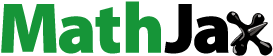
ABSTRACT
Plant polysaccharides exert a wide range of biological effects on the intestinal function. In this study, four polysaccharides containing β-D-Glup, including Dendrobium officinale polysaccharide (DOP), Dendrobium aphyllum polysaccharide (DAP), and glucans from yeast (G-Y) and barley (G-B), were investigated in vitro for their intestinal-promoting functions such as inhibition of digestive enzymes, effect of blood glucose and blood lipid levels by using ultraviolet spectrophotometry, as well as the relationship of their structures to those functions. The results indicated that all polysaccharides significantly slowed glucose diffusion rate, decreased protease, and pancreatic lipase activities. Increased branching degrees had a deleterious effect on the α-amylase activity but increased bile acid binding. Increased calcium concentration in glucans (G-Y and G-B) and increased mannose molar ratio in Dendrobium polysaccharides (DOP and DAP) were correlated with increased pancreatic lipase inhibition. The polysaccharides with higher glucose content and branching degrees had higher bile acid binding and disruption of cholesterol micelle formation. These polysaccharides exerted a variety of potential health-promoting effects attributed to their different structures, which should be further investigated in vivo.
Introduction
Currently, many studies highlighted the remarkable effects of polysaccharides on intestinal functions.[1] For example, Jin found that polysaccharide administration could significantly up-regulate occludin, nuclear factor-κB p65, and secretory immunoglobulin A expression in the ileum.[Citation2] Huanga reported that sage weed polysaccharide extracts might play a role in intestinal health maintenance by reducing the exposure of intestinal mucosa to toxic materials and other harmful compounds.[Citation3] Yang’s results indicated that Lycium barbarum polysaccharides might protect against ischemia-reperfusion injury (IRI)-induced intestinal damage, possibly by inhibiting IRI-induced oxidative stress and inflammation.[Citation4] Red microalga Porphyridium sp. polysaccharides increase the cross-sectional area of the mucosa and muscularis layers in the jejunum and cause hypertrophy in the muscularis layer.[Citation5] Moreover, Dendrobium candidum polysaccharides can regulate the balance of intestinal microbiota and intestinal enzyme activity, optimize the intestinal environment.[Citation6] Polysaccharides can lower blood sugar and cholesterol levels by inhibiting the activity of digestive enzymes.[Citation6]
Dendrobium has been a traditional health-promoting medicine and food source for many decades in Asia.[Citation7] Based on diverse reports, many functional substances have been found in D. officinale, a high proportion being polysaccharides with positive bioactivities, such as antioxidant activities, immunoregulation, and the treatment of Sjogren’s syndrome; while demanding cultivation contributes to a fairly high price in the current market.[Citation8,Citation9] In a previous study, high levels (yield 38.15% ± 0.20) of a novel polysaccharide (named as DAP) in D. aphyllum; and DAP structure was very similar to that of D. officinale polysaccharide (DOP).[Citation10] Meanwhile, the results of our previous comparative analysis of the structure-function relationships of these four polysaccharides during in vitro gastrointestinal digestion highlighted that the spatial structures of carbohydrates changed during gastric digestion. However, their primary structures were destroyed during intestinal digestion.[1] These four polysaccharides (DAP, DOP, G-Y, and G-B) all contain glycosidic bonds that mainly contain β-D-Glcp. In terms of differences, their spatial structures and molecular weights vary, the molar ratio between DOP and DAP is different; and the structure of G-B is 1,4-linked-β-D-Glcp combined with 1,3-linked-β-D-Glcp, whereas G-Y contains 1,3-linked-β-D-Glcp combined with long β-1,6 branches.
Polysaccharides are challenging to be absorbed in the intestinal tract due to their high molecular weight, so they can quickly react with digestive juices and enzymes or be utilized by the intestinal flora.[Citation11] Therefore, the four polysaccharides may lower blood glucose and blood lipid levels in vitro, and their structure-activity relationships were discussed. These plant polysaccharides can affect intestinal solutes and enzymes, and we hypothesized that their structures could influence their specific biological effects. Therefore, the aims of this study were to: a) investigate the in vitro effects of novel polysaccharides DAP, DOP, G-Y, and G-B on intestinal function, including glucose diffusion, digestive enzymes activities, binding effects on bile acids, and cholesterol-micelle disruption; b) characterize the differences between the four polysaccharides on in vitro intestinal functions in order to infer the relationship between their structures and intestinal functions. This experiment can be a reference for further study of digestion in vivo.
Materials and methods
Chemicals and samples
Fresh samples of D. aphyllum (1 kg) were collected from the Good Agricultural Practices farm located in Xishuangbanna, Yunnan province, China. These samples were authenticated by experts from the Department of Life Sciences, South China Agricultural University. DOP (UV ≥ 85%) was obtained from Solarbio (Beijing, China), β-glucans from yeast (G-Y) (BR, > 80%) and barley (G-B) (HPLC, > 95%) were purchased from Guangzhou Qiyun Biotechnology Co., Ltd. (Guangzhou, China) and Sigma (St. Louis, MO, USA), respectively. The structures of those three polysaccharides were characterized and performed in the s-Table1 in the supplementary information. The pancreatic α-amylase solution was purchased from Guangzhou QiYun Biotechnology Co., Ltd. Lipase (pig pancreatic Type II), bile acid mixture (T0750, 7.2 mM), and pig pancreatic enzyme (P1500, 10 mg/mL) were purchased from Sigma. Simvastatin was purchased from Dalian Meilun Biology Technology Co., Ltd. (Dalian, China). All other chemicals and reagents used were of the highest analytical grade commercially available.
