ABSTRACT
Effects of different temperatures (20–60°C) on gel characteristics, rheology, and protein conformations of pork batters produced by high pressure prior to heating were studied. Cooking yield of batters produced by high pressure was significantly higher than that by heat-only; however, L* values were not significantly different. Highest yield, hardness, springiness, chewiness, and G’ values were observed in batters made by high pressure at 20°C and 30°C. α-helix structure was significantly decreased, with significantly increased β-sheet, β-turn, and random coil structures at 20–40°C. Pork batter thermal gel quality can be improved using high pressure at 20°C and 30°C before heating at 80°C for 25 min.
Introduction
The mechanism of meat proteins unfolded, denaturation and formed gel caused by heat and high pressure is difference. It is well known that high pressure processing induced meat gels are based on the protein volume decline, while the thermal meat gels is caused by the violent movement of molecules and destruction of non-covalent bonds. Myofibrillar protein, the major muscle protein, constitutes approximately 55% of total muscle protein.[Citation1] The functionality of myofibrillar proteins dictates the gel characteristics, because the solubilization of the myofibrillar proteins determines the binding and textural properties of meat products.[Citation2,Citation3] High pressure processing, an emerging technology in the area of meat science, involves changes in the conformation of myofibrillar proteins.[Citation4–Citation6] These conformational changes of myofibrillar proteins are correlation with gel properties, such as the β-sheet structure in the gels. A higher β-sheet structure content imparts increased hardness and water-holding capacity to the gels.[Citation7] Chapleau et al.[Citation8] reported that the surface hydrophobicity and total sulfhydryl groups of rabbit myosin were increased under high pressure between 100 and 200 MPa. Zhang et al.[Citation9] studied the effect of high pressure on myofibrillar protein. The α-helix and β-sheet structures changed into random coil and β-turn structures as the pressure levels increased; moreover, the protein solubility and gel hardness reached their maximum values and the gel microstructure was dense and uniform at 200 MPa. A better understanding of the changes in gel properties and protein conformations occurring in meat products induced by combined high pressure and thermal conditions could be helpful to elucidate their role during gel formation and facilitate the development of new healthy meat products.
High pressure treatment prior to thermal processing improves the functionality of meat batters.[Citation10–Citation12] Khan et al.[Citation10] reported that high pressure treatment before heating could reduce cooking loss, improve color, and enhance textural properties of salted duck meat products. Tintchev et al.[Citation11] showed that the maximal solubilization of myofibrillar proteins occurred at 40°C, 200 MPa, with a reduction of salt content by 50% and improvement of functional properties, such as water-holding capacity and texture. Zheng et al.[Citation12] studied the effects of high pressure applied concurrently or prior to heating and heat alone; they found that high pressure with heating could change the properties of the gel by resisting heat-induced denaturation and gelation of myofibrillar proteins, whereas high pressure before heating altered gel properties by promoting denaturation of myofibrillar proteins. The temperature during high pressure processing also affected water- and fat-holding capacity and gel properties. Combining high pressure and heat treatment at meat protein denaturation temperatures in a single-step process has reportedly resulted in better water retention and texture than heat-only samples.[Citation13,Citation14]
However, the protein conformation changes and gel properties of thermal gels produced by high pressure treatment at 20°C to 60°C are unclear. The research aimed to investigate the physico-chemical and protein conformations of pork batters produced at 200 MPa at various temperatures, for 15 min, prior to heating to evaluate the potential of improving the functionality of meat proteins.
Materials and methods
Prepared pork batters
The longissimus dorsi muscles of chilled pork (after slaughter 24 h, Moisture, 72.12%; protein, 23.03%; fat, 2.06%; pH, 5.67) were derived from the landrace (100 ± 5 kg), which were slaughtered at approximately 6 months of age at ZhongPin Group (China). After removing the visible connective tissue and fat, the pork meat was minced using a meat chopper with a 6-mm hole plate (MGB-120, Shandong Jiaxin Food Machinery Co., Ltd., China). Raw pork batters were prepared by mixing 800 g pork meat with 200 g ice water, together with 20 g NaCl, using a bowl chopper (Stephan UMC-5C, Germany). Briefly, the pork meat was placed into the bowl chopper with NaCl. The mixture was chopped for 30 s with one-third volume of ice water. Another one-third volume of ice water was added and the chopping was continued for 30 s. Finally, the remaining ice water was added and chopping was performed for another 60 s. During the chopping process, the meat batters were maintained at a temperature below 10°C. The raw batters were then stuffed into 20-mm diameter polyamide casings (Xianyi casing Co., Ltd., Henan, China) using a sausage stuffer machine (Xiaojin Machinery Co., Ltd., Hebei Shijiazhuang, China) and linked every 120 mm. Finally, the batters were vacuum packed (Xiaojin Machinery Co., Ltd., Hebei Shijiazhuang, China) for subsequent pressure and thermal processing.
