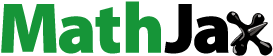
ABSTRACT
The potential of selected industrial food wastes from juice and nut production including apple peel, apple pomace, pomegranate peel, pomegranate seed, chestnut shell, and black carrot pomace as resources for natural antioxidants was investigated. Soluble free and insoluble-bound phenolics were extracted from the wastes and analyzed for total phenolic and flavonoid contents, phenolic profile and antioxidant activity. Total phenolic and total flavonoid contents of wastes were positively correlated with their antioxidant activity. The highest total phenolic and antioxidant activity were determined in soluble fraction of pomegranate peel due to a significant amount of punicalagin derivatives. Pomegranate peel and seed had the most phenolics and flavonoids in soluble form while other wastes had more than 45% of total phenolics in insoluble-bound form. Chestnut shell showed more antioxidant activity in insoluble-bound fraction compared to that of its soluble fraction. These findings showed that not only soluble but also an insoluble-bound fraction of the industrial wastes has good potential for valorization as a source of natural antioxidants.
Introduction
Fruits and vegetables are rich sources of bioactive compounds including flavonoids, phenolic acids, vitamins and pigments that have been associated with the improvement of human health. Phenolic compounds have gained much attention due to their potent antioxidant properties. Phenolics are commonly found in plant-based foods like fruits, vegetables, cereals, olive, legumes, nuts, coffee, and tea.[Citation1] Simple phenolic acids and flavonoids are the most common phenolic compounds and they are present in soluble free, soluble esterified and insoluble-bound forms in plants. Phenolics in the bound form are covalently bound to cell wall structural components such as pectin, cellulose, hemicellulose, lignin, arabinoxylan, and structural proteins. Conventional extraction methods are not sufficient for releasing bound phenolics from these structural components.[Citation1]
In numerous in-vitro antioxidant assays carried out, the bound phenolics have demonstrated a significantly higher antioxidant activity compared to that of soluble phenolics.[Citation2] Furthermore, bound phenolics may survive under conditions of human stomach and small intestine and reach the colon intact where they were released and exert bioactivity.[Citation3] In the food industry, waste is produced after the separation of the desired component or product from undesired components. The direct disposal of undesired components as a waste to the environment represents an important loss of biomass, which could be converted into different products with a higher commercial value. In recent years, extraction of phenolics and other food components from agri-industrial wastes has gained great attention because they could be cheap and safe sources of natural food supplements and ingredients.
One-third of food (around 1.3 billion tons) produced for human consumption in the world is wasted each year according to the Food and Agriculture Organization of the United Nations.[Citation4] This includes 45% of all fruits and vegetables which have the potential to be a valuable co-product. Processing fruits and vegetables leaves behind a substantial amount of residues in the form of peels, seeds, and pomace which have a high amount of valuable food components such as fiber, vitamins, protein, pigments, minerals, hydrocolloids, phenolics and other bioactive components. Processing of apples generate 10.9% waste including seed and pomace. Grape and wine processing industries generate 5 to 9 million metric tons of solid waste in a year. Canning and freezing processes of fruits and vegetables generate 6 million metric tons of leaves, stalks and stems annually.[Citation5]
Processing of fruits and vegetables generates large amount of waste which has valuable components such as bioactive compounds. Edible parts of fruits and vegetables contained 6.5% to 76.3% of total phenolics in bound form depending on the species.[Citation2,Citation3,Citation6] Overleaping of bound phenolics causes underestimation of total phenolic contents and antioxidant activities of fruits and vegetables. Especially fruit peels were reported to contain a significant amount of non-extractable phenolics.[Citation7,Citation8] The contributions of non-extractable polyphenols to total polyphenol content in common fruit peel were reported to be 82% in banana, 80% in orange, 79% in kiwi, 70% in pear, 57% in pineapple, 39% in grapefruit, 32% in apple and 23% in peach.[Citation7–Citation9] Although the presence of soluble phenolic compounds and their antioxidative activity have been reported in agro-industrial wastes, detailed information about the bound phenolics and their antioxidant activity is limited. The aim of the present study was to determine soluble-free and insoluble-bound phenolics and antioxidant activities of industrial plant wastes including apple peel, apple pomace, pomegranate peel, pomegranate seed, chestnut shell and black carrot pomace for providing a complete picture of the localization of phenolics and evaluation of their antioxidative potential as resources for natural antioxidants.
