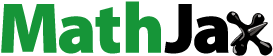
ABSTRACT
In this article, the influence of various cryogenic quick-freezing preservation methods on the quality and microstructure of cabbage (Brassica oleracea L. var. capitata L.) slices were investigated during a 28 d storage period. The results indicated that when compared to other methods, the microstructure of the cabbage slices that were frozen cabbage using dry ice displayed the least amount of modifications when stored. The content of chlorophyll, vitamin C (VC), and titratable acidity (TA) in these samples presented minimal changes at the end of storage, which were 1.5147 mg/g, 26.2 mg/100 g, and 0.2623%, respectively. Moreover, the samples exposed to dry ice freezing exhibited the highest content of total sugar that reduced following 28 d of storage. In addition, this combined treatment prevented textural changes in the cabbage slices. For these samples, the values representing the hardness, chewiness, springiness, and cohesiveness were 47.01 N, 14.54 N mm, 1.5147 mm and 3.363, respectively. Furthermore, dry ice freezing treatment could mostly retard the microbiological degradation of the cabbage slices. Finally, the sensory quality of samples treated with dry ice freezing scored 6.4 at the conclusion of the storage period. The samples that were frozen at −60°C displayed enhanced preservation properties, while the sensory quality scored only 5.2. These results indicated that dry ice freezing treatment and storage at −18°C could maintain the microstructure and nutritional quality of cabbage slices.
Introduction
Cabbage (Brassica oleracea L. var. capitata L.) is an economically essential vegetable which is cultivated and consumed worldwide due to its excellent adaptability, affordability, and year-round availability. It is also vegetable that typically produces good-quality high yields in the Sichuan-Tibet Plateau of China. Cabbage is an integral part of food production due to its rapid growth rate and the fact that it is not limited to one season a year. This vegetable is the source of several beneficial vitamins (A, C, E, K), sulfur-containing compounds, and other phytochemicals such as glucosinolates.[Citation1,Citation2] However, cabbage crops suffer severe post-harvest losses and exhibit high oxidase activity even at subzero temperatures. Therefore, its economic potential for significant profitability is usually limited.[Citation3] More importantly, many factors, including a variety of biotic, abiotic and environmental factors, can induce the short shelf life and bad quality of cabbage during prolonged storage periods.[Citation4,Citation5]
The continued post-harvest activity of a variety of enzymes may be responsible for the modified flavor, color, texture, and nutritional quality in vegetables.[Citation6] Moreover, these effects may also result from storage conditions and processing methods. During the storage of post-harvest vegetables, the enzymes in the tissue cells remain highly active and can destroy its sensory and nutritional quality.[Citation7] Therefore, blanching eliminates enzyme activity and is vital before the vegetables can be frozen.[Citation8] More importantly, an integrated or inbuilt antioxidant system scavenges free radicals and activates the defense system of the vegetables, extending its shelf life as a result.[Citation9–Citation12]
Before freezing, it is necessary to blanch green asparagus to deactivate the enzymes. This process allows for the retention of vegetable quality during frozen storage.[Citation13] Therefore, blanching is essential before vegetables are frozen to promote their preservation. Blanching deactivates the enzymes responsible for changes in the flavor and smell of the vegetables, as well as the nutritional properties such as the loss of vitamins during the storage period.[Citation14] Therefore, the application of blanching technology facilitates enhanced texture, color, and flavor of vegetables, while improving its nutritional value.[Citation8] Furthermore, freezing is considered to be one of the most efficient food preservation methods. Frozen vegetables can be stored for several months since the rate of decay is significantly lower than at high temperatures due to the water being suspended as ice. However, the formation of ice crystals accompanies the freezing process of products that contain moisture and destroys its structure. This damage is the result of both mechanical and osmotic factors. Therefore, rapid freezing rates and frozen storage at a low and constant temperature are two crucial prerequisites for the quick-frozen technology to deliver high-quality products.[Citation15] Consequently, the combined application of blanching and quick-freezing at a lower temperature is ideal for maintaining the storage quality of vegetables.