High pressure and temperature combinations treatment
The vacuum-packed meat batters were put into a high pressure vessel having a capacity of 0.3 L and a maximum pressure limit of 900 MPa (S-FL-850–9-W/FPG5620YHL, Stansted Fluid Power Ltd., Stansted, UK); the vessel also had a thermostated jacket with an operational temperature range of −20°C to 90°C. Water was used as a pressure transmitting medium and was adjusted to the desired temperatures by circulating water from a thermostating circulator bath (ILB-WCS, STIK Shanghai Co., Ltd., China). The heat-only batter treatment was denoted as T0; the high pressure treatments done with 200 MPa for 15 min at varying temperature levels were designated as follows: T1, 20°C; T2, 30°C; T3, 40°C; T4, 50°C; and T5, 60°C. Subsequently, all samples were heated in a water bath (HH-42, Changzhou Guohua Electrical Appliance Co., Ltd., China) at 80°C for 25 min (internal temperature 72°C), cooled immediately with running water, and stored at 4°C until analysis.
Cooking yield
The cooking yield of pork batters was calculated according to the following formula:
Cooking yield (%) = cooked meat batter/raw meat batter × 100.
Each measurement was replicated 4 times.
Color
Color measurements were carried out at the middle of the cooked pork batters using a CR-400 colorimeter (Minolta, Japan) and calibrated with a white plate (L* = 96.36, a* = −0.14, b* = 1.92). The results were expressed as L*, a*, and b*. Each measurement was replicated 5 times.
Instrumental texture
The texture profile analyzes of the samples (the cylindrical-shaped with a diameter of 20 mm and a height of 20 mm) were carried out, according to the method described by Kang et al.[Citation15], using a texture analyzer (TA-XT plus Texture analyzer, Stable Micro Systems, UK) with a P/36R aluminum cylindrical probe. The following parameters were used: pre-test speed 2 mm/s, test speed 2 mm/s, post-test speed 2 mm/s, compression ratio 40%, trigger force 5 g, and compression cycle intervals of 5 s. The indicators of hardness, springiness, cohesiveness, and chewiness were determined. Each measurement was replicated 4 times.
Rheology
Rheology is an important index for describing the functional properties of proteins; changes in these functional properties directly affect the gelation properties of pork batters.[Citation16,Citation17] The rheological properties of raw pork batters were measured according to the method described by Sun et al.[Citation18], with slight modifications, using a rotary rheometer (HAAKE MARS III rheometer, Thermo scientific, Germany) equipped with a P35TiL probe (35 mm parallel plate). For every test, approximately 3 g of each sample was evenly spread between the two plates, with a gap of 1 mm and a thin coating of silicone oil to prevent evaporation. The sample was allowed to stabilize at the desired temperature (20°C) for 10 min prior to heating to 80°C, at a rate of 2°C/min, by circulating water. The gelation process was monitored under controlled stress and a constant frequency of 0.1 Hz. During this process, the changes in the storage modulus (G’) were measured. Each measurement was replicated 3 times.
