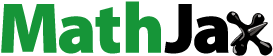
ABSTRACT
Effects of UV and X-ray treatments on oxidative stability of soybean oil were evaluated during accelerated storage. Furthermore, antioxidant activity of neroli oil incorporated into soybean oil was compared with those of BHT and β-carotene. UV and X-ray treated samples had significantly higher peroxide (105.00 and 191.99 meq O2/kg, respectively), anisidine (62.30 and 153.80 mg/kg, respectively), and Totox values (272.3 and 453.43, respectively) than non-irradiated samples (75.74 meq O2/kg, 26.50 mg/kg, and 177.98, respectively). The X-ray radiation accelerated the oxidation of soybean oil more significantly than UV radiation did. This could be due to the fact that X-ray radiation has a higher energy content. In addition, neroli oil proved its antioxidant activity in soybean oil. Nonetheless, neroli oil had a lower antioxidant activity than BHT – a chemical agent – while β-carotene was neither antioxidant nor pro-oxidant. In conclusion, neroli oil can improve the oxidative stability of irradiated and non-irradiated soybean oil.
Introduction
Irradiation relates to electromagnetic radiation through two categories of ionizing and non-ionizing mechanisms. X-rays, electron beams, and gamma-rays are forms of ionizing radiation. [Citation1] Ionizing radiation, as a process, produces charged atoms or molecules (ions) as they knock electrons out of their normal orbits in atoms. Considering the effects of radiation, the decomposition of these primary ions or their reaction with neighboring molecules (secondary effects) can chemically degrade major food components. Various products are formed from irradiated triglycerides (lipids) and fatty acids. Furthermore, the generated radicals can react with each other and create recombined products. [Citation2] The nature of lipids, the existence of antioxidants, and radiation conditions can play important roles in affecting the occurrence of oxidation reactions. [Citation3] Previous investigations have shown that ionizing types of radiation can induce oxidation. Braunrath et al. [Citation4] applied 4 and 10 kGy dosages of gamma-rays as initiators of oxidation processes in rapeseed oil and evaluated its effects on different tocopherols. They found higher peroxide values (PVs; primary oxidation products) in samples exposed to radiation and observed the antioxidant activity of gamma- and delta-tocopherols under the mentioned conditions. El-Rawas et al. [Citation5] studied the effect of electron beam on quality of peanut butter during storage and reported that the PV and thiobarbituric acid reactive substances (TBARS; secondary oxidation products) were significantly increased by increasing the dose of electron beam.
Non-ionizing radiations involving UV, visible light, microwave, and infrared are not strong enough to ionize atoms and molecules. [Citation1] UV radiation is a different initiator of reactions which activates the process of lipid oxidation. Nonetheless, UV radiation cannot generate free radicals easily. To provide the required amount of energy, heating is applied as another factor to catalyze the oxidation process. [Citation6] Wang et al. [Citation7] evaluated the effect of carnosic acid on oxidative stability of squalene exposed to UV radiation (UVA and UVB), as accelerated oxidation reactions, for 92 h at 25°C. Their results showed that carnosic acid exhibited antioxidant activity in squalene.
In 2014, the total soybean oil (SBO) production in the world was 45.70 million tons. [Citation8] SBO is a good source of ω-3 (7–8% alpha-linolenic acid) and ω-6 (50–57% linoleic acid) of polyunsaturated fatty acids (PUFAs) and also different tocopherols. For this reason, the SBO is important from a nutritional point of view. It is also appreciated for its abundance, economic value, and wide functionality. [Citation9] Despite these desirable features, the negative impact of oxidation reactions is a major issue in SBO. [Citation6]
The genus Citrus is from the Rutaceae family and is a suitable candidate for essential oil extraction. This genus comprises eight main species [Citation10], among which sour orange (Citrus aurantium L.) is an important species having essential oil. [Citation11] Sour orange natively belongs to South-east Asia, which then spread westward. [Citation12] Sour orange essential oil is known as neroli oil. Antifungal [Citation13], antimicrobial [Citation14], antioxidant [Citation15], and anti-inflammatory activities [Citation16] of neroli oil have been identified. Wang et al. [Citation17] evaluated the antioxidant activity of neroli oil. Their results showed that the reducing power of neroli bigarade oil (in comparison with butylated hydroxyanisole) and β-carotene bleaching activity were 5.05% and 8.14%, respectively.