The activity of chemical, biochemical, and physical factors are responsible for specific storage conditions, in particular, concerning storage time and temperature. These conditions significantly impact the characteristics of vegetables during frozen storage.[Citation16,Citation17] The challenge posed by ice crystals is not so much reliant on the solidification of water particles, but instead on the dissolution of food tissues due to large ice crystals. Consequently, ice needles resembling those present in deep-frozen cheeses can emerge from the surfaces of food.[Citation18] In some cases, frozen foods can display similar or enhanced sensorial qualities.[Citation19] Following pre-freezing processing, the chlorophyll, carotenoid, and phenol levels, as well as the antioxidant capability of broccoli florets, are comparatively higher than the glucosinolate accumulations.[Citation20] However, the dry ice flash-freezing method has not yet been applied for the preservation of fruits and vegetables. Furthermore, no literature exists involving the effects of cryogenic quick-freezing preservation treatment on the microstructure and quality of cabbage slices. Therefore, this investigation aimed to study the impact of blanching and freezing at −20°C, −60°C, and dry ice freezing, as well as −18°C frozen storage on the quality and structural composition of cabbage slices. Moreover, this study provides a theoretical basis for the commercialized storage of cabbage.
Materials and methods
Main materials
The cabbage was purchased from the Lvyin Agriculture Development Co. Ltd. (Ngawa Tibetan and Qiang Autonomous Prefecture, China), and transported to a pre-cooling facility where it was stored overnight at 4°C for further use.[Citation21–Citation23] Fresh-kept bags were purchased in Chengdu, China from a local wholesale market. Solid carbon dioxide (dry ice) was acquired from the Messer Gas Product Co., Ltd. (Chengdu, China), and transferred to the laboratory for overnight storage at −18°C before processing.
Color-protecting treatment and blanching
The technique used to prepare the color-protecting solutions was developed and described by Gorny et al.[Citation24] Cabbages were cut into strips (50 mm × 10 mm), and submerged in a solution for 2 min at 20°C. This solution was prepared by dissolving 2 g/100 g ascorbic acid, 1 g/100 g calcium lactate, and 0.5 g/100 g cysteine, and altered with NaOH to pH 7.0. According to the method reported by Petzold et al.[Citation25], the blanching treatments were conducted at 65°C for 1 min in a liquid containing 1 g/100 g citric acid and 1 g/100 g ascorbic acid. These samples were placed over a plastic sieve for 5 min, and then, at room temperature, air-dry naturally for 60 min until the surface of the sliced cabbage was dried.
Quick-freezing preparation
Following blanching, the cabbage slices were subjected to a cold-water cooling process for 3 min. After the samples were drained, as shown in , they were placed on metal trays in a blast freezer (Heto Co., Denm ark) at an average air temperature of −20°C, −60°C, and frozen using dry ice.[Citation26] The centre of each cabbage slice was exposed to a Micro Needle Type T Thermocouple Probe (Copper-Constantan) (Ellab A/S, Rodovre, Denmark). The quick-freezing test ended when the centre temperature of the test sample reached −18°C.
Table 1. The freezing experiment parameters
Immediately after the freezing process, about 500 g of cabbage slices were placed in polyethylene packaging bags and placed in a freezer for storage at −18°C (Heto Co., Denmark). During the storage period of 28 d, the sample indexes, including surface microstructure, texture, residual chlorophyll content, VC, TA, sugar, microbiological content, and sensory acceptability were measured at seven-day intervals. Moreover, the morphological observation of sample surfaces was conducted using by a Scanning Electron Microscope (SEM).
Freezing curve, freezing rate, and drip loss
The freezing curve of the cabbage was measured using a multichannel temperature recorder (Shenhuaxuan Technology CO. Ltd., China) according to the method reported by Liang et al.[Citation27] with one Micro Needle Type T Thermocouple Probe in the centre of the cabbage. Measurements were taken every 30 s until the centre temperature of the cabbage reached 18°C. The freezing rate is defined as the difference between the initial and final centre temperature of the product divided by freezing time (°C/min).[Citation28] The drip loss of the cabbage slices was evaluated using the method described by Kaale et al.[Citation29] The quantitative method used to evaluate drip loss dictates removing the sample from the vacuum bag after being thawed in the freezer at 4°C for approximately 4 h and then weighing the remaining liquid in the bag. The definition of drip loss is the percentage of the weight after drip loss compared with the unthawed sample weight.
