ABSTRACT
The physicochemical properties, shelf life and stability of pitaya pearls (PP) by reverse spherification from sodium alginate (PP Alginate), kappa carrageenan (PP Kappa) and a combined iota carrageenan-sodium alginate (PP Iota) were studied. The macronutrients of PP were not affected by the hydrocolloid types, except for ash. PP Alginate showed the strongest textural properties. Storage duration affected mainly elasticity of all PP. During storage, PP Alginate and PP Kappa had better morphological and textural stability. However, PP Kappa with lower values of calorie, hardness, and rupture force is the suggested PP for consumption in beverages.Abbreviations: ANOVA: Analysis of variance; AOAC: Association of Official Analytical Chemists; GLM: General Linear Model; PP: Pitaya pearl
Introduction
Tapioca pearls are small spheres (1–6 mm diameter) made from tapioca starch with a shiny surface and elastic texture.[Citation1] Produced mainly in Thailand, Indonesia and Malaysia, tapioca pearls increase the calorie in sweet drinks. The total calorie of milk tea containing tapioca pearl served in a small cup (473 mL) was 299 kcal as compared to milk tea alone (263 kcal).[Citation2] Multiple high-calorie intakes may contribute to obesity and diabetes.[Citation3] Besides, maleic acid which is a prohibited ingredient in food due to its toxicity effect[Citation4], is incorporated into tapioca starch to increase the elasticity.[Citation5] Therefore, healthier pearl ingredients such as pitaya and hydrocolloids are a good alternative to overcome the problems.
Pitaya (Hylocereus polyrhizus) or red dragon fruit originated from Mexico, Central, and South America.[Citation6,Citation7] It is planted in Thailand, Vietnam, and Malaysia. The fruit is firm, juicy, sweet and has an intense deep red-purple color caused by an antioxidant called the betalains. Pitaya contains high fiber, minerals, vitamin C and vitamin A.[Citation8,Citation9] The carbohydrate in pitaya consists of glucose (401 g/kg), fructose (158 g/kg) and oligosaccharides (89.6 g/kg), where the mixture of oligosaccharides in pitaya stimulates prebiotic activities.[Citation10] Pitaya also exhibits high antioxidant (betacyanins and betalanins) and antimicrobial activities.[Citation11] Pitaya has a fleshy-type texture that is suitable to produce puree or juice, fermented liquid, and wine.[Citation12] The flesh could be mixed with milk, soft drink, ice cream, jellies, and marmalade.[Citation13] A few researchers used pitaya as a food ingredient because of its natural red color and good antioxidant activity.[Citation14–Citation16] Due to its distinct color, taste, nutritional benefit and flavor acceptability, pitaya has the potential to provide good characteristics as fruit pearl’s core by using reverse spherification method.
Spherification is used to encapsulate ingredients with bad flavor, deliver active compound in drugs and ingredients in pharmaceuticals, immobilize enzyme and to protect active compound from the environmental threat.[Citation17] This technique is used to coat liquid material using polymer film by forming a sphere with a liquid core.[Citation18] Reverse spherification technique provides a better shape of pearl which requires a mixture of cation and targeted ingredient to be injected into the ionic biopolymer solution. One of the critical properties of pearl by spherification is to be able to have the sensation of flavor burst in the mouth when the mouth cavity releases light pressure. The sphere should burst readily and provide the explosion of flavor or element inside the sphere.[Citation17] The commonly used materials for spherification technique are edible biopolymers, namely proteins and hydrocolloids.
