ABSTRACT
Allelic variation at the Wx locus plays a vital role in regulating apparent amylose content (AAC) as well as other important starch properties in rice grain. However, the extent of Wx allelic variation affecting amylopectin fine structure and enzymatic hydrolysis properties of rice starch remains unknown. In this paper, a series of rice single-segment substitution lines with five different Wx alleles (wx, Wxt, Wxg1, Wxg2 and Wxg3) were used for comparative studies. The results showed that the five Wx alleles exhibited a wide variation in amylopectin molecular structure, hydrolysis and in vitro digestion properties of rice starch. Amylopectin chain length distribution as evaluated by fluorophore-assisted capillary electrophoresis was significantly different for the five Wx alleles, which might result in different functional properties. The hydrolysis degree of the five Wx genotype starches by α-amylase or amyloglucocidase was in the order wx > Wxt > Wxg1 > Wxg3 > Wxg2. The rapidly digestible starch (RDS) content of the tested rice starches followed the order wx = Wxg3 > Wxt = Wxg1 > Wxg2. The extent decrease of slowly digestible starch (SDS) content was wx = Wxt > Wxg1 > Wxg2 > Wxg3, while the trend in resistant starch (RS) content was Wxg2 > Wxg3 > Wxg1 > Wxt > wx. Correlation analysis indicated that AAC was significantly positively correlated with RS but significantly negatively correlated with SDS and hydrolysis. The RDS showed a significant positive correlation with amylopectin short chains (DP 6–12) but no significant correlations with other structural properties. The present results could provide important information for the application of rice starches with different Wx genotypes.
Intrduction
Rice (Oryza sativa L.) is a major and staple cereal crop in the world. Rice starch is not only the primary food source for the humans, but has also been widely utilized in various industrial applications.[Citation1] In rice grain, starch is stored as semicrystalline granules and comprises a mixture of two polysaccharides: linear amylose and highly branched amylopectin, respectively.[Citation2] Amylose, which makes up 2–30% of rice starch, is an important factor affecting the structural and functional properties of starch.[Citation3–Citation7]
The synthesis of amylose is catalyzed by the granule-bound starch synthase, which is encoded by the Waxy (Wx) gene.[Citation8] According to apparent amylose content (AAC) phenotypes, the rice Wx gene can be mainly divided into five common functional alleles, i.e., wx, Wxt, Wxg1, Wxg2 and Wxg3, which correspond to glutinous, low, intermediate, high I (24.36–25.20%), and high II (25.81–26.19%) AACs, respectively.[Citation9] Using single-segment substitution lines (SSSLs) harboring five different Wx alleles as materials, effects of the five different Wx alleles (and their AAC phenotypes) on crystalline structure and some functional properties of starch, including gel consistency, alkali spreading value, pasting viscosity traits, thermal and swelling properties, have been successfully obtained against a same rice genetic background of the recipient HJX74.[Citation6,Citation7] Moreover, we postulated that amylopectin molecular structure, apart from amylose content, also plays a significant role in determining the diversities in rice starch properties that result from the Wx allelic variation.[Citation6,Citation7] At present, however, there is a lack of information on the differences in amylopectin fine structure between the five functional Wx alleles..
Typically, application of starch requires the disruption of starch granules. Enzymatic hydrolysis of starch is involved in many biological and industrial processes, such as digestion, fermentation, or industrial production of glucose syrup and bioethanol.[Citation10] The enzymatic hydrolysis properties of starch are generally determined using α-amylase and amyloglucosidase in many studies.[Citation11–Citation14] These enzymes have the advantages of exoactivity of glucoamylase, which erodes the outer surface of starch granule and creates sharp and deep pinholes, as well as the endoactivity of α-amylase that enables widening of the pinholes and consequently leads to the breakdown of granule.[Citation11,Citation15] Starch digestion is a highly important metabolic process that occurs mostly in the small intestine, where the starch is hydrolyzed to produce glucose in the presence of amylase and maltase. The rate and extent of starch digestibility are nutritionally important, and are usually measured by in vitro methods using pancreatic α-amylase containing amyloglucosidase to simulate the digestion in the small intestine.[Citation16,Citation17] On the basis of digestion rate, starch has been classified into three fractions: rapidly digestible starch (RDS), slowly digestible starch (SDS), and resistant starch (RS).[Citation16] To date, the relationship between different Wx alleles and enzymatic hydrolysis properties of rice starch has not been well established. A few previous studies have reported that higher amylose content is associated with reduced susceptibility to enzymatic hydrolysis of rice starch.[Citation3–Citation5,Citation18,Citation19] However, to what extent the five Wx alleles (or their AAC phenotypes) affect hydrolysis and in vitro digestion properties of rice starch remains unclear, which restricts the utilization of different Wx genotype starches in practice.
This research continues the studies of Teng et al.[Citation6,Citation7] to discover the genetic effects of different Wx alleles on amylopectin fine structure and enzymatic hydrolysis properties of rice starch in a common rice genome background. We aimed to compare the chain length distribution of amylopectin, as well as the hydrolysis and in vitro digestion properties of starches from rice SSSLs with the five different Wx alleles, and we analyzed the relationship between starch structural features and enzymatic hydrolysis properties of rice starch. The results can be used to understand the structural mechanisms of how the Wx allelic variation affects starch functional properties.