The objective of this study was to evaluate the effects of UV radiation and X-ray radiation on oxidative stability of SBO. In addition, the antioxidant activity of neroli oil was compared to those of butylated hydroxytoluene (BHT) and β-carotene.
Materials and methods
Chemicals
All chemicals and reagents were of analytical grade and were purchased from Merck (Darmstadt, Germany) and Sigma-Aldrich (St. Louis, MO).
Neroli oil
Neroli oil was provided by the Manely Company (Shiraz, Iran). Its chemical composition and radical scavenging activity were also measured, as will be described subsequently.
Chemical composition
A gas chromatography (GC) (7890A, Agilent Technologies, Santa Clara, CA) equipped with a HP-5MS capillary column (5% Phenyl Polysilphenylene-siloxane; 30 m length × 0.25 mm internal diameter; 0.25 μm film thickness) was used. Mass spectrometer detector (5975C, Agilent Technologies, Santa Clara, CA) operated at 70 eV electron ionization energy, in the electronic ionization mode, 0.5 s/scan, and a mass range of 50–480 atomic mass units. One μL of diluted neroli oil (1:10 with n-hexane) was injected into the GC/MS. Helium was used as the carrier gas at a flow rate of 1 mL/min. The injector was in split mode (at a ratio of 1:50). The oven temperature was programmed to increase from 60°C to 220°C at a rate of 5°C/min. The final temperature was 220°C and remained accordingly for 8 min. The total run time was 40 min. The interface line temperature was 250°C. The MSD ChemStation Software (G1701EA, E.02.01.1177, Agilent Technologies, and Santa Clara, CA) was applied to analyze mass spectra and chromatograms. The compounds were identified by comparing their mass spectral fragmentation patterns with those in the data bank (Wiley/NBS). Furthermore, quantitative analysis of neroli oil compounds was made under the same chromatographic conditions using a GC coupled with a flame ionization detector (FID). The relative data for percentage were received from the electronic integration of chromatogram peak areas.
Free radical scavenging activity
The 2,2-diphenyl-1-picrylhydrazyl (DPPH) scavenging activity of neroli oil was evaluated according to the method used by Eblaghi et al. [Citation18] with some modifications. Three mL of methanolic solutions of neroli oil at different concentrations of 1, 2, 5, and 10 mg/mL were mixed with 1 mL of DPPH solution (0.2 mM). After 60 min of incubation at room temperature under dark conditions, the absorbance values were measured at 517 nm against blank (with the same chemicals except neroli oil). The IC50 value was then calculated. IC50 value is defined as a concentration of neroli oil inhibiting 50% DPPH activity, obtained from the graph plotting the inhibition percentage against neroli oil concentration.
SBO
Refined, bleached, and deodorized (RBD) SBO, without any added antioxidant, was provided by Narges Shiraz Oil Company (Shiraz, Iran) which was kept refrigerated until future use and analysis.
Initial characteristic of SBO
PV and anisidine value (AV) of SBO were determined according to AOCS (American Oil Chemists’ Society) Official Methods Cd 8–53 and Cd 18–90, respectively. [Citation19] The Totox value (TV = 2 PV + AV) was also calculated. Specific extinction coefficients at 232 nm (K232) and 268 nm (K268) were also measured according to the AOCS Official Method Ch 5–91. [Citation19]
Fatty acid composition of SBO
SBO fatty acid methyl esters (FAMEs) were prepared based on the method described by Golmakani et al. [Citation20] A GC apparatus (SP-3420A, Beijing, China) was equipped with an FID and a BPX-70 fused silica capillary column (30 m × 0.25 mm internal diameter, 0.25 μm film thickness). Injector was in the split mode with a ratio of 1:10. The carrier gas was nitrogen. One μL of FAMEs was injected. The oven temperature was programmed according to the following: the initial temperature was held at 140°C for 5 min. Thereafter, the temperature was increased to 180°C at a rate of 20°C/min and was kept for 9 min. Finally, the temperature was increased to 200°C at a rate of 20°C/min and was maintained for 3 min. Injector and detector temperatures were 250°C and 300°C, respectively. The results were expressed as percentages of relative peak areas. Based on the fatty acid profile, the iodine value (IV) was calculated according to AOCS Official Method Cd 1c-85.