Alginate, a hydrocolloid, is extracted from brown seaweeds and is a common material used for material encapsulation, hydrogels, and film gels. It is composed of 1–4 linked α-L-guluronic and β-D-mannoronic acid residue.[Citation19] Rigid hydrogel beads can be formed by simple gelation with divalent cation with higher affinity to Ca2+ and result in the egg-box formation.[Citation20] Alginate has broad biological and food applications due to its biodegradability, biocompatibility, non-toxic and is reasonably cheap.[Citation21,Citation22] However, alginate hydrogels had poor mechanical properties and porous structure which are decisive for food or drug delivery system.[Citation22] To enhance the gel properties, sodium alginate is commonly combined with other polysaccharides, for instance, guar gum, chitosan[Citation23,Citation24] or carrageenan.[Citation25,Citation26]
Carrageenan is a polysaccharide from certain red seaweed (rhodophyta) species, for instance, rhodophyceae, furcellariacaea, gigartinaceae, hypnaeceae, phyllophoraseae, and solieriaceae.[Citation27,Citation28] Kappa and iota carrageenan are commonly used as a gelling agent that have an affinity toward K+ and Ca2+, respectively. Both carrageenans forms a thermoreversible ordered helical structure during gelation.[Citation29] Kappa and iota carrageenan can form a film in spherification due to its gelling properties.[Citation30] A film formed from carrageenan and alginate mixture had increased moisture barrier and tensile properties than alginate alone.[Citation31] Furthermore, the combination of carrageenan and alginate as polymer microspheres had a lower bursting ability and had increased protection of internal material in acidic condition.[Citation32] The only study found on the usage of the carrageenan-alginate mixture in food is the development of encapsulated caffeine.[Citation33] Other studies in carrageenan-alginate encapsulation were in the pharmaceuticals field.[Citation25,Citation26,Citation34]
Many researchers have focused on the undesirable health effects of consuming sweetened beverages containing tapioca pearl.[Citation2,Citation35,Citation36] However, these studies were only limited to characterizing the nutritional properties and its adverse effects on health, without offering any alternative solutions. Hence, it is essential to find a healthier alternative for tapioca pearls to improve the nutritional properties of beverages. In this study, the development of pitaya pearls by reverse spherification by using carrageenan or combination of alginate-carrageenan are expected to have improved nutritional, morphological and physical properties. The objective of this study was to study the physicochemical properties of developed pitaya pearls by using reverse spherification method using alginate, kappa carrageenan and a combination of alginate-iota carrageenan. The shelf life and stability of pitaya pearls were also evaluated.
Methods and materials
Materials
Ripe red pitaya fruits were obtained from the local market in Sri Serdang, Selangor. Materials used to produce the pearls were sodium alginate (R&M), calcium chloride (BisChem), kappa carrageenan (TA150, Tacara) and iota carrageenan (TS150, Tacara). All chemicals used were food grade.
Preparation of pitaya pearl
shows the formulation of pitaya pearls (PP) using different hydrocolloids.
Table 1. Formulation of pitaya pearls by using different hydrocolloids
Preparation of pitaya puree
Pitaya fruit was peeled and cut into small chunks and fed into juice extractor (MJ-70M Juicer, Panasonic, Japan) to remove the seeds. The obtained puree was placed in a beaker, heated at 60°C for 30 min in a water bath (ThermoBath, Germany) and cooled in an ice bath. The chilled puree was then kept in a plastic container and stored at −18°C[Citation37] until further use.
Preparation of pearl solution
Frozen pitaya puree was thawed by leaving it at room temperature (25°C) for 30 min. The puree (500.0 g) was mixed with calcium chloride (4.0 g) using a hand blender (Tefal HB853866/700–0315-R, Japan). Then, the pearl solution was transferred into a 250 mL squeezable bottle and left to rest at room temperature (25°C) for 30 min prior to reverse spherification procedure.
Preparation of bath solution
Three formulations of hydrocolloid (sodium alginate, kappa carrageenan and a combination of iota carrageenan-sodium alginate) were used to prepare the bath solution as in . Bath solution was freshly prepared by mixing 3.0 or 5.0 g hydrocolloid with distilled water (100 mL) using a high-speed hand blender (Tefal HB853866/700–0315-R, Japan). The amount of hydrocolloid was determined based on preliminary study (not reported). The mixture was warmed and kept at 75°C for 2 min before transferred into a beaker.
Preparation of PP by reverse spherification
A 250 mL polypropylene squeezable bottle with a mouth dropper (internal diameter of 0.46 mm) (Toyogo, Malaysia), was used to extrude droplet by inverting the bottle using retort clamp. The distance of the mouth dropper to the bath solution surface was 3 cm. The prepared puree solution was dropped from the bottle into the bath solution and left for 5 min. The PPs formed were collected and rinsed in distilled water by swirling thrice using a perforated spoon. Then, the pearls were kept in an airtight container that was then placed in a chiller at 4°C for further analysis and storage study. Other than storage study, all analyses were carried out on fresh samples.
Chemical analysis and calorie
Determination of protein, fat, ash and moisture were determined based on AOAC Method 990.03, AOAC Method 920.39., AOAC Method 942.05, and AOAC Method 934.01, respectively. The calorie content of all the PP was determined according to the equation of the chemical compositions.[Citation38]
Physical analysis
Size
The PPs were dabbed with tissue to remove excess water and measured individually by using venire caliper (Mitutoyo, Japan). The measurement was done in triplicates.
