ABSTRACT
Peroxidase (POD) was extracted from Prunus domestica and partially purified by three methods: ammonium sulfate precipitation, hydrophobic interaction chromatography, and ion exchange chromatography, respectively. The selected procedure allowed a 26.33-fold purification, and the molecular mass estimated by SDS-PAGE was 58 kDa. The purified enzyme presented enzymatic activity toward guaiacol, pyrogallol, catechol, and showed no activity toward ferulic, caffeic, and p-coumaric acids. In terms of optimum parameters for activity, the pH was 6.5, whereas the temperature was 25°C. The enzyme exhibited high stability in the pH range of 5.0–7.0 and in the temperature range of 25–70°C. The most potent inhibitors of POD were L-cysteine and sodium metabisulfite. The thermal inactivation displayed a first-order kinetic model, with an activation energy of Ea 84.79 ± 2.2 kJ/mol. POD extracted from plums exhibited high stability at high-pressure treatment, maintaining over 50% of the initial activity even at 700 MPa.
Introduction
Fruits and vegetables are of great importance in terms of nutrition and functional quality of fresh or processed products, providing essential biologically active compounds, which are known for their health benefits. The Prunus genus is cultivated worldwide due to its fruits that are considered to be a very rich source of bioactive compounds such as anthocyanins, carotenoids, ascorbic acid, vitamin E, flavonoids, proanthocyanidins, and tannins.[Citation1] In Romania, plums are among the most consumed fruits in various forms as fresh fruit, dried fruit, jams, purees, juices or compotes, the fruits being also used as a source for bioactive compounds in regards to several pharmaceuticals. In this context, structurally speaking, plums are very susceptible to enzymatic browning because of their high phenolic composition. Presently, in the field of food industry, the prevention of enzymatic browning is one of the major concerns of the food industry during processing and storage of fruits and vegetables.[Citation2] This process is catalyzed by the presence of oxidative enzymes, mainly, polyphenol oxidase (PPO) (catechol oxidase, E.C.1.10.3.1) and peroxidase (POD) (hydrogen-peroxide oxidoreductase E.C.1.11.1.7). POD is one of the major enzymatic compounds which catalyze redox reactions of a wide range of phenolic and non-phenolic substrates, being found in bacteria, fungi, algae, plants, and animals. POD catalyzes the oxidation of several compounds such as phenols, aromatic amines, ascorbic acid, indole, in the presence of hydrogen peroxide as an electron acceptor.[Citation3] Plant PODs belong to Class III PODs, being involved in several processes of plant growth, including cell wall metabolism, lignification, defense against pathogens, wound healing, plant maturation. Their ability to catalyze various redox reactions for a wide range of substrates highlights POD as being one of the most important industrial enzymes with applications in different industrial sectors, such as bioremediation, synthetic dye decolorization, polymer synthesis, biosensor development for diagnostic kit – ELISA[Citation4], degradation of wastewater and drinking water, and preparation of detergents.[Citation5] Moreover, POD is one of the main quality deterioration indicator, such as flavor loss and different biodegradation reactions, being also relevant as a browning enzyme that contributes to the darkening of fruit and vegetable products during processing and storage.[Citation6]
The inactivation of POD is essential in order to minimize losses that are caused by enzymatic browning, in such a manner that several methods and technologies have been studied. Currently, one of the most applied methods for the inactivation of oxidative enzymes is thermal treatment, which is also being used to ensure product quality in the food industry. The enzyme represents quite a challenge for large industrial processors due to its high thermostability even at pasteurization temperatures, which can lead to organoleptic and nutritional properties deterioration. Nonetheless, knowing the inactivation parameters is an important factor in optimizing a treatment or a combination of treatments, in order to obtain the desired product. Therefore, each new processing technology provides a unique opportunity to control oxidative enzymes catalyzed reactions. Nowadays, high-pressure treatment is gaining more and more popularity in the food industry, thanks to its capacity to inactivate vegetative cells of microorganisms and enzymes involved in enzymatic browning at temperature values close to room temperature.[Citation7] In this manner, this new and alternative technology provides the possibility to minimize the effect on flavor, color, and nutritional properties in order to obtain food products of high-quality. The inactivation pattern for each enzyme depends on the holding time and the source of enzyme.[Citation7]
To the best of our knowledge, this is the first time that POD from plums was purified with different chromatographic techniques and biochemically characterized, and the first time when the optimum parameters regarding the thermal treatment, high-pressure treatment and also combined high-pressure with thermal treatment were determined for the purified enzyme. The data obtained may be considered as a reference for future research that is of scientific and applicative importance in order to understand the behavioral changes in regards to treatments involved in industrial processing and the determination of effective and modern treatment techniques of fruits and vegetables, thus ensuring the quality of products.
Materials and methods
Materials and chemicals
The fresh plums (P. domestica) of Vinete românești variety were acquired from a local market (Galați, România), during October and November 2016. The analytical grade reagents were purchased from Sigma (Sigma-Aldrich Co, St. Louis, MO).
Enzyme extraction and partial purification from plums
The extraction and purification procedure was performed according to the method proposed by Ioniţǎ et al..[Citation8] To purify the enzyme, three purification phases were taken into account, namely ammonium sulfate precipitation, hydrophobic interaction chromatography (HIC), and ion exchange chromatography (IEC). The crude enzyme extract was subjected to two stages of precipitation with ammonium sulfate 30% and 90%. The resulting extract was dialyzed and concentrated by freeze-drying. The further purification was performed with a ÄKTA pure 25 FPLC (Fast Protein Liquid Chromatography) equipment (GE Healthcare, Uppsala, Sweden). In order to eliminate the ammonium sulfate, the extract was passed through on a HiPrep Phenyl FF 16/10 column for HIC. The active fractions were eluted with 20 mM phosphate buffer (pH 7.5) by using a linear gradient of 0–100%. Afterward, the fractions that presented high enzymatic activities were pooled together and further purified on a Mono Q 5/50 GL Fast Flow IEC column. The elution took place with a linear NaCl gradient increasing from 0 to 1.0 M, in 20 mM phosphate buffer (pH 7.5), at a rate of 1 mL/min. The protein content was assessed in accordance with Bradford method using bovine serum albumin as standard.