Sample preparation
DAP was collected, as described in our previous study.[Citation10] The collection protocol for DAP is as follows: fresh D. aphyllum samples were washed thrice. Next, the sample was washed four times with 700 mL ultrapure water (1:70, w/v) at 85°C for 4.5 h in a water bath. The Sevag method was used to obtain the protein-free solution, which was then concentrated and precipitated by adding two volumes of 95% ethanol at 4°C overnight. The crude DAP (cDAP) was obtained by lyophilization. Sample of cDAP solution was loaded onto a DEAE-Sepharose Fast Flow chromatography column to get the DAPa fraction. DAPa was dissolved in ultrapure water and was loaded onto a Sephadex G-200 chromatography column. DAPa was washed with ultrapure water. Following elution and lyophilization similar to the above method, a total of DAP fraction was obtained. All samples, including DAP, DOP, G-Y, and G-B were stored at −20°C until use.
Determination of viscosity
All samples were measured using a DV2T viscometer (Brookfield Engineering, Middleboro, MA, USA). The viscometer was equipped with a rotor (NO. 63, Brookfield Engineering, Middleboro, MA, USA). Samples in 2.5 mg/mL and 10 mg/mL were determined using the rotor. The rotation speed was set to 200 rpm at 37°C for 1 min. All analyses were carried out in triplicate.
Evaluation of glucose diffusion in glucose–polysaccharide system
The evaluation of glucose diffusion in a glucose–polysaccharide system was determined according to the method of Hu et al.[Citation12] with some modifications. The glucose–polysaccharide system comprised glucose (100 mmol/L) and sample solution (either G-B, G-Y, DOP, or DAP, at three concentrations, 0 mg/mL, 2.5 mg/mL and 10 mg/mL). This solution (5 mL) was placed in dialysis bags with an 8000–14000 Da of molecular at 37°C, pH = 7, then underwent dialysis in deionized water. The glucose content in 0.5 mL of the dialysate was determined after 1, 2, 3, 4, 5, and 6 h. The glucose content was determined by the phenol-sulfuric acid method.[Citation13] All analyses were carried out in triplicate.
The maximum diffusion velocity of glucose (Vmax) was calculated as follows. The experimental data were fitted with a parabolic equation:
where y is the glucose content (mg/mL); x is time (h); and a, b, and c are coefficients. The equation to calculate the diffusion rate (dy/dx) at any time is dy/dx = 2ax + b. When x is close to 0, dy = Vmax = b.
Measurement of enzyme activities
The following activities were determined according to the method of Hu et al. with some modifications.[Citation12]
α-amylase activity
Pancreatic α-amylase solution (0.1 mL) was mixed with 1% potato starch solution (2 mL) and sample solution 0.4 of mL (either G-B, G-Y, DOP, or DAP, at three concentrations, 0mg/mL,2.5 mg/mL and 10 mg/mL) at 37°C for 1 h. The reaction was stopped by adding 2 mL of 0.1 mol/L NaOH. After centrifugation (4800 g, 10 min) (L600, Cence Test Instrument Co., Ltd., Hunan, China), the final glucose content in the supernatant was determined using the dinitrosalicylic acid method.[Citation14] All analyses were carried out in triplicate.
Protease activity
Bovine serum albumin solution (0.1 mL, 1%), 0.5 mL of sample solution (either G-B, G-Y, DOP, or DAP, at three concentrations, 0mg/mL, 2.5 mg/mL and 10 mg/mL), and 0.1 mL pepsin solution (1 mg of pepsin per 1 mL of 0.02 mol/L HCl) were mixed at pH 3.0, 37°C for 30 min. Controls contained deionized water in place of the sample solution. The reaction was stopped by adding 0.9 mL 5 % trifluoroacetic acid followed by centrifugation (4800 g, 10 min) (L600, Cence Test Instrument Co., Ltd., Hunan, China). The residual protein concentration in the supernatant was determined by the Coomassie brilliant blue method.[Citation15] Coomassie brilliant blue G-250 was employed as chromogenic agent to quantify the protein, measuring the absorbance at the wavelength of 595 nm. The digestibility of proteins was measured to determine pepsin activity. All analyses were carried out in triplicate.
Pancreatic lipase activity
Pancreatic lipase activity was determined with soybean oil as a substrate(1 mL), 5 mL phosphate buffer solution (0.1 mol/L, pH 7.2) and 1 mL of sample solution (either G-B, G-Y, DOP, or DAP, at three concentrations, 0mg/mL,2.5 mg/mL and 10 mg/mL), stirred for 10 min at 37°C. Then 1 mL lipase solution (0.71 mg/mL, pig pancreatic Type II) was added. The reaction mixture was slowly stirred at 37°C for 1 h. The reaction was stopped in a 5 min boiling water bath and then centrifuged (4800 g, 10 min) (L600, Cence Test Instrument Co., Ltd., Hunan, China), after being cooled in flowing water. The amount of free fatty acids in the reaction mixture was measured by titration with 0.05 mol/L NaOH and phenolphthalein as an indicator. The activity of pancreatic lipase was determined by measuring the production rate of free fatty acids. All analyses were carried out in triplicate.