Instrumental texture analysis
One pearl from each formulation was used for the texture profile measurement. Three replicates for each sample were randomly selected for the tests and placed on a compression plate. A small flat metal ring was used at the center of the plate to steadily hold the round sample. Samples were tested for texture profile analysis and burst-ability test using texture analyzer (TPA; Stable Micro Analyzer TA, XT2i, United Kingdom). A P36R cylinder probe was attached to 5 kg load cell and compression was set to 75% of its original height with a force of 5.0 g. The pre-test speed, test speed, and post-test speed was set at 1, 5 and 5 mm/sec, respectively. Properties measured were hardness, cohesiveness, springiness, and gumminess. The bursting force was determined using the similar parameter as texture profile analysis except the compression was set at 50% with test speed at 2 mm/sec and post-test speed at 10 mm/sec. The bursting force was applied to evaluate the rupture force and elasticity.
Colour
Colour analysis was performed on a single pearl for each formulation using a Hunter Lab spectrophotometer (UltraScan Pro, HunterLab, USA). Three replicates for each sample were randomly selected for the tests. The mode used was Reflectance Specular Excluded that was specifically for semi-solid sample with glossy properties. The standard color scale used was CIELAB with parameter L* (lightness/darkness), a* (+ a = redness, – a = greenness) and b* (+ b = yellowness, – b = blueness).
Storage study
For bursting force (rupture force and elasticity), all samples were collected for 5 (Day 0, 1, 2, 3, 4) consecutive days at 4 ± 1°C. The duration of storage was determined from a preliminary test (not reported) which showed that the pearls turned sour at a chilled temperature (4 ± 1°C) on day 5. Each sample was kept in a round polypropylene container (115 mL) and stored in a larger squared HDPE container (8 L) under two storage conditions which were chilled (4 ± 1°C) and frozen (−24 ± 5°C). Samples were thawed to room temperature and tested for rupture force and elasticity. For moisture, the samples were collected on day 0, 2 and 4; thawed to room temperature (25°C) and tested.
Morphological properties
The PPs at day 0 and 4 were stored at −24°C for 1 day and freeze-dried in a freeze dryer (77550-01H, Labconco, USA) for 2 days. The morphological properties of PPs at day 0 was observed using a digital microscope (Eclipse 80i, Nikon, Japan) at 40, 200 and 400 × magnifications. The PPs at day 4 during storage study were observed under a scanning electron microscope (S-3400N Hitachi, Japan). A single freeze-dried pearl was fixed to a holder and spurred with gold-palladium layer and viewed at 30 and 300 × magnifications.
Statistical analysis
Except for storage study, all data were analyzed by one-way ANOVA. Storage study data were analyzed using the general linear model (GLM). GLM on rupture force and moisture were carried out on 3 × 2 × 5 (formulation, temperature, duration) and 3 × 2 × 3 (formulation, temperature, duration) factorial designs, respectively. The data were expressed as mean ± standards deviation. The significant difference between the means of sample attributes was p ≤ 0.05. The means were expressed in triplicates unless stated otherwise.
Results and discussion
Nutritional compositions and calorie of PP
showed moisture, protein, fat, and ash of PP. Except for ash, no statistical differences were observed on all compositions of different hydrocolloids used for the production of PPs. The insignificant result showed that the nutritional compositions, especially fat were not the contributing factor to the calorie and the textural properties. Ash in PP Alginate (0.82%) was significantly higher by two times than PP Kappa (0.35%) and PP Iota (0.31%), indicating higher amounts of minerals. This is believed to occur due to the extracted alginate from the brown seaweed had higher ash content than the carrageenan extracted from the red seaweed.[Citation39] However, the result was in contradictory with Mohammadi et al.[Citation40] where ash was higher in the red seaweed as compared to the brown seaweed. The difference was probably contributed by separated geographical locations where Krisnaiah et al.[Citation39] collected seaweeds from the South China Sea, whereas Mohammadi et al.[Citation40] collected seaweeds from the Northern Persian Gulf. In terms of calorie, PP Kappa had the lowest (12.86 kcal), followed by PP Iota (15.35 kcal) and PP alginate (17.64 kcal/100 g). The calories were two times lower than the tapioca pearls (36 kcal) in the study reported by Min et al.[Citation2] proving a healthier option than the tapioca pearls.
Table 2. Nutritional compositions and calorie of pitaya pearls
Size and textural properties of PP
shows the size and texture profiles of PP. The size of PP Iota was 37% and 33% higher than PP Alginate and PP Kappa, respectively. When in contact with droplets containing pitaya puree-CaCl2, the high viscosity of bath solution increased the thickness and stickiness characteristics of PP Iota. Consequently, the size of PP Iota became bigger. This gelation process improves the cross-linking activity with calcium to form a gel.[Citation41] Istini et al.[Citation42] reported the viscosity of kappa was lower (68.17–246 cp) as compared to iota (147.7–790 cp) that may have produced smaller size of PP.