Molecular weight determination
The electrophoresis experiments were assessed in a Mini-Protean Tetra Vertical Electrophoresis Cell, Bio-Rad Laboratories (California, USA) according to the Laemmli method. For the stacking and separating gels, the acrylamide content was 4.5% and 12%, respectively. A volume of 25 μL was loaded onto each lane. The protein bands were observed by staining with Silver stain kit (protocol LIT34B, Bio-Rad).
Enzyme activity assay
The reaction mixture to determine the POD activity consisted of 1 mL substrate solution (guaiacol 80 mM and dissolved in McIlvaine buffer, pH 6.5), 100 µL hydrogen peroxide 3% and 100 µL enzyme extract. The blank sample consisted of 1 mL substrate solution and 100 µL ultrapure water. The absorbance at 470 nm was monitored for 10 min using a GBC Cintra 202 UV-Vis spectrophotometer (GBC, Melbourne, Australia). The enzymatic activity was calculated by taking into account the linear part of the curve (optical density vs. time). One unit of enzyme activity was defined as the amount of enzyme that produces an absorbance increase of 0.001/minute/mL at 25ºC. For all tested conditions, the enzymatic activity was calculated as the mean of three experiments.
Optimum pH and pH stability
The optimum pH of POD extracted from plums was determined by using McIlvaine buffer solution at different pH values ranging from 3.0 to 9.0 to prepare the substrate solutions that were further used to assay the POD activity. To establish the pH stability, the enzyme was incubated in McIlvaine buffers of pH 3.0–9.0 and the enzyme activity was assayed over 3 days at the optimum temperature and pH values.
Optimum temperature and temperature stability
The optimum temperature experiments were assessed in the temperature range from 4°C-65°C using a water bath (Julabo F33 MC, Seelbach, Germany). The substrate solutions were incubated for 10 min at the desired temperature. The thermal stability of POD from plums was evaluated at temperatures between 25°C and 85°C, at incubation periods of 0, 10, and 20 min. The thermal inactivation experiments took place at temperatures between 60°C and 85°C, with various incubation times ranging from 0 to 90 min. To achieve the isothermal conditions, the enzyme was introduced into Na-Hep glass capillaries of 200 µL volume (Hirschmann, Germany), which were heated until reaching the desired temperature in a water bath and rapidly cooled in ice to stop the thermal process.
Substrate specificity
To determine the substrate specificity, the enzymatic activity was assessed with various substrate solutions, with concentrations of 0 to 0.2 M. The change in absorbance was recorded at the specific wavelength of each substrate: 400 nm for catechol, 410 nm for ferulic acid, 420 nm for p-coumaric acid and caffeic acid, 430 nm for pyrogallol, and 470 nm for guaiacol. The estimation of kinetic parameters through Michaelis–Menten equation was determined using the SAS software (Statistical Analysis System, 2001).
The in silico approach was additionally employed to explore the atomic level contacts between POD and some specific substrates. The three-dimensional model of peroxidase from horseradish (1HCH.pdb from Brookhaven Protein Data Bank;[Citation9]), optimized and equilibrated at 25°C by means of Gromacs software[Citation10] as indicated by Stănciuc et al.[Citation11], was used as receptor in the docking procedure, for testing the enzyme affinity for different substrates or inhibitors. The ligand molecules considered in the study are different compounds reported in the literature as substrates (catechol, guaiacol, and pyrogallol) or of peroxidase. The PatchDock algorithm[Citation12] was used for the first rigid body docking step relying on shape complementarity criteria, whereas the FireDock algorithm[Citation13] was further used for refining the top scoring complex solutions.
Inhibitors
For the inhibitory studies on POD from plums, the following compounds were used: sodium metabisulfite, citric acid, L-cysteine, glycine, and ethylenediaminetetraacetic acid (EDTA). Each of the analyzed compounds with a potential inhibitory effect was dissolved in McIlvaine buffer at the appropriate pH value of 6.5. The experiments were performed by mixing 1 mL of substrate solution with 100 µL of inhibitor solution and 100 µL of enzyme extract. The mixture was allowed to stand for 1 min, and afterward, the absorbance was read at 470 nm, for 10 min.
Inactivation kinetics of POD
As expected for the oxidative enzymes, the inactivation of POD induced by thermal treatment was described by a first-order kinetic model. The equations used for modeling the inactivation kinetics were previously reported by Ioniţă et al.[Citation8]
Stability at high pressure
The high-pressure stability was determined as stipulated by Ioniţă et al.[Citation8] with a RESATO FPU 100–2000 food processor equipment (Resato International B.V., Netherlands). The partially purified extract was loaded into Eppendorf tubes and treated at a pressure range between 300 and 700 MPa, for 20 min, at 10°C, 30°C, and 50°C. Also, for the combined high pressure–temperature treatments, the POD activity was studied as follows: P1 – 300 MPa/5 min; P2 – 600 MPa/5 min; P3 – 300 MPa/5 min followed by 60°C/30 min; P4 – 600 MPa/5 min followed by 60°C/30 min; P5 – 60°C/30 min followed by 300 MPa/5 min; and P6 – 60°C/30 min followed by 600 MPa/5 min.