Bile acid binding ability
To simulate digesting gastric juice, 0.5 mL of sample solution (either G-B, G-Y, DOP, or DAP, at three concentrations, 0 mg/mL, 2.5 mg/mL, and 10 mg/mL) and 0.25 mL of 0.01 mol/L HCl were placed at 37°C with stirring for 1 h. The reaction was stopped by 0.1 mL of 0.1 mol/L NaOH. Then, 1 mL of bile acid mixture (T0750, 7.2 mM) was added and immersed. 2 mL of pig pancreatic enzyme solution (P1500, 10 mg/mL) was added and the mixture placed at 37°C for 1 h. The mixture was precipitated with 5 volumes of ethanol and centrifuged (4800 g, 10 min) (L600, Cence Test Instrument Co., Ltd., Hunan, China).[Citation12] The supernatant (1 mL) was added to 9 mL 45% (v/v) sulfuric acid and heated in a 70°C water bath for 20 min. Subsequently, the mixture was placed in an ice bath for 5 min, and absorbency measured using a spectrophotometer (TU-1900, Persee Co., Ltd., Beijing, China) at 387 nm.[Citation16] The positive control was simvastatin. The polysaccharides’ ability to bind bile acids was compared with simvastatin (defined as 100). All analyses were carried out in triplicate.
Cholesterol-micelle disruption
A cholesterol-micellar solution containing taurocholate (6.6 mM), lecithin (2.4 mM), cholesterol (0.5 mM) and sodium chloride (132 mM) in a phosphate buffer (15 mM, pH 6.3) was mixed and incubated at 37°C for 1 h. Cholesterol separated from the micelle solution by mixture addition can be precipitated by centrifugation (4800 g, 10 min) (L600, Cence Test Instrument Co., Ltd., Hunan, China). The supernatant was filtered through 0.22 μm Millipore (MilliporeSigma, Burlington, MA, USA).[Citation17] Cholesterol content in the filtrate was determined by the o-phthalaldehyde method.[Citation18] Samples mixed with 90% acetic acid at 1:6 (v/v). 0.1 ml of phthalaldehyde reagent and 2 mL H2SO4 were used as chromogenic agents to determine cholesterol. The absorbance was monitored at a wavelength of 550 nm for 20 min.
Calcium and iron concentrations
10 mg of samples (DOP, DAP, G-B, G-Y) were weighed in a beaker, then moistened with 5 ml water and 8 mL concentrated nitric acid added. The sample was heated and dissolved on a heating plate. After the dissolution finished, 5 mL of double distilled water was added, then it was reheated on the hot plate for 3 to 5 minutes, and the solution fixed in a 25 mL volumetric flask. Calcium and iron concentrations were measured by ICP-OES (NexION 300, Perkin Elmer., MA, USA).
Statistical analysis
Data are expressed as the mean ± standard deviation (SD) of three replicates. Significant differences between means were calculated using Duncan’s multiple-range test with SPSS 17.0 software (IBM, Armonk, NY, USA). p < .05 was considered to indicate statistical significance.
Results and discussion
Viscosity determination
The viscoelastic properties in a dispersed system can be employed to identify the structure of macromolecules, and viscosity is proven to be one of the most important factors influencing the intestinal functions of polysaccharides.[Citation19] Therefore, initially, the viscosity of the four polysaccharides was determined. There was a positive relationship between both particle size and molecular weight with viscosity in DOP and DAP, both polysaccharides with 1, 4-linked-β-D-glucomannan (). G-B and G-Y contain β-D-Glcp with different types of glycosidic bond, they had a positive relationship between molecular weight and viscosity at low concentration (2.5 mg/mL), whereas at relatively high concentration (10 mg/mL), the larger the particle sizes (G-B was 1379 ± 7.78 nm, and G-Y was 609.4 ± 5.62 nm) (S-Table 1), the higher the viscosity (G-B was 3.66 ± 0.12 cP, and G-Y was 1.19 ± 0.12 cP) (). When the concentration increased from 2.5 mg/mL to 10 mg/mL, the viscosity of glucomannose (DAP and DOP) increased, whereas the viscosity of glucans decreased. Electrostatic interactions affect viscosity at high polysaccharide concentration[Citation20], in line with our results. It may be the specific spatial structures that contribute to different dose-dependent effects on viscosity. Based on scanning electron microscopy, DOP appearance is foliated, rock-like with chaps on the surface, and DAP is filiform-shaped; these structures with abundant spaces may result in increased viscosity when concentration increases.[Citation1] In terms of G-B and G-Y, which respectively had a nebula-like shape with surface microgrooves, and an arc-shaped sheet structure, their relatively planar surfaces may lead to decreased viscosity when concentration increases, because the shear force is relatively lower.