Table 3. Size and texture profiles of pitaya pearls
Desired textural properties of pearls in drinks may vary according to consumer’s preference but should be soft enough to be burst with optimal chewing sensation. In the study, bursting properties consist of rupture force and elasticity. PP Alginate had higher hardness, cohesiveness, and gumminess than PP Kappa and PP Iota. However, the springiness of PP Kappa was two times higher than other samples. In terms of elasticity, PP Iota had a significantly lower value. The low elasticity was mostly due to thicker gel coating (), which will be further discussed in the morphological structure of PP.
Figure 1. Microstructure of pitaya pearls (PP) at day 0 under a digital microscope at 40, 200 and 400 × magnifications
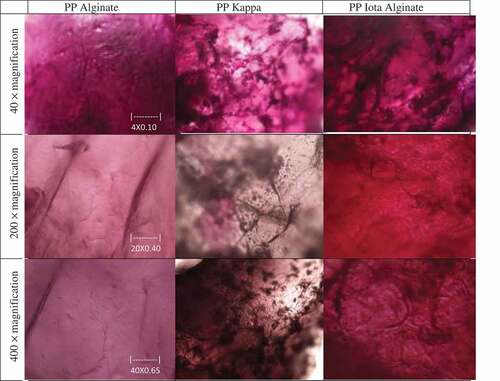
Hardness indicates the stability of the pearl from bursting during processing and storage (Tsai et al., 2017). The hardness of PP Alginate was 42% higher as compared to PP Kappa and PP Iota. Hardness was positively correlated with cohesiveness and gumminess since gumminess was a result of hardness × cohesiveness. PP Alginate had significantly higher cohesiveness and gumminess by 1.5 times and 2.6 times, respectively, than the other two samples. The possible reason was that alginate gel gave firmer PP encapsulation due to G-block of alginate (alginate oligosaccharide) that performed coordination with calcium ions better than other two hydrocolloids.[Citation17] In comparison, the hardness of all PP in this study was relatively higher as compared to liquid core hydrogel bead from alginate produced by Tsai et al.[Citation17], most likely due to the bigger size of all PPs by 10 times.
The springiness of PP Kappa was almost double the value than the other two hydrocolloids. This result was probably due to kappa carrageenan having a lower affinity to Ca2+ which weakened the gelation formed. Kappa carrageenan forms a firmer gel in the presence of K+.[Citation43] Rupture force required to break PP was significantly higher for PP Iota (35% and 68% higher than PP Alginate and PP Kappa, respectively). In contrast, the elasticity of PP Iota was significantly lower than the other PP. Low elasticity and high force rupture had explained the resolving texture of the PP Iota after biting during the preliminary study of sensory evaluation (not reported in the study).
Overall, PP Kappa had the best textural properties as compared to the other formulations. The low hardness, cohesiveness and rupture force with high springiness showed the suitability of PP Kappa to be burst and chewed at the optimum textural sensations without melting too fast in the mouth or portraying a chewing gum-like texture. The high hardness, cohesiveness, and gumminess of PP Alginate may not provide the best textural sensation of PP. Although PP Iota had a few similar textural properties as PP Kappa, the low elasticity and springiness were not desirable traits of PP. The bursting properties, moisture content () and microstructure () instabilities of PP Iota during four days of storage further supported the unsuitability of PP Iota Alginate as the gel coating of PP.
Colour of PP
The average color value (L*, a*, b*) characteristics of PPs are given in . The L* value of PP was not affected by the different hydrocolloids used for reverse spherification. The a* of PP Kappa was 1.7–1.8 times lower than PP Alginate and PP Iota. Although b* values of PP Alginate was lesser and PP Kappa was higher than PP Iota, both were not significantly different from b* value of PP Iota. These differences in a* and b* indicated the refraction of ultraviolet color during gel formation due to the pigment present in the red pitaya that contributed to red-purple color, consequently being responsible for a* and b* chroma.[Citation44]
Table 4. Colour attributes of pitaya pearls
Table 5. Storage variables (temperature, duration, and formula) effect on rupture force, elasticity and moisture content of pitaya pearls
Storage effect on rupture force, elasticity and moisture content
shows the interaction of the main effect of variables (temperature, duration, and formula) and interacted effect between all the variables for rupture force, elasticity and moisture during storage by using GLM. Storage duration (main effect) and all variables in the interacted effect gave an impact to all parameters. Based on all parameters, the optimum conditions during storage were at 4°C (temperature) for 2 days (duration).