Results and discussion
Extraction and partial purification of POD from plums
Following each stage of purification, the purification fold was determined. For the collected fractions, the enzymatic activity was tested using a substrate solution containing 0.08 M guaiacol and 3% hydrogen peroxide, at 25°C and pH of 6.5.
presents the elution profile of the partially purified extract by ammonium sulfate precipitation that was applied to HIC column. The linear gradient elution with 20 mM phosphate buffer (pH 7.5) revealed the presence of two peaks. Nevertheless, the HIC active fractions presented very high values of specific activity for POD, so that the fractions were pooled together and concentrated by freeze-drying in order to be further applied on the IEC column. shows the elution profile of the active fractions (collected from HIC column) that were applied on the IEC column. Vertical gradient elution (0–100%) with 20 mM phosphate buffer and 1.0 M NaCl (pH 7.5) revealed the presence of only one well-defined peak (. Furthermore, the purification fold was determined for each step of purification.
Figure 1. Chromatographic elution profile of POD from plums: A. Separation on HiPrep Phenyl FF 16/10 (HIC); B. Separation on Mono Q 5/50 GL (IEC). The active fractions were monitored by UV absorbance at 280 nm, and the enzymatic activity was determined with a substrate solution with guaiacol and 3% hydrogen peroxide, at 470 nm.
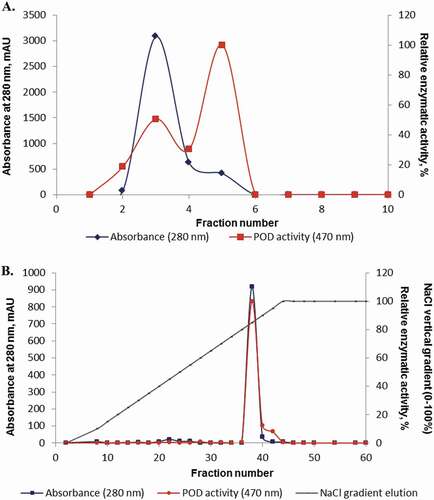
As seen in , the amount of protein is significantly different for each purification step, hence the crude extract contained a protein content of 2.031 mg/mL, while after the ammonium sulfate precipitation the obtained fraction contained 1.134 mg/mL protein. The HIC step revealed two main peaks, peak I corresponding to 9.0-fold purification with a specific activity of 801644 units/mg and a recovery rate of 11.11%, while for the second peak a 15.0-fold purification was calculated. In the last step of purification, IEC, the active fraction contained a very small amount of protein, with a specific enzyme activity of 2345865 units/mg, with a 26.33-fold purification and an enzyme recovery rate of 3.79%. Our results obtained for POD extracted from plums can easily be compared to those presented by Ajila and Rao[Citation14] who extracted and purified the POD from black gram husk and obtained, after IEC purification step, the yield of 63% with 26-fold purification. Rani and Abraham[Citation15] followed a three-step purification strategy, namely ammonium sulfate saturation and two stages of IEC. The enzyme was purified from Eupatorium odoratum as to obtain a 114-fold by IEC, with a specific activity of 7094 units/mg. Also, the purification protocol for peroxidase from date palm leaves that included concentration by ultrafiltration, anion exchange on DEAE-Sepharose, and gel filtration chromatography on Superdex 200 allowed producing a homogeneous enzyme with a 17-fold purification and a 5.8% yield.[Citation16] Our results are in contradiction with the studies of Cai et al.[Citation17] who purified the peroxidase from Jatropha curcas leaves and reported that after the final purification step, a 216-fold was achieved with a yield of 37.6%. The specific activity of the purified POD was 24800 U/mg.
Table 1. Partial purification of POD from plums and protein content determined by Bradford method.
The molecular weight and the purity of POD from plums were examined by SDS-PAGE and native-PAGE electrophoresis (). After the Silver staining protocol, the presence of two protein bands with different intensities was observed both for the HIC active fractions (lane 3) and also for the IEC fractions (lane 4). Enzymatic browning, as a process, presents a high complexity, and is determined mainly by two oxidative enzymes, PPO and POD. The presence of both enzymes was studied in several occasions by many researchers, who affirmed that the two oxidative enzymes are part of any plant that has phenolic compounds, thus making it susceptible to oxidation.[Citation18,Citation19] Despite this fact, the relationship between PPO and POD is not yet fully explained, although PPO is being considered a promoter for POD activity due to the production of hydrogen peroxide during the PPO-catalyzed oxidation of phenolic compounds.[Citation7] Nonetheless, although both enzymes were present, they displayed different catalytic behavior in regards to substrate specificity, pH, temperature and high pressure stability and, also, the enzymes displayed different concentrations. In this manner, the substrate specificity of each oxidative enzyme was assessed with different compounds, providing the possibility to identify them based on their catalytic behavior toward different specific substrates. In accordance with the specific enzymatic activity values, the results suggested the presence of both enzymes, PPO[Citation8] and POD, corresponding to the two protein bands separated by SDS-PAGE. Taken into consideration the specific enzymatic activities and also the catalytic behavior, we estimated that the second protein band corresponds to POD. Furthermore, the identification of the two enzymes was also achieved through Native-PAGE by incubating the gels with different substrate solutions, namely catechol for PPO, and guaiacol with hydrogen peroxide for POD. The presence of specific bands formed after the catalyzed reaction of each enzyme with the specific substrate, and also their concentration allowed us to draw the conclusion that POD has lower molecular weight than PPO. According to its relative rate of migration (Rf) in SDS-PAGE gel, the molecular weight of POD was approximated to 58 kDa based on the regression equation with R2 = 0.9934.
Figure 2. SDS-PAGE of POD from plums. Lane 1: Precision Plus Protein Dual Xtra molecular weight marker; Lane 2: HIC fractions; Lane 3: IEC fractions; Native PAGE of POD from plums. Lane 4: partially purified extract (30–90% ammonium sulfate).