Table 1. The viscosity of GB, GY, DOP, and DAP†
Calcium and iron concentrations
Calcium and iron concentrations were determined in the polysaccharides (). DOP contained the highest calcium ion concentration, and G-Y had the highest iron ion concentration. Elemental metals are found in various polysaccharides, for example, calcium ions are in the polysaccharide of the seeds of Plantago asiatica[Citation21], and boron and calcium are in the pectic polysaccharides of higher plant cell walls.[Citation22] Some authors report that the presence of elemental metals affects polysaccharide intestinal function.[Citation23] Calcium ions were found to mainly affect the lipid digestive process[Citation24], in which calcium reacts with liberated free fatty acids using ionic complexation, thereby removing them from the surface of the lipid droplets and preventing them from inhibiting lipase activity.[Citation25] Therefore, we conclude that the different contents of calcium and iron in our four polysaccharides may affect their intestinal functions to some extent.
Table 2. Calcium and iron concentrations of the polysaccharide from G-B, G-Y, DOP, DAP.†
Effects on glucose diffusion and α-amylase activity
Many studies indicate polysaccharides, especially those with high viscosity, can bind and adsorb glucose, leading to relatively low glucose levels in the intestine. In this study, the maximum velocity of glucose diffusion in a glucose-polysaccharide system was evaluated (), and the diffusion rates of glucose with all four polysaccharides at both concentrations were significantly (p < .05) lower than the control; and DOP had the best binding or adsorbing capacity for glucose. The glucose diffusion rate with each polysaccharide was generally in line with polysaccharide viscosity. Glucose can be embedded in a porous network[Citation26]; and porous networks with obvious gaps and large cavities have more significant capillary action, form a larger specific surface area and have better adsorption performance.[Citation27] Therefore, we could conclude that the polysaccharides with abundant cavities and high viscosity may have increased glucose binding or adsorbing activities and therefore, lower glucose diffusion rate.
Table 3. Maximum velocity of glucose diffusion in Glucose-polysaccharide system.†
α-amylase can increase glucose levels.[Citation28] As compared to the control group, DOP and DAP significantly decreased α-amylase activity, and DOP had the most significant effect, indicating that the polysaccharides containing 1,4-β-D-glucomannan were able to lower α-amylase activity. As the concentration of DOP and DAP increased, the viscosity increased, resulting in a decreased α-amylase activity. However, the glucans had different characteristics as compared with the control group, G-Y had no significant (p < .05) effects on α-amylase activity, but G-B at 10 mg/mL could significantly lower (p > 0.05) α-amylase activity (). It was reported that many structure-associated factors, especially the composition of monosaccharides and the uric acid level, can vary the activities of digestive enzymes.[Citation29] The types and quantities of exposed side groups in polysaccharides could be an influence on their interactions with glucose.[Citation30] For example, a larger number of free carboxyl groups has a negative effect on α-amylase activity.[Citation31] Also, because of the expansive phenomenon, the exposure of polar and non-polar groups increased, leading to increased interaction between polysaccharides and glucose molecules.[Citation30] One mechanism for polysaccharides to restrain α-amylase activity may be the binding effect of amylase on polysaccharides.[Citation32] In this study, taking the structure-associated physical properties of the four polysaccharides into consideration, it may be that 1,3 glycosidic bonds and relatively low viscosity had little influence on α-amylase activity, while the branching degree especially had great negative effect on α-amylase activity. Meanwhile, cavities in polysaccharides may facilitate the insertion of α-amylase or amylase into polysaccharides.[Citation26]
Table 4. Effect of the polysaccharide from G-B, G-Y, DOP, and DAP on the activity of α-amylase.†
Effects on protease activities
At all polysaccharide concentrations (2.5 and 10 mg/mL), all four polysaccharide treatment groups had significantly lower protease activity (p < .05) than the control group (). G-Y at low concentration and DOP at high concentration had the strongest protease inhibitory effect. This highlights that these polysaccharides reduce in vitro protein digestibility to some extent, possibly not because of viscosity, but their monosaccharide compositions, that the polysaccharides with a higher mannose content had a weaker inhibitory effect on protease activity. Other polysaccharides also decrease protein digestibility, such as polysaccharides from Astragalus, Lycium barbarum, cortex phellodendri, radix Sophora flavescens, radix codonopsis, and inulin.[Citation33] Some polysaccharides directly inhibit the enzymatic activities of digestive enzymes[Citation34], but the interactions between carboxylic and amino groups on the peptide chains of proteins and the negatively charged groups on polysaccharides can also prevent protein hydrolysis.[Citation35,Citation36] Despite some studies demonstrating the role of viscosity in polysaccharide reduction of protein digestibility[Citation37], in our results, there was little relationship between polysaccharide viscosity and protease activity. Therefore, there may be multiple mechanisms by which polysaccharides affect protease activity, and protein digestibility can be concluded.
