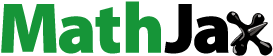
ABSTRACT
This study aimed to determine the efficacy of seven predominant wine terpenoids (i.e. α-pinene, limonene, myrcene, geraniol, linalool, nerol, and terpineol) against foodborne pathogenic bacteria, as well as to observe their antioxidant activities. Antibacterial activities were observed against foodborne pathogenic bacteria. MIC50 and MBC values for Escherichia coli, Salmonella enterica, and Staphylococcus aureus were in the ranges of 0.420–1.598 mg/mL and 0.673–3.432 mg/mL, respectively. The terpenoid α-pinene showed the strongest DPPH free radical scavenging (IC50 value = 12.57 ± 0.18 mg/mL) and the highest reducing power (213.7 ± 5.27 μg/mL of L-ascorbic acid equivalents). However, the DPPH free radical scavenging of the terpenoids was found to be lower than that of butylated hydroxytoluene, which is known to be a strong reducing agent. The seven predominant terpenoids in wines that were identified in this study could be new potential sources of natural antibacterial and antioxidant agents for use in the food industry.
Introduction
A wide variety of aromatic substances are found in wines; the volatile composition is one of the most important parameters responsible for determining wine quality and consumer acceptance.[Citation1,Citation2] Thus, studies have identified hundreds of species, such as terpenoids, aliphatic compounds, that are present in wine. Terpenoids are responsible for a typical aroma in wine, and they can, therefore, be used analytically for varietal characterization. Some popular wine products, such as Agudelo, Chardonnay, Gewurztraminer, Macabeo, Muscat, and Riesling, have characteristic terpenoids.[Citation3] The flavor threshold of nerol and terpineol is three to four times higher than that of linalool.[Citation4] Linalool has a flavor threshold of 25.2 μg/L.[Citation5] PangzhenZhang et al.[Citation6] reported five monoterpenoids, 24 sesquiterpenes, and four norisoprenoids were detected in grape samples. The terpenoids have also application to cancer treatment, prevention, cardioprotection, endocrine toxicity/ reproductive dysfunction, food supplement, traditional medicine, immunology, inflammation, menopausal syndromes, and neuroprotection.[Citation7]
Foodborne pathogenic bacteria and oxidation in foods not only result in food deterioration and shelf life reduction but also lead to disease and economic losses. Currently, these two factors are considered major problems in the food industry and human nutrition. Many studies have demonstrated the antibacterial and antioxidant activities of wines[Citation8–Citation10], especially owing to essential oils or aromatic compounds found in them. Essential oils and their main components possess a wide spectrum of biological activity, which may be of great importance in several fields such as food chemistry, pharmacology, as well as pharmaceutics.[Citation11] The main advantage of essential oils is that they can be used in any foods and are generally recognized as safe (GRAS).[Citation12] However, the antibacterial activity of terpenoids in wines against foodborne pathogenic bacteria is yet to be studied, in addition to their potential antioxidant properties. Previous literature has only explored these properties in some essential oils.[Citation13,Citation14] The terpenoids – α-pinene, limonene, myrcene, geraniol, linalool, nerol, and terpineol – have been identified as the seven most predominant terpenoids in wines.[Citation1,Citation15]
Synthetic food additives generate a negative perception in consumers. Therefore, food manufacturers search for safer natural alternatives such as plant essential oils. The objective of this study was to determine the antibacterial activity of these seven predominant wine terpenoids. These activities were tested against typical foodborne pathogenic bacteria (Escherichia coli, Salmonella enterica, and Staphylococcus aureus). DPPH free radical and reducing power assays were used to evaluate the antioxidant activities of the generated mutants.
Materials and methods
Samples
Myrcene, limonene, geraniol, linalool, and nerol were purchased from Tokyo Chemical Industry (Tokyo, JAPAN); α-pinene was purchased from Sigma-Aldrich (St. Louis, MO, USA), and terpineol from ACROS Organics (Pittsburgh, PA, USA).
Chemicals
L-Ascorbic acid and 2,2-diphenyl-1-picrylhydrazyl (DPPH) were purchased from Sigma (St. Louis, MO, USA), potassium hexacyanoferrate and butylated hydroxyanisole (BHA) were from Acros Organics (Pittsburgh, PA, USA), iron(III) chloride from Showa (Saitama, JAPAN), and trichloroacetic acid (TCA) from Alfa Aesar (Ward hill, MA, USA).