Materials and methods
Materials
All chemicals were analytical grade and purchased from VWR International (Leuven, Belgium) and all HPLC standards were purchased from Sigma-Aldrich (Steinheim, Germany). Apple peel, apple pomace, pomegranate peel, pomegranate seed, and black carrot pomace were collected after separate productions of clear and concentrated juice processes of a local company (Targid, Mersin, Turkey). Chestnut shell (a mixture of the outer brown peel and the inner pellicle) was supplied from a local producer (Kafkas Confectionery, Bursa, Turkey).
Sample preparation
All waste samples were dried separately using a freeze dryer (Labconco Freezone 2.5 Plus, Canada) for 24 h at 0.63 mBar (−48°C). Dried samples were ground finely using stainless steel grinder and then the powered samples were stored at −20°C for use in subsequent analyses.
Extraction of soluble-free and insoluble-bound phenolic compounds
Soluble phenolic compounds were extracted by using the method described by Gonzales et al.[Citation10] with slight modification. Approximately 2 g of freeze-dried sample of each waste was homogenized in 15 mL of 80% methanol in water using ultraturrax (IKA, Wilmington, North Carolina, USA) for 45 s at 10000 rpm. Sample was cooled on ice for 15 min and then the mixture was centrifuged at 2500g for 10 min at 4°C. The residue was re-extracted with 10 mL of 80% methanol following the same procedure and then the volume was completed to 25 mL with 80% methanol. These extracts will be subsequently referred to as soluble phenolics extracts throughout the text. Analysis yielded only soluble-free phenolics not soluble esterified ones which require hydrolysis.[Citation8] The residues were dried overnight at room temperature and analyzed for insoluble-bound phenolic compounds.
Insoluble-bound phenolic compounds were extracted by alkaline hydrolysis method as described by Gonzales et al.[Citation10] Dried residue (0.1 g) was hydrolyzed using 2 mL of 2 N NaOH in a screw-capped test tube which was placed in a probe-sonicated water bath at 100% amplitude (Hielscher UP400S, GmbH, Hamm, Germany) at 60°C for 30 min. The samples were then neutralized with 2 N HCl and extracted with 100% methanol containing 0.1% formic acid. The tubes were centrifuged at 2500g for 10 min at 4°C. Supernatants were collected and extraction was repeated two more times. All supernatants were pooled in the same flask and volume was completed to 20 mL with 90% methanol. These extracts will be subsequently referred to as bound phenolics extracts throughout the text.
Determination of total phenolic and flavonoid contents
Total phenolic content (TPC) was determined according to the Folin-Ciocalteau (FC) method as described by Singleton et al.[Citation11] Deionized water (1 mL) and 0.5 mL of FC reagent (10%, v/v) were added to 1 mL of sample or standard and the contents were vortexed. After 6 min incubation at ambient temperature, 1.5 mL of Na2CO3 (20%, w/v) solution was added and the mixture was incubated for 2 h at room temperature in dark. The absorbance of the solution was measured at 760 nm with a spectrophotometer. Gallic acid was used as a standard in a concentration range between 0 and 50 mg/L. TPC was expressed in mg gallic acid equivalents (GAE) per g of dry matter based on the calibration curve.
Total flavonoids content (TFC) was determined by the colorimetric method as described by Dewanto et al.[Citation12] To 1 mL sample or standard, 0.3 mL NaNO2 (5%, w/v) at the beginning, 0.3 mL AlCl3 (10%, w/v) after 5 min and 2 mL NaOH (1M) after 6 min were added. Finally, 2.4 mL distilled water was added and the absorbance of the resulting solution was measured with a spectrophotometer at 510 nm wavelength. Catechin was used as a standard and TFC were expressed as mg catechin equivalents (CE) per g dry matter.