Materials and methods
Plant materials
Four rice SSSLs carrying four different Wx alleles (wx, Wxt, Wxg1 and Wxg3) and the recipient HJX74 with the Wxg2 allele used by Teng et al.[Citation6,Citation7] were investigated in this study (). All the rice accessions were grown on the experimental farm of Anhui Academy of Agricultural Sciences, Hefei, P. R. China, in the normal planting season (from May to September) in 2011. Grain samples from the five tested rice used for the study of amylopectin molecular structure and enzymatic hydrolysis properties were the same samples previously harvested by Teng et al.,[Citation7] who reported the crystalline, thermal and swelling properties of these starch samples.
Table 1. Single-segment substitution lines with different Wx alleles used in the present study.
Isolation of native rice starches
Native starches were isolated from mature grains following the method described by Wei et al.[Citation20] Briefly, the brown rice was steeped in deionized water for 2 days and homogenized in a mortar with a pestle. The homogenate was squeezed through five layers of cotton cloth and then filtered with 100-, 200-, and 400-mesh sieves, successively. The starch was washed with deionized water and centrifuged at 3000 g for 10 min. After removing the dirty layer on the top of starch precipitate, the starch was further washed with deionized water and dehydrated with anhydrous ethanol. Finally, the starch was dried under atmosphere, ground into powder, and passed through a 100-mesh sieve.
Preparation of linear glucans for structure characterization
The starch was deproteinized with protease and sodium bisulfite and then debranched with isoamylase following the methods of Syahariza et al.[Citation21] and Lin et al.[Citation22] with some modifications. Briefly, 4 mg of dry starch was transferred to a 2 mL microcentrifuge tube and incubated with 0.5 mL of protease in tricine buffer (2.5 U/mL) using a ThermoMixer (350 rpm) at 37°C for 30 min. The sample was centrifuged at 4000 g for 10 min, and then the precipitation was mixed with 0.5 mL of sodium bisulfite solution (0.45%, w/v) using a ThermoMixer (350 rpm) at 37°C for 30 min. The sample was centrifuged again, and the precipitation was suspended in 1.5 mL LiBr/DMSO solution (0.5%, w/v) at 80°C for 24 h using a ThermoMixer with continuous shaking (350 rpm). The sample was centrifuged at 4000 g for 10 min, and the supernatant was mixed with 10 mL absolute ethanol to precipitate the starch. The precipitation was dispersed in 0.9 mL warm deionized water and then incubated in boiling water for 10 min. The sample was cooled to room temperature, and then 0.1 mL of 0.1 M acetate buffer (pH 3.5), 5 μL of sodium azide solution (4%, w/v), and 2.5 μL of isoamylase (Megazyme) was added. The whole mixture was incubated at 37°C for 3 h and then neutralized with 0.1 mL NaOH (0.1 M). The obtained linear glucans were freeze-dried using liquid nitrogen and then stored at −80°C until used for structure analysis.
Determination of chain length distribution in amylopectin
The chain length distribution of amylopectin was determined using Fluorophore-assisted capillary electrophoresis (FACE). The debranched starch (0.3 mg) was labeled with 1.5 μL of 8-aminopyrene −1,3,6, trisulfonic acid (APTS) labeling dye (0.2 M APTS, 1.0 M sodium cyanoborohydride in 15% acetic acid) in a 2 mL microcentrifuge tube. The mixture was incubated in a water bath at 60°C for 90 min, diluted by adding 80 μL of water, and then centrifuged at 4000 g for 2 min. The supernatant (50 μL) was analyzed using a FACE (Beckman Coulter PA800, Fullerton, CA, USA) system according to the method as described by Wang et al.[Citation23] The experiments were performed in duplicate. According to the degree of polymerization (DP), amylopectin branch chains are categorized into four chain types: A chains (DP 6–12), B1 chains (DP 13–24), B2 chains (DP 25–36), and B3+ chains (DP ≥ 37).[Citation24] To eliminate the influence of starch sample weight, all FACE weight distributions were normalized into the same area. The average branch chain length of amylopectin was obtained by calculating the ratio of total glucose (DP 6–100 × their areas) to total areas of DP 6–100. The ratio of (A+ B1)/(B2+ B3+) was used to represent the short to long chains distribution of amylopcetin.
Hydrolysis degree determination
Starch was hydrolyzed by porcine pancreatic-amylase (PPA, Sigma A3176) or Aspergillus niger amyloglucosidase (AAG, Megazyme E-AMGDF) alone using the methods of Cai et al.[Citation5] with some modifications. For PPA hydrolysis, 50 mg of starch was suspended in 10 mL of enzyme solution (0.1 M phosphate sodium buffer, pH 6.9, 25 mM NaCl, 5 mM CaCl2, 0.02% NaN3, and 50 U PPA). For AAG hydrolysis, 50 mg of starch was suspended in 10 mL of enzyme solution (0.05 M acetate buffer, pH 4.5, and 5 U AAG). The enzymatic hydrolysis was conducted in a ThermoMixer with continuous shaking (800 rpm) at 37°C for PPA or at 55°C for AAG hydrolysis. After hydrolysis, the enzymatic reaction was stopped by placing the mixture in an ice bath, and the starch slurry was quickly centrifuged (5000 g) at 4°C for 5 min. The supernatant was used for measuring the soluble carbohydrates to quantify the degree of hydrolysis with the anthrone-H2SO4 method. The experiments were repeated in triplicate.