Antibacterial activity
Microbial strains
Escherichia coli, Salmonella enterica, and Staphylococcus aureus were used in all experiments. These strains represent foodborne pathogenic bacteria. These strains were supplied by the author’s laboratory, National Kaosiung University Science and Technology. Strains were stored at −80°C through cryopreservation. For preparation of the inoculum, strains were cultured in tryptone soya broth (TSB) (Oxoid, Basingstoke, Hampshire, England) overnight. All microbial cultures were sub-cultured before the assay and were later diluted with phosphate buffer saline (pH 7.4) to obtain a microbial suspension of 1.00 × 106 colony-forming units (CFUs)/mL for further experiments.
Minimum inhibitory concentration (MIC50) assay
The minimum inhibitory concentration (MIC50) was determined using the agar dilution method described in a previous report[Citation16] with slight modifications. Samples were diluted with sterilized 10% Tween-80 to a final concentration of 8 mg/mL to 0.4 mg/mL. Each dilution was inoculated with 100-µl aliquots of bacterial culture with a concentration of approximately 1.00 × 106 CFU/mL. This was done for each of the strains in triplicate, followed by incubation at 37°C for 16 h at 125 rpm. Sterilized 10% (v/v) Tween-80 without the inoculum was used as the growth control, while the medium without the inoculum was used as the blank control (negative). The growth in each test group was compared with that of the growth control. The MIC50 was defined as the lowest concentration of test samples with a growth reduction greater than or equal to 50%, compared with the growth control.
Minimum bactericidal concentration (MBC) assay
MBC was defined as the lowest concentration of the samples wherein no visible turbidity or colonies were observed.[Citation17] In order to determine the MBC, the solution for concentration being tested was spread on sterile PCA (Oxoid, Basingstoke, Hampshire, England) plates and incubated at 37°C for 24 h. This was done for all three bacterial strains in triplicate.
Time-kill curve assay
The time-kill curve assay was conducted based on methods used in the previous research[Citation18,Citation19] with slight modifications. Experiments were performed using 25 mL of TSB (Oxoid, Basingstoke, Hampshire, England) broth and inoculated with the sub-cultured sample to obtain a solution with approximately 1.00 × 106CFU/mL as the final concentration. Time-kill curve assay was conducted for each bacterial strain using the predetermined concentrations of MIC50.
Samples for viable count determination were taken during the experiment at the 0, 1, 2, 4, 8, 12, 16, and 24 h during the experiment. Each sample was serially diluted and pour plating was used to prepare the plates. The number of CFUs per mL were determined following an incubation at 37°C for 24 h. Samples were compared with growth control (un-inoculated sterilized 10% (v/v) Tween-80) and the time-kill curves were generated using the log of CFU/mL over time. This was done for all three bacterial strains in triplicate.
Antioxidant activity
2,2-diphenyl-1-picrylhydrazyl (DPPH) free radical scavenging activity
The free radical scavenging activity was determined using the 2,2-Diphenyl-1-picrylhydrazyl (DPPH) method. The hydrogen atom or electron donation ability of the sample was measured using a methanol solution of 2,2-diphenyl-1-picrylhydrazyl (DPPH). A 20-µL aliquot of different sample concentrations (6.25–50 mg/mL) was mixed with 180 µL of DPPH solution (180 μM) in methanol in a 96-well plate.
DPPH solution was used as the blank sample, and BHA was used as positive control. After 40 min of incubation in the dark at room temperature, the samples were observed using a 96-well plate reader (HT 340 Elisa reader, Bio Tec) to monitor the decrease in absorbance at 517 nm. This was done for all three bacterial strains in triplicate. The free radical scavenging activity was expressed as the percentage inhibition of DPPH and was calculated using the following formula:
where A0 and AA are the absorbance values of the blank and test samples, respectively.[Citation20] The absorbance of the samples was measured at 517 nm against a blank and IC50 values were calculated using linear regression analysis. IC50 value was defined as concentration required for 50% inhibition, as compared to the control.[Citation21]
Reducing power assay
A sample volume of 1 mL was mixed with 1 mL of phosphate buffer (0.2 M, pH 6.6) and 1 mL of 1% potassium ferricyanide. This mixture was then incubated at 50°C for 20 min. This was then subjected to centrifugation at 3000 rpm for 10 min. A volume of 1 mL trichloroacetic acid solution (10%) was then added to the mixture followed by centrifugation again at 3000 rpm for 10 min. A volume of 100 μL supernatant was mixed with 100 μL of distilled water and 100 μL of ferric chloride (0.1%), and the absorbance was measured at 700 nm. This was done for all three bacterial strains in triplicate. L-Ascorbic acid was used as a positive control was used to generate a linear regression analysis for the calibration curve. The results of reducing power were expressed in micrograms of L-ascorbic acid equivalents per milliliter (μg/mL).