Determination of phenolic profile of wastes
Phenolic compounds in soluble and bound phenolics extracts of each waste were determined by high-performance liquid chromatography (HPLC, Waters 2695, W600 Waters, Milford, MA, USA) according to the method of Bino et al.[Citation13] Soluble and bound phenolic compounds were separated on a supelcosil LC-18 25 cm×4.60 mm, 5-µm column (Sigma-Aldrich; Steinheim, Germany). The mobile phase consisted of solvent A (Milli-Q water with 0.1% (v/v) TFA) and solvent B (acetonitrile with 0.1% (v/v) TFA) at a flow rate of 1 mL/min. Injection volume of 10 µL was used. Detection was carried out at 280 nm for ellagic acid, gallic acid, catechin, protocatechuic acid, 320 nm for p-coumaric acid, ferulic acid, chlorogenic acid, 360 nm for quercetin, and 520 nm for malvinidin, cyanidin, and peonidin. Phenolic compounds except punigalacin were quantified by using available standards. Punigalacin was identified with UV-spectra from the literature[Citation14] and concentrations of punicalagin derivatives were calculated as ellagic acid equivalent. All concentrations were expressed as mg per 100 g dry matter.
Measurement of antioxidant activity
DPPH (2,2-diphenyl-1-picrylhydrazyl) free-radical scavenging activity was determined by the method described by Rai et al.[Citation15] The CUPRAC (Cupric reducing antioxidant capacity) method was performed as described by Apak et al.[Citation16] Trolox was used as a standard and the results were expressed in terms of mg trolox equivalents (TE) per g dry matter.
Statistical analysis
Experiments and analyses were carried out in triplicate. Data were subjected to one-way analysis of variance (ANOVA). Means were compared by Tukey’s test at a significance level of 0.05. Correlation between phenolic content and antioxidant activity was determined according to the Pearson’s correlation test (Minitab 16, Minitab Inc., Coventry, UK).
Results and discussion
Total phenolic and flavonoid contents of wastes
The highest TPC was found in soluble phenolics extract of pomegranate peel (). The bound phenolics were 88%, 53%, 47%, 45%, 25%, and 16% of TPC of apple peel, black carrot pomace, chestnut shell, apple pomace, pomegranate seed, and pomegranate peel, respectively. The highest TFC was determined in bound phenolics extract of chestnut shell (). About 88%, 86%, 54%, 54%, 49%, and 35% of TFC were present in bound form in apple peel, chestnut shell, black carrot pomace, apple pomace, pomegranate seed, and pomegranate peel, respectively.
Figure 1. Total phenolic (a) and total flavonoid (b) contents of soluble free (black) and insoluble-bound (gray) extracts of plant wastes. Means of soluble and insoluble-bound phenolics for each waste marked with different lowercase letters are significantly different (p < .05). Means of soluble or insoluble-bound phenolics of wastes marked with different uppercase letters are significantly different (p < .05)
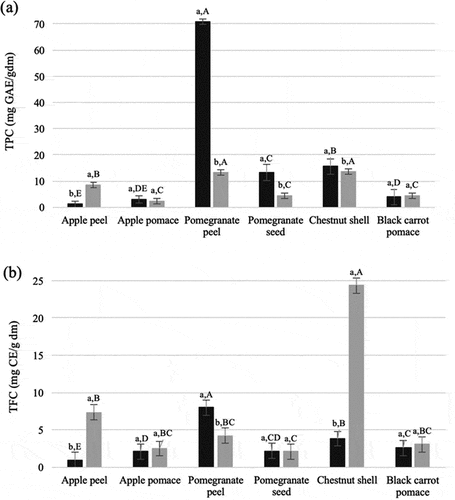
Pomegranate peel contained the highest amount of phenolics in soluble form among the wastes. It also contained higher TPC and TFC in its soluble phenolics extract than those in its bound phenolics extract. Similar distribution in pomegranate seed, juice and peel was also reported by Ambigaipalan et al.[Citation8,Citation17] and Chan et al.[Citation18] This distribution of TPC and TFC in pomegranate peel shows that the majority of phenolics could be extracted at one stage. Pomegranate peel was richer in TPC and TFC than pomegranate seed. Previous studies also showed that pomegranate peel had a higher amount of soluble phenolics than its pulp and seed.[Citation19]
Chestnut shell contained the highest TPC and TFC in its bound phenolics extract among the wastes. While TPC of soluble and bound phenolics extracts of chestnut shell was slightly different, TFC of bound phenolics extract was about 5-times higher than that of soluble phenolics extract. Amount of soluble phenolics of chestnut shell found in this study had a similar order of magnitude with that reported in the study conducted by Squillaci et al.[Citation20]
TPC of black carrot was found 350 mg per 100 g fresh weight by Kaur and Kapoor.[Citation21] Assuming the moisture content of black carrot as 86%, we can conclude that black carrot pomace retains phenolics of fresh black carrot at a level around 16 and 18% in soluble and bound form, respectively.