Raman spectroscopic analysis
Raman experiments on the cooked pork batters were performed according to the procedure described by Zhu et al.[Citation19] The batters were evaluated using a Jobin Yvon Labram HR800 spectrometer (Horiba/Jobin. Yvon, Longjumeau, France). A microscope equipped with a 50× lens was used to focus the excitation laser beam (514.5 nm excitation line of a Spectra Physics Ar-laser) on the batter; the Raman signal was collected in the back scattered direction. The spectra were obtained in the range of 400 cm−1 to 4000 cm−1. Each spectrum of cooked pork batters was measured under the following conditions: three scans each, exposure time 30 s, resolution 2 cm−1, sampling speed 120 cm−1/min, and data collection every 1 cm−1. Spectra were smoothed and baselines corrected and normalized against the phenylalanine band at 1003 cm−1[Citation20] using Labspec version 3.01c (Horiba/Jobin. Yvon, Long-jumeau, France). According to the changes of amide I frequencies, the secondary structures of the cooked pork proteins were determined as percentages of α-helix, β-sheet, β-turn, and random coil structures.[Citation21] Each measurement was replicated 3 times.
Statistical analysis
Each experiment had four replicates. The data were analyzed using one-way ANOVA program (SPSS v.18.0 for Windows). The differences between means were considered significant at p < .05.
Results and discussion
Cooking yield
The effect of high pressure at various temperatures on the cooking yield of pork batters is shown in . Compared with the heat-only batter, cooking yields of all pork batters obtained by high pressure significantly increased (p < .05). The higher cooking yield of the pork batters by high pressure reflects a better water-holding capacity. Yang et al.[Citation22] reported that high pressure processing (200 MPa for 2 min) significantly decreased (p < .05) the loss on cooking and changed the P2 peak ratio of the four water components in raw pork sausages. The largest cooking yields of pork batters by high pressure were obtained at 20°C, 30°C, and 40°C; the yields were significantly decreased (p < .05) over 40°C. Excessive or insufficient temperature treatment of meat batters during pressurization was not conducive to the formation of a gel network structure.[Citation23] Marcos et al.[Citation24] reported that a higher cooking yield was observed at 40°C than at 60°C in ostrich meat sausage by pressurization before heating. In contrast, Mcardle et al. found that a higher cooking yield was observed at 40°C than at 20°C in beef sausage by pressurization before heating. On the other hand, Mcardle et al.[Citation25] found that the higher cooking yield was observed at 40°C compared to 20°C in beef by pressurization before heating. The main reason for this difference could be that the batters had different formulae and high pressure processing conditions.
Figure 1. Effect of different temperature intervals (from 20°C to 60°C) at 200 MPa on the cooking yield of pork batter before heated in a water bath at 80°C for 25 min. a-d Different letters indicate significant differences (p < .05). T0: only-heated; T1, 20°C/200 MPa/15 min; T2, 30°C/200 MPa/15 min; T3, 40°C/200 MPa/15 min; T4, 50°C/200 MPa/15 min; T5, 60°C/200 MPa/15 min. All values are the means ±standard deviation of 4 replicates
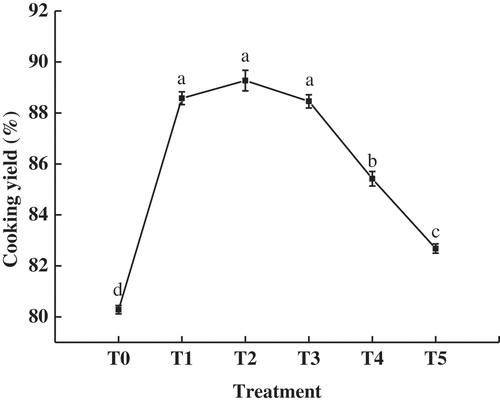
Color
The effect of high pressure at various temperatures on the color of cooked pork batters is shown in . Compared with the heat-only batter, the L* values of pork batters obtained by high pressure were not significantly different (p > .05). One possible reason was that protein denaturation caused by high pressure led to the formation of a denser meat protein network. Another reason could be the changed oxidative states of the pigment myoglobin.[Citation5,Citation26] Yang et al.[Citation27] found that sausages treated at 200 MPa were much lighter and whiter than those treated at 0.1 MPa. The difference was due to the duration of the high pressure treatment. The a* values were significantly increased (p < .05) at 30°C, and the largest a* values were obtained at 40 °C and 50 °C. The b* values of pork batters obtained by high pressure were significantly increased (p < .05) with the increased temperature, which was also an indication of the oxidation and denaturation of myoglobin between the high pressure and thermal conditions.