Statistical analysis
Data were expressed as mean ± standard deviation of three independent replicates. Statistical analysis was performed using the software SPSS for Windows 14.0. All data were analyzed using one-way analysis of variance (ANOVA). Post hoc analysis of group differences was performed by Tukey’s multiple comparison tests. The criterion for significance was set at p < 0.05.
Results and discussion
Antibacterial activity
Comparative antibacterial activity results of the seven predominant terpenoids in wines are shown in and . The three test microorganisms that were used to represent foodborne bacteria included one Gram-positive bacterium, Staphylococcus aureus, and two Gram-negative bacteria, Escherichia coli and Salmonella enterica. MIC50 values ranging from 0.420 to 0.747 mg/mL were obtained for the seven terpenoids against Staphylococcus aureus, 0.421–0.747 mg/mL for Escherichia coli, and 0.420–1.598 mg/mL for Salmonella enterica, as shown in . For all seven terpenoids, the concentration of 3.432 mg/mL was determined to be the MBC, as shown in . At a concentration of 0.421 mg/mL, limonene showed the strongest antibacterial activity among the three terpenoids without a hydroxyl group – α-pinene, limonene, and myrcene.
Table 1. Minimum inhibitory concentrations (MIC) of seven predominant terpenoids in wines against foodborne pathogenic bacteria
Table 2. Minimum inhibitory concentrations (MIC) of seven predominant terpenoids in wines against foodborne pathogenic bacteria
These results are similar to those reported previously.[Citation22] Nerol and geraniol exhibited similar antibacterial activity among the four terpenoids with a hydroxyl group–geraniol, linalool, nerol, and terpineol. The effects of bioactivity could be due to the diastereomeric structure.[Citation23,Citation24] For the results of MIC50 and MBC values, Gram-positive bacteria were observed to be slightly more sensitive than Gram-negative bacteria, possibly due to the hydrophilic cell wall structure of Gram-negative bacteria.[Citation25] The cell wall of Gram-negative bacteria is constituted mainly by a lipo-polysaccharide, which blocks the penetration of hydrophobic components of terpenoids and for this reason, Gram-positive bacteria are found to be more sensitive to the seven terpenoids.[Citation26]
Furthermore, the time-kill curve assay was used to determine the antibacterial activity at the predetermined MIC50 values. The time-kill dynamic process of seven predominant terpenoids in wines against all three bacterial samples is presented in . At the time points of 16 h to 24 h, the antibacterial activity of all seven predominant terpenoids was shown to reach a plateau, while the growth control showed a steep increase in antibiotic activity. Therefore, we found that the seven predominant terpenoids in wines exhibited effective antibacterial activity against all three bacterial samples at their predetermined MIC50 after 16 h.
Figure 1. Time-kill curve of seven predominant terpenoids in wines against foodborne pathogenic bacterias (Escherichia coli, Salmonella enterica and Staphylococcus aureus) at concentrations of MIC50. The time-kill curve of used for α-pinene (a), limonene (b), myrcene (c), geraniol (d), linalool (e) nerol (f), terpineol (g) and control (h)
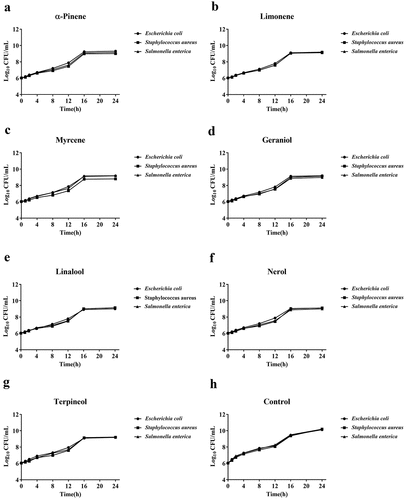
Antioxidant activity
Increases in free radicals in the body have been implicated in damaging human life.[Citation27,Citation28] Antioxidant properties are important in counteracting the deleterious effects of free radicals in foods and biological systems.[Citation29] For this reason, the antioxidant activity of seven predominant terpenoids in wines was determined using two methods, the determination of DPPH free radical scavenging activity and the reducing power assay.