Morphological observation using SEM
Following various quick-freezing preparations, samples of about 10 mm2 each were cut from the cabbage slices and dried for 12 h in a vacuum freeze-drying machine. The dried cabbage slices with a scan size of 40 mm×40 mm were observed using SEM at a voltage level of 5 kV acceleration after being adhered to the stub with double-sided tape and Pt sputtering.[Citation30,Citation31]
Chlorophyll, VC, and TA content
Besides minor alterations, the chlorophyll content was established using the method delineated by Albanese et al.[Citation32] A shaker bath was employed to homogenize a 5 g sample in 10 mL of 80 mL/100 mL acetone solution for 20 min at 4°C. The specimen was then centrifuged at 13,500 × g (Biofuge Primo, Italy). This process was followed by the filtration of the supernatant which produced a 10 mL dilution with 80 mL/100 mL acetone. Subsequently, a DU 640B spectrophotometer was used to measure the absorbance (A) at 645 nm and 663 nm, respectively (Beckman, USA). Concentration levels of chlorophyll a and b were calculated as follows:
The VC levels in the cabbage slices were determined using High-Performance Liquid Chromatography (HPLC)-Ultraviolet (UV), as described by Duthie et al.[Citation33] Xing et al. [6Citation6]and Xing et al.[Citation34] Ten grams of cabbage was homogenized with 25 mL of the solution, including 7.2 g/L of DL-1.4-dithiothreitol, and 45 g/L of metaphosphoric acid. Moreover, the mixture was centrifuged at 10,000 × g for 15 min at 4°C. A Millipore 0.45 membrane was used to filter the obtained supernatant, which was then introduced into the HPLC system. Each stationary phase sample consisted of 20 mL and was inserted into a reverse-phase C18 HPLC column, followed measurement at 245 nm. The mobile phase consisted of a solution containing a 0.01 g/100 g sulfuric acid concentration that was altered to a level of pH 2.6. The VC content was measured in conditions similar to the mobile phase at a flow rate of 1.0 mL/min. The TA of the cabbage slices was evaluated according to the technique suggested by Xing et al.[Citation35] Briefly, 90 mL of water was used to dilute 10 g of cabbage, which was titrated with 0.1N NaOH to pH 8.1, and expressed as a percentage of citric acid.
Total sugar and reducing sugar content
A technique involving ferricyanide oxidation was employed to measure both the total sugar and reducing sugar content.[Citation36] The intensity of the resultant ferrocyanide generated by this oxidation process was evaluated. Furthermore, Nelson’s arsenomolybdate reagent was added and measured at 515 nm. A standard curve of glucose at 100°C for 10 min was used to calculate the sugar concentrations.
Instrumental texture determination
The texture analysis of the samples was performed during storage in refrigerated conditions. Following a thawing period of 1 h at 5 °C, the samples were carefully examined. Specimens measuring 10 mm3 each, were excised from the cabbage slices. A TA.XT Plus Texture Analyzer (Stable Microsystems Ltd., UK) with the following parameters was used[Citation37]: load cell = 1 kg, pretests peed = 1.0 mm/s, test-speed = 5.0 mm/s, posttest speed = 5.0 mm/s, compression degree = 60%, time = 5.0 s, and trigger force = 0.05 N. Moreover, a standard cylindrical probe (P/0.5) was used for the texture profile analysis (TPA). Analysis of the typical TPA texture character curves rendered four textural criteria namely cohesiveness, springiness, chewiness, and hardness. Each sample was measured 20 times, and the results regarding the peak force of compression (N) were reported.
