ABSTRACT
Tartary buckwheat has become popular healthful food due to its antidiabetic and antioxidant activities. In the current study, the in-vitro α-glucosidase inhibitory and antioxidative activities of different parts of Tartary buckwheat were evaluated throughout the growth stages. In addition, the phytochemical profiles and quantitative analysis were further achieved by HPLC-DAD. Tartary buckwheat root extracts at a concentration of 0.5 mg/mL showed significant inhibition more than 80% in the flowering and yield stage, while acarbose was 87.4% inhibition at 5 mg/mL. It also showed good antioxidant capacity using 2,2-diphenyl-1-picryl-hydrazyl, 2, 2ʹ-azino-bis-(3-ethylbenzothiazoline-6-sulfonic acid) diammonium salt, and ferric reducing antioxidant power determination. The antioxidant capacities of roots ranged from 128 to 158 μM VC, from 226 to 409 μM VC, and from 77 to 205 μM butylated hydroxytoluene (BHT) (equaling VC or BHT per 1 g of dry weight), respectively. Chemical composition analysis showed that the leaf contained more rutin and the root more phenylpropanoid. The results may provide a better understanding of the variation of Tartary buckwheat as a functional food for preventing diabetes and antioxidant.
Introduction
Tartary buckwheat (Fagopyrum tataricum Gaertn) is an important alternative cereal crop that belongs to the Polygonaceae family. It is considered to be a nutritional food due to its benefits for human health. Tartary buckwheat may be a promising food resource for the antioxidant and antidiabetic agents.
The pharmacological studies demonstrated that the hulls, brans, flours, groats, and seeds of Tartary buckwheat had various pharmacological activities such as antioxidant activity, which was determined by 2,2-diphenyl-1-picryl-hydrazyl (DPPH), 2, 2ʹ-azino-bis-(3-ethylbenzothiazoline-6-sulfonic acid) diammonium salt (ABTS) free radical scavenging ability,[Citation1–Citation6] ferric reducing antioxidant power (FRAP),[Citation5–Citation7] angiotensin-I converting enzyme inhibitory activity,[Citation7] antitumor activity of Tartary buckwheat protein against human mammary cancer cell Bcap37,[Citation8] Tartary buckwheat bran extracts against antiproliferative of human liver cancer cells (HepG2) in vitro,[Citation9] and antidiabetic activity of Tartary buckwheat bran extract being investigated through male KK-Ay mice (type 2 diabetic) and C57BL/6 mice (the control).[Citation10] The studies reported that three flavonoids, such as quercetin, isoquercetin, and rutin from Tartary buckwheat bran were effective inhibitors against α-glucosidase.[Citation11,Citation12] In addition, Tartary buckwheat could reduce the serum level of total cholesterol and triglyceride, lower the concentration of blood glucose and regulate the lipid profile.[Citation2,Citation13]
Previous phytochemical studies on Tartary buckwheat had identified some types of compounds such as quinic acid derivatives, flavan-3-ol derivatives, flavonol derivatives, phenylpropanoid glycosides, nitrogen compounds,[Citation14] and other compounds such as D-chiro-inositol, anthraquinones, fagopyrin, 2ʹʹ-hydroxynicotianamine, and nicotianamine.[Citation15]
Diabetes has affected millions of people in China. The inhibition of carbohydrate hydrolyzing enzymes is an effective approach to control postprandial hyperglycemia for the prevention and treatment of diabetes. α-Glucosidase is one of the most important therapeutic targets for type 2 diabetes (T2D). Inhibition of α-glucosidase can decrease postprandial blood glucose level through delaying the digestion of carbohydrates to prevent and manage T2D.[Citation16] Furthermore, chronic oxidative stress by free radicals can lead to diabetic complications, and thus antioxidants also have an important role for the prevention and treatment of T2D.[Citation17,Citation18]
Numerous studies mainly focused on the germination of Tartary buckwheat sprout and accumulation of bioactive components,[Citation19–Citation24] antioxidant,[Citation12,Citation21,Citation23] and α-glucosidase inhibitory activities.[Citation12] However, there are few reports about the antioxidant activity, α-glucosidase inhibitory activity, phytochemical profiles, and quantitative analysis in the whole growth stages and various parts (i.e. root, stem, and leaf) of tartary buckwheat. We propose the following hypothesis: specific growth stage and part of Tartary buckwheat might be a more effective antidiabetic and antioxidant activity.