Table 1. Effect of different temperature intervals (from 20°C to 60°C) at 200 MPa on the instrumental color (L*, a*, and b* values) of pork batter before heated in a water bath at 80°C for 25 min
Instrumental texture
The texture of the cooked pork batters was significantly affected (p < .05) by the high pressure and temperature combinations (). Compared with heat-only, the hardness, springiness, cohesiveness, and chewiness of batters by high pressure at 20°C, 30°C, and 40°C were significantly increased (p < .05). Yang et al.[Citation27] reported that, compared with the values of 0.1 MPa-treated sausages, those treated at 200 MPa for 2 min at 10°C showed significantly increased (p < .05) values for texture parameters. Some researchers reported that high pressure processing can induce muscle protein gels to form a firmer texture.[Citation28,Citation29] Zheng et al.[Citation14] showed that the sausages produced by high pressure before heating had significantly higher values for hardness, springiness, cohesiveness, chewiness, and resilience than the heat-only sausages. Compared with the 20°C and 30°C treatments, all the texture parameters of 40°C, 50°C, and 60°C treated groups were significantly decreased (p < .05). High pressure causes protein denaturation with increased pressure and temperature.[Citation11,Citation30] The reason for this is that the combination of pressure and heat improve the efficiency of protein aggregation and gelation and lead to heat-induced helix-coil transition.[Citation31] Thus, here, excessive temperature resulted in the weakening of molecular interactions and the destruction of the network structure in gels.[Citation32] In addition, the temperature and high pressure treatment affected the moisture in the pork batters, which, in turn, affected the texture of the gel.[Citation33] Zheng et al.[Citation12] found that combined high pressure and heat changed the gel properties by resisting the heat-induced denaturation and gelation of myofibrillar proteins, which caused these texture parameters values to be lower than those of batters produced with high pressure before heating.
Table 2. Effect of different temperature intervals (from 20°C to 60°C) at 200 MPa on the texture profile analysis of pork batter before heated in a water bath at 80°C for 25 min
Rheological properties
The G′ values of pork batters produced by high pressure at various temperatures are shown in . A significant difference was observed in the G′ of pork batters at different temperatures; the initial G′ values were increased with the increase of temperature from 20°C to 60°C; the main reason for this could be the difference in the thermodynamic properties and thermal stability between the two caused by meat protein denaturation.[Citation15] T0, T1, and T2 displayed similar heating curves, with three phases evident in the G′ during the heating process of raw pork batters. The first phase showed that the G′ values tended to slightly increase as the temperatures were increased from 42°C to 52°C (T0) and 44°C to 52°C (T1 and T2) as gelation was initiated following interactions between the proteins.[Citation34,Citation35] As the pressure protected the meat protein from subsequent thermal denaturation, T1 and T2 had a higher denaturation temperature than T0.[Citation36] In the second phase, the G′ values slightly decreased from 53°C to 58°C (T0) and 53°C to 59°C (T1 and T2) as a result of the denaturation of the myosin tails, possibly by the disruption of the protein network that had previously formed at lower temperatures.[Citation37] G′ then increased rapidly as the temperature increased to 80°C, as a result of the transformation from a viscous sol to an elastic gel network.[Citation38] T3 had an unique heating curve; there was a slight increase from 47°C to 54°C and a slight decrease from 55°C to 59°C because of the myosin denaturation caused by high pressure and heat. T4 and T5 had a similar heating curve, and two phases were involved during the heating process[Citation39]; as the myosin was completely denatured during the combined high pressure and thermal treatment, the G′ values tended to slightly decrease with temperature from 21°C to 60°C in the first phase, which probably resulted from fat softening and the rupture of the hydrogen bonds. In the second phase, a slight increase in G′ values was observed from 61°C to 80°C, as gelation was initiated, following protein-protein interactions, mainly by the formation of hydrophobic interactions and covalent disulfide bonds.[Citation40,Citation41]