Phenolics of apple peel were present in bound form more than soluble form. On the other hand, soluble and bound phenolics extracts of apple pomace had similar TPC. Sun et al.[Citation6] also found that edible parts of apple contained more soluble phenolics than bound ones. In addition, Henríquez et al.[Citation22] reported that apple peel had a higher amount of soluble phenolics than those in pulp and whole fruit.
There are differences in TPC and TFC contents reported in this study and others in the literature. This could be explained by the cultivar of plant material, sample preparation and extraction conditions applied in the analysis. Quantification of soluble and bound phenolics presents a complete picture of the localization of phenolics in the wastes.
Antioxidant activity of soluble and insoluble-bound fractions of wastes
The chemical activities of polyphenols in terms of their reducing properties as hydrogen or electron-donating agents predicts their potential for their action as a free-radical scavenger. Antioxidant activity of extracts was determined by DPPH and CUPRAC assays () which based on the ability of an antioxidant to reduce the stable deep purple DPPH radical and electron transfer-based antioxidant activity assay, respectively.
Figure 2. Antioxidant activity of soluble free (black) and insoluble-bound (gray) extracts of plant wastes by DPPH (a) and CUPRAC (b) assays. Means of soluble and insoluble-bound phenolics for each waste marked with different lowercase letters are significantly different (p < .05). Means of soluble or insoluble-bound phenolics of wastes marked with different uppercase letters are significantly different (p < .05)
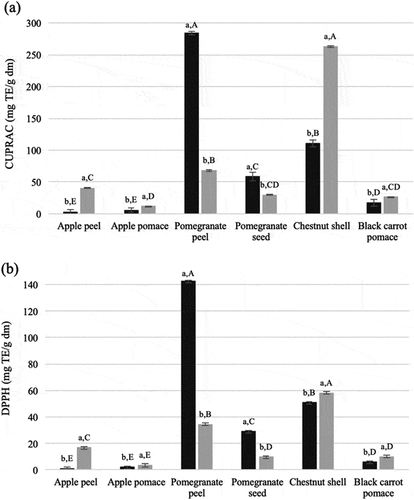
Pomegranate peel and chestnut shell had higher antioxidant activity than other wastes. While soluble phenolics extract of pomegranate peel had the highest antioxidant activity, bound phenolics extract of chestnut shell had the highest antioxidant activity among corresponding extracts of the wastes. Antioxidant activities of bound phenolics extract of apple peel were found to be 17 and 20-fold higher than those of corresponding soluble phenolics extract by CUPRAC and DPPH assays, respectively. Bound phenolics extracts of chestnut shell and black carrot pomace had about 2-fold higher antioxidant activity than those of their soluble phenolics extracts. In addition, black carrot pomace had antioxidant activity of fresh black carrot at a level around 10% and 24% in soluble and bound form, respectively.[Citation23]
Phenolic profile of wastes
Gallic acid, ellagic acid, cyanidin, and punicalagin derivatives were identified in soluble phenolics extract of pomegranate peel (). Punicalagin was reported as the major soluble phenolic of pomegranate husk followed by gallic acid, catechin, epicatechin, ellagic acid, and other hydrolyzable tannins.[Citation24] Ambigaipalan et al.[Citation8] found punicalagin, trace amount of ellagic acid and no gallic acid in soluble free fraction but more of these phenolics were present in soluble esterified fraction of pomegranate peel. In this study, soluble esterified phenolics were not determined. The differences in phenolic profile reported in the studies could arise from extraction and analysis conditions, especially solvents, and fruit cultivar used in the analysis.