Therefore, the objective of the current study is to extensively evaluate the dynamic changes of α-glucosidase inhibitory, antioxidative activity, and the chemical constituents during the germination, growth, flowering, and yield stages of tartary buckwheat.
Materials and methods
Chemicals and plant materials
α-Glucosidase (EC 3.2.1.20) from Saccharomyces cerevisiae, type I, lyophilized powder, ABTS, and DPPH reagent were purchased from Sigma-Aldrich (St. Louis, MO, USA). p-Nitrophenyl-α-D-glucopyranoside (PNPG), sodium phosphate (dihydrate), disodium phosphate, 2,4,6-tris (2-pyridyl)-s-triazine, potassium persulfate (K2O8S2), butylated hydroxytoluene (BHT), L-ascorbic acid (VC), and acarbose were purchased from J&K Chemical Co. Ltd (Beijing, China). Acetonitrile of HPLC grade was purchased from Mallinckrodt Baker, Inc. (Phillipsburg, NJ, USA). HPLC-grade acetic acid (AS1102–002) was purchased from TEDIA, Inc. (Fairfield, USA). Deionized water was purified by a Millipore water purification system (Millipore, MA, USA).
Tartary buckwheat (F. tataricum Gaertn) was cultivated under agricultural field plantation, geographically located on 35°45ʹ46.9” N and 119°53ʹ95.8” E and authenticated by Professor Jian-an Wang. The Tartary buckwheat samples were collected every 10 days from July to October, and then classified as root, stem, and leaf in Table S1. At the stages of 19 Jul and 29 Jul, the Tartary buckwheat sprouts were not completely differentiated, so the samples during the two stages were studied as a whole plant. The germination, growth, flowering, and yield of Tartary buckwheat are shown in . The voucher specimens (No. 20151018) were deposited at the Department of Pharmacy, Jining Medical University, China.
Sample preparation
The dry Tartary buckwheat was pulverized into powder and sieved through a 65-mesh size (about 250 ± 9.9 μm) before use. An aliquot (0.2 g) of powder was weighed into a glass scintillation vial with 4 mL of 70% aqueous ethanol, which was soaked for 60 min at room temperature. Then, it was sonicated (200 W and 40 KHz) for 30 min. After centrifugation at 10278 × g for 10 min, the supernatant was lyophilized. The extract was used for analysis of its antioxidant and enzyme inhibition activities.
DPPH radical scavenging activity
DPPH scavenging activity was determined according to the method reported by Brand-Williams et al. with some modifications.[Citation25] The different parts of Tartary buckwheat throughout the growth stages were evaluated using a DPPH radical scavenging assay. The extract solution was diluted five times (0.2–1 mL) and mixed with 2 mL of methanol solution containing DPPH radicals (0.1 mM). The reaction mixtures were incubated in darkness at room temperature for 60 min. The absorbance was measured at 517 nm. All tests were carried out in triplicate and the results were mean values ± standard deviation (SD). VC was used as a reference standard. The standard curves were prepared using VC ranging from 283.9 to 851.7 μM r2 = 0.970. The antioxidant activities were expressed as μM VC equivalents per 1 g of dry weight (DW).
ABTS radical cation scavenging activity
The ABTS+ assay was based on the procedure previously described by Re et al.[Citation26] In short, 10 mg of ABTS was diluted in 2.6 mL of potassium persulfate solution (2.45 mM) for the development of ABTS+ radicals (7 mM). This solution was kept in the dark at ambient temperature for 12–16 h. The ABTS+ solution was diluted to an absorbance of 0.70 ± 0.02 in methanol (about 1:89). Ten microliter of diluted extract (0.2–1 mL) was added with 2 mL ABTS+ solution and kept in the dark at room temperature for 60 min. The absorbance was measured at 734 nm. All determinations were performed in triplicate and the results were mean values ± SD. The standard curves were prepared using VC ranging from 5.68 to 340.7 μM r2 = 0.998. The results were expressed as equivalent antioxidant values (μM VC equivalents/g DW).