The main effect for rupture force was significant for temperature and duration. Lower temperature and final day of storage had significantly higher rupture force. The temperature at −24°C and longer storage duration may have caused moisture leaching that caused shrinkage of PP, consequently increasing the rupture force. There was a significant interaction effect observed between hydrocolloid formula and duration as well as interactions between all variables with R2 of 68.08%.
The elasticity of pearls was all affected by main effect and each of their interacted effects. The elasticity of all PP stored at −24°C significantly reduced as compared to 4°C condition, most likely due to water expulsion from the gel at a lower temperature. The main effect of the formula was in agreement with the elasticity measurement () where PP Alginate had the highest value, followed by PP Kappa and PP Iota. In addition, the longer duration of storage caused higher elasticity. This result can be explained by possible cross-linking of gel during storage that enhanced the hydrocolloid’s molecular interaction, consequently increasing the elasticity. The R2 of elasticity for all interaction effect for all variables was 82.30%, which indicated a well-fitted model.
Moisture content was only being affected by storage duration where a significant decrease was observed on day 4. This result was expected because moisture loss occurrence is common during storage that may have affected the other variables. Although insignificant, the moisture content of PP Iota was lower than other PP formula. For moisture, all variables had interacted effects with R2 of 58.02%.
Morphology structure of PP
The digital microscope photos of PPs on day 0 are presented in and morphology of PPs after 4 days of storage by SEM is presented in . Generally, PP Alginate had the smoothest surface morphology, followed by PP Kappa and PP Iota. PP Alginate had dense and fine lines that indicated small folds. However, the surface was smooth, consistent and showed a homogenous thickness probably due to sturdy gelation. This factor contributed to the high hardness, cohesiveness, gumminess, and elasticity (). The good elasticity and moisture retention during storage were also reflected by its maintained smooth surface and minimal shrinkage at day four (). This result is supported by Xu et al.[Citation45] where a membrane film developed from alginate showed a lower degree of roughness.
At day 0, PP Iota appeared thicker, less smooth and had more folds and wrinkles than PP Alginate (). The structure probably resulted from the interaction of sodium alginate and iota carrageenan that formed a gel with higher cross-linking, consequently increasing the folds and unevenness. When the membrane was replaced with an increased amount of carrageenan, holes appeared on the surface[Citation45], which was more evident after 4 days of storage (). PP Iota had a more intense red color with a thicker gel coating. The thicker coating may be the cause of significantly higher rupture force and lower elasticity (). After storage, PP Iota had a rougher surface, cracks and was porous (), which correlates with the low retention of moisture content during storage (). The heterogeneous structure of PP Iota may cause brittleness, which probably was caused by the expulsion of water during storage at frozen temperature.[Citation45] This structural surface was related to the severe loss of moisture after storage at −24°C for 4 days as discussed in the interaction of all variables for moisture content during storage.
PP Kappa at day 0 had more folds and thinner structure than the other PPs (), which may explain the significantly lower rupture force (). PP Kappa also had a lighter red color, but appeared to be more blue purplish in hue than other PP’s, which was in proportion with its a* and b* values (). The blue hue was more evident probably due to the thinner membrane of PP Kappa. Although PP Kappa appeared less uniform than PP Alginate after storage (), the morphological structure appeared consistently homogenous in terms of smoothness and was not as degraded as PP Iota.
Conclusion
Except for ash, different hydrocolloids used in the formulation did not affect the macro nutritional composition of PPs. However, PP Kappa showed the best performance in the textural properties with intermediate values which was suitable for optimum textural sensation in mouth, which can be confirmed in sensory test as a future analysis. PP Kappa also had lower calorie, optimum elasticity and acceptable morphological structure stability in the storage study. Storage study showed that interacted effect of variables (formula, duration, and temperature) prominently affected elasticity compared to rupture force and moisture of PPs. For better textural properties, it is suggested that PPs produced from reverse spherification method is best to be stored up to 2 days at 4°C. Further studies on the stability of pitaya’s antioxidant and nutritional properties during storage should be considered. Additionally, stability of PPs in different beverage temperatures and its effect on the viscosity of beverages should be studied too. PPs formed by reverse spherification method was able to encapsulate the pitaya puree and has the potential of replacing the unhealthy tapioca pearls. The study showed a promising potential of kappa carrageenan as pearls gelling agent for pitaya puree filling in dessert and beverage industries.
Acknowledgments
We thank the Faculty of Food Science and Technology and Institute of Bioscience, both located in Universiti Putra Malaysia for funding and usage of equipment.