Table 1. Concentration of soluble free and insoluble-bound phenolic compounds in wastes
Soluble phenolics extract of pomegranate seed contained ellagic and gallic acids and punicalagin derivatives but at lower concentrations than those in pomegranate peel. Ambigaipalan et al.[Citation17] reported phenolic acids, flavonoids, hydrolysable tannins and anthocyanins in soluble form in pomegranate seed. He et al.[Citation19] also identified caffeic acid, pedunculagin, procyanidin dimer and trimer, catechin, p-coumaric acid, quercitrin, kaempferol and ferulic acid in pomegranate seed.
The major phenolics identified in the bound phenolics extract of pomegranate peel was ellagic and gallic acids (). The concentrations of these compounds were lower in this extract compared to those in the corresponding soluble phenolics extract. Ambigaipalan et al.[Citation8] also reported that gallic acid as the major phenolic acid and ellagic acid present in the insoluble-bound fraction of pomegranate peel. Bound extract of pomegranate seed also contained only ellagic acid in significant amount. Similarly, Ambigaipalan et al.[Citation17] reported that ellagic acid was the main hydrolysable tannin in bound form in pomegranate seed.
There was no punicalagin in bound phenolics extract of pomegranate peel. Antioxidant activity of punicalagin was reported to be 6-fold and 2.5-fold higher than those of ellagic and gallic acids[Citation24] which explains the high antioxidant activity of soluble phenolics extract of pomegranate peel. In addition, a high amount of free ellagic acid possibly gave rise to the antioxidant activity of the peel.[Citation24]
Gallic acid, ellagic acid, and catechin in soluble form and gallic acid, protocatechuic acid and ellagic acid in bound form were identified in chestnut shell. Among the detected compounds, ellagic acid was the most abundant in both soluble and bound phenolics extracts. Squillaci et al.[Citation20] reported that gallic acid was the most abundant phenolic compound identified in an aqueous soluble extract of chestnut shell followed by protocatechuic acid, ellagic acid, epicatechin, catechin, p-coumaric acid, and scopoletin.
Phenolic profile of plants can vary depending on cultivar grown in different geographical conditions. The individual phenolic compounds present in plants can be influenced by soil quality, climate and stress conditions where plants are grown as well as different extraction and analysis conditions.[Citation17]
Bound phenolics extract of chestnut shell had more antioxidant activity than its soluble phenolics extract even though it was not rich in phenolic compounds. Especially antioxidant activity by CUPRAC assay was higher for bound phenolics extract of chestnut shell which was comparable to that of the soluble phenolics extract of pomegranate peel. TFC content of bound phenolics extract of chestnut shell was also higher than those of other wastes. There could be other flavonoid compounds in chestnut shell contributing to the antioxidant activity that were not identified by the HPLC analysis in this study.
Soluble phenolics extract of black carrot pomace contained chlorogenic acid, cyanidin, and malvidin derivatives while bound phenolics extract of it had ferulic acid, chlorogenic acid, sinapic acid, protocatechuic acid, p-coumaric acid and 4-hydroxybenzoic acid. Kamiloglu et al.[Citation25] also found that chlorogenic acid as the most abundant soluble phenolic in black carrot pomace accounting for approximately 90% of the total phenolic acids. They also identified cyanidin derivatives and ferulic acid. Soluble phenolics extract of black carrot pomace contained chlorogenic acid and anthocyanins at a higher level than those of the other wastes. According to phenolic profiles, soluble phenolics extract of black carrot pomace was expected to have higher antioxidant activity than that of its bound phenolics extract but this was not the situation. Bound phenolics extract might have other components that could contribute to its antioxidant activity.