In vitro digestion analysis of starch
In vitro digestion of starch was measured by a method modified from Carciofi et al.[Citation12] following the procedure of Englyst et al.[Citation16] A total of 20 mg of native starch was incubated in 4 mL of enzyme solution (20 mM sodium phosphate buffer, pH 6.0, 6.7 mM NaCl, 0.01% NaN3, 2.5 mM CaCl2, 4 U PPA, 4 U AAG) at 37°C with continuous shaking (800 rpm) for 20 min and 1, 2, 6, 12, and 24 h. Enzyme treatment was terminated by adding 480 μL 0.1 M HCl and 2 mL of 50% ethanol on ice and centrifuging (14,000 g, 5 min). The supernatant was collected, and the amounts of glucose were determined by the D-glucose (GOPOD Format) Assay Kit (Megazyme K-GLUC). Starch nutritional fractions are defined based on the rate of hydrolysis. The amount of rapidly digestible starch (RDS) was obtained by measuring the glucose released after 20 min of incubation. The slowly digestible starch (SDS) fraction was the starch hydrolyzed between 20 and 120 min. The starch that remained unhydrolyzed after 120 min was measured as resistant starch (RS). The experiments were carried out in triplicate.
Data analysis
All statistical analyses were performed using SAS software version 7 (SAS Institute, Inc., Cary, NC). Means were tested by least significant difference at the P = 0.05 level (Duncan0.05). Correlation analysis was conducted to characterize the relationships between structural and functional characteristics of starch. The data of AAC and crystalline structure properties of the five starch samples used for correlation calculations was cited from Teng et al.[Citation7]
Results and discussion
Molecular structure of amylopectin
Genotypic differences in amylopectin fine structure between different Wx alleles were analyzed using FACE. As expected, perceptible differences could be observed among the normalized chromatograms of the five Wx alleles (). The chain number distribution of debranched amylopectin from FACE is shown in . All starch samples displayed typical number distribution of debranched chains, which was concordant with that of normal rice starches.[Citation23,Citation25] Comparison of structural characteristics of amylopectin clearly discriminated the five Wx alleles from each other (). Among the tested rice starches, the glutinous (wx) and Wxg3 genotype starches displayed obviously higher proportions of short A chains (DP 6–12) than those of other Wx genotypes, and the lowest amount of short A chains was found in the Wxg2 genotype starch. The proportion of amylopectin short B1 chains (DP 13–24) followed the order wx = Wxt > Wxg1 = Wxg2 > Wxg3. For long B2 chains (DP 25–36), no significant differences were observed between different Wx alleles. The relative effects of the five Wx alleles on the amount of very long B3+ chains (DP ≥ 37) were in the order Wxg1 = Wxg2 = Wxg3 > Wxt > wx. The average chain length of amylopectin of the tested starches ranged between 22.07 and 23.39, and the wx and Wxt alleles exhibited significantly shorter values than the Wxg1, Wxg2 and Wxg3 alleles. Contrary to this finding, the ratio of (A+ B1)/(B2+ B3+) of starches was found to be in the order wx = Wxt > Wxg1 = Wxg2 = Wxg3.
Table 2. Amylopectin chain length distribution of rice starches from single-segment substitution lines with different Wx allelesa.
Figure 1. FACE chromatogram of amylopectin in endosperm from rice single-segment substitution lines with different Wx alleles. (a) Molar percentage distribution of isoamylase-debranched amylopectin and (b) chain length number distribution. All the chromatograms of branch chain length distribution of amylopectin were normalized in order to avoid the effect of different sample concentrations.
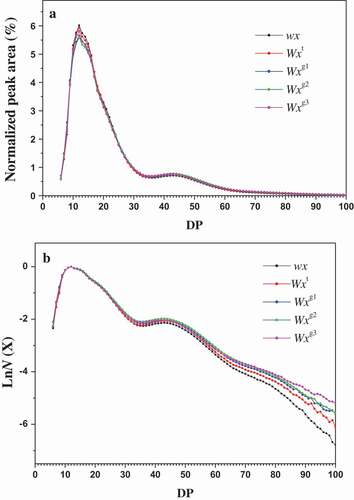
In this study, the associations between AAC, amylopectin molecular structure, and crystalline structure properties were also examined (). The results showed that the proportion of amylopectin short A chains (DP 6–12) had no significant correlations with AAC and crystalline structure characteristics. The proportion of short amylopectin chains (DP 13–24) was significantly positively correlated with relative crystallinity and significantly negatively correlated with AAC. In contrast, the proportions of long chains DP 25–36 and DP ≥ 37 were significantly positively correlated with AAC and negatively correlated with relative crystallinity and the ratio of 1047/1022 cm−1. The amylopectin average chain length exhibited a significant positive correlation with AAC but a negative correlation with relative crystallinity. In addition, it was observed that amylopectin chain ratio of (A+ B1)/(B2+ B3+) was significantly positively correlated with relative crystallinity and the ratio of 1047/1022 cm−1, but significantly negatively correlated with AAC.