Microbiological content and sensory acceptability
The microbiological evaluation was conducted according to the technique suggested by Rojas-Graü et al.[Citation38] and Xing et al.[Citation39] A Stomacher was used to homogenize a 10 g sample cabbage slice and 90 mL sterile solution for 60 s. The 1 mL volume of the diluted sample solution was pour-plated on plate count agar (PCA) and incubated at 30°C for 24 h to obtain the total bacterial count. Furthermore, the solution was introduced onto CGA and incubated at 28°C for 3 d to obtain the yeast and mold counts.
The taste, visual appearance, and flavor of each sample were examined to determine its sensory acceptability. Cabbage slice samples were examined following a thawing period of 1 h at 5°C and randomly presented to a 14-member panel for sensory assessment. A nine-point hedonic scale was used to rate the samples (9 – excellent; 7 – very good; 5 – good; 3 – fair, and 1 – poor). A higher numerical value indicated an increase in acceptability, off-flavor, and visual appearance.[Citation40]
Statistical analysis
The data analysis in this research was conducted using SPSS13.0 software (SPSS, Inc., Chicago, IL). The mean values were calculated and reported as the mean ± S.D. (n = 3). One-way analysis of variance followed by the least significant difference (P ≤ 0.05) was performed on the data involving the texture, chlorophyll, VC, TA content, and sensory quality of the cabbage slices to determine the significant factors.
Results and discussion
Freezing curve, freezing rate, and drip loss
The freezing curve reflects the freezing characteristics of the different quick-freezing methods used on the cabbage. The freezing curves () of the cabbage during three quick-freezing processes demonstrated significantly different profiles. The curve of dry ice quick-freezing was the steepest, followed by −60°C quick-freezing, and −20°C quick-freezing. The freezing temperature plateau occurred at −0.4°C and lasted for 8 min in −60°C quick-freezing, while no visible temperature plateau appeared in quick-freezing involving dry ice and −20°C. The corresponding freezing times were 6 min, 18 min, and 128 min for the dry ice, −60°C and −20°C, respectively. Therefore, the freezing rate is the most important factor influencing the quality of frozen food,[Citation41,Citation42] while the drip loss directly quantifies the loss of salable weight and the deterioration of appearance and further facilitates surface microbial growth.[Citation43] The freezing rate and drip loss measured during the sample trial are presented in . The freezing rates and drip loss measured for the three quick-freezing processes were significantly different (P < .05). The freezing rates of dry ice quick-freezing, −60°C quick-freezing, and −20°C quick-freezing were 0.2°C/min, 2.3°C/min and 7.6°C/min, respectively. The freezing rate of dry ice was the fastest, which was 3.3 times that of −60°C and 38 times that of – 20°C, respectively. In addition, the drip loss in cabbage during dry ice quick-freezing was 0.259%, which was significantly lower than that of −60°C quick-freezing (0.3542%), and – 20°C quick-freezing (0.6128%).
Table 2. The freezing rate and drip loss of different quick-frozen cryogenic treatments
Most researchers believe that the quality of frozen foods is directly related to the size of the ice crystals, which depends on the freezing rate.[Citation44] Quick-freezing produces fine crystals that are evenly distributed both inside and outside the cells, causing minimal damage to the cellular structure, ultimately resulting in superior frozen products. However, slow-freezing produces substantial amounts of large ice crystals in the extracellular areas and causes significant damage to the tissue in which they are formed.[Citation45–Citation47] Ferreira et al.,[Citation48] confirmed that rapid freezing was beneficial for the texture of green beans. Furthermore, employing the quick-freezing method facilitates the formation of a large number of nuclei with small and evenly distributed ice crystals, therefore, resulting in less damage to the structure of the food and reducing the drip loss.[Citation49] Martino et al.[Citation50] stressed that a higher freezing rate protected against structure damage reduced the drip loss, and maintained a better quality than a lower freezing rate. Therefore, applying dry ice in conjunction with a significantly accelerated freezing rate revealed considerable advantages with regard to energy-saving characteristics and efficiency. Similarly, dry ice freezing reduced the time required for food to reach the point of maximum ice crystallization, therefore, significantly improving its quality.