While soluble phenolics extract of apple peel was rich in daidzein, quercetin, quercitrin and phlorizin, that of apple pomace contained high amounts of chlorogenic acid, p-coumaric acid, catechin, epicatechin, naringenin, and phlorizin. Similar soluble phenolics were presented by Suárez et al.[Citation26] for apple pomace. The presence of quercetin derivatives in soluble phenolics extract of apple peel which was not found in bound form in apple peel could be a result of degradation of these phenolics by alkali.
Major phenolics in bound form in apple peel and apple pomace were protocatechuic, vanillic and p-coumaric acids, catechin was also present in apple peel. Catechin in bound phenolics extract of apple peel could originate from residual pomace present. Bound phenolics extract of apple peel had higher antioxidant activity than that of its soluble one. Presence of catechin in bound phenolics extracts of apple peel possibly affected its antioxidant activity positively compared to that of apple pomace.
There was a positive correlation between phenolic content and antioxidant activity. Correlation coefficients were over 0.95 between soluble TPC or TFC and their antioxidant activities by DPPH or CUPRAC tests. Correlation coefficients for bound phenolics and antioxidant activity were relatively lower (0.884 for bound TPC-DPPH, 0.717 for bound TPC-CUPRAC, 0.867 for bound TFC-DPPH, 0.946 for bound TFC-CUPRAC). Correlation coefficient between soluble TPC and antioxidant activity was also reported to be higher than that between soluble TFC and antioxidant activity by DPPH or ORAC tests for different fruit samples.[Citation27]
Even though bound phenolics extracts of wastes except those of pomegranate had higher antioxidant activity, concentrations and number of phenolics in them were generally lesser compared to those of corresponding soluble phenolics extract. This could be due to the presence of unidentified constituents with reducing activity other than phenolics in bound extracts that are released by alkali hydrolysis. In addition, the synergistic action of phenolics in a particular profile can enhance antioxidant activity.
Conclusion
Industrial plant wastes were found to be good resources for phenolic antioxidants. Soluble-free phenolics of pomegranate peel had higher antioxidant activity than that of bound phenolics which shows that most phenolics can be extracted directly. Bound phenolics extracts of pomegranate seed, apple peel, chestnut shell, and black carrot pomace had a higher or equal amount of phenolics and antioxidant activity compared to those of their soluble phenolics extracts. Antioxidant activities of the extracts were not completely related to the phenolic profiles determined by HPLC analysis. There could be other components of the wastes with reducing activity, metal catalysts, or synergistic effect of phenolics affecting the antioxidant activity of the soluble and bound phenolics which require further investigation. Agro-industrial wastes analyzed in this study were found to be rich in phenolic antioxidants in soluble-free and insoluble-bound forms. Production processes need to be developed accordingly to extract both soluble and bound phenolics with high yield and bioactivity.
Correction Statement
This article has been republished with minor changes. These changes do not impact the academic content of the article.
Acknowledgments
Authors would like to thank Ghent University for international mobility and cooperation in research. The study was supported by Istanbul Technical University, Scientific Research Projects Fund (BAP Project no: 39306).
Additional information
Funding
References
- Shahidi, F.; Yeo, J. Insoluble-bound Phenolics in Food. Molecules. 2016, 21, 1–22. DOI: 10.3390/molecules21091216.
- Acosta-Estrada, B. A.; Gutierrez-Uribe, J. A.; Serna-Saldivar, S. O. Bound Phenolics in Foods, a Review. Food Chem. 2014, 152, 46–55. DOI: 10.1016/j.foodchem.2013.11.093.
- Chu, Y. F.; Sun, J.; Wu, X.; Liu, R. H. Antioxidant and Antiproliferative Activities of Common Vegetables. J. Agric. Food Chem. 2002, 50(23), 6910–6916. DOI: 10.1021/jf020665f.