Table 3. Correlation coefficients between structural characteristics of starches from rice single-segment substitution lines with different Wx allelesa.
Hydrolysis properties of starch
In this study, the enzymatic hydrolysis of starch was performed using two types of amylases, PPA and AAG. Generally, PPA hydrolyzes interior α-1,4-glucosidic bonds, whereas amyloglucosidase hydrolyzes α-1,4 and α-1,6- glucosidic bonds and releases glucose.[Citation11] The hydrolysis patterns of native starches with different Wx genotypes measured by PPA or AAG alone are presented in . The hydrolysis degree increased with increasing hydrolysis time. The time course of hydrolysis was characteristic of biphasic, with a relatively rapid rate at the initial stage (0 to 12 h), and then followed by a progressively decreased rate until the end of 72 h. Similarly, this biphasic trend of amylase hydrolysis has also been observed in other starch samples.[Citation11,Citation13,Citation14,Citation26,Citation27]
Figure 2. Hydrolysis properties of native starches from rice single-segment substitution lines with different Wx alleles. Vertical bars represent mean values ± standard deviations (n = 3). The hydrolysis degrees are expressed as the percentage of soluble carbohydrates released from native starches by PPA (a) and AAG (b).
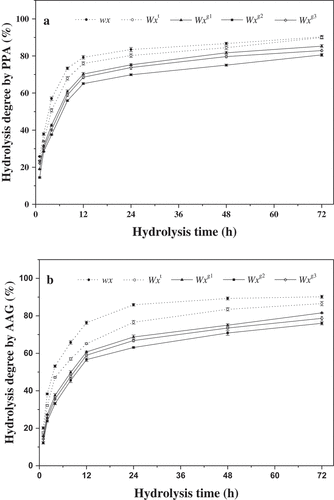
As shown in , the resistance to hydrolysis was very different between the five Wx genotype starches. The wx and Wxt alleles had high values of hydrolysis, whereas the Wxg1, Wxg2 and Wxg3 alleles had relatively low hydrolysis degrees. On the whole, the influence of different Wx alleles on hydrolysis degrees of PPA and AAG both followed the order wx > Wxt > Wxg1 > Wxg3 > Wxg2 (). Though the hydrolysis degree of starches by PPA was similar to that by AAG, the former was relatively higher than the latter (Supplementary Table 1). Susceptibility of native starch to amylase is influenced by many factors, including amylose content, amylopectin molecular structure, crystalline structure, granule size and integrity, and structural in-homogeneities.[Citation3,Citation5,Citation14,Citation18,Citation19,Citation26–Citation29] It has generally been considered that the rate of starch hydrolysis by amylase is inversely related to amylose content.[Citation5,Citation11,Citation14,Citation18,Citation19,Citation30] Consistently, in this study, starches with larger amounts of amylose tended to have higher resistance to enzymatic hydrolysis, with the exception of Wxg3 genotype starch ( and ). When compared with the Wxg2 allele, the Wxg3 genotype starch was found to have significantly higher AAC but lower hydrolysis by amylase ( and ). In native starch granules, amylopectin long chains can make long helices, strengthen hydrogen bonds between chains, producing a superior crystalline structure. On the other hand, amylopectin short chains form short or weak double helices and produce inferior crystalline structures, which are more susceptible to hydrolysis than the perfect crystalline structure.[Citation31,Citation32] In the present study, the Wxg3 genotype starch exhibited significantly higher proportion of short A chains (DP 6–12) than that of the Wxg2 genotype starch (), which might lead to its relatively higher values of hydrolysis by PPA or AAG. Finally, it is worth noting that the rate of enzyme hydrolysis of starch influenced by the Wx allelic variation is not only dependent on the level of amylose, but also dependent on the structural properties of amylopectin.
In vitro digestion properties of starch
In vitro digestion by PPA and AAG was carried out to simulate the effects of small intestine hydrolysis and the subsequent glycemic response of starch. The rate of digestibility of native starches from the tested rice SSSLs at various digestion times (0 to 24 h) is presented in . Similar to the time courses for PPA and AAG hydrolysis, two phases were distinguished in the in vitro digestion of starch. The initial rapid digestion phase was from 0 to 6 h, which was then followed by a relatively slower phase. In general, the differences in digestion degree between the five Wx alleles followed the order wx > Wxt > Wxg1 > Wxg3 > Wxg2, which was in agreement with that of hydrolysis degree in this study ( and ).
Figure 3. Digestion properties of native starches from rice single-segment substitution lines with different Wx alleles. Vertical bars represent mean values ± standard deviations (n = 3). The digestion degrees are expressed as the percentage of glucose released from native starches by PPA and AAG.