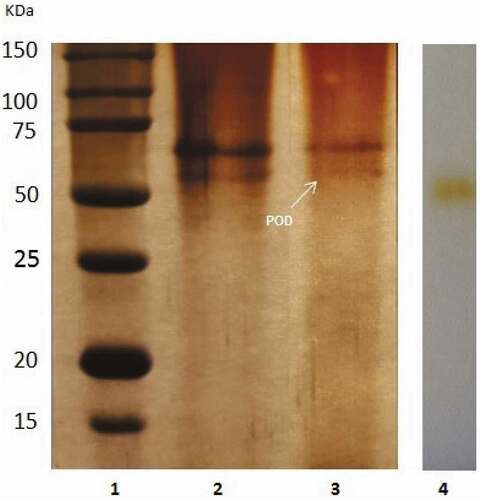
The molecular weights of PODs extracted from various sources have been reported to range from 30 to 60 kDa and the differences are attributed to several post-translational changes of the polypeptide chain including the number and the glycan chains composition.[Citation20] Our results are in accordance with those obtained by Al-Senaidy and Ismael[Citation16] who studied POD from date palm (Phoenix dactylifera L.) and assessed a molecular mass of 55 kDa. Rani and Abraham[Citation15] revealed the same molecular weight for POD from Eupatorium odoratum, 55 kDa, respectively. Also, Sonkar et al.[Citation21] identified a molecular mass of 53 kDa for POD extracted from the medicinal plant Artocarpus lakoocha.
Effect of pH and pH stability
It is widely known that the peroxidase stability assessed under extreme conditions is a conclusive factor for its industrial applications.[Citation21] The effect of pH on POD from plums was studied in the pH range of 3.0–9.0, at 25°C. Regarding the pH, the enzymatic activity of POD, the highest enzyme activity was found at pH 6.5, whereas at pH 6.0, the enzyme retained 97 ± 1.06% of the initial activity.
In the acidic pH range of 3.0 to 6.0, the enzymatic activity of POD displayed a progressive increase, while in the alkaline pH range from 7.5 to 9.0, a significant decrease of the enzymatic activity was observed. The lowest values of the relative enzymatic activity were recorded at pH values of 3.0 and 9.0, representing 37 ± 0.7% and 49 ± 0.9%, respectively, compared to values obtained at pH 6.5. Our results are in accordance with the values reported by other studies which indicate the fact that PODs present the maximum activity in the pH range of 4.5–6.5.[Citation22] Sonkar et al.[Citation21] studied the peroxidase extracted from Artocarpus lakoocha in the pH range 0.5–12.0 and registered the highest activity at pH 6.0. Ajila and Rao[Citation14] extracted black gram (Vigna mungo) husk peroxidase and identified the optimum pH of the enzyme as being 5.5. Al-Senaidy and Ishmael[Citation16] studied POD extracted from the leaves of palm (Phoenix dactylifera L.) in the range of pH from 4.0 to 9.0, the enzyme showing high activity in the pH range from 5.0 to 8.0 and maximum activity at pH 5.5. Fortea et al.[Citation23] reported the optimum pH of 4.5 for the activity of POD extracted from table grapes (Crimson Seedless), by using ABTS as substrate. Several other studies revealed rather similar values for the optimum pH, respectively, pH 8.0 for POD extracted from papaya[Citation4], pH 6.0 for POD from kiwi[Citation24], pH 5.2 for POD from spinach.[Citation25] The pH stability of POD from plums was evaluated during a period of 3 days by incubating the enzyme at the appropriate pH values ranging from 3.0 to 9.0. In the acidic pH range, after 48 h, at the values of 3.0, 4.0, and 5.0, the enzyme retained about 29 ± 0.4%, 20 ± 2.7%, and 30 ± 0.8% of the initial activity, respectively. Also, after 48 h, at pH values of 6.0, 6.5, and 7.0 POD maintained approximately 27 ± 1.8%, 31 ± 0.9%, and 58 ± 6.3% of initial activity. In the alkaline range, at the pH value 8.0 and 9.0, the enzyme maintained 28 ± 0.6%, and 26 ± 3.4% of the initial enzyme activity, respectively, after 48 h. The results are in good agreement with those reported by Fortea et al.[Citation23] who studied the pH stability of POD extracted from table grapes (Crimson Seedless) by using ABTS substrate. It was observed that the enzymatic activity developed an inversely proportional effect in correlation to the pH of the reaction medium. Pandey and Dwivedi[Citation4] determined the pH stability in a wide pH range for the POD extracted from papaya, which exhibited a high stability in the pH range 7.0–9.0, while at lower pH values the enzyme activity gradually decreased. Thus, depending on the source from which the enzyme is extracted, the pH stability varies within a wide range of values. Al-Senaidy and Ismail[Citation16] found that the POD extracted from the persimmon leaves presented a high stability in the pH range of 4.0–8.0. POD lost about 80% of enzymatic activity at a pH smaller than 3.0, and greater than 9.0. Sonkar et al.[Citation21] studied the POD from the medicinal plant Artocarpus lakoocha, the enzyme being stable under the tested conditions. In this context, the enzyme displayed more than 60% stability in the pH range of 5.5–12.0. Regarding the enzymatic activity, in the pH range of 5.0–8.5, POD retained more than 50% of its activity. pH is a very important parameter that affects the ionization of the exposed amino acids of an enzyme in such a manner that the low stability at very acidic pH values can be attributed to the instability of the binding site of the enzyme.[Citation7]
Optimum temperature and temperature stability
To determine the optimum temperature of POD from plums, the activity was assessed at different temperatures ranging from 4°C to 60°C. POD presented the highest activity at the temperature of 25°C. As the temperature increased, a gradual decrease of the relative enzyme activity was observed, as such at 40°C 88.7 ± 4.9% of activity is maintained, at 50°C 82.7 ± 2.9%, while at 60°C the enzyme maintained 79.7 ± 0.5% of its activity. In the range 40–60°C, the process of thermal inactivation begins although the enzymatic activity does not decrease significantly. The results obtained for POD from plums are in accordance with the study of Yadav et al.[Citation26] who investigated the POD from banana (Musa paradisiaca) in the 15–35°C temperature range, and reported the optimum temperature of 25°C. In contrast, Rani and Abraham[Citation15] determined the optimum temperature of 55°C for the activity of POD from Eupatorium odoratum, whereas Pandey and Dwivedi[Citation4] studied POD from papaya and assessed an optimum temperature of 40°C.