References
- Xu, J. B.; Bartley, J. P.; Johnson, R. A. Preparation and Characterization of Alginate-carreegenan Hydrogel Films Crosslinked Using a Water-soluble Carbodimide (WSC). J. Membr. Sci. 2003, 218, 131–136. DOI: 10.1016/S0376-7388(03)00165-0.
- Min, J. E.; Green, D. B.; Kim, L. Calories and Sugars in Boba Milk Tea: Implications for Obesity Risk in Asian Pacific Islanders. Food Sci. Nutr. 2016, 5, 38–45. DOI: 10.1002/fsn3.362.
- Malik, V. S.; Popkin, B. M.; Bray, G. A.; Despres, J. P.; Hu, F. B. Sugar-sweetened Beverages, Obesity, Type 2 Diabetes Mellitus and Cardiovascular Disease Risk. Circulation. 2010, 121, 1356–2364. DOI: 10.1161/CIRCULATIONAHA.109.876185.
- Wong, P. K.; (2013) Local Bubble Tea Shops Assure Customers on Safety with Lab Test Results. The Star online web. https://www.thestar.com.my/news/nation/2013/05/31/our-pearls-safe-to-consume-local-bubble-tea-shops-assure-customers-with-lab-test-results/ (accessed Nov 20, 2017).
- Fu, H. Y.; Li, H. D.; Xu, L.; Yin, Q. B.; Yang, T. M.; Ni, C.; Cai, C. B.; Yang, J.; She, Y. B. Detection of Unexpected Frauds: Screening and Quantification of Maleic in Cassava Starch by Fourier Transform Near-infrared Spectroscopy. Food Chem. 2017, 227, 322–328. DOI: 10.1016/j.foodchem.2017.01.096.
- Benzing, D. H.;. Vascular Epiphytes, General Biology and Related Biodata; Cambridge University Press: Cambridge, 1990.
- Haber, W. A.;. Hylocereus Costaricensis (Pitahaya Silvestre), Wild Pitahaya. In Costarican Natural History; Janzen, D. H., Ed.; University of Chicago Press: Chicago, 1983; pp 252–253).
- Jaafar, A.; Nazri, M.; Khairuddin, W. Proximate Analysis of Dragon Fruit (hylecereus Polyhizus). Am. J. Appl. Sci. 2009, 6, 1341–1346. DOI: 10.3844/ajassp.2009.1341.1346.
- Nurul, S. R.; Asmah, R. Variability in Nutritional Composition and Phytochemical Properties of Red Pitaya (hylocereus Polyrhizus) from Malaysia and Australia. Int. Food Res. J. 2014, 21, 1689–1697.
- Wichienchot, S.; Jatupornpipat, M.; Rastali, R. A. Oligosaccharides of Pitaya (dragon Fruit) Flesh and Their Prebiotic Properties. Food Chem. 2010, 120, 850–857. DOI: 10.1016/j.foodchem.2009.11.026.
- Tenore, G. C.; Novellino, E.; Basile, A. Nutraceutical Potential and Antioxidant Benefits of Red Pitaya (hylocereus Polyrhizus) Extracts. J. Funct. Foods. 2012, 4, 129–134. DOI: 10.1016/j.jff.2011.09.003.
- Minh, N. P.;. Various Factors Influencing to Red Dragon Fruit (hylocereus Polyrhizus) Wine Fermentation. Int. J. Multidiscip. Res. Dev. 2014, 1, 94–98.
- Luders, L.; McMahon, G. The Pitaya or Dragon Fruit (hylocereus Undatus). In Crops. Forestry and Horticulture, Darwin, Department of Primary Industry, Fisheries and Mines, Northern Territory Government, Australia, 2006; pp 1–4.
- Tze, N. G.; Han, C. P.; Yusof, Y. A.; Ling, C. N.; Talib, R.; Taip, S. F.; Aziz, M. G. Physicochemical and Nutritional Properties of Spray Dried Pitaya Fruit Powder as Natural Colorant. Food Sci. Biotechnol. 2012, 21, 675–682. DOI: 10.1007/s10068-012-0088-z.
- Moshfeghi, N.; Mahdavi, O.; Shahhosseini, F.; Malekifar, S.; Taghizadeh, S. K. Introducing a New Natural Product from Dragon Fruit into the Market. Int. J. Res. Rev. Appl. Sci. 2013, 15, 269–272.
- Safura, S.; Hasanah, M. G.; Syed Hamed, M.; Kharidah, M. Stability of Betanin in Pitaya Powder and Confection as Affected by Resistant Maltodextrin. LWT Food Sci. Technol. 2017, 84, 129–134. DOI: 10.1016/j.lwt.2017.05.031.