Accelerated storage of SBO samples
Neroli oil (0.10%, w/w), BHT (0.01%, w/w), and β-carotene (0.01%, w/w) were added to SBO. The control was SBO without any added antioxidant. All samples were exposed to UVC radiation (31.40 KGy, energy content of 8 eV; TUV30W G30T8, Philips lightening, Amsterdam, Netherland) and X-ray radiation (1 KGy, 140 KVp, radiography system, Gilardoni, Italy). Irradiated (UV and X-ray radiations) and non-irradiated samples were then stored in an oven at 65 ± 1°C for 21 days. PV, AV, and TV of samples were determined every 7 days. Also, K232 and K268 of samples were measured at the end of storage period (day 21).
Induction period (IP), Fvalue, and relative antioxidant activity (RAA) indices were also measured. IP was the number of days required to reach the PV of 20 meq O2/kg. PVs higher than 20 indicated poor flavor of SBO. [Citation9] The Fvalue and RAA of samples were determined according to the following equations:
where IPs is the IP of SBO containing antioxidant (neroli oil, BHT, and β-carotene) and IPc is the IP of control SBO. AH is the concentration of antioxidant in proper units. [Citation21,Citation22]
Statistical analysis
All experiments were performed in triplicate determinations and the data are reported as mean values. Standard deviation values and bars are presented in tables and figures, respectively. A general linear model procedure from SAS (Statistical Analysis Software, version 9.1; SAS Institute Inc. Cary, NC) was used for mean comparison and significant differences (P < 0.05) were calculated using Duncan’s multiple range test.
Results and discussion
Neroli oil
Chemical composition
illustrates the chemical composition of neroli oil. Monoterpenes (78%) were the main class of compounds found in neroli oil. Linalool (oxygenated monoterpene; 23.19%), limonene (monoterpene hydrocarbon; 15.39%), farnesol (2Z,6E) (oxygenated sesquiterpene; 9.09%), nerolidol-E (oxygenated sesquiterpene; 8.91%), β-pinene (monoterpene hydrocarbon; 8.71%), and trans-β-ocimene (monoterpene hydrocarbon; 7.95%) were the main components of neroli oil.
Table 1. Chemical composition of neroli oil.
Free radical scavenging activity
IC50 value of neroli oil was 7.91 mg/mL. Oxygenated monoterpenes are generally known to have high antioxidant activity due to their strongly activated methylene groups. The antioxidant activity of neroli oil is likely to be powered by such compounds. [Citation23] Duarte et al. [Citation24] evaluated DPPH radical scavenging activity and performed β-carotene bleaching test on linalool. They reported that linalool had adequate radical scavenging activity and also capable of inhibiting lipid peroxidation to some extent.
SBO
Initial characteristics of SBO
PV, AV, and TV of SBO were 1.90 ± 0.00, 2.18 ± 0.04, and 6.16 ± 0.04, respectively. Furthermore, K232 and K268 values of SBO were 0.10 ± 0.00 and 0.16 ± 0.00, respectively.
Fatty acid composition of SBO
Palmitic acid (11.82%), stearic acid (4.12%), oleic acid (ω-9; 24.44%), linoleic acid (ω-6; 57.06%), and alpha-linolenic acid (ω3; 8.64%) were identified in SBO. The major category in SBO was PUFA which comprised 65.70% of total fatty acids. Moreover, the IV of SBO was 142.