The temperature stability of POD from plums was studied in the temperature domain between 25°C and 85°C for different periods of time 0, 10, and 20 min. POD from plums displayed the maximum enzymatic activity at the optimum temperature value of 25°C. Above this value, POD activity started to decrease in a continuous rate so that at 70°C by 64 ± 0.7%, while the highest decrease rate was observed at 85°C, when the activity decreased by 75 ± 1.3% after 20 min of treatment. In the temperature range of 25–65°C, POD from plums displayed a high stability, whereas between 70°C and 85°C, POD activity began to decrease sharply. Although, even at the highest temperature the complete inactivation was not achieved suggesting that the enzyme needs higher temperature values or longer periods of treatment. Terefe et al.[Citation18] determined the temperature stability of POD extracted from strawberry puree in the temperature range 25–100°C. POD extracted from Festival strawberry variety presented higher thermal stability compared to the enzyme extracted from Flavor strawberries variety under similar treatment conditions. Al-Senaidy and Ismael[Citation16] determined the thermal stability of POD extracted from persimmon by incubating the enzyme at different temperatures for 60 min. Thus, in the temperature range 60–70°C, the enzyme maintained its enzyme activity almost completely, throughout the treatment, indicating that POD extracted from persimmon is a stable enzyme at high temperatures. Chen, Chung, and Chang[Citation5] determined the temperature stability of POD extracted from papaya by incubating the enzyme at temperatures varying between 30°C and 80°C for 3 to 40 min. The relative enzyme activity was comparable to the activity values under optimal conditions. The enzyme showed high stability up to 50°C. At the temperature of 60°C, POD retained more than 85% of activity after 40 min, while at 70°C, after 20 min, the enzyme was completely inactivated.
Substrate specificity
The substrate specificity of POD extracted from plums was determined in the presence of hydrogen peroxide for several compounds such as pyrogallol, guaiacol, catechol, ferulic acid, p-coumaric acid, and caffeic acid, with concentrations ranging from 0 to 0.2 M. SAS software was used to calculate the values of kinetic parameters Km and Vmax.
POD displayed the highest activity for guaiacol (Vmax 1749 ± 58.8 OD/min and Km 113.2 ± 69.8 mM) followed by catechol (Vmax 168.4 ± 18.1 OD/min and Km 23.4 ± 12 mM), and pyrogallol (Vmax 164 ± 31 OD/min and Km 20.8 ± 6 mM). The enzyme showed no catalytic activity toward all other tested substrates, namely ferulic acid, p-coumaric acid, and caffeic acid. Regarding the substrate affinity, the Km values suggest that the enzyme presented the highest affinity toward hydroxybenzoic acids, and no activity toward hydroxycinnamic acids. Nevertheless, guaiacol was considered the most suitable substrate for POD extracted from plums because the compound presented the highest Vmax value. These results are in line with those reported by Cai et al.[Citation17] who studied POD from Jatropha curcas leaves. They investigated the six substrates, and reported that POD had the highest affinity for guaiacol, ABTS, and o-phenylenediamine with Km values of 0.17 mM, 0.19 mM and 0.67 mM, respectively.
Nonetheless, in contrast with our results, Chen, Chung, and Chang[Citation5] showed that POD extracted from papaya shows the highest affinity for pyrogallol, catechol, and ABTS. Pandey and Dwivedi[Citation4] studied the substrate specificity of POD extracted from papaya, toward pyrogallol, o-dianisidine, and guaiacol, to a predetermined concentration of hydrogen peroxide. The Km values suggested that POD extracted from papaya exhibited the highest affinity for o-dianisidine, followed by pyrogallol and guaiacol.
Further insights into the interaction between peroxidase and several specific substrates, namely guaiacol, catechol, and pyrogallol were provided after performing docking simulations. For each investigated compound only the docking model characterized by the highest interaction surface and the lowest interaction energy was considered for checking the atomic level particularities (). Detailed analysis of the best fits indicated that peroxidase has different binding sites for the three investigated compounds. Catechol and guaiacol share 10 amino acids (Pro4, Val14, Ser15, Ile103, Gln106, Gln107, Trp117, Arg118, Val119, and Leu121), whereas the binding site for pyrogallol appears to be located in a different side of the enzyme (). Among the amino acids interfacing the pyrogallol molecule, Arg38 residue was suggested to play an important role in the horseradish peroxidase-catalyzed conversion of the dioxygen species. When investigating the catalytic pathway of horseradish peroxidase, Berglund et al.[Citation9] showed the existence of one hydrogen bond linking the ferryl oxygen to the Nε atom of Arg38 residue, in the high-resolution crystal structure of the enzyme.
Table 2. Single molecule level details on the interaction between POD and different specific substrate molecules.
Among the enzyme-substrate complexes investigated through in silico approach, the highest interaction surface was observed in case of guaiacol (), which is in line with the experimental observations. Moreover, as indicated by the negative value of the solvation free energy gain upon complex formation, the POD has a positive affinity for guaiacol molecule and the interfaces within the assembly are hydrophobic. On the other hand, surfaces involved in the interaction between POD and catechol or pyrogallol molecules are connected through hydrogen bonds (), which might compensate for the reduced contribution of hydrophobic interactions within the two molecules of the complexes.