Morphological characterization
Food quality can benefit significantly from the observation analysis of product microstructure, the importance of which is generally underestimated. SEM was used to observe the morphological properties of the sample surfaces to determine the impact of different freezing processes. As illustrated by (a) and A (b), the SEM photos revealed that the surface of fresh cabbage slices appeared extraordinarily smooth and the cell structure was complete. Moreover, the cells did not appear noticeably torn or irregular in shape, and no tissue distortion was observed after the blanching process, compared to the results shown in (a) and B (b). However, the images in ,e) reveal that after exposure to various quick-freezing treatments, the microparticles displayed a partially damaged cell structure, but remained structured and well-defined individual cells. This might be due to the efficacy of ice sublimation during the freeze-drying process in preparation for SEM. Moreover, the cells on the vegetable surface showed a significant collapse ()), demonstrating that the formation of large, extracellular ice crystals can be induced by the lower freezing rate, leading to textural damage.[Citation41] Compared to other freezing methods, quick-freezing processing at −20°C might be responsible for the most substantial destruction of tissue texture. Furthermore, using this method prompted extremely uneven surfaces in the cabbage slices. Therefore, dry ice quick-freezing processing seemed to be the ideal freezing method to retain the surface microstructure of the vegetables.
Figure 2. Morphology of cabbage slices treated by different freezing processing observed by SEM (a: fresh cabbage slices, b: color protecting+blanching, c: Color protecting + blanching + −20°C quick-frozen + stored at −18°C, d: Color protecting + blanching + dry ice quick-frozen + stored at −18°C, e: Color protecting + blanching + −60°C quick-frozen + stored at −18°C; a: 500×, b: 2000×)
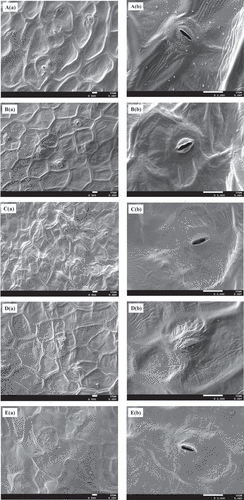
Ice formation and the related inherent challenges are responsible for mechanical damage that causes structural changes in unblanched frozen vegetables.[Citation51] These challenges specifically include the spatial modification of water transforming into ice, the dispersion of the ice in the tissue, as well as the size of the ice crystals. These factors might be responsible for damage to the membranes of the cell walls, and the pectin induced cellular connections within the tissues of the vegetables.[Citation52] Research by Charoenrein and Ireechathammawong[Citation53] indicated that a higher level of rigidity was evident in the thawed frozen-gel containing 8 g/100 g starch. This result could be ascribed to the regression of starch that occurred during the slow-freezing process. Prolonging the storage time for up to 28 d did not cause any additional modifications in the microstructure of the vegetables, which correlated with the research findings of Seetapan et al.[Citation54] The achievement of a balance between the unfrozen stage and the ice during the frozen state at −18°C could be responsible for this efficacy.[Citation55] The results from the texture examination in this study confirmed this point and indicated that the ideal freezing technique appeared to be quick-freezing processing using dry ice to ensure optimal texture retention. Dry ice quick-freezing induced the formation of a large number of small ice crystals and left a fraction of unfrozen water while freezing at −20°C led to fewer ice crystals that were larger in size.[Citation56] The low dehydration associated with small ice crystals resulted in less breakage of cell walls, and consequently, less texture deterioration compared to the slow-freezing rate.[Citation57] Therefore, the vegetables were firmer after thawing, which was indicative of an excellent cell wall structure present on the vegetable surface.[Citation58]
Residual content of total chlorophyll, VC and TA