Accelerated storage of SBO samples
PV, AV, and TV
), ), and ) show PVs of non-irradiated, UV irradiated, and X-rayed samples, respectively. Irradiated samples had significantly higher PVs than non-irradiated samples. UV radiation can cause the production of radicals and, as a result, initiate autoxidation. [Citation25] Despite the fact that UV radiation was applied at higher doses to the samples, but the X-ray radiation resulted in significantly higher PVs due to its higher energy content. Bhatti et al. [Citation26] evaluated PV of gamma-irradiated peanut. Their results showed that PV of peanut oil increased by increasing radiation dose. They stated that this PV increase can be due to the bond cleavage of peanut oil.
Figure 1. (a) Peroxide values, anisidine values, and (c) Totox values of non-irradiated soybean oil samples during accelerated storage.
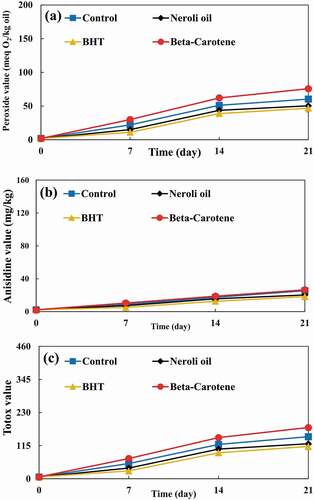
Figure 2. (a) Peroxide values, anisidine values, and (c) Totox values of UV irradiated soybean oil samples during accelerated storage.

Figure 3. (a) Peroxide values, anisidine values, and (c) Totox values of X-rayed soybean oil samples during accelerated storage.
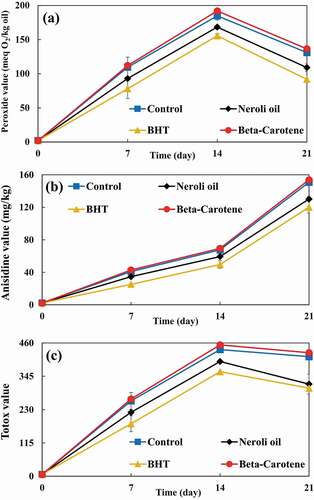
In all samples, PVs were increased during storage except for the PVs of X-rayed samples which decreased after 14 days of storage. This phenomenon is due to the higher energy content of X-ray radiation and, thus, its ability to accelerate lipid oxidation in SBO samples. Therefore, X-ray radiation can decompose the SBO’s hydroperoxides faster and convert them into secondary oxidation products. X-ray radiation (as an ionizing radiation) leads to the autoxidation of fatty acids and triglycerides. Furthermore, it can accelerate the autoxidation process. [Citation2]
The PVs of samples supplemented with neroli oil and BHT were significantly lower than that of the control. In contrast, β-carotene caused a significantly higher PV than that of the control. Wang et al. [Citation27] evaluated the effect of UV radiation on oxidative stability of pine nut oil. They reported that the PV of control was significantly higher than PVs of samples supplemented with carnosic acid and BHT after 4 days of storage.
The AVs of non-irradiated, UV irradiated, and X-rayed samples are illustrated in ), ), and ), respectively. The AVs of irradiated and non-irradiated samples were significantly increased by prolonging the storage period. The X-rayed samples had the highest AVs. Also, irradiated samples had significantly higher AVs than non-irradiated ones. UV radiation is able to degrade hydroperoxides, carbonyl, and other oxygen-containing compounds. [Citation25] The degradation can result in the production of more primary and secondary oxidation products in SBO.
Although samples supplemented with neroli oil and BHT had significantly lower AVs than the control, but the AV of sample supplemented with β-carotene was significantly higher than that of the control. In other words, β-carotene caused secondary oxidation products to be produced at a significantly higher rate. Kanatt et al. [Citation28] investigated TBARS of gamma-rayed lamb meat supplemented with mint extract. Their results demonstrated that samples containing mint extract had significantly lower TBARS than samples without mint extract.
), ), and ) show the TVs of non-irradiated, UV irradiated, and X-rayed samples, respectively. TVs of all samples showed increasing trend during the storage period, with the exception of X-rayed samples. The TVs of X-rayed samples decreased after 14 days of storage until the end of the storage period. Nonetheless, their TVs were significantly higher than those of non-irradiated and UV irradiated samples. Also, non-irradiated samples had the lowest TVs. Furthermore, the TV of sample supplemented with β-carotene was significantly higher than that of the control. Even so, samples added with neroli oil and BHT had significantly lower TVs than the control.