Table 5. Effect of the polysaccharide from G-B, G-Y, DOP, DAP on protease activity.†
Effects on pancreatic lipase activity
Pancreatic lipase is responsible for digestion and absorption of triglycerides, and its targeted inhibition is one of the most studied reference methods used to determine the potential of natural products to inhibit dietary fat absorption. Indeed, decrease energy intake from dietary fat through inhibition of this enzyme is an essential strategy adopted to prevent and treat obesity.[Citation38] In this study, pancreatic lipase activity was analyzed after polysaccharides addition, and all polysaccharides significantly (p < .05) decreased pancreatic lipase activity, with inhibitory effects that were proportional to the concentration of polysaccharides (p < .05) (). As we mentioned above, calcium reacts with liberated free fatty acids using ionic complexation, thereby removing them from the surface of the lipid droplets and preventing them from inhibiting the lipase activity, and therefore calcium concentration has a negative dose-dependent inhibitory effect on pancreatic lipase.[Citation39] In this study, two elemental metals were investigated, and in polysaccharides containing β-D-glucans (G-Y and G-B), increased calcium concentration correlated with increased inhibition of pancreatic lipase activity; and there was no evident correlation between both calcium and iron concentration with lipase inhibitory capability in polysaccharides containing 1,4-linked-β-D-Glcmanp (DAP and DOP). However, Hu and co-authors[Citation12,Citation40] suggest that polysaccharide structure, including monosaccharide composition, glycosidic bonds, and anionic groups, can inhibit pancreatic lipase activity, therefore it could be inferred that the molar ratio of 1,4-linked-β-D-Glcmanp can affect the activity of pancreatic lipase, as an increasing percentage of mannose decreased pancreatic lipase activity. It could be thought that further investigation is needed to unveil the relationship between ions concentration and inhibitory effects of polysaccharides with the different structure on pancreatic lipase activity.
Table 6. Effect of the polysaccharide from G-B, G-Y, DOP, DAP on pancreatic lipase activity.†
Effects on the binding ability of bile acids in vitro
It was reported that simvastatin was able to improve bile acid excretion and meanwhile, interrupt cholesterol absorption.[Citation41] Therefore, simvastatin was employed as the positive control group, which was expected to bind a considerable amount of bile acid (). At low concentration (2.5 mg/mL), only glucans (G-Y and G-B) had significantly (p < .05) higher binding of bile acids than the control. While at high concentration (10 mg/mL), both the glucans and DAP had a significant (p < .05) increase in bile acid binding over control. Some authors found that the bile acid binding ability of a polysaccharide is related to their anionic, cationic, physical, and chemical structure.[Citation12,Citation16] For instance, polysaccharides with highly branched heteroxylan and high viscosity readily bind bile acids through molecular association, resulting in health-promoting effects that increased fecal excretion.[Citation21,Citation42] In this study, we observed a weak relationship between viscosity and binding affinity, but a robust correlation between binding ability and the type of glycosidic linkages and monosaccharide composition. In detail, the glucans containing 1,3-β-D-Glup had remarkable binding effects on bile acids, and the polysaccharide with a highly branched 1,6-β-D-Glup structure (G-Y) performed better, which may result from the highly branched glucan backbone enhancing interaction with bile acids.[Citation12] Taking glucose concentration into consideration, it appears a monosaccharide composition with high glucose molar ratio enhanced binding effects. Glucose content in DAP is higher than DOP, and at high concentration, DAP bound bile acids significantly more than control (p < .05), meanwhile both glucans (composed of glucose) had high bile acid binding activity.
Table 7. Bile acids binding abilities of the polysaccharide from G-B, G-Y, DOP, DAP relative to simvastatin.†
Effects on disruption of cholesterol-micelle in vitro
During the digestion of cholesterol in the intestine, cholesterol-micelle formation is key to the absorption of cholesterol.[Citation43] Therefore, the cholesterol-micelle disruption could facilitate the escape of cholesterol from the absorption pathway in the digestive system, and cholesterol could be excreted in the feces, resulting in a lower cholesterol level in the body. Some authors found that the dose, viscosity, and molecular weight of polysaccharides were the important factors to lower the cholesterol level in the blood.[Citation27] In this study, the concentration of unbroken cholesterol-micelle and therefore, characterized the disruption capability of the polysaccharides on cholesterol-micelle were determined. At low concentration (2.5 mg/mL) only glucans significantly (p < .05) decreased unbroken cholesterol-micelle concentration, and G-Y had the highest disruption activity (); at high concentration (10 mg/mL), both glucans and DAP significantly (p < .05) lowered the percentage of cholesterol-micelle. These data were in line with the results of these four polysaccharides binding effects on bile acids, indicating a close correlation between binding bile acids and lowering cholesterol absorption. These tendencies are in line with previous research.[Citation44,Citation45] Meanwhile, it could be inferred that the branching degree and monosaccharide molar ratio also affected the disruption of cholesterol-micelle by polysaccharides; structures with a high branching degree and glucose percentage showed great blocking capability against cholesterol-micelle. It was reported that glucans could reduce the solubility of cholesterol colloids and cause the breakdown of cholesterol[Citation44], and especially that arabinoxylan containing 1,3/1,4-β-D-glucans can lower the content of cholesterol and fat[Citation46]; these results are in line with our data.