Figure 2. Effect of different temperature intervals (from 20°C to 60°C) at 200 MPa on the cooking yield storage modulus (G’, kPa) of pork batter before heated in a water bath at 80°C for 25 min. T0: only-heated; T1, 20°C/200 MPa/15 min; T2, 30°C/200 MPa/15 min; T3, 40°C/200 MPa/15 min; T4, 50°C/200 MPa/15 min; T5, 60°C/200 MPa/15 min
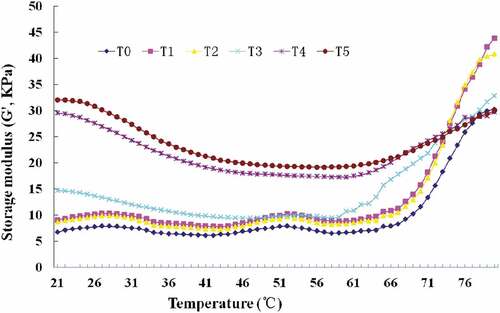
Raman spectroscopic
The percentages of the secondary structures of proteins in the pork batters are shown in . The secondary structures of the cooked meat batters were affected by temperature and pressure. There was a significant decrease (p < .05) in the content of the α-helix structure with increasing temperature and pressure; however, the content of the random coil structure was significantly increased (p < .05). The main reason was the pressure-induced gel formation of myosin, which was attributable to the denaturation of myosin, and the increase of protein surface hydrophobicity and sulfhydryl content.[Citation42] The secondary structures of meat protein are sensitive to changes in the hydrogen bonding scheme involving the peptide linkages of amide I band, which is attributable to α-helix, β-sheet, β-turn, and random coil structures, respectively.[Citation43,Citation44] Berhe et al.[Citation45] reported that meat protein cooked above 60°C positively correlates to the high intensity of bands at the amide I regions. The results indicated a significant (p < .05) increase in the β-sheet and β-turn structure content accompanied by a concomitant decrease in α-helix content. Zhang et al.[Citation9] found that at increased pressure levels, α-helix and β-sheet changed into random coil and β-turn structures; moreover, the surface hydrophobicity and formation of disulfide bonds were strengthened. Compared with the heat-only batters, in the batters produced by high pressure at 200 MPa for 15 min, the content of β-sheet and β-turn structures were significantly increased (p < .05) from 20°C to 40°C. Additionally, there were no significant differences (p > .05) from 50°C to 60°C because the myofibrillar protein had completely denatured during high pressure processing.
Table 3. Effect of different temperature intervals (from 20°C to 60°C) at 200 MPa on the percentages of protein secondary structures α-helix, β-sheet, β-turn, random coil of pork batter before heated in a water bath at 80°C for 25 min
Conclusion
The effects of high pressure and temperature combinations on the thermal gel properties and protein conformations of pork batters were significantly different. Compared with the heat-only batter, the cooking yield and texture parameters of high pressure batters were significantly increased (p < .05) at 20°C, 30°C, and 40°C. In the pork batters prepared at high pressure, the highest cooking yield and texture parameters were obtained at 20°C and 30°C. The initial G’ values improved with increased temperatures. Meanwhile, the secondary structures changed from the α-helix structure to the random coil, β-sheet, and β-turn structures from 20°C to 40°C. Overall, the gel properties of pork batters could be improved using high pressure at 20°C and 30°C.
Additional information
Funding
References
- Lawrie, R. A.;. Awrie’s Meat Science, 7th ed.; Woodhead Publishing Limited: Abington, 2006.
- Chen, X.; Tume, R. K.; Xu, X.; Zhou, G. Solubilization of Myofibrillar Proteins in Water or Low Ionic Strength Media: Classical Techniques, Basic Principles and Novel Functionalities. Crit. Rev. Food Sci. Nutr. 2017, 57(15), 326–3280. DOI: 10.1080/10408398.2015.1110111.
- Kang, Z.-L.; Sheng-Jie., H.; Dong-Yang., Z.; Han-jun, M. Effect of Sodium Chloride and Processing Methods on Protein Aggregation, Physical-Chemical and Rheological Properties of Pork Batters. Int. J. Food Eng. 2018, 14. DOI: 10.1515/ijfe-2017-0319.
- Supavititpatana, T.; Apichartsrangkoon, A. Combination Effects of Ultra-high Pressure and Temperature on the Physical and Thermal Properties of Ostrich Meat Sausage (yor). Meat Sci. 2007, 76(3), 555. DOI: 10.1016/j.meatsci.2007.01.007.
