mater. Sci. Eng. C. 2013, 33, 4994–5000. DOI: 10.1016/j.msec.2013.08.029.
- Park, J. H.; Choi, N. S. Equivalent Young’s Modulus of Composite Resin for Simulation of Stress during Dental Restoration. Dental Mater. 2017, 33, e79–e85. DOI: 10.1016/j.dental.2016.10.007.
- Divakar, D. D.; Jastaniyah, N. T.; Altamimi, H. G.; Alnakhli, Y. O.; Muzaheed; Alkheraif, A. A.; Haleem, S.; et al. Enhanced Antimicrobial Activity of Naturally Derived Bioactive Molecule Chitosan Conjugated Silver Nanoparticle against Dental Implant Pathogens. Int. J. Biol. Macromol. 2018, 108, 790–797. DOI: 10.1016/j.ijbiomac.2017.10.166.
- Leal, F. B.; Lima, G. S.; Collares, F. M.; Samuel, S. M.; Petzhold, C. L.; Piva, E.; Ogliari, F. A. Iodonium Salt Improves the Dentin Bonding Performance in an Experimental Dental Adhesive Resin. Int. J. Adhes. Adhesives. 2012, 38, 1–4. DOI: 10.1016/j.ijadhadh.2012.05.008.
- Wang, F.; Shi, L.; He, W. X.; Han, D.; Yan, Y.; Niu, Z. Y.; Shi, S. G. Bioinspired Micro/Nano Fabrication on Dental Implant-Bone Interface. Appl. Surf. Sci. 2013, 265, 480–488. DOI: 10.1016/j.apsusc.2012.11.032.
- Lee, J. H.; Jo, J. K.; Kim, D. A.; Patel, K. D.; Kim, H. W.; Lee, H. H. Nano-Graphene Oxide Incorporated into PMMA Resin to Prevent Microbial Adhesion. Dental Mater. 2018, 34, e63–e72. DOI: 10.1016/j.dental.2018.01.019.
- Pandey, S.; Goswami, G. K.; Nanda, K. K. Green Synthesis of Biopolymer-Silver Nanoparticle Nanocomposite: An Optical Sensor for Ammonia Detection. Int. J. Biol. Macromol.. 2012, 51, 583–589. DOI: 10.1016/j.ijbiomac.2012.06.033.
- Zeng, S.; Yong, K.-T.; Roy, I.; Dinh, X.-Q.; Yu, X.; Luan, F. A Review on Functionalized Gold Nanoparticles for Biosensing Applications. Plasmonics. 2011, 6, 491–506. DOI: 10.1007/s11468-011-9228-1.
- Chabot, V.; Higgins, D.; Yu, A.; Xiao, X.; Chen, Z.; Zhang, J. A Review of Graphene and Graphene Oxide Sponge: Material Synthesis and Applications to Energy and the Environment. Energy Environ. Sci. 2014, 7, 1564. DOI: 10.1039/c3ee43385d.
- Jia, X.; Wang, C.; Ranganathan, V.; Napier, B.; Yu, C.; Chao, Y.; Forsyth, M.; Omenetto, F. G.; MacFarlane, D. R.; Wallace, G. G. A Biodegradable Thin-Film Magnesium Primary Battery Using Silk Fibroin-Ionic Liquid Polymer Electrolyte. ACS Energy Lett. 2017, 2, 831–836. DOI: 10.1021/acsenergylett.7b00012.
- Shevach, M.; Maoz, B. M.; Feiner, R.; Shapira, A.; Dvir, T. Nanoengineering Gold Particle Composite Fibers for Cardiac Tissue Engineering. J. Mater. Chem. B. 2013, 1, 5210. DOI: 10.1039/c3tb20584c.
- Canché-Escamilla, G.; Duarte-Aranda, S.; Toledano, M. Synthesis and Characterization of Hybrid Silica/PMMA Nanoparticles and Their Use as Filler in Dental Composites. Mater. Sci. Eng. C. 2014, 42, 161–167. DOI: 10.1016/j.msec.2014.05.016.