The DPPH free radical is characterized as a stable free radical, which has been widely accepted as a method for estimating the free radical scavenging activity of antioxidant.[Citation30] Both are spectrophotometric methods for determining antioxidant activity, based on electron transfer reactions, which visually relies on the reduction of a colored oxidant, commonly used to investigate the in vitro antioxidant capacity of foods and biological samples.[Citation31] The results obtained from these methods present an excellent correlation.
The results of the assessment of the antioxidant activity by DPPH free radical scavenging activity are shown in . In this study, the antioxidant activity of seven predominantly terpenoids in wines were expressed as IC50, wherein a low IC50 value indicates that the sample is an effective DPPH scavenger.
Figure 2. IC50 values for DPPH free radical scavenging activity of seven predominant terpenoids in wines. Results are the mean of three separate determinations ± SEM
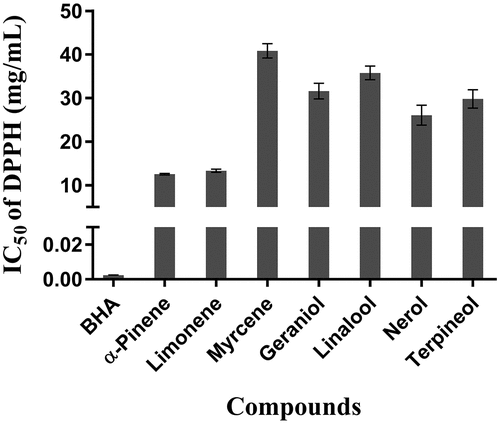
The DPPH free radical scavenging activity measures the ability of the sample to donate hydrogen to the DPPH radical, resulting in bleaching of the stable DPPH free radical from the purple color of DPPH cation to the yellow-colored diphenylpricryhydrazine. The greater the bleaching action observed, the higher the antioxidant activity, which is reflected in a lower IC50.[Citation32]
All seven terpenoids were determined to be effective DPPH scavengers with α-pinene showing the strongest DPPH free radical scavenging effect at 12.57 ± 0.18 mg/mL. This was followed by limonene at 13.35 ± 0.26 mg/mL and nerol at 26.08 ± 2.28 mg/mL. Myrcene (40.83 ± 1.68 mg/mL) showed the lowest scavenging activity. The determined IC50 values were in the order BHT < α-pinene < limonene < nerol < terpinol < geraniol < linalool < myrcene.
The reducing power of antioxidants is an important indicator of potential antioxidant activity. The antioxidant effect exponentially increases as a function of the reducing power, indicating that the antioxidant properties are concomitant with the development of reducing power.[Citation33] In the reducing power assay, the presence of reductants (antioxidants) in the seven predominant wine terpenoids causes the reduction of the Fe3+ or ferricyanide complex to its ferrous form.[Citation34]
The reducing power activity of the seven predominant wine terpenoids was determined using L-ascorbic acid as a standard. Results were expressed as micrograms of L-ascorbic acid equivalents per milliliter (μg/mL). The reducing power of the seven predominant wine terpenoids is presented in . Among them, α-pinene showed the highest reducing power at 213.7 ± 5.27 μg/mL, while myrcene showed the lowest reducing power at 21.59 ± 1.14 μg/mL. The reducing power was in the order: α-pinene (213.7 ± 5.27 μg/mL) > limonene (133.48 ± 6.22 μg/mL) > nerol (79.15 ± 3.75 μg/mL) > terpinol (73.92 ± 3.34 μg/mL) > geraniol (73.78 ± 3.94 μg/mL) > linalool (43.97 ± 1.09 μg/mL) > myrcene (21.59 ± 1.14 μg/mL).