- Bak, K. H.; Lindahl, G.; Karlsson, A. H.; Orlien, V. Effect of High Pressure, Temperature, and Storage on the Color of Porcine Longissimus Dorsi. Meat Sci. 2012, 92(4), 374. DOI: 10.1016/j.meatsci.2012.02.002.
- Zheng, H.; Han, M.; Yang, H.; Tang, C.; Xu, X.; Zhou, G. Application of High Pressure to Chicken Meat Batters during Heating Modifies Physicochemical Properties, Enabling Salt Reduction for High-quality Products. LWT - Food Sci. Technol. 2017, 84, 693–700. DOI: 10.1016/j.lwt.2017.06.006.
- Shao, J. H.; Zou, Y. F.; Xu, X. L.; Wu, J. Q.; Zhou, G. H. Evaluation of Structural Changes in Raw and Heated Meat Batters Prepared with Different Lipids Using Raman Spectroscopy. Food Res. Int. 2011, 44, 2955–2961. DOI: 10.1016/j.foodres.2011.07.003.
- Chapleau, N.; Mangavel, C.; Compoint, J. P.; de Lamballerie-Anton, M. Effect of High-pressure Processing on Myofibrillar Protein Structure. J. Sci. Food Agric. 2004, 84, 66–74. DOI: 10.1002/jsfa.1613.
- Zhang, Z.; Yang, Y.; Zhou, P.; Zhang, X.; Wang, J. Effects of High Pressure Modification on Conformation and Gelation Properties of Myofibrillar Protein. Food Chem. 2017, 217, 678–686. DOI: 10.1016/j.foodchem.2016.09.040.
- Khan, M. A.; Ali, S.; Abid, M.; Ahmad, H.; Zhang, L.; Tume, R. K.; Zhou, G. Enhanced Texture, Yield and Safety of a Ready-to-eat Salted Duck Meat Product Using a High Pressure-heat Process. Innovative Food Sci. Emerging Technol. 2014, 21, 50–57. DOI: 10.1016/j.ifset.2013.10.008.
- Tintchev, F.; Bindrich, U.; Toepfl, S.; Strijowski, U.; Heinz, V.; Knorr, D. High Hydrostatic Pressure/temperature Modeling of Frankfurter Batters. Meat Sci. 2013, 94(3), 376–387. DOI: 10.1016/j.meatsci.2013.02.012.
- Zheng, H.; Han, M.; Bai, Y.; Xu, X.; Zhou, G. Combination of High Pressure and Heat on the Gelation of Chicken Myofibrillar Proteins. Innovative Food Sci. Emerging Technol. 2019, 52, 122–130. DOI: 10.1016/j.ifset.2018.10.014.
- Jimenezcolmenero, F.; Fernandez, P.; Carballo, J. L.; Fernandezmartin, F. High-pressure-cooked Low-fat Pork and Chicken Batters as Affected by Salt Levels and Cooking Temperature. J. Food Sci. 1998, 63(4), 656–659. DOI: 10.1111/j.1365-2621.1998.tb15806.x.
- Zheng, H.; Xiong, G.; Han, M.; Deng, S.; Xu, X.; Zhou, G. High Pressure/thermal Combinations on Texture and Water Holding Capacity of Chicken Batters. Innovative Food Sci. Emerg. Technol. 2015, 30, 8–14. DOI: 10.1016/j.ifset.2015.06.002.
- Kang, Z. L.; Li, B.; Ma, H. J.; Zhu, Y. P.; Song, Z. J.; Pan, R. S. Effect of Soybean Protein Isolate Addition Method on Gel Properties of Pork. Mod. Food Sci. Technol. 2016, 32(6), 220–224.
- Fernández-Martín, F.; Fernández, P.; José Carballo, A.; Colmenero, F. J. Pressure/heat Combinations on Pork Meat Batters: Protein Thermal Behavior and Product Rheological Properties. J.Agric.Food Chem. 1997, 45(11), 4440–4445. DOI: 10.1021/jf9702297.
- Debusca, A.; Tahergorabi, R.; Beamer, S. K.; Partington, S.; Jaczynski, J. Interactions of Dietary Fibre and Omega-3-rich Oil with Protein in Surimi Gels Developed with Salt Substitute. Food Chem. 2013, 141(1), 201–208. DOI: 10.1016/j.foodchem.2013.02.111.