IP, Fvalue, and RAA
shows indices of IP, Fvalue, and RAA of SBO samples. Among non-irradiated samples, the IP of sample supplemented with neroli oil was significantly higher than that of the control, implying its capability of improving SBO shelf life. Samples added with BHT and β-carotene had the highest and the lowest IPs, respectively. There were no significant differences among IPs of X-rayed samples. X-ray radiation caused severe oxidation due to its high energy content; therefore, IPs of the control and other samples were not significantly different. Among UV-irradiated samples, significant differences were not observed between IPs of sample supplemented with neroli oil and the control. Also, incorporating sample with β-carotene caused the lowest IP, even lower than that of the control. Accordingly, β-carotene was pro-oxidant under UV radiation. Relevant to this context, Hashemi et al. [Citation29] evaluated the effect of Marzeh Khuzestani essential oil on oxidation of UV irradiated canola oil. They reported that the IP of canola oil containing Marzeh Khuzestani essential oil was higher than that of control.
Table 2. Induction period (IP), Fvalue, and relative antioxidant activity (RAA) of soybean oil samples during accelerated storage.
Fvalue of neroli oil was significantly higher than that of the control. In other words, neroli oil can significantly protect SBO against UV and X-ray radiations. BHT had the highest Fvalue. There were no significant differences between the Fvalue of β-carotene and the control. In other words, β-carotene cannot effectively prevent the oxidation of SBO.
RAA depends on the concentration of antioxidant agents. RAA of neroli oil was similar to that of the control. The RAA of BHT, as a synthetic antioxidant, was significantly higher than that of neroli oil. Nonetheless, neroli oil is basically a natural antioxidant and can be applied at higher concentrations with optimistic results.
K232 and K268
shows K232 (conjugated diene compounds) and K268 (conjugated triene compounds) of SBO samples at the end of storage period. The K232 of X-rayed samples were significantly higher than that of non-irradiated and UV-irradiated samples. There were no significant differences among the K232 of neroli oil, BHT, and the control. β-Carotene had the highest conjugated diene compounds. Keramat et al. [Citation30] evaluated oxidative stability of virgin olive oil supplemented with Zataria multiflora and Rosmarinus officinalis essential oils in comparison with those of BHT and β-carotene. They reported that virgin olive oil supplemented with BHT and essential oils had significantly lower K232 than control at the end of storage period, while K232 of virgin olive oil containing β-carotene was higher than that of control at the same time.
There were no significant differences among the K268 of non-irradiated, UV-irradiated, and X-rayed samples. Also, there were no significant differences between the K268 of the control and the samples that were supplemented with the various antioxidants.
Conclusion
In this study, the effects of UV and X-ray radiations were evaluated on the oxidative stability of SBO that was incorporated with neroli oil. Irradiation, especially X-ray radiation, accelerated the oxidation of SBO. Irradiated samples had higher primary and secondary oxidation products compared to non-irradiated samples. Neroli oil showed antioxidant activity in irradiated and non-irradiated SBO, although to different extents.
Acknowledgments
This research project was financially supported by Shiraz University. We would like to thank Narges Shiraz Oil Company for providing SBO. In addition, we thank Mohsen Hamedpour-Darabi for editing the research language.
Additional information
Funding
References
- Alothman, M.; Bhat, R.; Karim, A. A. Effects of Radiation Processing on Phytochemicals and Antioxidants in Plant Produce. Trends Food Sci. Technol. 2009, 20, 201–212. DOI: 10.1016/j.tifs.2009.02.003.
- Fan, X. Radiation Chemistry of Food Components. In Food Irradiation Research and Technology, 2nd ed.; Fan, X., Sommers, C. H., Eds.; Wiley-Blackwell: Ames, IA, 2013, pp. 76–78.
- Molins, R. A. Food Irradiation: Principles and Applications; John Wiley & Sons: New York, NY, 2001.