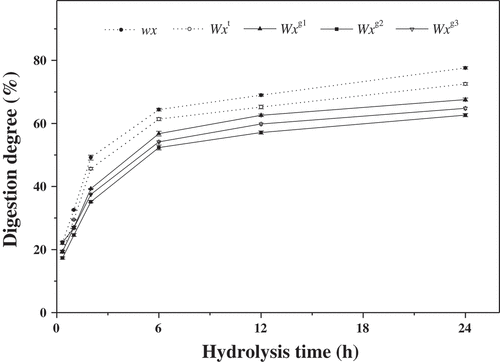
The amounts of RDS, SDS and RS in the native starches are presented in . The RDS, SDS and RS showed significant differences among the five Wx alleles and ranged from 17.33 to 22.23%, 15.26 to 27.06%, and 50.81 to 64.83%, respectively. These results indicate that the Wx allelic variation plays an important role in regulating starch digestion. The digestive rate of starch is affected by various factors, including amylose content, molecular structure of amylopectin, and degree of crystallinity.[Citation3–Citation5,Citation33–Citation35] Previous studies have shown that the content of RDS is negatively correlated with amylose content.[Citation3,Citation5] However, in the current study, RDS content of the tested starches was found to be in the order of wx = Wx3 > Wxt = Wxg1 > Wxg2, which was not inversely related to their amylose levels ( and ). For example, the wx and Wxg3 alleles showed no differences in RDS content () but had the largest differences in their AAC phenotypes (). This difference could be ascribed to the different genetic materials tested, since the rice materials investigated by previous studies[Citation3,Citation5] might not possess the Wxg3 allele. On the other hand, it was suggested that RDS content was positively correlated with the number of amylopectin short branch chains (DP 6–12).[Citation3,Citation36] In the present study, it was observed that the proportion of DP 6–12 in amylopectin showed the same variation trend with the amount of RDS ( and ), further demonstrating that the DP 6–12 in amylopectin plays an important role in determining RDS content. Thus, from the above results, it could be inferred that the Wx allelic variation may affect RDS content mainly through the influence of amylopectin short chains with DP 6–12 but not AAC. Presumably, of this study, the reason that the glutinous and Wxg3 genotype starches had the largest amounts of RDS could be attributed to their relatively higher proportions of amylopectin short chains (DP 6–12) compared to other Wx genotype starches. The Wxg2 genotype starch having the lowest percentage of RDS might be explained by it having the lowest proportion of DP 6–12 in amylopectin ().
Table 4. In vitro digestion properties of native starches from rice single-segment substitution lines with different Wx allelesa.
Slowly digestible starch is related to the digestion fraction, which is completely but slowly digested in the small intestines.[Citation16] As seen in , the wx and Wxt genotype starches displayed obviously higher levels of SDS (26.33–27.06%) than those of other Wx genotypes (15.26–19.89%). The effects of different Wx alleles on SDS content followed the order wx = Wxt > Wxg1 > Wxg2 > Wxg3, showing an opposite trend to their corresponding amylose levels ( and ). Similarly, Cai et al.[Citation5] reported that the SDS content decreased with increasing AAC in various rice starches. It was claimed that SDS could be influenced by crystalline structures, as dictated by its relative crystallinity and ratio of 1047/1022 cm−1,[Citation3,Citation5,Citation33,Citation37,Citation38] since the large fraction of crystalline structures could be hydrolyzed during the intermediate and late stages of digestion, resulting in increased SDS content.[Citation38] Amylopectin has generally been considered responsible for starch crystallinity, while amylose disrupts the crystalline packing of amylopectin.[Citation39] Therefore, it is not difficult to understand that the amount of SDS decreased with increasing AAC. In this study, the wx and Wxt genotype starches consisted of lower AACs and higher values of relative crystallinity and ratio of 1047/1022 cm−1 as determined in our previous study,[Citation7] which might result in their higher SDS contents. On the other hand, amylopectin chain length distribution could also influence SDS content. The present results showed that higher proportion of short B1 chains (DP 13–24) and lower proportion of long B3+ chains (DP≥37) could contribute to a higher percentage of SDS ( and ).
Resistant starch is defined as the fraction of starch not digested in the small intestine.[Citation16] In the present study, significant differences in RS content were observed between the five Wx alleles, and the order for RS content was Wxg2 > Wxg3 > Wxg1 > Wxt > wx, which was similar to the order of their AAC phenotypes ( and ). This result was in keeping with previous reports that the content of RS increased with increasing amylose content.[Citation3–Citation5] However, it should be noted that the variation in RS content among different Wx alleles could not be solely explained by the influence of AAC. For example, the Wxg2 allele showed higher RS content but lower AAC than the Wxg3 allele ( and ), indicating that other factors such as amylopectin molecular structure may also have an impact on RS content. As suggested by Jane et al.,[Citation36] short branch chains (DP 6–12) of amylopectin could induce weak points in the starch crystalline structure, resulting in a greater susceptibility to enzymatic hydrolysis. In this study, the Wxg2 allele significantly reduced the proportion of short chains (DP 6–12) in amylopectin compared with the Wxg3 allele (), which might retard the digestion of starch. As a result, the Wxg2 genotype starch displayed a higher RS content than the Wxg3 genotype starch (). These results suggested that the differences in AAC and amylopectin structure features that resulted from the Wx allelic variation codetermined the observed variations in the content of RS.
Enzyme hydrolysis properties in relation to structural characteristics of starch
To understand the structural foundation of how the Wx allelic variation influences the functional properties of rice starch, the relationships between structural characteristics and enzyme hydrolysis properties of the tested starches were determined using Pearson correlation analysis (). According to the hydrolysis process, the hydrolysis degree at 12 h (end stage of the initial rapid phase) could maximally reflect the variance among all starch samples (), so this was selected as the parameter for correlation analysis. As expected, the AAC was significantly negatively correlated with PPA hydrolysis (r = −0.954, P < 0.05) and AAG hydrolysis (r = −0.954, P < 0.05), which was in accordance with previous reports.[Citation5,Citation11,Citation14,Citation18,Citation19,Citation30] On the other hand, the hydrolysis by PPA and AAG was significantly positively correlated with relative crystallinity and the ratio of (A+ B1)/(B2+ B3+) but significantly negatively correlated with amylopectin long chains (DP 25–36 and DP ≥ 37) and average chain length of amylopectin.