Figure 3. Reducing power assay of seven predominant terpenoids in wines. Results are the mean of three separate determinations ± SEM and expressed as ascorbic acid equivalents (μg/mL)
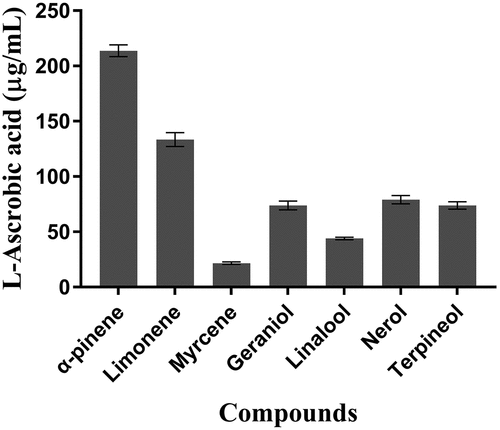
The results of the reducing power test were shown to agree with the results of the DPPH free radical scavenging activity. Additionally, a similar correlation or relative activity was observed between the DPPH free radical scavenging activity and the reducing power assay, indicating that the test samples had comparable activities in two independent methods for the determination of antioxidant activity. The antioxidant in vivo effects of α-pinene has previously been reported using the peroxidation assay.[Citation35]
In a separate study, the antioxidant activity of limonene has been demonstrated to have an important role in the ulcerative colitis (UC) rat model.[Citation36] Therefore, our results suggest that these seven predominant wine terpenoids have significant antioxidant activity. These results can be used as a reference for the food industry and nutrition in the future.
Conclusion
In the present study, antibacterial activity and antioxidant activity of seven predominant wine terpenoids were observed. α-pinene was found to have the highest antioxidant activity, as determined through DPPH free radical activity and reducing power, while limonene was found to have the highest antibacterial activity against Gram-positive/Gram-negative foodborne pathogenic bacteria. The seven predominant wine terpenoids displayed effective antibacterial activity against Staphylococcus aureus, Escherichia coli, and Salmonella enterica at a predetermined MIC50 after the 16-h timepoint. In addition, the samples’ DPPH free radical scavenging effectivity is of main interest to the food industry as oxidation in foods is accepted to be a major factor in determining food quality. For this reason, the use of seven predominant wine terpenoids in foods will require the identification of bioactive compounds as well as the determination of their mechanism of action. These could potentially be a source for natural, raw materials for nutrient products, cosmetic products, and food additives. In conclusion, this study verified the antibacterial activity and antioxidant activity of seven predominant wine terpenoids, which can be used as a helpful reference for the food industry in the future. The authors declare no conflicts of interest.
Acknowledgments
The author would thank all those who volunteered for assisting with the study.
Additional information
Funding
References
- Mateo, J. J.; Jiménez, M. Monoterpenes in grape juice and wines. Journal of Chromatography A 2000, 881 (1), 557-567 DOI: 10.1016/S0021-9673(99)01342-4.
- Vilanova, M.; Genisheva, Z.; Bescansa, L.; Masa, A.; Oliveira, J. M. Changes in Free and Bound Fractions of Aroma Compounds of Four Vitis Vinifera Cultivars at the Last Ripening Stages. Phytochemistry. 2012, 74, 196–205. DOI: 10.1016/j.phytochem.2011.10.004.
- Skinkis, P. A.; Bordelon, B. P.; Wood, K. V. Comparison of Monoterpene Constituents in Traminette, Gewürztraminer, and Riesling Winegrapes. Am. J. Enol. Vitic. 2008, 59(4), 440.
- Rapp, A. Wine Analysis; Springer-Verlag: Berlin, 1988; Vol. 6. pp 28.
- Cheng, G.; Liu, Y.; Yue, T.-X.; Zhang, Z.-W. Comparison between Aroma Compounds in Wines from Four Vitis Vinifera Grape Varieties Grown in Different Shoot Positions. Food Sci. Technol. 2015, 35, 237–246. DOI: 10.1590/1678-457X.6438.
- Zhang, P.; Fuentes, S.; Siebert, T.; Krstic, M.; Herderich, M.; Barlow, E. W. R.; Howell, K. Terpene Evolution during the Development of Vitis Vinifera L. Cv. Shiraz Grapes. Food Chem. 2016, 204, 463–474. DOI: 10.1016/j.foodchem.2016.02.125.
- Kiyama, R. Estrogenic Terpenes and Terpenoids: Pathways, Functions and Applications. Eur. J. Pharmacol. 2017, 815, 405–415. DOI: 10.1016/j.ejphar.2017.09.049.