Chlorophyll and VC are the main compounds responsible for the color of vegetables, which is a critical index for the assessment of fresh cabbage slices. As shown in ), the results indicated that the total chlorophyll content of the cabbage slices decreased with extended storage time for all treatments. Moreover, the total chlorophyll content exhibited a decline of 39.4%-87.1% in the vegetable slices during the storage period. The original chlorophyll content of the cabbage slices was approximately 2.568 mg/g. However, during the control treatment, it stabilized at around 0.3453 mg/g at the end of the storage period. On day 28, the chlorophyll content in the rest of the treated samples ranged from 0.6067 mg/g to 1.5147 mg/g, and significant differences were evident at the end of the storage period. Frozen vegetables are prone to color changes during the blanching process or frozen storage conditions.[Citation59] Furthermore, freezing using dry ice and storage at −18°C retained the quality of the microstructure and nutritional value of the sample slices. VC is not only is the main compound responsible for the color but is also a vital nutritional ingredient in frozen vegetables which is often used as an index for measuring the impact on nutrient preservation.[Citation60] As shown in ), the VC content in the cabbage slices exhibited different levels of change and was attributed to each of the four treatments. The original VC content was about 34.3 mg/100 g in the fresh slices, while it decreased dramatically during the first 7 d, and remained relatively constant during the final 21 d. After 28 d of storage, the VC content in the samples processed via −20°C freezing, dry ice freezing, and −60°C freezing was 21.4 mg/100 g, 26.2 mg/100 g and 24.0 mg/100 g, respectively. However, the VC content in the control samples and the blanched slices were only 12.6 mg/100 g and 16.3 mg/100 g, respectively. The VC levels were higher in the samples treated with blanching + freezing and blanching than those in the control sample. According to Sidonia Martínez et al.[Citation61], this fact, combined with the extraction of the acids, do not reflect an increase in pH due to the neutralization of the acids by other extracted components. Furthermore, the TA was used as a quality parameter and was related to the presence of organic acid concentrations. According to the results shown in ), the TA of the fresh cabbage slices were between 0.3287% and 0.3393%, while the TA values of all the samples exhibited a decline during each of the storage periods. Although all the control samples displayed elevated levels of TA on the seventh day, it was still significantly lower than in the treated samples. However, relatively small changes were observed in the TA levels during storage and freezing treatment. At the end of storage, the TA levels for the −20°C freezing, dry ice freezing, and −60°C freezing were 38.8%, 20.2%, and 28.7% lower than that at the beginning of storage. The highest TA levels were found in the samples with the combined treatment of color protection + blanching + dry ice quick-freezing + storage at −18°C and were 0.2623% at the end of storage.
Figure 3. Effects of different quick-frozen cryogenic preservation treatments on total chlorophyll, VC and TA of purple cabbage slices during storage (■, Control; ●, Color protecting+blanching; ▲, Color protecting + blanching + −20°C quick-frozen + stored at −18°C; ▼, Color protecting + blanching + dry ice quick-frozen + stored at −18°C; ◆, Color protecting + blanching + −60°C quick-frozen + stored at −18°C)
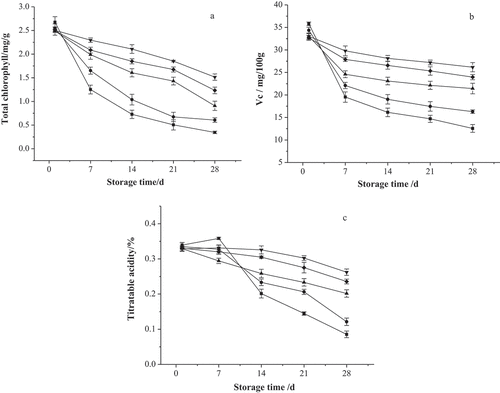
These results indicated the significant degradation of the chlorophyll content in the processed samples during storage, compared with fresh cabbage slices. This degradation might be attributed to the blanching process, the transformation of chlorophyll and deaeration.[Citation62–Citation64] Blanching promotes the breakdown of the organelles that contain chlorophyll, causing them to spread within the cell, making the pigment more susceptible to degradation.[Citation25] Several studies reported that the deterioration of chlorophyll is restricted by thermal passivity.[Citation63] In this work, processing the blanched samples by quick-freezing them at −18°C for 60 s, induced the degradation of the chlorophyll (39.4%). These results corresponded with the findings of the investigation conducted by Muftugil.[Citation65] The study indicated that the loss of chlorophyll content in fresh broad bean samples could be due to blanching treatments with hot water. Moreover, this loss could increase by extending the treated time. Kmiecik et al.[Citation66] also confirmed that exposure to the heat of cooking or blanching could be responsible for the loss of chlorophyll in three types of brassicas. However, frozen storage significantly inhibited degradation. After five months of frozen storage, 31% and 34% total chlorophyll deterioration was evident in minimally blanched beans and over-blanched bean samples, respectively. Oruña-Concha et al.