Table 8. Effect of the polysaccharide from G-B, G-Y, DOP, DAP on the disruption of cholesterol micelle.†
Discussion
In this study, intestinal functions of four polysaccharides containing β-D-Glup, including polysaccharide purified from D. officinale and D. aphyllum, and glucans isolated from yeast and barley, were investigated in vitro, as well as the relationship between structure and intestinal functions. Viscosity was closely correlated with molecular weight, particle size, and most importantly, cavity content. Besides, calcium and iron were detected in these four carbohydrates, with the highest calcium content in DOP, and the most abundant level of iron in G-Y. Intestinal functions were characterized, and the results indicated that these polysaccharides could significantly improve intestinal functions, including significantly lowering glucose levels, and protease and pancreatic lipase activities. DOP, with high viscosity and abundant cavities, performed the best at lowering glucose diffusion rate and α-amylase activity, and G-Y with branched 1,6-linked-β-D-Glcp showed a promising binding effect on bile acids and had a remarkable blocking capability against cholesterol absorption. In this study, it can be in general concluded that these carbohydrates exert a variety of potential health-promoting effects attributed to their different structures: (a) branching degree negatively affected α-amylase activity; abundant cavity spaces may result in higher viscosity, lowering glucose diffusion rate, and depressing α-amylase activity; (b) there may be multiple ways in which polysaccharides affect protease activity and protein digestibility; (c) calcium concentration in β-D-glucans and the mannose molar ratio in 1,4-linked-β-D-Glcmanp polysaccharides positively correlate with inhibitory effects on pancreatic lipase in a dose-dependent manner; (d) polysaccharide structures with branched Glcp and a higher glucose molar ratio presented excellent binding affinities for bile acids, and lowered intestinal cholesterol absorption. Our hypothesis that these polysaccharides would have structure-associated in vitro intestinal functions was confirmed, and whether they can affect blood sugar and cholesterol in intestinal functions in vivo can develop a further study by using an animal model.
Highlights
Dendrobium spp. polysaccharides reduced glucose diffusion and α-amylase activity
·Barley and yeast glucans inhibited lipase activity and cholesterol absorption
·Functional properties of polysaccharides were related to their molecular structure
·The health-promoting effects of these polysaccharides argue for in vivo studies
Declarations of interest
Authors declare no conflicts of interest to report.
References
- Liu, H.; Gong, F. ; Wei, F. Artificial Simulated Gastrointestinal Digestion of Four Carbohydrates Containing Beta‐D‐1 → 4 Linkages and New GC‐TQ/MS‐MS Method for Characterising Released Monosaccharides. Int. J. Food Sci. Technol. 2018, 53(8), 1992-2005.
- Jin, M.; Zhu, Y.; Shao, D.; Zhao, K.; Xu, C.; Li, Q.; Yang, H.; Huang, Q.; Shi, J. Effects of Polysaccharide from Mycelia of Ganoderma Lucidum on Intestinal Barrier Functions of Rats. Int. J. Biol. Macromol. 2017, 94(Pt A), 1–9.
- Huanga, Y.-L.; Chau, C.-F. Improvement in Intestinal Function of Hamsters as Influenced by Consumption of Polysaccharide-Rich Sage Weed Extracts. Food. Chem. 2012, 133(4), 1618–1623. DOI: 10.1016/j.foodchem.2012.02.062.
- Yang, X.; Bai, H.; Cai, W.; Li, J.; Zhou, Q.; Wang, Y.; Han, J.; Zhu, X.; Dong, M.; Hu, D. Lycium Barbarum Polysaccharides Reduce Intestinal Ischemia/Reperfusion Injuries in Rats. Chem. Biol. Interact. 2013, 204(3), 166–172. DOI: 10.1016/j.cbi.2013.05.010.
- Dvir, I.; Chayoth, R.; Sod-Moriah, U.; Shany, S.; Nyska, A.; Stark, A. H.; Madar, Z.; Arad, S. M. Soluble Polysaccharide and Biomass of Red Microalga Porphyridium Sp. Alter Intestinal Morphology and Reduce Serum Cholesterol in Rats. Br. J. Nutr. 2000, 84(4), 469–476. DOI: 10.1017/S000711450000177X.
- Long, C.X.;He, L.; Guo Y.F. Effects of Dendrobium Candidum Polysaccharide on Immunity,Intestinal Microbiota and Enzyme Activity in Mice with Spleen Deficiency Constipation. Nat. Prod. Res. Dev. 2017,06, 1020-1024.
- Silva, J. A. T. D.; Jin, X.; Dobránszki, J.; Lu, J.; Wang, H.; Zotz, G.; Cardoso, J. C.; Zeng, S. Advances in Dendrobium Molecular Research: Applications in Genetic Variation, Identification and Breeding ☆. Mol. Phylogenet. Evol. 2016, 95, 196–216. Doi:10.1016/j.ympev.2015.10.012.
- Lin, X.; Shaw, P.-C.; Sze, S. C.-W.; Tong, Y.; Zhang, Y. Dendrobium Officinale Polysaccharides Ameliorate the Abnormality of Aquaporin 5, Pro-Inflammatory Cytokines and Inhibit Apoptosis in the Experimental Sjögren’s Syndrome Mice. Int. Immunopharmacol. 2011, 11(12), 2025–2032. DOI: 10.1016/j.intimp.2011.08.014.
- Zhao, D., et al. Structural Characterization, Immune Regulation and Antioxidant Activity of a New Heteropolysaccharide from Cantharellus Cibarius Fr. Int. J. Mol. Med. 2018, 41(5), 2744-2754.
- Liu, H.; Ma, J.; Gong, F. Structural Characterisation and Immunomodulatory Effects of Polysaccharides Isolated from Dendrobium Aphyllum. Int. J. Food Sci. Technol.. 2017, 53(5), 1185-1194.
- Flint, H.; Bayer, E. A.; Rincon, M. T.; Lamed, R.; White, B. A. Polysaccharide Utilization by Gut Bacteria: Potential for New Insights from Genomic Analysis. Nat. Rev. Microbiol. 2008, 6(2), 121–131. DOI: 10.1038/nrmicro1817.