Table 5. Correlation coefficients between molecular structure and enzyme hydrolysis of starches from rice single-segment substitution lines with different Wx allelesa.
Prior to this study, a few researches have been undertaken to clarify the relationship between structural properties and starch nutritional fractions of rice starch.[Citation3–Citation5,Citation29,Citation37,Citation38] However, it is difficult to get a clear description of the correlations between the structural and digestibility properties, since the rice samples used in these studies have great diversity in their genetic background. The SSSLs having different Wx alleles provided an opportunity for us to effectively investigate the relationship between structural and functional characteristics of rice starch because the diversification in starch properties caused by the Wx allelic variation could be associated against a common genetic background.[Citation6,Citation7] The present study indicated that the content of RDS had a significant positive correlation with the proportion of DP 6–12 (r = 0.981, P < 0.01) but did not have significant correlations with any other structural characteristics. Slowly digestible starch was significantly positively correlated with relative crystallinity (r = 0.955, P < 0.05), the proportion of DP 13–24 (r = 0.944, P < 0.05) and the ratio of (A+ B1)/(B2+ B3+) (r = 0.886, P < 0.05) but significantly negatively correlated with AAC (r = −0.959, P < 0.01), the proportion of long B3+ chains (DP ≥ 37) (r = −0.895, P < 0.05), and amylopectin average chain length (r = −0.945, P < 0.05). Contrary to SDS, the opposite trend was observed for RS, which exhibited significant positive correlations with AAC (r = 0.976, P < 0.01), long B3+ chains (r = 0.963, P < 0.01), long B2 chains (r = 0.944, P < 0.05) and amylopectin average chain length (r = 0.972, P < 0.01) but significant negative correlations with relative crystallinity (r = −0.952, P < 0.05) and the ratio of (A+ B1)/(B2+ B3+) (r = −0.965, P < 0.01). Overall, the above results may help us understand how the five functional Wx alleles affect enzymatic hydrolysis properties through the influence of AAC and structural characteristics of rice starch.
Conclusion
Genetic effects of different Wx alleles on the amylopectin molecular structure and enzymatic hydrolysis properties of rice starch against a common rice genetic background were obtained using a set of single-segment substitution lines harboring five different Wx alleles. The present results showed that the Wx allelic variation plays a substantial role in the regulation of amylopectin chain length distribution, hydrolysis and in vitro digestibility of rice starch. Further comparison and correlation analyses revealed that AAC and amylopectin molecular structure were the major factors affecting the enzymatic hydrolysis properties of rice starch, which might contribute to a better understanding of how the Wx allelic variation affects starch functional properties through influencing starch structure. The current study can provide valuable information to food and nonfood industries regarding the use of rice starches with different Wx genotypes.
Supplemental Material
Download ()Supplementary Material
Supplemental data for this article can be accessed here
Additional information
Funding
References
- Champagne, E. T. Rice Starch Composition and Characteristics. Cereal Foods World. 1996, 41, 833–838.
- Fujita, N. Starch Biosynthesis in Rice Endosperm. AGri Biosci. Monogr. 2014, 4, 1–18. DOI: 10.5047/agbm.2014.00401.0001.
- Chung, H. J.; Liu, Q.; Lee, L.; Wei, D. Z. Relationship between the Structure, Physicochemical Properties and in Vitro Digestibility of Rice Starches with Different Amylose Contents. Food Hydrocoll. 2011, 25, 968–975. DOI: 10.1016/j.foodhyd.2010.09.011.
- Zhu, L. J.; Liu, Q. Q.; Wilson, J. D.; Gu, M. H.; Shi, Y. C. Digestibility and Physicochemical Properties of Rice (Oryza Sativa L.) Flours and Starches Differing in Amylose Content. Carbohydr. Polym. 2011, 86, 1751–1759. DOI: 10.1016/j.carbpol.2011.07.017.
- Cai, J.; Man, J.; Huang, J.; Liu, Q.; Wei, W.; Wei, C. Relationship between Structure and Functional Properties of Normal Rice Starches with Different Amylose Contents. Carbohydr. Polym. 2015, 125, 35–44. DOI: 10.1016/j.carbpol.2015.02.067.
- Teng, B.; Zhang, Y.; Wu, J.; Cong, X.; Wang, R.; Han, Y.; Luo, Z. Association between Allelic Variation at the Waxy Locus and Starch Physicochemical Properties Using Single-Segment Substitution Lines in Rice (Oryza Sativa L.). Starch/Stärke. 2013, 65, 1069–1077. DOI: 10.1002/star.201300034.
- Teng, B.; Zhang, Y.; Du, S.; Wu, J.; Li, Z.; Luo, Z.; Yang, J. Crystalline, Thermal and Swelling Properties of Starches from Single-Segment Substitution Lines with Different Wx Alleles in Rice (Oryza Sativa L.). J. Sci. Food Agric. 2017, 97, 108–114. DOI: 10.1002/jsfa.7693.