[Citation67] studied green beans and found that frozen storage contributed significantly to the degradation of the chlorophyll, which became particularly evident during the initial two months of storage. In addition, Kmiecik et al.[Citation66] indicated that a gradual decline in the chlorophyll content of frozen brassicas could occur during storage conditions with temperatures of −20°C and −30°C, respectively. Samples treated over a short time with a high temperature retained a larger amount of chlorophyll than those treated with a low temperature over a longer time.[Citation68] This result could be attributed the combined action of blanching and freezing. The freezing treatment inhibited the enzymatic activity, restricting the degradation rate of VC,[Citation69] and could be ascribed to enzymatic (via ascorbic acid oxidase) oxidation in the presence of oxygen. In frozen products, enzymatic reactions are slow but are not completely eliminated since enzymatic reactions still occur in the presence of non-frozen water.[Citation70] Furthermore, the results of Hunter and Fletcher[Citation71] indicated that the blanching step might explain the significantly diminished quantities of VC content. The losses during hot water blanching could probably be ascribed to leaching and the thermal degradation of ascorbic acid to dehydroascorbic acid, as well as further oxidation.[Citation72] However, it should also be noted that since it is a water-soluble compound, part of the VC content may be lost through drip loss.[Citation73–Citation75] As reported by Martins and Silva[Citation76], the quality of beans was retained during frozen storage. Evidence indicated that the VC content in the cabbage slices could be protected by the application of ascorbic acid and citric acid that act as anti-browning elements.[Citation38,Citation77] Furthermore, several researchers concur that storage caused the gradual loss of VC content in blanched vegetables.[Citation78] The TA content in raw samples might be modified during frozen storage due to hydrolytic enzymes that remain active, causing physicochemical changes. The enzymes in the sample tissues were deactivated by blanching the vegetables or by adding an acidulant. However, the samples blanched in water containing 0.1 g of citric acid/100 ml caused a minimal elevation in the TA content of the vegetable slices during storage. This was consistent with the results of the investigation conducted by Lisiewska and Kmiecik,[Citation19] who observed that the TA content in frozen tomato cubes increased during storage.
Total sugar and reducing sugar
Water-soluble total sugar and reducing sugar content in fresh vegetables are essential quality indicators. The effect of freezing treatments on the total sugar and reducing sugar content in cabbage during storage was investigated. As shown schematically in , significant differences (P ≤ 0.05) were evident from day 14 in both the total sugar and reducing sugar content of the cabbage slices when subjected to different treatments. This phenom enon was primarily caused by the disintegration or decay of sucrose and was likely the result of enzymatic activity in the samples.[Citation69] Moreover, the freezing treatments produced ice crystals, which might degrade the cell structure of the product and, therefore, facilitate the extraction of sugars.[Citation56] It was initially discovered that the total sugars ranged from 15.5 g/100 g to 17.1 g/100 g in the cabbage slices exposed to different treatments (), while the reducing sugar content was between 11.3 g/100 g and 11.6 g/100 g, which comprised 60% to 80% of the total sugar content. The cabbage slices in the treated samples labeled 3, 4, and 5 showed a high sugar content after being submerged in a color-protecting solution. Moreover, blanching was also found to increase the sugar content. The total sugar content of samples that were quick-frozen using dry ice and stored at −18 °C did not change significantly at the 14 d mark. Zhao et al. observed similar changes,[Citation79] indicating that the reducing sugars (fructose and glucose) in date fruits gradually declined during a twelve-month frozen storage period. Another study discovered a decrease in reducing sugars in green beans when stored in frozen conditions.[Citation80] Dry ice quick-freezing treatment and storage at −18°C significantly decreased the loss of the total sugar content, as well as reducing sugar content of the cabbage slices.