Ferric reducing antioxidant power (FRAP) assay
The modified FRAP method was used for antioxidant determination of Tartary buckwheat extracts. Briefly, fresh FRAP reagent was prepared daily by mixing 2.5 mL TPTZ solution (10 mM TPTZ in 40 mM HCl, 2.5 mL of FeCl3 · 6H2O solution (20 mM), and 25 mL acetate buffer (300 mM, pH 3.6). The reagent was warmed to 37℃ in a water bath, then 2 mL FRAP reagent was placed in a cuvette and the initial absorbance was measured. Hundred microliter of sample was added to the cuvette and the absorbance was measured after 60 min at 593 nm in the dark. All the tests were measured in triplicate and the results expressed as means ± SD. BHT was used as a standard. The standard curves were prepared using BHT ranging from 226.9 to 2269.1 μM r2 = 0.991. The extracts activities were expressed in μM BHT equivalents per 1 g of DW.
Determination of α-glucosidase inhibition
The α-glucosidase inhibitory activity of Tartary buckwheat extract samples was measured as described with slight modifications.[Citation12,Citation27,Citation28] The different parts of Tartary buckwheat sample solution (10 μL, from Section 2.2.) throughout the growth stages were lyophilized and dissolved in 1 mL of 100 mM phosphate buffer (pH 6.8) to concentrations of 0.5 mg/mL (dry original raw material). Briefly, 50 μL of diluted sample solution and 50 μL of 100 mM phosphate buffer (pH 6.8) containing α-glucosidase solution (0.1 U/mL) were incubated in 96-well plates. After incubation for 10 min at 37°C, 50 μL of 2.5 mM PNPG substrate solution was added and incubated at 37°C for 30 min. The reaction was terminated by the addition of 100 μL of 0.2 M Na2CO3. Then, 250 μL of the reaction mixture was taken and added into a 96-well plate. The absorbance was measured at 405 nm using a micro-plate reader (Bio-Rad iMark, USA) and compared to a control which had 50 μL of buffer solution instead of the extract sample. The α-glucosidase inhibitory of acarbose was evaluated and compared as a positive control. All determinations were carried out in triplicates. The percentage inhibition was calculated by the following formula: α-glucosidase inhibitory (%) = [Acontrol − (Atest − Abackground)]/Acontrol × 100. Where Acontrol, Atest, Abackground are defined as the absorbance of 100% enzyme activity (only the solvent with the enzyme), test sample with the enzyme, and test sample without the enzyme, respectively.
Phytochemical profiles and quantification by HPLC−DAD
The experiments were performed using an Agilent 1260 infinity liquid chromatography system (Agilent Technologies, Waldbroon, Germany). The instrument was configured with binary pumps, a programmable auto-sampler, a compartment column oven, a multi-diode array UV-vis detector, and a degasser. Agilent chemstation software was used to control the HPLC system and process the data. The chromatographic separation was performed on Kinetex C18 column (100 × 4.6 mm, 2.6 μm, Phenomenex, S/N: 6840360-30). The column temperature was maintained at 35°C, the injection volume was 2 μL, and UV absorbance was detected at a wavelength of 320 nm. The mobile phase consisted of 0.1% aqueous acetic acid (v/v) (A) and acetonitrile (B). The gradient program was applied as follows: 0–20 min for 6–16% B; 20–38 min for 16–20% B; 38–48 min for 20–33% B; 48–68 min for 33–38% B; 68–70 min 38–100% B; 70–75 min 100%, followed by re-equilibration of the column for 8 min. Elution was set to a flow rate of 0.8 mL/min.
Statistical analysis
Statistical analysis was performed using GraphPad Prism 6.07 (GraphPad Software, CA, USA). Statistical significances were calculated by One-way ANOVA and the Tukey’s Multiple Comparison Test was used in order to obtain the p values. Differences among the means were considered significant at p < 0.05.
Results and discussion
Antioxidant capacities of Tartary buckwheat
Due to the chemical diversity and structural complexity of antioxidant compounds in plant extracts, it is difficult to precisely determine the antioxidant activity for all compounds present in a sample using a single assay. Hence, the use of multiple assays is more suitable. Therefore, the DPPH, ABTS, and FRAP assays were used to evaluate the antioxidant capacities of the different growth stages and parts of Tartary buckwheat. VC and BHT were used as reference compounds. The antioxidant activities were expressed as equivalent antioxidant values (μM of VC or BHT equivalents/g DW), which were calculated using standard curves.