- Tsai, F. H.; Chiang, P. Y.; Kitmura, Y.; Kokowa, M.; Islam, M. Z. Producing Liquid-core Hydrogel Beads by Reverse Spherification: Effect of Secondary Gelation on Physical Properties and Release Characteristics. Food Hydrocolloids. 2017, 62, 140–148. DOI: 10.1016/j.foodhyd.2016.07.002.
- Lee, P. M.; Rogers, A. Effect of Calcium Source and Exposure Time on Basic Caviar Spherification Using Sodium Alginate. Int. J. Gastronomy Food Sci. 2012, 1, 96–100. DOI: 10.1016/j.ijgfs.2013.06.003.
- Draget, K. I.; Taylor, C. Chemical, Physical and Biological Properties of Alginates and Their Biomedical Implications. Food Hydrocolloids. 2011, 25, 251–256. DOI: 10.1016/j.foodhyd.2009.10.007.
- Ching, S. H.; Bansal, N.; Bhandari, B. Alginate Gel Particles – A Review of Production Techniques and Physical Properties. Crit. Rev. Food Sci. Nutr. 2015, 57, 1133–1152. DOI: 10.1080/10408398.2014.965773.
- Anderson, T.; Melvik, J. E.; Gaserod, O.; Alsberg, E.; Christensen, B. E. Ionically Gelled Alginate Foams: Physical Properties Controlled by Operational and Macromelecular Parameters. Biol. Macromol. 2012, 13, 3703–3710.
- Keppeler, A.; Ellis, A.; Jacquier, J. C. Cross-linked Carrageenan Beads for Controlled Release Delivery System. Carbohydr. Polym. 2009, 78, 973–977. DOI: 10.1016/j.carbpol.2009.07.029.
- Anbinder, P. S.; Deladino, L.; Navarro, A. S.; Amalvy, J. I.; Martino, M. N. Yerba Mate Extract Encapsulation with Alginate and Chitosan Systems: Interactions between Active Compound Encapsulation Polymers. J. Encapsulation Adsorpt. Sci. 2011, 1, 80–87. DOI: 10.4236/jeas.2011.14011.
- Belščak-Cvitanović, A.; Stojanović, R.; Manojlović, V.; Komes, D.; Juranović Cindrić, I.; Nedović, V.; Bugarski, B. Encapsulation of Polyphenolic Antioxidants from Medicinal Plant Extracts in Alginate-chitosan System Enhanced with Ascorbic Acid by Electrostatic Extrusion. Food Res. Int. 2011, 44, 1094–1101. DOI: 10.1016/j.foodres.2011.03.030.
- Mohamadnia, Z.; Zohuriaan-Mehr, M. J.; Kabiri, K.; Jamshidi, A.; Mobedi, H. Ionically Cross-linked Carrageenan-alginate Hydrogel Beads. J. Biomater. Sci., Polym. Ed. 2008, 19, 47–59. DOI: 10.1163/156856208783227640.
- Popa, E. G.; Gomes, M. E.; Reis, R. L. Cell Delivery Systems Using Alginate_carrageenan Hydrogel Beads and Fibers for Regenerative Medicine Applications. Biomacromolecules. 2011, 12, 3952–3961. DOI: 10.1021/bm200965x.
- Torres, M. D.; Chenlo, F.; Moreira, R. Structural Features and Water Sorption Isotherms of Carrageenans: A Prediction Model for Hybrid Carrageenans. Carbohydr. Polym. 2018, 180, 72–80. DOI: 10.1016/j.carbpol.2017.10.010.
- Stenner, R.; Matubayasi, N.; Shimizu, S. Gelation of Carrageenan: Effects of Sugars and Polyols. Food Hydrocolloids. 2016, 54, 284–292. DOI: 10.1016/j.foodhyd.2015.10.007.
- Mangione, M. R.; Giacomazza, D.; Bilone, D.; Martorana, V.; Biaro, P. L. Thermoreversible Gelation of K-carrageenan: Relation between Conformational Transition and Aggregation. Biophys. Chem. 2003, 104, 95–105. DOI: 10.1016/S0301-4622(02)00341-1.
- JECFA. Carrageenan. In FAO JECFA Monographs 16: Compedium of Food Additives Specifications (79th Meeting) FAO/WHO, Food and Agriculture Organization of the United Nations, World Health Organizations, Rome, 2014; pp 7–12.