- FAO. FAOSTAT Online Database. 2011. http://faostat.fao.org/ (accessed June 2018)
- Laufenberg, G.; Kunz, B.; Nystroem, M. Transformation of Vegetable Waste into Value Added Products: (a) the Upgrading Concept; (b) Practical Implementations. Bioresour. Technol. 2003, 87, 167–198.
- Sun, J.; Chu, Y. F.; Wu, X.; Liu, R. H. Antioxidant and Antiproliferative Activities of Common Fruits. J. Agric. Food Chem. 2002, 50(25), 7449–7454. DOI: 10.1021/jf0207530.
- Pérez-Jiménez, J.; Saura-Calixto, F. Fruit Peels as Sources of Non-extractable Polyphenols or Macromolecular Antioxidants: Analysis and Nutritional Implications. Food Res. Int. 2018, 111, 148–152. DOI: 10.1016/j.foodres.2018.05.023.
- Ambigaipalan, P.; de Camargo, A. C.; Shahidi, F. Phenolic Compounds of Pomegranate Byproducts (outer Skin, Mesocarp, Divider Membrane) and Their Antioxidant Activities. J. Agric. Food Chem. 2016, 64(34), 6584–6604. DOI: 10.1021/acs.jafc.6b02950.
- Nayak, B.; Liu, R. H.; Tang, J. Effect of Processing on Phenolic Antioxidants of Fruits, Vegetables, and Grains-a Review. Crit. Rev. Food Sci. Nutr. 2015, 55(7), 887–918. DOI: 10.1080/10408398.2011.654142.
- Gonzales, G. B.; Smagghe, G.; Raes, K.; Van Camp, J. Combined Alkaline Hydrolysis and Ultrasound-assisted Extraction for the Release of Nonextractable Phenolics from Cauliflower (brassica Oleracea Var. Botrytis) Waste. J. Agric. Food Chem. 2014, 62, 3371–3376. DOI: 10.1021/jf500835q.
- Singleton, V. L.; Orthofer, R.; Lamuela-Raventós, R. M. Analysis of Total Phenols and Other Oxidation Substrates and Antioxidants by Means of Folin-ciocalteu Reagent. Methods Enzymol. 1999, 299, 152–178.
- Dewanto, V.; Wu, X. Z.; Adom, K. K.; Liu, R. H. Thermal Processing Enhances the Nutritional Value of Tomatoes by Increasing Total Antioxidant Activity. J. Agric. Food Chem. 2002, 50(10), 3010–3014. DOI: 10.1021/jf0115589.
- Bino, R. J.; De Vos, C. R.; Lieberman, M.; Hall, R. D.; Bovy, A.; Jonker, H. H.; Tikunov, Y.; Lommen, A.; Moco, S.; Levin, I. The Light‐hyperresponsive High Pigment-2dg Mutation of Tomato: Alterations in the Fruit Metabolome. New Phytol. 2005, 166(2), 427–438. DOI: 10.1111/j.1469-8137.2005.01362.x.
- Mininel, F. J.; Junior, L.; Sérgio, C.; Espanha, L. G.; Resende, F. A.; Varanda, E. A.; Leite, C. Q. F.; Vilegas, W.; Dos Santos, L. C. Characterization and Quantification of Compounds in the Hydroalcoholic Extract of the Leaves from Terminalia Catappa Linn. (combretaceae) and Their Mutagenic Activity. Evid Based Complement Alternat Med. 2014, 2014, 1–11. DOI: 10.1155/2014/676902.
- Rai, S.; Wahile, A.; Mukherjee, K.; Saha, B. P.; Mukherjee, P. K. Antioxidant Activity of Nelumbo Nucifera (sacred Lotus) Seeds. J. Ethnopharmacol. 2006, 104(3), 322–327. DOI: 10.1016/j.jep.2005.09.025.
- Apak, R.; Güçlü, K.; Özyürek, M.; Karademir, S. E. Novel Total Antioxidant Capacity Index for Dietary Polyphenols and Vitamins C and E, Using Their Cupric Ion Reducing Capability in the Presence of Neocuproine: CUPRAC Method. J. Agric. Food Chem. 2004, 52(26), 7970–7981. DOI: 10.1021/jf048741x.