- Sun, J.; Wu, Z.; Xu, X.; Li, P. Effect of Peanut Protein Isolate on Functional Properties of Chicken Salt-soluble Proteins from Breast and Thigh Muscles during Heat-induced Gelation. Meat Sci. 2012, 91(1), 88–92. DOI: 10.1016/j.meatsci.2011.12.010.
- Zhu, D.-Y.; Kang, Z.-L.; Ma, H.-J.; Xu, X.-L.; Zhou, G.-H. Effect of Sodium Chloride or Sodium Bicarbonate in the Chicken Batters: A Physico-chemical and Raman Spectroscopy Study. Food Hydrocolloids. 2018, 83, 222–228.
- Herrero, A. M.;. Raman Spectroscopy A Promising Technique for Quality Assessment of Meat and fish: A Review. Food Chem. 2008, 107, 1642–1651.
- Alix, A. J. P.; Pedanou, G.; Berjot, M. Determination of the Quantitative Secondary Structure of Proteins by Using Some Parameters of the Raman Amide I Band. J. Mol. Struct. 1988, 174, 159–164. DOI: 10.1016/0022-2860(88)80151-0.
- Yang, H.; Han, M.; Bai, Y.; Han, Y.; Xu, X.; Zhou, G. High Pressure Processing Alters Water Distribution Enabling the Production of Reduced-fat and Reduced-salt Pork Sausages. Meat Sci. 2015, 102, 69–78. DOI: 10.1016/j.meatsci.2014.10.010.
- Fernández-Martín, F.;. Bird Muscles under Hydrostatic High-pressure/temperature Combinations. J. Therm. Anal. Calorim. 2007, 87(1), 285–290. DOI: 10.1007/s10973-006-7809-6.
- Marcos, B.; Kerry, J. P.; Mullen, A. M. High Pressure Induced Changes on Sarcoplasmic Protein Fraction and Quality Indicators. Meat Sci. 2010, 85(1), 115. DOI: 10.1016/j.meatsci.2009.12.014.
- Mcardle, R.; Marcos, B.; Kerry, J. P.; Mullen, A. Monitoring the Effects of High Pressure Processing and Temperature on Selected Beef Quality Attributes. Meat Sci. 2010, 86(3), 629. DOI: 10.1016/j.meatsci.2010.05.001.
- Duranton, F.; Marée, E.; Simonin, H.; Chéret, R.; Marie, D. L. Effect of High Pressure–high Temperature Process on Meat Product Quality. High Pressure Res. 2011, 31(1), 163–167. DOI: 10.1080/08957959.2010.541242.
- Yang, H.; Khan, M. A.; Yu, X.; Zheng, H.; Han, M.; Xu, X.; Zhou, G. Changes in Protein Structures to Improve the Rheology and Texture of Reduced-fat Sausages Using High Pressure Processing. Meat Sci. 2016, 121, 79–87. DOI: 10.1016/j.meatsci.2016.06.004.
- Villamonte, G.; Simonin, H.; Duranton, F.; Cheret, R.; de Lamballerie, M. Functionality of Pork Meat Proteins: Impact of Sodium Chloride and Phosphates under High Pressure Processing. Innovative Food Sci. Emerg. Technol. 2013, 18, 15–23. DOI: 10.1016/j.ifset.2012.12.001.
- Ma, H. J.; Ledward, D. A. High Pressure/thermal Treatment Effects on the Texture of Beef Muscle. Meat Sci. 2004, 68(3), 347–355. DOI: 10.1016/j.meatsci.2004.04.001.
- Truong, B. Q.; Buckow, R.; Nguyen, M. H.; Furst, J. E. Gelation of Barramundi (lates Calcarifer) Minced Muscle as Affected by Pressure and Thermal Treatments at Low Salt Concentration. J. Sci. Food Agric. 2017, 97(11), 3781–3789. DOI: 10.1002/jsfa.8242.
- Buckow, R.; Sikes, A. L.; Tume, R. K. Effect of High Pressure on Physicochemical Properties of Meat. Crit. Rev. Food Sci. Nutr. 2013, 53(7), 770–786. DOI: 10.1080/10408398.2011.560296.