- Paula, G. A.; Benevides, N. M. B.; Cunha, A. P.; Oliveira, A. P.; Pinto, A. M. B.; Morais, P. S.; Azeredo, H. M. C. Development and Characterization of Edible Films from Mixtures of κ-carrageenan, ι-carrageenan and Alginate. Food Hydrocolloids. 2015, 47, 140–145. DOI: 10.1016/j.foodhyd.2015.01.004.
- Kolesnyk, I.; Konovalova, V.; Burban, A. Alginate/κ-carrageenan Microspheres and Their Application for Protein Drug Controlled Release. Chem. Chem. Technol. 2015, 9, 486–492. DOI: 10.23939/chcht09.04.485.
- Belščak-Cvitanović, A.; Komesa, D.; Karlović, S.; Djaković, S.; Igor, Š.; Gordan, M.; Ježeka, M. Improving the Controlled Delivery Formulations of Caffeine in Alginate Hydrogel Beads Combined with Pectin, Carrageenan, Chitosan and Psyllium. Food Chem. 2015, 167, 378–386. DOI: 10.1016/j.foodchem.2014.07.011.
- Krishnamoorthy, B.; Basu, S. K. Chitosan Coated Alginate-carrageenan Particulate Systems for Sustained Release of Naproxen. Int. J. Pharm. Health Care. 2013, 1, 96–108.
- Lin, W. T.; Huang, H. L.; Chan, T. F.; Ciou, S. Y.; Lee, C. Y.; Chiu, Y. W.; Duh, T. H.; Lin, P. L.; Wang, T. N.; Liu, T. Y.; et al. Effect on Uric Acid, Body Mass Index and Blood Pressure in Adolescents of Consuming Beverages Sweetened with High-fructose Corn Syrup. Int. J. Obes. 2013, 37, 532–539. DOI: 10.1038/ijo.2012.48.
- Chan, T. F.; Lin, W. T.; Chen, Y. L.; Huang, H. L.; Yang, W. Z.; Lee, C. Y.; Chen, M. H.; Wang, T. N.; Huang, M. C.; Chiu, Y. W.; et al. Elevated Serum Triglyceride and Retinol-binding Protein 4 Levels Associated with Fructose-sweetened Beverages in Adolescents. Plos One. 2014, 9, 1–9.
- Wong, Y. M.; Siow, L. F. Effects of Heat, pH, Antioxidant, Agitation and Light on Betacyanin Stability Using Red-fleshed Dragon Fruit (hylocereus Polyrhizus) Juice and Concentrate as Models. J. Food Sci. Technol. 2015, 5, 3086–3092. DOI: 10.1007/s13197-014-1362-2.
- Shurikhin, I. M.; Tutelian, V. A. Chemical Composition of Russian Food Products: Handbook; DeLi Print: Moscow, Russia, 2002.
- Krisnaiah, D.; Sarbatly, R.; Prasad, D. M. R.; Bono, A. Mineral Content of Some Seaweeds from Sabah’s South China Sea. Asian J. Sci. Res. 2008, 1, 166–170. DOI: 10.3923/ajsr.2008.166.170.
- Mohammadi, M.; Tajik, H.; Hajeb, P. Nutritional Composition of Seaweeds from the Northern Persian Gulf. Iran. J. Fish. Sci. 2013, 12, 232–240.
- Saha, D.; Bhattacharya, S. Hydrocolloids as Thickening and Gelling Agents in Food: A Critical Review. J. Food Sci. Technol. 2010, 47, 587–597. DOI: 10.1007/s13197-010-0114-1.
- Istini, S.; Ohno, M.; Kushunose, M. Methods of Analysis for Agar, Carrageenan and Alginate in Seaweed. Bull. Dep. Mar. Sci. Univ. Cochin. 1994, 14, 49–55.
- Mangione, M. R.; Giacomazza, D.; Bulone, D.; Martorana, V.; Cavallaro, G.; San Biago, P. L. K (+) and Na (+) Effects on the Gelation Properties of Kappa-carrageenan. Biophys. Chem. 2005, 113, 129–135. DOI: 10.1016/j.bpc.2004.08.005.
- Castellar, M. R.; Obón, J. M.; Fernández-López, J. A. The Isolation and Properties of Red-purple Betacyanin Food Colourant from Opuntia Stricta Fruits. J. Sci. Food Agric. 2006, 86, 122–128. DOI: 10.1002/jsfa.2285.
- Xu, A.; Bartley, J. P.; Johnson, R. A. Preparation and Characterization of Alginate–Carrageenan Hydrogel Films Crosslinked Using a Water-soluble Carbodiimide (WSC). J. Membr. Sci. 2003, 218, 131–146. DOI: 10.1016/S0376-7388(03)00165-0.