- Ambigaipalan, P.; de Camargo, A. C.; Shahidi, F. Identification of Phenolic Antioxidants and Bioactives of Pomegranate Seeds following Juice Extraction Using HPLC-DAD-ESI-MS. Food Chem. 2017, 221, 1883–1894. DOI: 10.1016/j.foodchem.2016.10.058.
- Chan, C. L.; Gan, R. Y.; Corke, H. The Phenolic Composition and Antioxidant Capacity of Soluble and Bound Extracts in Selected Dietary Spices and Medicinal Herbs. Int. J. Food Sci. Technol. 2016, 51(3), 565–573. DOI: 10.1111/ijfs.13024.
- He, L.; Xu, H.; Liu, X.; He, W.; Yuan, F.; Hou, Z.; Gao, Y. Identification of Phenolic Compounds from Pomegranate (punica Granatum L.) Seed Residues and Investigation into Their Antioxidant Capacities by HPLC–ABTS+ Assay. Food Res. Int. 2011, 44(5), 1161–1167. DOI: 10.1016/j.foodres.2010.05.023.
- Squillaci, G.; Apone, F.; Sena, L. M.; Carola, A.; Tito, A.; Bimonte, M.; Lucia, A. D.; Colucci, G.; Cara, F. L.; Morana, A. Chestnut (castanea Sativa Mill.) Industrial Wastes as a Valued Bioresource for the Production of Active Ingredients. Process Biochem. 2018, 64, 228–236. DOI: 10.1016/j.procbio.2017.09.017.
- Kaur, C.; Kapoor, H. C. Anti-oxidant Activity and Total Phenolic Content of Some Asian Vegetables. Int. J. Food Sci. Technol. 2002, 37(2), 153–161. DOI: 10.1046/j.1365-2621.2002.00552.x.
- Henríquez, C.; Speisky, H.; Chiffelle, I.; Valenzuela, T.; Araya, M.; Simpson, R.; Almonacid, S. Development of an Ingredient Containing Apple Peel, as a Source of Polyphenols and Dietary Fiber. J. Food Sci. 2010, 75(6), H172–H181. DOI: 10.1111/j.1750-3841.2010.01700.x.
- Koley, T. K.; Singh, S.; Khemariya, P.; Sarkar, A.; Kaur, C.; Chaurasia, S. N. S.; Naik, P. S. Evaluation of Bioactive Properties of Indian Carrot (daucus Carota L.): A Chemometric Approach. Food Res. Int. 2014, 60, 76–85. DOI: 10.1016/j.foodres.2013.12.006.
- Gil, M. I.; Tomás-Barberán, F. A.; Hess-Pierce, B.; Holcroft, D. M.; Kader, A. A. Antioxidant Activity of Pomegranate Juice and Its Relationship with Phenolic Composition and Processing. J. Agric. Food Chem. 2000, 48(10), 4581–4589. DOI: 10.1021/jf000404a.
- Kamiloglu, S.; Capanoglu, E.; Bilen, F. D.; Gonzales, G. B.; Grootaert, C.; Van de Wiele, T.; Van Camp, J. Bioaccessibility of Polyphenols from Plant-processing Byproducts of Black Carrot (daucus Carota L.). J. Agric. Food Chem. 2015, 64(12), 2450–2458. DOI: 10.1021/acs.jafc.5b02640.
- Suárez, B.; Álvarez, Á. L.; García, Y. D.; Del Barrio, G.; Lobo, A. P.; Parra, F. Phenolic Profiles, Antioxidant Activity and in Vitro Antiviral Properties of Apple Pomace. Food Chem. 2010, 120(1), 339–342. DOI: 10.1016/j.foodchem.2009.09.073.
- Kalita, D.; Jayanty, S. S. Comparison of Polyphenol Content and Antioxidant Capacity of Colored Potato Tubers, Pomegranate and Blueberries. J. Food Process. Technol. 2014, 5(8), 1–7.