Inhibitors
From an industrial point of view, the possibility to identify the most potent inhibitory compound that can prevent enzymatic browning is very important in improving the sensorial properties not only for fresh fruit and vegetables, but also for their derived products. Based on the studies available in the literature, the process of enzymatic browning catalyzed by POD may be prevented by inhibition of the enzyme. In this context, several chemical compounds were tested as antibrowning agents or otherwise known as inhibitors. In the present study, the potential inhibitory effect of five compounds at concentrations of 0.5, 5, and 50 mM was tested, respectively, sodium metabisulfite, citric acid, L-cysteine, glycine, and EDTA. From , it can be seen that the most effective inhibitors were L-cysteine, with a 42.4% inhibition degree at the concentration of 50 mM, and sodium metabisulfite, with 42% inhibition degree at 50 mM. The lowest inhibitory effect was observed for citric acid, with a degree of inhibition of 22.8%.
Table 3. Effects of various inhibitors on the activity of POD from plums.
Thermal inactivation
The thermal inactivation of POD was described by a first-order model in the studied temperature range (). The process of inactivation started at 60°C, and after 90 min of thermal treatment, the enzyme maintained only 50 ± 5.8% of the initial activity. At the temperature of 75°C, after 30 min of heat treatment, POD retained 26.9 ± 1.9% of the initial enzyme activity, whereas at 85°C, after 20 min of treatment, the enzyme retained only 24.8 ± 1.3% of its initial activity.
Figure 3. The temperature effect on POD from plums: A. Thermal inactivation at different temperature values: 60°C (





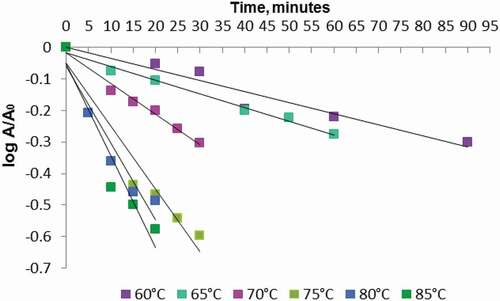
The kinetic parameters values, D, and inactivation rate constant, k, were determined using the several equations reported in our previous study.[Citation8] It can be observed that the increase of temperature determined the decrease of D values (). In order to describe the temperature dependence of the rate constant, a linear correlation was established, with an R2 = 0.921. The value of activation energy, Ea, was 84.79 ± 2.2 kJ/mol, with a z value of 27.02 ± 0.76°C.
Table 4. Estimated kinetic parameters of POD from plums inactivation.
The results for POD extracted from plums are consistent with those obtained by Gonçalves et al.[Citation27] who studied the inactivation of peroxidase from the pumpkin that followed a first-order Arrhenius model in the temperature range of 75–95°C, with an activation energy of 86.20 ± 5.57 kJ/mol. Chen, Chung, and Chang[Citation5] conducted experiments in order to achieve the thermal inactivation of POD extracted from papaya. The inactivation process was described by a first-order kinetic model at three temperatures 60°C, 70°C and 80°C. The inactivation constants were calculated to be 9.73x10−3, 6.8x10−2, and 1.3 × 10−1 min−1.
In contrast with our study, Chakraborty, Rao, and Mishra[Citation3] investigated the heat inactivation of POD from pineapple at temperatures between 30°C and 95°C and reported that the inactivation of the enzyme is described by a two-phase kinetic model in the temperature range 45–75°C and a first-order kinetic model in the temperature range 85–95°C, whereas the D parameter values ranged between 103 and 517 min. Suha, Babiker and Babiker[Citation28] studied the POD extracted from potatoes, carrots, eggplants, and tomatoes and concluded that the rate of inactivation of the enzyme increased with the increasing temperature and time of treatment. POD inactivation extracted from eggplant was performed at 80°C for 4 to10 minutes at 90ºC, whereas the complete inactivation of POD from tomato was achieved at 90ºC, after a period of treatment ranging from 6 to 10 min.
Enzyme stability at high-pressure processing
To determine the enzyme-substrate interactions, it is necessary to know the exact pressure-temperature range during processing, at which the enzyme and substrate are stable so that the cell membrane would break and lead to the release of enzymes and substrates in order to achieve the highest rate of inactivation.[Citation7] In this context, the POD from plums exhibited high stability in the selected temperature and pressure ranges. presents the residual activity of POD from plums after different types of treatment.
Figure 4. High pressures stability (the enzyme activity was determined under optimum conditions of pH 6.5 and 25°C using guaiacol and 3% hydrogen peroxide as substrate).
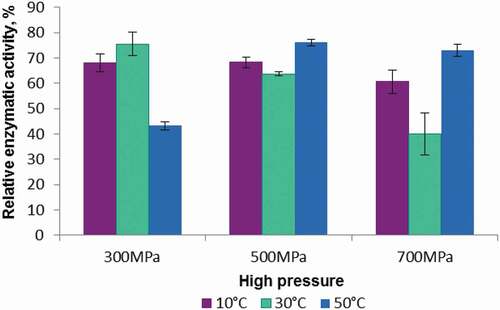
The most effective type of treatment was at 30°C and pressure of 700 MPa, after 20 min the enzyme maintaining only 40 ± 8.26% of the initial activity, whereas the least effective treatment was at 500 MPa and 50°C, the enzyme presenting after 20 min of treatment an activity of 76 ± 1.33% when compared to its initial activity. POD extracted from plums exhibited rather different stability regarding the treatment in a wide range of values. The P1 and P2 combined treatments produced a loss of 31 ± 3.8% and 21 ± 3.1% for POD from plums compared to its initial activity. The combined treatments at P3 and P4 reduced the enzyme activity by 29 ± 1.2% and 20 ± 7.3%, respectively, whereas the P5 and P6 treatments reduced the activity by 18 ± 1.9% and 28 ± 2.7%, respectively. As a general remark, when the heat treatment was performed first and afterward the high-pressure treatment was applied, the enzyme presented a higher degree of inactivation. The results for POD extracted from Prunus domestica, are different from the results reported by Fang, Jiang and Zhang[Citation24] for POD extracted from kiwi. Regarding the relative activity of POD from kiwi, the combined high pressure-temperature treatment produced a slight activation at 200 MPa at a temperature of 10°C for 10–20 minutes, while in the pressure range from 400 to 600 MPa, the enzymatic activity decreased in comparison to the enzymatic activity obtained at 200 MPa. Terefe et al.[Citation18] studied the effect of combined high pressure-temperature treatment on POD extracted from strawberries puree. The inactivation of the enzyme was substantial, especially at the highest pressure (690 MPa) and the highest temperature values. Nonetheless, the complete inactivation of POD was observed after the treatment at 90°C for 5 min, regardless of pressure. Similarly, the pressure of 690 MPa led to the enzyme inactivation degree of 72%, and the inactivation effect increased with increasing temperature. In general, the prolonged treatment resulted in an increased inactivation of POD. Chakraborty, Rao, and Mishra[Citation3] studied the inactivation of POD from pineapple by combined treatment temperature and high pressure. The treatment regarded temperatures in the range 30°C-70°C, while the applied values of pressure (in MPa) were between 200 and 600 MPa. POD extracted from pineapple exhibited a relative activity of 70% and 53%, after the pressure treatment at 200 MPa and 600 MPa, at a temperature of 30°C, for 20 min. As a result of combined high pressure-temperature treatment at 200 MPa/70°C for 20 min and 600 MPa/70°C for 20 min, the enzyme displayed high inactivation degree of 63% and 96%, respectively.