- Jimenez, C. F.;. Muscle Protein Gelation by Combined Use of High Pressure/temperature. Trends Food Sci. Technol. 2002, 13(1), 22–30. DOI: 10.1016/S0924-2244(02)00024-9.
- Cando, D.; Moreno, H. M.; Tovar, C. A.; Herranz, B.; Borderias, A. J. Effect of High Pressure And/or Temperature over Gelation of Isolated Hake Myofibrils. Food Bioprocess Technol. 2014, 7(11), 3197–3207. DOI: 10.1007/s11947-014-1279-9.
- Xiong, Y. L.; Brekke, C. J. Physical and Gelation Properties of Pre- and Postrigor Chicken Salt-soluble Proteins. J. Food Sci. 1990, 55, 1544. DOI: 10.1111/j.1365-2621.1990.tb03564.x.
- Perezmateos, M.; Montero, P. Effects of Hydrocolloids and High-pressure-heating Processing on Minced Fish Gels. Eur. Food Res. Technol. 2002, 214(2), 119–124. DOI: 10.1007/s00217-001-0426-5.
- Kang, Z.; Wang, P.; Xu, X.; Zhu, C.; Zou, Y.; Li, K.; Zhou, G. Effect of a Beating Process, as a Means of Reducing Salt Content in Chinese-style Meatballs (kung-wan): a Dynamic Rheological and Raman Spectroscopy Study. Meat Sci. 2014, 96(2), 669–674. DOI: 10.1016/j.meatsci.2013.09.024.
- Tornberg, E.;. Effects of Heat on Meat Proteins - Implications on Structure and Quality of Meat Products. Meat Sci. 2005, 70(3), 493. DOI: 10.1016/j.meatsci.2004.12.008.
- Álvarez, D.; Xiong, Y. L.; Castillo, M.; Payne, F. A.; Garrido, M. D. Textural and Viscoelastic Properties of Pork Frankfurters Containing Canola-olive Oils, Rice Bran, and Walnut. Meat Sci. 2012, 92(1), 8–15. DOI: 10.1016/j.meatsci.2012.03.012.
- Colmenero, F. J.;. Muscle Protein Gelation by Combined Use of High Pressure/temperature. Trends Food Sci. Technol. 2002, 13(1), 22–30. DOI: 10.1016/S0924-2244(02)00024-9.
- Esturk, O.; Park, J. W. Comparative Study on Degradation, Aggregation and Rheological Properties of Actomyosin from Cold, Temperate and Warm Water Fish Species. Turk J Fish Quat Sci 2014, 14(1), 67–75.
- Yin, T.; Park, J. W. Effects of Nano-scaled Fish Bone on the Gelation Properties of Alaska Pollock Surimi. Food Chem. 2014, 150(2), 463–468. DOI: 10.1016/j.foodchem.2013.11.041.
- Cao, Y.; Xia, T.; Zhou, G.; Xu, X. Themechanism of High Pressure-induced Gels of Rabbit Myosin. Innovative Food Sci. Emerging Technol. 2012, 16, 41–46. DOI: 10.1016/j.ifset.2012.04.005.
- Kang, Z. L.; Li, B.; Ma, H. J.; Chen, F. S. Effect of Different Processing Methods and Salt Content on the Physicochemical and Rheological Properties of Meat Batters. Int. J. Food Prop. 2016, 19(7), 1604–1615
- Ngarize, S.; Herman, H.; Adams, A.; Howell, N. K. Comparison of Changes in the Secondary Structure of Unheated, Heated, and Highpressure-treated β-lactoglobulin and Ovalbumin Proteins Using Fourier Transform Raman Spectroscopy and Self-deconvolution. J. Agric. Food Chem. 2004, 52, 6470–6477. DOI: 10.1021/jf030649y.
- Berhe, D. T.; Engelsen, S. B.; Hviid, M. S.; Lametsch, R. Raman Spectroscopic Study of Effect of the Cooking Temperature and Time on Meat Proteins. Food Res. Int. 2014, 66, 123–131. DOI: 10.1016/j.foodres.2014.09.010.