- Topouzi, M.; Kontonasaki, E.; Bikiaris, D.; Papadopoulou, L.; Paraskevopoulos, K. M.; Koidis, P. Reinforcement of a PMMA Resin for Interim Fixed Prostheses with Silica Nanoparticles. J. Mech. Behav. Biomed. Mater. 2017, 69, 213–222. DOI: 10.1016/j.jmbbm.2017.01.013.
- Kojima, N.; Yamada, M.; Paranjpe, A.; Tsukimura, N.; Kubo, K.; Jewett, A.; Ogawa, T. Restored Viability and Function of Dental Pulp Cells on Poly-Methylmethacrylate (PMMA)-Based Dental Resin Supplemented with N-Acetyl Cysteine (NAC). Dental Mater. 2008, 24, 1686–1693. DOI: 10.1016/j.dental.2008.04.008.
- Ouyang, L.; Sun, Z.; Wang, D.; Qiao, Y.; Zhu, H.; Ma, X.; Liu, X. Smart Release of Doxorubicin Loaded on Polyetheretherketone (PEEK) Surface with 3D Porous Structure. Colloids Surf. B Biointerfaces. 2018, 163, 175–183. DOI: 10.1016/j.colsurfb.2017.12.045.
- Najeeb, S.; Zafar, M. S.; Khurshid, Z.; Siddiqui, F. Applications of Polyetheretherketone (PEEK) in Oral Implantology and Prosthodontics. J. Prosthodont. Res. 2016, 60, 12–19. DOI: 10.1016/j.jpor.2015.10.001.
- Galler, K. M.; D’Souza, R. N.; Hartgerink, J. D. Biomaterials and Their Potential Applications for Dental Tissue Engineering. J. Mater. Chem. 2010, 20, 8730. DOI: 10.1039/c0jm01207f.
- Zhao, X.; Lui, Y. S.; Choo, C. K. C.; Sow, W. T.; Huang, C. L.; Ng, K. W.; Tan, L. P.; Loo, J. S. C. Calcium Phosphate Coated Keratin–PCL Scaffolds for Potential Bone Tissue Regeneration. Mater. Sci. Engineering: C. 2015, 49, 746–753. DOI: 10.1016/j.msec.2015.01.084.
- Wan, C.; Chen, B. Synthesis and Characterization of Biomimetic Hydroxyapatite/Sepiolite Nanocomposites. Nanoscale. 2011, 3, 693–700. DOI: 10.1039/c0nr00650e.
- Suhailath, K.; Jayakrishnan, P.; Naufal, B.; Periyat, P.; Jasna, V. C.; Ramesan, M. T. Synthesis by In Situ-Free Radical Polymerization, Characterization, and Properties of Poly (N-Butyl Methacrylate)/Samarium-Doped Titanium Dioxide Nanoparticles Composites. Adv. Polymer Technol. 2018, 37, 1114–1123. DOI: 10.1002/adv.21770.
- Ramesan, M. T.; Santhi, V.; Bahuleyan, B. K.; Al-Maghrabi, M. A. Structural Characterization, Material Properties and Sensor Application Study of In Situ Polymerized Polypyrrole/Silver Doped Titanium Dioxide Nanocomposites. Mater. Chem. Phys. 2018, 211, 343–354. DOI: 10.1016/j.matchemphys.2018.02.040.
- Khattab, R. M.; Badr, H. A.; Zawrah, M. F. Effect of Processing Techniques on Properties of Porous TiO2 and TiO2/hydroxyapatite Composites. Ceramics Int. 2018, 44, 8643–8649. DOI: 10.1016/j.ceramint.2018.02.084.
- Shim1, S.-C.; Choe1, B.-H.; Jang2, I.-S.; Choi2, D.-S.; Lee2, J.-K.; Cha2, B.-K.; cwy@gwnu. ac. k.; Choi1, W.-Y. Effect of TiO2 Nanotube Arrays on Osseointegration for Dental Implant. Advanced Mater. Res. 2014, 922, 71–74. DOI: 10.4028/www.scientific.net/AMR.922.71.