- Hu, J.-L.; Nie, S.-P.; Li, C.; Xie, M.-Y. In Vitro Effects of a Novel Polysaccharide from the Seeds of Plantago Asiatica L. On Intestinal Function. Int. J. Biol. Macromol. 2013, 54(2), 264–269.
- Yu, X.H.; Liu, Z.Y; Yang, J.S. Controlling Quality of Astragalus Polysaccharide Meal by Combined TLC and Phenol-Sulfuric Acid Method. Med. Plant. 2010, (4), 58–61.
- Zhao, H.X.; Yuan, D.; He, Y.M. Quantitative Determination of Panax Japonicus Polysaccharide by DNS Method. Sci. Technol. Food. Ind. 2010, 06, 327-329.
- Yang, J.; Bai, B.; Wang, N. Determination of Protein Content in Reconstituted Tobacco Coating Liquid by Coomassie Brilliant Blue Method. Hubei. Agric. Sci. 2017, 05, 946-947.
- Kahlon, T. S. C. L.;. Woodruff.In Vitro Binding of Bile Acids by Rice Bran, Oat Bran, Barley and β‐Glucan Enriched Barley. Cereal Chem. 2003, 80(3), 260–263.
- Huang, Y. L.; ChowY., C. J.; Tsai, H. Composition, Characteristics, and In-Vitro Physiological Effects of the Water-Soluble Polysaccharides from Cassia Seed. Food Chem. 2012, 134(4), 1967–1972. DOI: 10.1016/j.foodchem.2012.03.127.
- Wen-Hsin, W. U.; LillardC., D. A.; Akoh, C. Direct Saponification: A Simple and Rapid Method for Determination of Total Cholesterol and Fatty Acid Composition of Aquatic Foods. J Food Lipids. 2010, 4(2), 97–107.
- Chen, L.;Gao, L.X.; Huang, Q.H. Viscous and Fermentable Nonstarch Polysaccharides Affect Intestinal Nutrient and Energy Flow and Hindgut Fermentation in Growing Pigs. J. Anim. Sci. 2017, 95(11), 5054–5063.
- Liu, H.; Gao, H.N.; Liu,J.S. Effects of Sodium and Calcium Ions on Viscosity Behavior of Soy Pectic Polysaccharides. Food Sci. 2013, 34(11), 10–12.
- Yin, Y.; Nie, S.-P.; Li, J.; Li, C.; Cui, S. W.; Xie, M.-Y. Mechanism of Interactions between Calcium and Viscous Polysaccharide from the Seeds of Plantago Asiatica L. J. Agric. Food. Chem. 2012, 60(32), 7981–7987. DOI: 10.1021/jf302052t.
- Matoh, T. M.; Kobayashi, M. Kobayashi.Boron and Calcium, Essential Inorganic Constituents of Pectic Polysaccharides in Higher Plant Cell Walls. J. Plant. Res. 1998, 111(1), 179–190.
- Hur, S. J.; Lim, B. O.; Decker, E. A.; McClements, D. J. In Vitro Human Digestion Models for Food Applications. Food. Chem. 2011, 125(1), 1–12. DOI: 10.1016/j.foodchem.2010.08.036.
- Zangenberg, N. H.; Müllertz, A.; Kristensen, H. G.; Hovgaard, L. A Dynamic in Vitro Lipolysis Model. I. Controlling the Rate of Lipolysis by Continuous Addition of Calcium. Eur. J. Pharm. Sci. 2001, 14(2), 115–122. DOI: 10.1016/S0928-0987(01)00169-5.
- Fatouros, D. G.; Mullertz, A. In Vitro Lipid Digestion Models in Design of Drug Delivery Systems for Enhancing Oral Bioavailability. Expert. Opin. Drug. Metab. Toxicol. 2007, 4(1), 65.
- Xie, F.; Wang, Y.; Wu, J.; Wang, Z. Insoluble Dietary Fibers from Angelica Keiskei By-Product and Their Functional and Morphological Properties: Functional and Morphological Properties of Insoluble Dietary Fibers. Starch. Stärke. 2017, 69, 1600122. Doi:10.1002/star.201600122.
- Zhu, Y.; Chu, J.; Lu, Z.; Lv, F.; Bie, X.; Zhang, C.; Zhao, H. Physicochemical and Functional Properties of Dietary Fiber from Foxtail Millet (Setaria Italic) Bran. J. Cereal. Sci. 2018, 79, 456–461. DOI: 10.1016/j.jcs.2017.12.011.
- Kobayashi, K.; Ishihara, T.; Khono, E.; Miyase, T.; Yoshizaki, F. Constituents of Stem Bark of Callistemon Rigidus Showing Inhibitory Effects on Mouse α-Amylase Activity. Biol. Pharm. Bull. 2006, 29(6), 1275–1277. DOI: 10.1248/bpb.29.1275.
- Wang, L.; Liu, F.; Wang, A.; Yu, Z.; Xu, Y.; Yang, Y. Purification, Characterization and Bioactivity Determination of a Novel Polysaccharide from Pumpkin (Cucurbita Moschata) Seeds. Food. Hydrocolloids. 2017, 66, 357–364. DOI: 10.1016/j.foodhyd.2016.12.003.