Conclusions
In terms of applied research, the results are very important because they offer valuable information regarding the behavior of POD from plums under different thermal or high-pressure processing conditions, with a great impact on the food industry. The enzyme was partially purified by a three-step procedure, mainly, ammonium sulfate precipitation, HIC, and IEC. After the final purification step, the 26.33-fold purification was obtained, with a recovery of 3.79%. The presence of POD was checked by native-PAGE, and the molecular weight was approximated to 58 kDa by SDS-PAGE. POD from plums displayed the highest enzymatic activity towards hydroxybenzoic acids, whereas the optimum conditions for the enzyme activity were pH 6.5 and 25°C. Regarding its stability, the enzyme presented the highest stability in the pH range 5.0–7.0 and in the temperature domain 25–70°C. Furthermore, at the high-pressure treatment of 700 MPa, the enzyme presented also a high stability. The inhibition of the enzyme was achieved in the presence of ascorbic acid and L-cysteine. The thermal stability of POD from plums may cause a significant loss of activity, at temperatures higher than 85°C. When combined, the high pressure–temperature treatment proved to be more useful in limiting the enzyme activity, thus highlighting the fact that a series of complex treatments must be applied to successfully inactivate this enzyme.
Acknowledgments
The authors would also like to thank the Bioaliment Research Platform (www.bioaliment.ugal.ro) for technical support.
Additional information
Funding
References
- Sojka, M.; Kołodziejczyk, K.; Milala, J.; Abadias, M.; Viñas, I.; Guyot, S.; Baron, A. Composition and Properties of the Polyphenolic Extracts Obtained from Industrial Plum Pomaces. J. Funct. Foods. 2015, 12, 168–178. DOI: 10.1016/j.jff.2014.11.015.
- Tomas-Barberan, F.; Espin, J. C. Phenolic Compounds and Related Enzymes as Determinants of Quality in Fruits and Vegetables. J. Sci. Food Agric. 2001, 81, 853–876. DOI: 10.1002/jsfa.885.
- Chakraborty, S.; Rao, P.; Mishra, H.; Kinetic Modeling of Polyphenoloxidase and Peroxidase Inactivation in Pineapple (Ananas Comosus L.) Puree during High-Pressure and Thermal Treatments. Innovative Food Sci. Emerg. Technol. 2015, 27, 57–68. DOI:10.1016/j.ifset.2014.11.003
- Pandey, V.; Dwivedi, U. A Ripening Associated Peroxidase from Papaya Having A Role in Defense and Lignification: Heterologous Expression and In-Silico and In-Vitro Experimental Validation. Gene. 2015, 555, 438–447. DOI: 10.1016/j.gene.2014.11.013.
- Chen, L.-C.; Chung, Y.-C.; Chang, C.-T. Characterisation of an Acidic Peroxidase from Papaya (Carica Papaya L. Cv Tainung No. 2) Latex and Its Application in the Determination of Micromolar Hydrogen Peroxide in Milk. Food Chem. 2012, 135, 2529–2535. DOI: 10.1016/j.foodchem.2012.06.106.
- Jang, J.-H.; Moon, K.-D. Inhibition of Polyphenol Oxidase and Peroxidase Activities on Fresh-Cut Apple by Simultaneous Treatment of Ultrasound and Ascorbic Acid. Food Chem. 2011, 124, 444–449. DOI: 10.1016/j.foodchem.2010.06.052.
- Terefe, N.; Buckow, R.; Versteeg, C. Quality-Related Enzymes in Fruit and Vegetable Products: Effects of Novel Food Processing Technologies, Part 1: High-Pressure Processing. Crit. Rev. Food Sci. Nutr. 2014, 54(1), 24–63. DOI: 10.1080/10408398.2011.566946.
- Ioniţă, E.; Gurgu, L.; Aprodu, I.; Stănciuc, N.; Dalmadi, I.; Bahrim, G.; Râpeanu, G. Characterization, Purification, and Temperature/Pressure Stability of Polyphenol Oxidase Extracted from Plums (Prunus Domestica). Process Biochem. 2017, 56, 177–185. DOI: 10.1016/j.procbio.2017.02.014.
- Berglund, G. I.; Carlsson, G. H.; Smith, A. T.; Szoke, H.; Henriksen, A.; Hajdu, J. The Catalytic Pathway of Horseradish Peroxidase at High Resolution. Nature. 2002, 417, 463–468. DOI: 10.1038/417463a.