- Kandiah, K.; Muthusamy, P.; Mohan, S.; Venkatachalam, R. TiO2-graphene Nanocomposites for Enhanced Osteocalcin Induction. Mater. Sci. Eng. C. 2014, 38, 252–262. DOI: 10.1016/j.msec.2014.02.010.
- Ronald, S.; Mills, D. Fibrochondrocyte Growth and Functionality on TiO2 Nanothin Films. J. Funct. Biomater. 2016, 7, 15. DOI: 10.3390/jfb7020015.
- Wu, S.; Weng, Z.; Liu, X.; Yeung, K. W. K.; Chu, P. K. Functionalized TiO2 Based Nanomaterials for Biomedical Applications. Adv. Funct. Mater. 2014, 24, 5464–5481. DOI: 10.1002/adfm.201400706.
- Amaravathy, P.; Sathyanarayanan, S.; Sowndarya, S.; Rajendran, N. Bioactive HA/TiO2 Coating on Magnesium Alloy for Biomedical Applications. Ceramics Int. 2014, 40, 6617–6630. DOI: 10.1016/j.ceramint.2013.11.119.
- Kong, H.; Song, J.; Jang, J. Photocatalytic Antibacterial Capabilities of TiO2-biocidal Polymer Nanocomposites Synthesized by a Surface-Initiated Photopolymerization. Environ. Sci. Technol. 2010, 44, 5672–5676. DOI: 10.1021/es1010779.
- Chen, S.; Guo, Y.; Chen, S.; Yu, H.; Ge, Z.; Zhang, X.; Zhang, P.; Tang, J. Facile Preparation and Synergistic Antibacterial Effect of Three-Component Cu/TiO2/CS Nanoparticles. J. Mater. Chem. 2012, 22, 9092. DOI: 10.1039/c2jm00063f.
- Pant, H. R.; Pant, B.; Sharma, R. K.; Amarjargal, A.; Kim, H. J.; Park, C. H.; Tijing, L. D.; Kim, C. S. Antibacterial and Photocatalytic Properties of Ag/TiO2/ZnO Nano-Flowers Prepared by Facile One-Pot Hydrothermal Process. Ceramics Int. 2013, 39, 1503–1510. DOI: 10.1016/j.ceramint.2012.07.097.
- Dey, T.; Roy, P.; Fabry, B.; Schmuki, P. Anodic Mesoporous TiO2 Layer on Ti for Enhanced Formation of Biomimetic Hydroxyapatite. Acta Biomater. 2011, 7, 1873–1879. DOI: 10.1016/j.actbio.2010.11.011.
- Liu, J.; Wang, Y.; Delai Sun, D. Enhanced Performance of Hybrid Solar Cells Using Carboxylic Acid-Functionalized Graphene Oxide Supported TiO2nanorod Composites. Mater Lett. 2013, 95, 178–181. DOI: 10.1016/j.matlet.2012.12.092.
- Totu, E. E.; Nechifor, A. C.; Nechifor, G.; Aboul-Enein, H. Y.; Cristache, C. M. Poly(Methyl Methacrylate) with TiO2 Nanoparticles Inclusion for Stereolitographic Complete Denture Manufacturing − The Fututre in Dental Care for Elderly Edentulous Patients? J. Dent. 2017, 59, 68–77. DOI: 10.1016/j.jdent.2017.02.012.
- Pastrana-Martínez, L. M.; Morales-Torres, S.; Carabineiro, S. A. C.; Buijnsters, J. G.; Figueiredo, J. L.; Silva, A. M. T.; Faria, J. L. Photocatalytic Activity of Functionalized Nanodiamond-TiO2 Composites Towards Water Pollutants Degradation under UV/Vis Irradiation. Appl. Surf. Sci. 2018, 458, 839–848. DOI: 10.1016/j.apsusc.2018.07.102.