- Friedman, M. Antibacterial, Antiviral, and Antifungal Properties of Wines and Winery Byproducts in Relation to Their Flavonoid Content. J. Agric. Food Chem. 2014, 62(26), 6025–6042. DOI: 10.1021/jf501266s.
- Cueva, C.; Mingo, S.; Muñoz-González, I.; Bustos, I.; Requena, T.; Del Campo, R.; Martín-Álvarez, P. J.; Bartolomé, B.; Moreno-Arribas, M. V. Antibacterial Activity of Wine Phenolic Compounds and Oenological Extracts against Potential Respiratory Pathogens. Lett Appl. Microbiol. 2012, 54(6), 557–563. DOI: 10.1111/j.1472-765X.2012.03248.x.
- Carmona-Jiménez, Y.; García-Moreno, M. V.; Igartuburu, J. M.; Garcia Barroso, C. Simplification of the DPPH Assay for Estimating the Antioxidant Activity of Wine and Wine By-Products. Food Chem. 2014, 165, 198–204. DOI: 10.1016/j.foodchem.2014.05.106.
- Cristani, M.; D’Arrigo, M.; Mandalari, G.; Castelli, F.; Sarpietro, M. G.; Micieli, D.; Venuti, V.; Bisignano, G.; Saija, A.; Trombetta, D. Interaction of Four Monoterpenes Contained in Essential Oils with Model Membranes: Implications for Their Antibacterial Activity. J. Agric. Food Chem. 2007, 55(15), 6300–6308. DOI: 10.1021/jf070094x.
- Kabara, J.J. Phenols and Chelators. In Food Preservatives; Russell, N.J., Gould, G.W., Eds.; Blackie: London, UK, 1991, 200-214
- Joshi, S.; Chanotiya, C. S.; Agarwal, G.; Prakash, O.; Pant, A. K.; Mathela, C. S. Terpenoid Compositions, and Antioxidant and Antimicrobial Properties of the Rhizome Essential Oils of Different Hedychium Species. Chem. Biodiversity. 2008, 5(2), 299–309. DOI: 10.1002/cbdv.200890027.
- Zengin, H.; Baysal, H. A. Antibacterial and Antioxidant Activity of Essential Oil Terpenes against Pathogenic and Spoilage-Forming Bacteria and Cell Structure-Activity Relationships Evaluated by SEM Microscopy. Molecules. 2014, 19(11). DOI: 10.3390/molecules190811211.
- Genovese, A.; Gambuti, A.; Piombino, P.; Moio, L. Sensory Properties and Aroma Compounds of Sweet Fiano Wine. Food Chem. 2007, 103(4), 1228–1236. DOI: 10.1016/j.foodchem.2006.10.027.
- Wiegand, I.; Hilpert, K.; Hancock, R. E. W. Agar and Broth Dilution Methods to Determine the Minimal Inhibitory Concentration (MIC) of Antimicrobial Substances. Nat. Protoc. 2008, 3, 163. DOI: 10.1038/nprot.2007.521.
- Olajuyigbe, O. O.; Afolayan, A. J. In Vitro Antibacterial and Time-Kill Evaluation of the Erythrina Caffra Thunb. Extract against Bacteria Associated with Diarrhoea. Sci. World J. 2012, 2012, 738314. DOI: 10.1100/2012/738314.
- May, J.; Chan, C. H.; King, A.; Williams, L.; French, G. L. Time–Kill Studies of Tea Tree Oils on Clinical Isolates. J. Antimicrob. Chemother. 2000, 45(5), 639–643.
- Foerster, S.; Unemo, M.; Hathaway, L. J.; Low, N.; Althaus, C. L. Time-Kill Curve Analysis and Pharmacodynamic Modelling for in Vitro Evaluation of Antimicrobials against Neisseria Gonorrhoeae. BMC Microbiol. 2016, 16, 216. DOI: 10.1186/s12866-016-0838-9.
- Yen, G. C.; Duh, P. D. Scavenging Effect of Methanolic Extracts of Peanut Hulls on Free-Radical and Active-Oxygen Species. J. Agric. Food Chem. 1994, 42(3), 629–632. DOI: 10.1021/jf00039a005.