- Zeeman, S. C.; Kossmann, J.; Smith, A. M. Starch: Its Metabolism, Evolution, and Biotechnological Modification in Plants. Annu. Rev. Plant Biol. 2010, 61, 209–234. DOI: 10.1146/annurev-arplant-042809-112301.
- Teng, B.; Zeng, R.; Wang, Y.; Liu, Z.; Zhang, Z.; Zhu, H.; Ding, X.; Li, W.; Zhang, G. Detection of Allelic Variation at the Wx Locus with Single-Segment Substitution Lines in Rice (Oryza Sativa L.). Mol. Breed. 2012, 30, 583–595. DOI: 10.1007/s11032-011-9647-x.
- Tawil, G.; Viksø-Nielsen, A.; Rollandsabaté, A.; Colonna, P.; Buléon, A. In Depth Study of a New Highly Efficient Raw Starch Hydrolyzing α-amylase from Rhizomucor Sp. Biomacromolecules. 2011, 12, 34–42. DOI: 10.1021/bm100913z.
- Li, J. H.; Vasanthan, T.; Hoover, R.; Rossnagel, B. G. Starch from Hull-Less Barley: V. In-Vitro Susceptibility of Waxy, Normal, and High-Amylose Starches Towards Hydrolysis by Alpha-Amylases and Amyloglucosidase. Food Chem. 2004, 84, 621–632. DOI: 10.1016/S0308-8146(03)00287-5.
- Carciofi, M.; Blennow, A.; Jensen, S. L.; Shaik, S. S.; Henriksen, A.; Buléon, A.; Holm, P. B.; Hebelstrup, K. H. Concerted Suppression of All Starch Branching Enzyme Genes in Barley Produces Amylose-Only Starch Granules. BMC Plant Biol. 2012, 12, 223. DOI: 10.1186/1471-2229-12-223.
- Cai, J.; Yang, Y.; Man, J.; Huang, J.; Wang, Z.; Zhang, C.; Gu, M.; Liu, Q.; Wei, C. Structural and Functional Properties of Alkali-Treated High-Amylose Rice Starch. Food Chem. 2014, 145, 245–253. DOI: 10.1016/j.foodchem.2013.08.059.
- Lin, L.; Guo, D.; Huang, J.; Zhang, X.; Zhang, L.; Wei, C. Molecular Structure and Enzymatic Hydrolysis Properties of Starches from High-Amylose Maize Inbred Lines and Their Hybrids. Food Hydrocolloids. 2016, 58, 246–254. DOI: 10.1016/j.foodhyd.2016.03.001.
- Li, X.; Gao, W.; Wang, Y.; Jiang, Q.; Huang, L. Granule Structural, Crystalline, and Thermal Changes in Native Chinese Yam Starch after Hydrolysis with Two Different Enzymes– α-amylase and Gluco-Amylase. Starch/Stärke. 2011, 65, 75–82. DOI: 10.1002/star.201000104.
- Englyst, H. N.; Kingman, S. M.; Cummings, J. H. Classification and Measurement of Nutritionally Important Starch Fractions. Eur. J. Clin. Nutr. 1992, 45, S33–S50.
- McCleary, B. V.; Monaghan, D. A. Measurement of Resistant Starch. J. AOAC Int. 2002, 85, 665–675.
- Noda, T.; Nishiba, Y.; Sato, T.; Suda, I. Properties of Starches from Several Low-Amylose Rice Cultivars. Cereal Chem. 2003, 80, 193–197. DOI: 10.1094/CCHEM.2003.80.2.193.
- Lin, L.; Zhang, L.; Cai, X.; Liu, Q.; Zhang, C.; Wei, C. The Relationship between Enzyme Hydrolysis and the Components of Rice Starches with the Same Genetic Background and Amylopectin Structure but Different Amylose Contents. Food Hydrocoll. 2018, 84, 406–413. DOI: 10.1016/j.foodhyd.2018.06.029.
- Wei, C. X.; Xu, B.; Qin, F. L.; Yu, H. G.; Chen, C.; Meng, X. L.; Zhu, L.; Wang, Y.; Gu, M.; Liu, Q. C-Type Starch from High-Amylose Rice Resistant Starch Granules Modified by Antisense RNA Inhibition of Starch Branching Enzyme. J. Agric. Food Chem. 2010, 58, 7383–7388. DOI: 10.1021/jf100385m.
- Syahariza, Z. A.; Li, E.; Hasjim, J. Extraction and Dissolution of Starch from Rice and Sorghum Grains for Accurate Structural Analysis. Carbohydr. Polym. 2010, 82, 14–20. DOI: 10.1016/j.carbpol.2010.04.014.
- Lin, L.; Cai, C.; Gilbert, R. G.; Li, E.; Wang, J.; Wei, C. Relationships between Amylopectin Molecular Structures and Functional Properties of Different-Sized Fractions of Normal and High-Amylose Maize Starches. Food Hydrocoll. 2016, 52, 359–368. DOI: 10.1016/j.foodhyd.2015.07.019.