The DPPH radical scavenging ability was determined in order to evaluate the antioxidant activity of the different parts of Tartary buckwheat during the growth stages. As shown in , antioxidant activities were greater than 150 μM VC/g DW in the whole plants at the stage of 19 July and 29 July (germination time), the root and stem of 5 August (growth time), the root and leaf of 23 August, 2 September, and 13 September (growth and flowering time), and the leaf of 22 September, 2 October, 12 October 12th (flowering and yield time). Meanwhile, the antioxidant activity was less than 90 μM VC/g DW in the stem at the stage of 13 September–12 October (flowering and yield time). Furthermore, the highest antioxidant activity was observed with the leaf of 2 September showing 162 μM VC/g DW. The lowest antioxidant capacity was presented with the stem of 12 October (61 μM VC/g DW).
Figure 2. DPPH and ABTS radical scavenging and FRAP activities of the different parts of Tartary buckwheat throughout the growth stages.
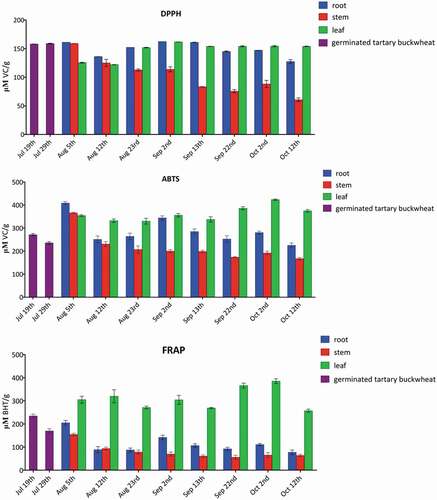
ABTS radical scavenging assay was also employed for evaluation of free radical scavenging properties. The antioxidant activities were higher than 330 μM VC/g DW in the root, stem, and leaf of at the stage of 5 August (growth time), the leaf of 12 August, 23 August, 2 September, 13 September, 22 September, 2 October, and 12 October (growth, flowering, and yield time), and the root of 2 September (flowering time). In addition, the antioxidant activities were lower than 200 μM VC/g DW in the stem of 13 September,, 22 September, 2 October, and 12 October (flowering and yield time). Moreover, the leaf of 2 October possessed the strongest radical scavenging capacity (424 μM VC/g DW). The stem of 12 October exhibited the weakest activity (168 μM VC/g DW).
In FRAP method it measured the capacity of an extract to reduce the ferric ions into the ferrous ions by electron transfer. The antioxidant activities were higher than 200 μM BHT/g DW in the leaf of 5 August, 12 August, 23 August, 2 September, 13 September, 22 September, 2 October, and 12 October (growth, flowering, and yield time), the root of 5 August (growth time). Whereas the antioxidant activities were less than 80 μM BHT/g DW in the stem of 23 August, 2 September, 13 September, 22 September, 2 October, and 12 October (growth, flowering, and yield time), and the root of 12 October (yield time).
The results indicated the three kinds of antioxidant activity assessments shared similar tendency. shows that all samples display antioxidant activities. The leaf exhibited the highest antioxidant activities, followed by Tartary buckwheat sprout, the root, and the stem. These data suggest that the antioxidant activities may be correlated with the contents of total phenolics, flavonoids, and phenylpropanoid glycosides in Tartary buckwheat. At the same time, the HPLC profiles in revealed that probably the leaf contained more rutin and the root more phenylpropanoid with high antioxidant activity.
α-Glucosidase inhibitory activity of Tartary buckwheat
α-Glucosidase is an important enzyme, which is involved in the carbohydrate digestion. α-Glucosidase inhibitory activity was measured for the different growth periods and parts of Tartary buckwheat at a concentration of 0.5 mg/mL. The results are as shown in . The root was more effective than the leaf, stem, and acarbose. The α-glucosidase inhibition rate of root ranged from 50.7% to 87.9%. The maximum α-glucosidase inhibition (87.9%) was achieved by the root of 12 October. The inhibition rates were more than 80% in the root of 23 August, 2 September, 13 September, 22 September, 2 October, and 12 October during the flowering and yield stages. However, the inhibition rate of acarbose excerted 87.4% inhibition at a concentration of 5 mg/mL.