- Yu, G.; Bei, J.; Zhao, J.; Li, Q.; Cheng, C. Modification of Carrot (Daucus Carota Linn. Var. Sativa Hoffm.) Pomace Insoluble Dietary Fiber with Complex Enzyme Method, Ultrafine Comminution, and High Hydrostatic Pressure. Food. Chem. 2018, 257, 333–340. DOI: 10.1016/j.foodchem.2018.03.037.
- Gourgue, M. P.; Champ, M. M. J.; Lozano, Y.; Delort-Laval, J. Dietary Fiber from Mango Byproducts: Characterization and Hypoglycemic Effects Determined by in Vitro Methods. J. Agric. Food. Chemi. 1992, 40(10), 1864–1868. DOI: 10.1021/jf00022a027.
- Annison, G. D. L.; Topping, D. L. Topping.Nutritional Role of Resistant Starch: Chemical Structure Vs Physiological Function. Annu. Rev. Nutr. 1994, 14(1), 297–320. DOI: 10.1146/annurev.nu.14.070194.001501.
- Fan, Y.; Yu, X.Q.; Li, L. Effect of Different Immunostimulants on Digestive Enzyme Activities and Histological Structure in Intestine of Sea Cucumber, Apostichopus Japonicus. Chin J Fish. 2014, 04, 46-51.
- Sukhum, P.; JiraratM, T. Chirakarn.Isolation and Rheological Properties of Tamarind Seed Polysaccharide from Tamarind Kernel Powder Using Protease Enzyme and High-Intensity Ultrasound. J. Food. Sci. 2010, 75(5), E253–E260.
- Girard, M.; TurgeonS., S. L.; Gauthier, F. Interbiopolymer Complexing between β-lactoglobulin and Low- and High-Methylated Pectin Measured by Potentiometric Titration and Ultrafiltration. Food Hydrocolloids. 2002, 16(6), 585–591. DOI: 10.1016/S0268-005X(02)00020-6.
- Mouécoucou, J.; Villaume, C.; Sanchez, C.; Méjean, L. Effects of Gum Arabic, Low Methoxy Pectin and Xylan on in Vitro Digestibility of Peanut Protein. Food Res. Int. 2004, 37(8), 777–783. DOI: 10.1016/j.foodres.2004.04.002.
- Edwards, C. A.; Johnson, I. T.; Read, N. W. Do Viscous Polysaccharides Slow Absorption by Inhibiting Diffusion or Convection? Eur. J. Clin. Nutr. 1988, 42(4), 307–312.
- Kim, G.N., et al. Study of Antiobesity Effect through Inhibition of Pancreatic Lipase Activity ofDiospyros kakiFruit andCitrus unshiuPeel. Biomed Res. In. 2016, 2016(7), 1–7.
- Li, Y.; Hu, M.; Du, Y.; Xiao, H.; McClements, D. J. Control of Lipase Digestibility of Emulsified Lipids by Encapsulation within Calcium Alginate Beads. Food Hydrocolloids. 2011, 25(1), 122–130. DOI: 10.1016/j.foodhyd.2010.06.003.
- Hu, M.; Li, Y.; Decker, E. A.; McClements, D. J. Role of Calcium and Calcium-Binding Agents on the Lipase Digestibility of Emulsified Lipids Using an in Vitro Digestion Model. Food Hydrocolloids. 2010, 24(8), 719–725. DOI: 10.1016/j.foodhyd.2010.03.010.
- Hoving, L. R.; Katiraei, S.; Heijink, M.; Pronk, A.; van der Wee-Pals, L.; Streefland, T.; Giera, M.; Willems van Dijk, K.; van Harmelen, V. Dietary Mannan Oligosaccharides Modulate Gut Microbiota, Increase Fecal Bile Acid Excretion, and Decrease Plasma Cholesterol and Atherosclerosis Development. Mol. Nutr. Food. Res. 2018, 62(10), e1700942. DOI: 10.1002/mnfr.201700942.
- Anderson, J. W.; Siesel, A. E. Hypocholesterolemic Effects of Oat Products; Springer: US, 1990; pp 17.
- Raederstorff, D. G.; Schlachter, M. F.; Elste, V.; Weber, P. Effect of EGCG on Lipid Absorption and Plasma Lipid Levels in Rats. J. Nutr. Biochem. 2003, 14(6), 326–332. DOI: 10.1016/S0955-2863(03)00054-8.
- Sawiris, P. G.; Enwonwu, C. O. Ascorbate Deficiency Impairs the Muscarinic-Cholinergic and Ss-Adrenergic Receptor Signaling Systems in the Guinea Pig Submandibular Salivary Gland. J. Nutr. 2000, 130(12), 2876–2882. DOI: 10.1093/jn/130.12.2876.
- Carr, T. P.; Wood, K. J.; Hassel, C. A.; Bahl, R.; Gallaher, D. D. Raising Intestinal Contents Viscosity Leads to Greater Excretion of Neutral Steroids but Not Bile Acids in Hamsters and Rats. Nutr. Res. 2003, 23(1), 91–102. DOI: 10.1016/S0271-5317(02)00476-1.
- Devi, P. B.; Vijayabharathi, R.; Sathyabama, S.; Malleshi, N. G.; Priyadarisini, V. B. Health Benefits of Finger Millet (Eleusine Coracana L.) Polyphenols and Dietary Fiber: A Review. J. Food Sci. Technol. 2014, 51(6), 1021–1040. DOI: 10.1007/s13197-011-0584-9.