- Braunrath, R.; Isnardy, B.; Solar, S.; Elmadfa, I. Influence of α-, γ-, and δ-tocopherol on the Radiation Induced Formation of Peroxides in Rapeseed Oil Triacylglycerols. Food Chem. 2009, 117, 349–351. DOI: 10.1016/j.foodchem.2009.03.104.
- El-Rawas, A.; Hvizdzak, A.; Davenport, M.; Beamer, S.; Jaczynski, J.; Matak, K. Effect of Electron Beam Irradiation on Quality Indicators of Peanut Butter over a Storage Period. Food Chem. 2012, 133, 212–219. DOI: 10.1016/j.foodchem.2011.12.078.
- Shahidi, F. Bailey’s Industrial Oil and Fat Products: Chemistry, Properties, and Health Effects, 6th ed.; Wiley–Interscience: Hoboken, NJ, 2005, 1, 49
- Wang, H.; Wang, H.; Yang, L.; Zu, Y. G.; Liu, F.; Liu, T. T. Comparative Effect of Carnosic Acid, BHT and α-tocopherol on the Stability of Squalene under Heating and UV Irradiation. Food Res. Int. 2011, 44, 2730–2734. DOI: 10.1016/j.foodres.2011.05.036.
- FAOSTAT. http://www.fao.org/faostat/en/#data/QD/visualize
- O’Brien, R. D. Fats and Oils: Formulating and Processing for Applications; CRC press: Boca Raton, FL, 2008.
- Shahidi, F.; Zhong, Y. Citrus Oils and Essences. In Bailey’s Industrial Oil and Fat Products: Specialty Oils and Oil Products, Shahidi, F., Ed.; Wiley-Interscience: Hoboken, NJ, 2005, 3, 49.
- Başer, K. H. C.; Buchbauer, G. Handbook of Essential Oils: Science, Technology, and Applications; CRC: New York, 2009.
- Khan, I. A. Citrus Genetics, Breeding and Biotechnology; CABI: Wallingford, UK, 2007.
- Trabelsi, D.; Hamdane, A. M.; Said, M. B.; Abdrrabba, M. Chemical Composition and Antifungal Activity of Essential Oils from Flowers, Leaves and Peels of Tunisian Citrus Aurantium against Penicillium Digitatum and Penicillium Italicum. J. Essent. Oil Bear. Plants. 2016, 19, 1660–1674. DOI: 10.1080/0972060x.2016.1141069.
- Rahimi, A.; Hashemi, P.; Talei, G. R.; Borzuei, M.; Ghiasvand, A. R. Comparative Analyses of the Volatile Components of Citrus Aurantium L. Flowers Using Ultrasonic-Assisted Headspace SPME and Hydrodistillation Combined with GC-MS and Evaluation of Their Antimicrobial Activities. Anal. Bioanal. Chem. Res. 2014, 1, 83–91.
- Hsouna, A. B.; Hamdi, N.; Halima, N. B.; Abdelkafi, S. Characterization of Essential Oil from Citrus Aurantium L. Flowers: Antimicrobial and Antioxidant Activities. J. Oleo Sci. 2013, 62, 763–772. DOI: 10.5650/jos.62.763.
- Khodabakhsh, P.; Shafaroodi, H.; Asgarpanah, J. Analgesic and Anti-Inflammatory Activities of Citrus Aurantium L. Blossoms Essential Oil (Neroli): Involvement of the Nitric Oxide/Cyclic-Guanosine Monophosphate Pathway. J. Nat. Med. 2015, 69, 324–331. DOI: 10.1007/s11418-015-0896-6.
- Wang, H. F.; Yih, K. H.; Yang, C. H.; Huang, K. F. Anti-Oxidant Activity and Major Chemical Component Analyses of Twenty-Six Commercially Available Essential Oils. J. Food Drug Anal. 2017, 25, 881–889. DOI: 10.1016/j.jfda.2017.05.007.
- Eblaghi, M.; Khajehie, N.; Golmakani, M.-T.; Eskandari, M. H. Investigating the Effects of Microwave-Assisted Hydrodistillation on Antioxidant and Antifungal Activities of Tanacetum Polycephalum and Artemisia Chamaemelifolia Essential Oils. J. Essent. Oil Res. 2016, 28, 528–539. DOI: 10.1080/10412905.2016.1175977.