The differences in the chemical composition of the root, stem, and leaf resulted in the above distinct inhibition ability. The phenylpropanoid glycosides were the predominant components in both the root and stem as shown in . However, the α-glucosidase inhibition rate of stem was relatively low, and the inhibition rate was generally from 2.9% to 9.0%. So, it is supposed that the phenylpropanoid glycosides should not have α-glucosidase inhibitory activity. Based on the chemical identification of the sample solution of root, it was presumed that the components in the root that played a role in the α-glucosidase inhibitory activity may be polysaccharides. In addition, the α-glucosidase inhibition rate of leaf ranged from 9.2% to 53.8%. Rutin was the main component in the leaf. The α-glucosidase inhibitory activity was not related to the change of rutin content, which indicated that rutin might not contribute to α-glucosidase inhibition. However, the leaf of 5 August (early growth stage) had a significant α-glucosidase inhibition rate as 53.8%, which was consistent with the literature,[Citation12] presumably that D-chiro-inositol plays a role in α-glucosidase inhibitory activity. The results indicated that the Tartary buckwheat root and young seedling had a great potential to alleviate postprandial hyperglycemia. Inhibitors from food sources might minimize side effects such as abdominal distention, nausea, vomiting, and diarrhea and be used as an auxiliary treatment of diabetes for chemical drugs.[Citation29]
Phytochemical profiles and quantitative analysis
Phytochemical profiles can be used for direct and efficient exhibition and recognition of chemical compositions in different growth stages and various parts of Tartary buckwheat as shown in . The rutin and chlorogenic acid were the major components in leaves and stems. Comparatively, the phenylpropanoid glycosides were predominant in roots.[Citation30] In addition, the phenylpropanoid glycosides were not detected in the germinated Tartary buckwheat sprouts (19 July and 29 July).
An HPLC-DAD procedure[Citation14,Citation30] was developed for simultaneous quantification of rutin and chlorogenic acid in Tartary buckwheat collected at different growth stages and from different parts. Because the germination growth is not fully differentiated, there is no distinction between the various parts of the Tartary buckwheat of 19 July and 29 July. The rutin and chlorogenic acid concentrations ranged from 157.6 to 3112.2 μg/g and from 58.1 to 137.2 μg/g in Tartary buckwheat sprouts.
From 5 August to 12 October, the Tartary buckwheat was differentiated, thus the samples were classified as the root, stem, and leaf. The contents of rutin and chlorogenic acid in the root ranged from 8.2 to 1277.7 μg/g and 18.1 to 62.3 μg/g. It is worth noting that during the early growth stage the highest contents of two components in the root were 1277.7 and 62.3 μg/g, respectively. With the growth of the plant, the contents of two compounds gradually decreased, and chlorogenic acid was not detected after 12 August. The changes of contents of rutin and chlorogenic acid followed the same pattern in the stem. In Tartary buckwheat stem, rutin varied from 8.2 to 1305.7 μg/g, while chlorogenic acid from 19.4 to 144.3 μg/g. However, the changes of the content of rutin and chlorogenic acid in leaf were slightly different from those in the root and stem. In the early growth stage and in the later yield stage, the contents of rutin and chlorogenic acid were relatively low, but the contents are relatively high in the flowering period. The specific range of rutin was from 2763.3 to 4424.7 μg/g, while chlorogenic acid from 21.5 to 77.2 μg/g. The above quantitative results during different growth stages and parts had been listed in . Lee et al. reported that the rutin content was 10.7 and 1.99 mg/g in Tartary buckwheat groat and hull, respectively.[Citation6] Li et al. reported that ethephon-induced phenylpropanoid accumulation in Tartary buckwheat hairy root. In particular, the main rutin was increased by 20% from 15.56 to 19.06 mg/g in with 0.5 mg/L ethephon treatment.[Citation31]
Table 1. Content of two compounds in the different growth stages and parts of Tartary buckwheat (μg/g).
For phenylpropanoid glycosides, there was no quantitative determination due to the lack of standard substances. However, according to the chromatographic peak area, it was found that the contents of phenylpropanoid glycosides in roots and stems were relatively high in the flowering and yield stages.