- Wang, K.; Jovin, H.; Wu, A. C.; Li, E.; Henry, R. J.; Gilbert, R. G. Roles of GBSSI and SSIIa in Determining Amylose Fine Structure. Carbohydr. Polym. 2015, 127, 264–274. DOI: 10.1016/j.carbpol.2015.03.072.
- Hanashiro, I.; Abe, J.; Hizukuri, S. A. Periodic Distribution of the Chain Length of Amylopectin as Revealed by High-Performance Anion-Exchange Chromatography. Carbohydr. Res. 1996, 283, 151–159. DOI: 10.1016/0008-6215(95)00408-4.
- Wu, A. C.; Gilbert, R. G. Molecular Weight Distributions of Starch Branches Reveal Genetic Constraints on Biosynthesis. Biomacromolecules. 2010, 11, 3539–3547. DOI: 10.1021/bm1010189.
- Man, J.; Yang, Y.; Huang, J.; Zhang, C.; Zhang, F.; Wang, Y.; Gu, M.; Liu, Q.; Wei, C. Morphology and Structural Properties of High-Amylose Rice Starch Residues Hydrolysed by Amyloglucosidase. Food Chem. 2013, 138, 2089–2098. DOI: 10.1016/j.foodchem.2012.12.009.
- Man, J.; Yang, Y.; Zhang, C.; Zhang, F.; Wang, Y.; Gu, M.; Liu, Q.; Wei, C. Morphology and Structural Characterization of High-Amylose Rice Starch Residues Hydrolyzed by Porcine Pancreatic α-amylase. Food Hydrocoll. 2013, 31, 195–203. DOI: 10.1016/j.foodhyd.2012.11.003.
- Blazek, J.; Gilbert, E. P. Effect of Enzymatic Hydrolysis on Native Starch Granule Structure. Biomacromolecules. 2010, 11, 3275–3289. DOI: 10.1021/bm101124t.
- Sasaki, T.; Kohyama, K.; Suzuki, Y.; Okamoto, K.; Noel, T. Y.; Ring, S. G. Physicochemical Characteristics of Waxy Rice Starch Influencing the in Vitro Digestibility of a Starch Gel. Food Chem. 2009, 116, 137–142. DOI: 10.1016/j.foodchem.2009.02.024.
- Tester, R. F.; Qi, X.; Karkalas, J. Hydrolysis of Native Starches with Amylases. Anim. Feed Sci. Technol. 2006, 130, 39–54. DOI: 10.1016/j.anifeedsci.2006.01.016.
- Chung, H. J.; Liu, Q.; Donner, E.; Hoover, R.; Warkentin, T. D.; Vandenberg, B. Composition, Molecular Structure, Properties, and in Vitro Digestibility of Starches from Newly Released Canadian Pulse Cultivars. Cereal Chem. 2008, 85, 471–479. DOI: 10.1094/CCHEM-85-4-0471.
- Srichuwong, S.; Sunarti, T. C.; Mishima, T.; Isono, N.; Hisamatsu, M. Starches from Different Botanical Sources I: Contribution of Amylopectin Finestructure to Thermal Properties and Enzyme Digestibility. Carbohydr. Polym. 2005, 60, 529–538. DOI: 10.1016/j.carbpol.2005.03.004.
- Chung, H. J.; Lim, H. S.; Lim, S. T. Effect of Partial Gelatinization and Retrogradation on the Enzymatic Digestion of Waxy Rice Starch. J. Cereal Sci. 2006, 43, 353–359. DOI: 10.1016/j.jcs.2005.12.001.
- Srichuwong, S.; Jane, J. Physicochemical Properties of Starch Affected by Molecular Composition and Structure: A Review. Food Sci. Biotechnol. 2007, 16, 663–674.
- Ambigaipalan, P.; Hoover, R.; Donner, E.; Liu, Q.; Jaiswal, S.; Chibbar, R.; Nantanga, K. K. M.; Seetharaman, K. Structure of Faba Bean, Black Bean and Pinto Bean Starches at Different Levels of Granule Organization and Their Physicochemical Properties. Food Res. Int. 2011, 44, 2962–2974. DOI: 10.1016/j.foodres.2011.07.006.
- Jane, J.; Wong, K. S.; McPherson, A. E. Branch-Structure Difference in Starches of A- and B-Type X-Ray Patterns Revealed by Their Naegeli Dextrins. Carbohydr. Res. 1997, 300, 219–227. DOI: 10.1016/S0008-6215(97)00056-6.
- You, S. Y.; Oh, S. K.; Kim, H. S.; Chung, H. J. Influence of Molecular Structure on Physicochemical Properties and Digestibility of Normal Rice Starches. Int. J. Biol. Macromol. 2015, 77, 375–382. DOI: 10.1016/j.ijbiomac.2015.02.054.
- You, S. Y.; Lim, S. T.; Lee, J. H.; Chung, H. J. Impact of Molecular and Crystalline Structures on in Vitro Digestibility of Waxy Rice Starches. Carbohydr. Polym. 2014, 112, 729–735. DOI: 10.1016/j.carbpol.2014.05.096.
- Cheetham, N. W. H.; Tao, L. Variation in Crystalline Type with Amylose Content in Maize Starch Granules: An X-Ray Powder Diffraction Study. Carbohydr. Polym. 1998, 36, 277–284. DOI: 10.1016/S0144-8617(98)00007-1.