- van der Spoel, D.; Lindahl, E.; Hess, B.; Groenhof, G.; Mark, A. E.; Berendsen, H. J. GROMACS: Fast, flexible, and Free. J. Comput. Chem. 2005, 26, 1701–1718. DOI: 10.1002/jcc.20291.
- Stănciuc, N.; Aprodu, I.; Ioniță, E.; Bahrim, G.; Râpeanu, G.; Exploring the Process–Structure–Function Relationship of Horseradish Peroxidase through Investigation of pH-and Heat Induced Conformational Changes. Mol. Biomol. Spectrosc. 2015, 147, 43–50. DOI:10.1016/j.saa.2015.03.023
- Schneidman-Duhovny, D.; Inbar, Y.; Nussinov, R.; Wolfson, H. J. PatchDock and SymmDock: Servers for Rigid and Symmetric Docking. Nucleic Acids Res. 2005, 33, 363–367. DOI: 10.1093/nar/gki481.
- Andrusier, N.; Nussinov, R.; Wolfson, H. J. FireDock: Fast Interaction Refinement in Molecular Docking. Proteins. 2007, 69, 139–159. DOI: 10.1002/prot.21495.
- Ajila, C. M.; Rao, P. U. J. S. Purification and Characterization of Black Gram (Vigna Mungo) Husk Peroxidase. J. Mol. Catal. B: Enzym. 2009, 60, 36–44. DOI: 10.1016/j.molcatb.2009.03.014.
- Rani, D. N.; Abraham, T. E. Kinetic Study of a Purified Anionic Peroxidase Isolated from Eupatorium Odoratum and Its Novel Application as Time Temperature Indicator for Food Materials. J. Food Eng. 2006, 77, 594–600. DOI: 10.1016/j.jfoodeng.2005.07.018.
- Al-Senaidy, A.; Ismael, M.; Purification and Characterization of Membrane-Bound Peroxidase from Date Palm Leaves (Phoenix Dactylifera L.). Saudi J. Biol. Sci. 2011, 18, 293–298. DOI:10.1016/j.sjbs.2011.04.005
- Cai, F.; OuYang, C.; Duan, P.; Gao, S.; Xu, Y.; Chen, F. Purification and Characterization of a Novel Thermal Stable Peroxidase from Jatropha Curcas Leaves. J. Mol. Catal. B: Enzym. 2012, 77, 59–66. DOI: 10.1016/j.molcatb.2011.12.002.
- Terefe, N.; Yang, Y.; Knoerzer, K.; Buckow, R.; Versteeg, C.; High Pressure and Thermal Inactivation Kinetics of Polyphenol Oxidase and Peroxidase in Strawberry Puree. Innovative Food Sci. Emerg. Technol. 2010, 11, 52–60. DOI:10.1016/j.ifset.2009.08.009
- Gong, Z.; Li, D.; Liu, C.; Cheng, A.; Wang, W. Partial Purification and Characterization of Polyphenol Oxidase and Peroxidase from Chestnut Kernel. LWT - Food Sci. Technol. 2015, 60, 1095–1099. DOI: 10.1016/j.lwt.2014.10.012.
- Thongsook, T.; Barrett, D. M. Heat Inactivation and Reactivation of Broccoli Peroxidase. J. Agric. Food Chem. 2005, 53, 3215–3222. DOI: 10.1021/jf048162s.
- Sonkar, S. K.; Pachauri, M.; Kumar, A.; Shukla, A.; Patel, M.; Jagannadham, M. V.; Heme-Peroxidase from Medicinal Plant Artocarpus Lakoocha: Purification, Characterization and Wound Healing Studies. Biocatal. Agric. Biotechnol. 2015, 4, 180–190. DOI:10.1016/j.bcab.2015.03.002
- Sakharov, I.; Vesgac Blanco, M.; Galaev, I.; Sakharova, I.; Pletjushkina, O.; Peroxidase from Leaves of Royal Palm Tree Roystonea Regia: Purification and Some Properties. Plant Sci. 2001, 161, 853–860. DOI:10.1016/S0168-9452(01)00466-6
- Fortea, M.; López-Miranda, S.; Serrano-Martínez, A.; Carreńo, J.; Núńez-Delicado, E. Kinetic Characterization and Thermal Inactivation Study of Polyphenol Oxidase and Peroxidase from Table Grape (Crimson Seedless). Food Chem. 2009, 113, 1008–1014. DOI: 10.1016/j.foodchem.2008.08.053.
- Fang, L.; Jiang, B.; Zhang, T. Effect of Combined High Pressure and Thermal Treatment on Kiwifruit Peroxidase. Food Chem. 2008, 109, 802–807. DOI: 10.1016/j.foodchem.2008.01.017.
- Köksal, E.;. Peroxidase from Leaves of Spinach (Spinacia Oleracea): Partial Purification and Some Biochemical Properties. Int. J. Pharmaceutics. 2011, 7(1), 135–139.
- Yadav, P.; Singh, V. K.; Yadav, M.; Singh, S. K.; Yadava, S.; Yadav, K. D. S. Purification and Characterization of Mn-Peroxidase from Musa Paradisiaca (Banana) Stem Juice. Indian J. Biochem. Biophys. 2012, 49, 42–48.
- Gonçalves, E. M.; Pinheiro, J.; Abreu, M.; Brandão, T. R. S.; Silva, C. L. M.; Modelling the Kinetics of Peroxidase Inactivation, Colour and Texture Changes of Pumpkin (Cucurbita Maxima L.) During Blanching. J. Food Eng. 2007, 81, 693–701. DOI:10.1016/j.jfoodeng.2007.01.011
- Suha, O. A.; Babiker, E. M.; Babiker, E. E. Thermostability at Different pH Levels of Peroxidase Extracted from Four Vegetables. Int. Food Res. J. 2013, 20(2), 715–719.