- AOCS. Official Methods and Recommended Practices of the American Oil Chemists’ Society; AOCS Press: Champaign, IL, 2000.
- Golmakani, M.-T.; Rezaei, K.; Mazidi, S.; Razavi, S. H. Effect of Alternative C2 Carbon Sources on the Growth, Lipid, and γ-linolenic Acid Production of Spirulina (Arthrospira Platensis). Food Sci. Biotechnol. 2012, 21, 355–363. DOI: 10.1007/s10068-012-0047-8.
- Antolovich, M.; Prenzler, P. D.; Patsalides, E.; McDonald, S.; Robards, K. Methods for Testing Antioxidant Activity. Analyst. 2002, 127, 183–198. DOI: 10.1039/b009171p.
- Keramat, M.; Golmakani, M.-T.; Aminlari, M.; Shekarforoush, S. S. Comparative Effect of Bunium Persicum and Rosmarinus Officinalis Essential Oils and Their Synergy with Citric Acid on the Oxidation of Virgin Olive Oil. Int. J. Food Prop. 2016, 19, 2666–2681. DOI: 10.1080/10942912.2015.1126722.
- Ammar, A. H.; Bouajila, J.; Lebrihi, A.; Mathieu, F.; Romdhane, M.; Zagrouba, F. Chemical Composition and in Vitro Antimicrobial and Antioxidant Activities of Citrus Aurantium L. Flowers Essential Oil (Neroli Oil). Pak. J. Biological Sci. 2012, 15, 1034–1040. DOI: 10.3923/pjbs.2012.1034.1040.
- Duarte, A.; Luís, Â.; Oleastro, M.; Domingues, F. C. Antioxidant Properties of Coriander Essential Oil and Linalool and Their Potential to Control Campylobacter Spp. Food Control. 2016, 61, 115–122. DOI: 10.1016/j.foodcont.2015.09.033.
- Scrimgeour, C. Chemistry of Fatty Acids. In Bailey’s Industrial Oil and Fat Products: Chemistry, Properties, and Health Effects, 6th ed.; Shahidi, F., Ed.; Wiley–Interscience: Hoboken, NJ. 2005, 1, 17-18.
- Bhatti, I. A.; Ashraf, S.; Shahid, M.; Asi, M. R.; Mehboob, S. Quality Index of Oils Extracted from γ-irradiated Peanuts (Arachis Hypogaea L.) Of the Golden and Bari Varieties. Appl. Radiat. Isot. 2010, 68, 2197–2201. DOI: 10.1016/j.apradiso.2010.05.017.
- Wang, H.; Zu, G.; Yang, L.; Zu, Y. G.; Wang, H.; Zhang, Z. H.; Zhang, Y.; Zhang, L.; Wang, H. Z. Effects of Heat and Ultraviolet Radiation on the Oxidative Stability of Pine Nut Oil Supplemented with Carnosic Acid. J. Agric. Food Chem. 2011, 59, 13018–13025. DOI: 10.1021/jf203454v.
- Kanatt, S. R.; Chander, R.; Sharma, A. Antioxidant Potential of Mint (Mentha Picata L.) In Radiation-Processed Lamb Meat. Food Chem. 2007, 100, 451–458. DOI: 10.1016/j.foodchem.2005.09.066.
- Hashemi, S. M. B.; Mousavi Khaneghah, A.; Khoshnoudi-Nia, S.; Mahdavian Mehr, H. Marzeh Khuzistani Essential Oil as a Natural Antioxidant in Canola Oil under Forced Conditions. Int. Food Res. J. 2013, 20, 2091–2096.
- Keramat, M.; Golmakani, M.-T.; Aminlari, M.; Shekarforoush, S. Oxidative Stability of Virgin Olive Oil Supplemented with Zataria Multiflora Boiss. And Rosmarinus Officinalis L. Essential Oils during Accelerated Storage. J. Food Process. Preserv. 2017, 41, e12951. DOI: 10.1111/jfpp.12951.