- Sebaugh, J. L. Guidelines for Accurate EC50/IC50 Estimation. Pharm. Stat. 2011, 10(2), 128–134. DOI: 10.1002/pst.426.
- Espina, L.; Gelaw, T. K.; de Lamo-Castellví, S.; Pagán, R.; García-Gonzalo, D.; Hozbor, D. F. Mechanism of Bacterial Inactivation by (+)-Limonene and Its Potential Use in Food Preservation Combined Processes. PLoS ONE. 2013, 8(2), e56769. DOI: 10.1371/journal.pone.0056769.
- Banthorpe, D. V.; Le Patourel, G. N. J.; Francis, M. J. O. Biosynthesis of Geraniol and Nerol and Their β-d-glucosides in Perlargonium Graveolens and Rosa Dilecta. Biochem. J. 1972, 130(4), 1045–1054.
- Jimenez-Aleman, G. H.; Machado, R. A. R.; Görls, H.; Baldwin, I. T.; Boland, W. Synthesis, Structural Characterization and Biological Activity of Two Diastereomeric JA-Ile Macrolactones. Org. Biomol. Chem. 2015, 13(21), 5885–5893. DOI: 10.1039/C5OB00362H.
- Silhavy, T. J.; Kahne, D.; Walker, S. The Bacterial Cell Envelope. Cold Spring Harbor Perspect .Biol. 2010, 2(5), a000414. DOI: 10.1101/cshperspect.a000414.
- Beveridge, T. J. Structures of Gram-Negative Cell Walls and Their Derived Membrane Vesicles. J. Bacteriol. 1999, 181(16), 4725–4733.
- Lobo, V.; Patil, A.; Phatak, A.; Chandra, N. Free Radicals, Antioxidants and Functional Foods: Impact on Human Health. Pharmacogn. Rev. 2010, 4(8), 118–126. DOI: 10.4103/0973-7847.70902.
- Zweier, J. L.; Talukder, M. A. H. The Role of Oxidants and Free Radicals in Reperfusion Injury. Cardiovasc. Res. 2006, 70(2), 181–190. DOI: 10.1016/j.cardiores.2006.02.025.
- Pham-Huy, L. A.; He, H.; Pham-Huy, C.; Radicals, F. Antioxidants in Disease and Health. Int. J. Biomed. Sci. 2008, 4(2), 89–96.
- Kedare, S. B.; Singh, R. P. Genesis and Development of DPPH Method of Antioxidant Assay. J. Food Sci. Technol. 2011, 48(4), 412–422. DOI: 10.1007/s13197-011-0251-1.
- Hidalgo, G.-I.; Almajano, M. P. Red Fruits: Extraction of Antioxidants, Phenolic Content, and Radical Scavenging Determination: A Review. Antioxidants. 2017, 6(1), 7. DOI: 10.3390/antiox6010007.
- Lim, Y.; Theng, L. I. M.; Tee, T.; Jhi, J. Antioxidant Properties of Guava Fruit: Comparison with Some Local Fruits. Sunway Acad. J.. 2006, 3, 9–20.
- Oyaizu, M. Studies on Products of Browning Reaction Antioxidative Activities of Products of Browning Reaction Prepared from Glucosamine. Jpn. J. Nutr. Diet. 1986, 44(6), 307–315. DOI: 10.5264/eiyogakuzashi.44.307.
- Rahman, M. M.; Islam, M. B.; Biswas, M.; Khurshid Alam, A. H. M. In Vitro Antioxidant and Free Radical Scavenging Activity of Different Parts of Tabebuia Pallida Growing in Bangladesh. BMC Res. Notes. 2015, 8, 621. DOI: 10.1186/s13104-015-1618-6.
- Silva, A. C. R. D.; Lopes, P. M.; Azevedo, M. M. B. D.; Costa, D. C. M.; Alviano, C. S.; Alviano, D. S. Biological Activities of a-Pinene and β-Pinene Enantiomers. Molecules. 2012, 17(6). DOI: 10.3390/molecules17066305.
- Yu, L.; Yan, J.; Sun, Z. D-Limonene Exhibits Anti-Inflammatory and Antioxidant Properties in an Ulcerative Colitis Rat Model via Regulation of iNOS, COX-2, PGE2 and ERK Signaling Pathways. Mol. Med. Rep. 2017, 15(4), 2339–2346. DOI: 10.3892/mmr.2017.6241.