In our previous research, an online stop-flow heart-cutting two-dimensional high performance liquid chromatography was designed and constructed to determine the four phenylpropanoid glycosides, i.e. 1,3,6-tri-p-coumaroyl-6′-feruloyl sucrose (0.01–0.56 mg/g) 3,6-di-p-coumaroyl-1,6′-di-feruloyl sucrose (0.01–1.19 mg/g)), 1,6,6′-tri-feruloyl-3-p-coumaroyl sucrose (0.02–0.44 mg/g), and 1,3,6,6′-tetra-feruloyl sucrose (0.01–0.32 mg/g).[Citation30]
Conclusion
In this study, we found that the Tartary buckwheat root extracts showed significant activity of α-glucosidase inhibition and remarkable antioxidant activity in the flowering and yield stage. The α-glucosidase inhibition rate of root (0.5 mg/mL) ranged from 50.7% to 87.9%. However, the inhibition rate of acarbose excerted 87.4% inhibition at a concentration of 5 mg/mL. Furthermore, the antioxidant capacity were determined using DPPH, ABTS, and FRAP. The leaf extracts exhibit the highest antioxidant activities, followed by the root, and the stem extracts. In addition, HPLC-DAD method was established to profile chemical compositions in the different growth stages and parts of Tartary buckwheat. Rutin was the main component in the leaf. The phenylpropanoid glycosides were the predominant components in the root. Above all, the tartary buckwheat root during the flowering and yield stage might be a valuable antidiabetic food supplement. The Tartary buckwheat leaves could be used as natural antioxidants.
Highlights
The Tartary buckwheat root extracts showed significant inhibition of α-glucosidase in the flowering and yield stage.
It also showed good antioxidant capacity using DPPH, ABTS, and FRAP determination.
The leaf exhibited the highest antioxidant activities, followed by the root and stem.
The phytochemical profiles showed the leaf contained more rutin and the root more phenylpropanoid.
Supplemental Material
Download ()Acknowledgments
This investigation was supported by grants from NSFC cultivation project of Jining Medical University (No. JYP201717) and Fund for Teachers’ research of Jining Medical University (JYFC2018KJ042). We also thank Shandong Provincial Natural Science Foundation, China (No. ZR2015HL124).
Supplementary material
Supplementary data for this article can be accessed here.
References
- Liu, C. L.; Chen, Y. S.; Yang, J. H.; Chiang, B. H. Antioxidant Activity of Tartary (Fagopyrum Tataricum (L.) Gaertn.) And Common (Fagopyrum Esculentum Moench) Buckwheat Sprouts. J. Agric. Food Chem. 2008, 56, 173–178. DOI: 10.1021/jf072347s.
- Wang, M.; Liu, J. R.; Gao, J. M.; Parry, J. W.; Wei, Y. M. Antioxidant Activity of Tartary Buckwheat Bran Extract and Its Effect on the Lipid Profile of Hyperlipidemic Rats. J. Agric. Food Chem. 2009, 57, 5106–5112. DOI: 10.1021/jf900194s.
- Guo, X. D.; Wu, C. S.; Ma, Y. J.; Parry, J.; Xu, Y. Y.; Liu, H.; Wang, M. Comparison of Milling Fractions of Tartary Buckwheat for Their Phenolics and Antioxidant Properties. Food Res. Int. 2012, 49, 53–59. DOI: 10.1016/j.foodres.2012.07.019.
- Qin, P. Y.; Wu, L.; Yao, Y.; Ren, G. X. Changes in Phytochemical Compositions, Antioxidant and α-glucosidase Inhibitory Activities during the Processing of Tartary Buckwheat Tea. Food Res. Int. 2013, 50, 562–567. DOI: 10.1016/j.foodres.2011.03.028.
- Bao, T.; Wang, Y.; Li, Y. T.; Gowd, V.; Niu, X. H.; Yang, H. Y.; Chen, L. S.; Chen, W.; Sun, C. D. Antioxidant and Antidiabetic Properties of Tartary Buckwheat Rice Flavonoids after in Vitro Digestion. J. Zhejiang Univ. Sci. B. 2016, 17, 941–951. DOI: 10.1631/jzus.B1600243.
- Lee, L. S.; Choi, E. J.; Kim, C. H.; Sung, J. M.; Kim, Y. B.; Seo, D. H.; Choi, H. W.; Choi, Y. S.; Kum, J. S.; Park, J. D. Contribution of flavonoids to the Antioxidant Properties of Common and Tartary Buckwheat. J. Cereal Sci. 2016, 68, 181–186. DOI: 10.1016/j.jcs.2015.07.005.
- Tsai, H.; Deng, H.; Tsai, S.; Hsu, Y. Bioactivity Comparison of Extracts from Various Parts of Common and Tartary Buckwheat: Evaluation of the Antioxidant and Angiotensin-Converting Enzyme Inhibitory Activities. Chem. Cent. J. 2012, 6, 78–82. DOI: 10.1186/1752-153X-6-110.
- Guo, X. N.; Zhu, K. X.; Zhang, H.; Yao, H. Y. Purification and Characterization of the Antitumor Protein from Chinese Tartary Buckwheat (Fagopyrum Tataricum Gaertn.) Water-Soluble Extracts. J. Agric. Food Chem. 2007, 55, 6958–6961.
- Li, F. H.; Zhang, X. L.; Zheng, S. J.; Lu, K. K.; Zhao, G. H.; Ming, J. The Composition, Antioxidant and Antiproliferative Capacities of Phenolic Compounds Extracted from Tartary Buckwheat Bran [Fagopyrum Tartaricum (L.) Gaerth]. J. Funct. Foods. 2016, 22, 145–155. DOI: 10.1016/j.jff.2016.01.027.
- Yao, Y.; Shan, F.; Bian, J. S.; Chen, F.; Wang, M. F.; Ren, G. X. D-Chiro-Inositol-Enriched Tartary Buckwheat Bran Extract Lowers the Blood Glucose Level in KK-Ay Mice. J. Agric. Food Chem. 2008, 56, 10027–10031. DOI: 10.1021/jf801879m.
- Li, Y. Q.; Zhou, F. C.; Gao, F.; Bian, J. S.; Shan, F. Comparative Evaluation of Quercetin, Isoquercetin and Rutin as Inhibitors of α-glucosidase. J. Agric. Food Chem. 2009, 57, 11463–11468. DOI: 10.1021/jf903083h.
- Qin, P. Y.; Wei, A. C.; Zhao, D. G.; Yao, Y.; Yang, X. S.; Dun, B. Q.; Ren, G. X. Low Concentration of Sodium Bicarbonate Improves the Bioactive Compound Levels and Antioxidant and α-glucosidase Inhibitory Activities of Tartary Buckwheat Sprouts. Food Chem. 2017, 224, 124–130. DOI: 10.1016/j.foodchem.2016.12.059.
- Tomotake, H.; Kayashita, J.; Kato, N. Hypolipidemic Activity of Common (Fagopyrum Esculentum Moench) and Tartary (Fagopyrum Tataricum Gaertn.) Buckwheat. J. Sci. Food Agric. 2015, 95, 1963–1967. DOI: 10.1002/jsfa.2015.95.issue-10.
- Ren, Q.; Wu, C. S.; Ren, Y.; Zhang, J. L. Characterization and Identification of the Chemical Constituents from Tartary Buckwheat (Fagopyrum Tataricum Gaertn) by High Performance Liquid Chromatography/Photodiode Array Detector/Linear Ion Trap FTICR Hybrid Mass Spectrometry. Food Chem. 2013, 136, 1377–1389. DOI: 10.1016/j.foodchem.2012.09.052.
- Zhu, F. Chemical Composition and Health Effects of Tartary Buckwheat. Food Chem. 2016, 203, 231–245. DOI: 10.1016/j.foodchem.2016.02.050.
- Van de Laar, F. A.; Lucassen, P. L.; Akkermans, R. P.; Van de Lisdonk, E. H.; Rutten, G. E.; Van Weel, C. Alpha-Glucosidase Inhibitors for Type 2 Diabetes Mellitus. Cochrane Database Syst. Rev. 2005, 18, CD003639.
- Yang, T. K.; Lee, Y. H.; Paudel, U.; Bhattarai, G.; Yun, B. S.; Hwang, P. H.; Yi, H. K. Davallialactone from Mushroom Reduced Premature Senescence and Inflammation on Glucose Oxidative Stress in Human Diploid Fibroblast Cells. J. Agric. Food Chem. 2013, 61, 7089–7095. DOI: 10.1021/jf401691y.
- Ahmad, W.; Ljaz, B.; Shabbiri, K.; Ahmed, F.; Rehman, S. Oxidative Toxicity in Diabetes and Alzheimer’s Disease: Mechanisms behind ROS/RNS Generation. J. Biomed. Sci. 2017, 24, 76–86. DOI: 10.1186/s12929-017-0379-z.
- Wang, L.; Li, X. D.; Niu, M.; Wang, R.; Chen, Z. X. Effect of Additives on Flavonoids, D-chiro-Inositol and Trypsin Inhibitor during the Germination of Tartary Buckwheat Seeds. J. Cereal Sci. 2013, 58, 348–354. DOI: 10.1016/j.jcs.2013.07.004.
- Lee, S. W.; Seo, J. M.; Lee, M. K.; Chun, J. H.; Antonisamy, P.; Arasu, M. V.; Suzuki, T.; Al-Dhabi, N. A.; Kim, S. J. Influence of Different LED Lamps on the Production of Phenolic Compounds in Common and Tartary Buckwheat Sprouts. Ind. Crops Prod. 2014, 54, 320–326. DOI: 10.1016/j.indcrop.2014.01.024.
- Ghimeray, A. K.; Sharma, P.; Phoutaxay, P.; Salitxay, T.; Woo, S. H.; Park, S. U.; Park, C. H. Far Infrared Irradiation Alters Total Polyphenol, Total flavonoid, Antioxidant Property and Quercetin Production in Tartary Buckwheat Sprout Powder. J. Cereal Sci. 2014, 59, 167–172. DOI: 10.1016/j.jcs.2013.12.007.
- Peng, L. X.; Zou, L.; Su, Y. M.; Fan, Y.; Zhao, G. Effects of Light on Growth, Levels of Anthocyanin, Concentration of Metabolites in Fagopyrum Tataricum Sprout Cultures. Int. J. Food Sci. Technol. 2015, 50, 1382–1389. DOI: 10.1111/ijfs.12780.
- Zhou, X. L.; Hao, T. F.; Zhou, Y. M.; Tang, W.; Xiao, Y.; Meng, X. X.; Fang, X. Relationships between Antioxidant Compounds and Antioxidant Activities of Tartary Buckwheat during Germination. J. Food Sci. Technol. 2015, 52, 2458–2463. DOI: 10.1007/s13197-014-1290-1.
- Pongrac, P.; Potisek, M.; Fraś, A.; Likar, M.; Budič, B.; Myszka, K.; Boros, D.; Nečemer, M.; Kelemen, M.; Vavpetič, P.; et al. Composition of Mineral Elements and Bioactive Compounds in Tartary Buckwheat and Wheat Sprouts as Affected by Natural Mineral-Rich Water. J. Cereal Sci. 2016, 69, 9–16. DOI: 10.1016/j.jcs.2016.02.002.
- Brand-Williams, W.; Cuvelier, M. E.; Berset, C. Use of a Free Radical Method to Evaluate Antioxidant Activity. Food Sci. Technol. 1995, 28, 25–30.
- Re, R.; Pellegrini, N.; Proteggente, A.; Pannala, A.; Yang, M.; Rice-Evans, C. Antioxidant Activity Applying an Improved ABTS Radical Cation Decolorization Assay. Free Radical Biol. Med. 1999, 26, 1231–1237. DOI: 10.1016/S0891-5849(98)00315-3.
- Dong, H. Q.; Li, M.; Zhu, F.; Liu, F. L.; Huang, J. B. Inhibitory Potential of Trilobatin from Lithocarpus Polystachyus Rehd against α-glucosidase and α-amylase Linked to Type 2 Diabetes. Food Chem. 2012, 130, 261–266.
- Sheliya, M. A.; Rayhana, B.; Ali, A.; Pillai, K. K.; Aeri, V.; Sharma, M.; Mir, S. R. Inhibition of α-glucosidase by New Prenylated Flavonoids from Euphorbia Hirta L. Herb. J. Ethnopharmacol. 2015, 176, 1–8. DOI: 10.1016/j.jep.2015.10.018.
- Kwon, Y. I.; Apostolidis, E.; Shetty, K. Inhibitory Potential of Wine and Tea against α-amylase and α-glucosidase for Management of Hyperglycemia Linked to Type 2 Diabetes. J. Biol. Chem. 2008, 32, 15–31.
- Ren, Q.; Wu, C. S.; Zhang, J. L. Use of On-Line Stop-Flow Heart-Cutting Two-Dimensional High Performance Liquid Chromatography for Simultaneous Determination of 12 Major Constituents in Tartary Buckwheat (Fagopyrum Tataricum Gaertn). J. Chromatogr. A. 2013, 1304, 257–262. DOI: 10.1016/j.chroma.2013.07.008.
- Li, X. H.; Thwe, A. A.; Park, C. H.; Kim, S. J.; Arasu, M. V.; Al-Dhabi, N. A.; Lee, S. Y.; Park, S. U. Ethephon-Induced Phenylpropanoid Accumulation and Related Gene Expression in Tartary Buckwheat (Fagopyrum Tataricum (L.) Gaertn.) Hairy Root. Biotechnol. Biotechnol. Equip. 2017, 31, 304–311. DOI: 10.1080/13102818.2017.1282835.