Table 3. Effects of different quick-frozen cryogenic preservation treatments on the content of total sugars and reducing sugars of purple cabbage slices
Instrumental texture
The TPA values of all the cabbage slices declined in varying degrees when the storage time was increased (). The control samples and treatment samples were compared, and the results revealed a considerable decline in the firmness during the cold storage period ()). Following a 28 d storage period, the springiness in the cabbage slices of the control sample decreased by 87.09%. Furthermore, quick-freezing conditions involving −20°C, dry ice, and −60°C, caused the samples to display respective values of 63.55%, 39.44%, and 50.57% ()). Cabbage slices exposed to a long storage time lost between 18.35% and 77.17% of their original chewiness by day 28. Moreover, dry ice quick-freezing, and −60°C quick-freezing facilitated the optimal retention in chewiness, with values of 14.542 N mm and 13.219 N mm, respectively, at the end of the cold storage time ()). Furthermore, the cohesiveness exhibited a rapid decline in the control samples from the original value of 6.755 to 0.3833 on day 60, while the cohesiveness of the frozen samples showed a minimal reduction from 6.545 to 1.795–3.363 at the end of cold storage ()). Moreover, all TPA values in the cabbage slices that were quick-frozen using dry ice were higher than those treated with the −60°C quick-freezing and −20°C quick-freezing methods. These results were obtained even though the texture parameters were lower in all treatments. Additionally, a TPA of the control samples could not be performed due to severe degeneration.
Figure 4. Effects of different quick-frozen cryogenic preservation treatments on TPA values of purple cabbage slices during storage (■, control; ●, color protecting + blanching; ▲, color protecting + blanching + −20 °C quick-frozen + stored at −18 °C; ▼, color protecting + blanching + dry ice quick-frozen + stored at −18 °C; ◆, color protecting + blanching + −60 °C quick-frozen + stored at −18 °C)
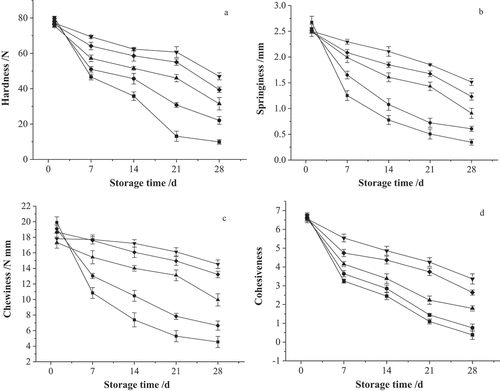
The softening of fruit is caused by a gel that forms when osmotic pressure introduces pectin into the cell sap.[Citation81] Previous studies revealed that textural changes in the tissue of fruit during storage could be ascribed to middle lamella structure decay and primary cell wall deterioration.[Citation82] Xu et al.[Citation83] indicated that extended cooking did not eliminate the enhanced texture of carrot slices obtained by a rapid freezing process using liquid nitrogen and other cold liquids. In this study, the results indicated that the blanching and freezing treatments might delay the softening in samples by reducing the loss of water and restricting the activity of enzymes responsible for cell wall deterioration. The likely cause for this was the reduction in the damaging effects of crystallization and recrystallization on the microstructure of the cabbage tissue during quick-freezing, frozen storage, and thawing.[Citation84] Therefore, the ice crystals that formed rapidly during quick-freezing can then grow faster during thawing. As molecular mobility increased, the migratory recrystallization of ice enabled the crystallization of residual water that is kinetically inhibited during cooling.[Citation56] The investigation of Grayson et al.[Citation85], found that quick-freezing rather than blast-freezing improved the consistency of meat tenderness. Water loss was responsible for the decline in turgor pressure in the cells and led to changes in fruit firmness.[Citation86,Citation87] Research indicated that the Ca2+ absorbed from water or released from cell walls is transferred to the middle lamella or the cell wall. This process is followed by cross-linking with carboxyl pectin groups.[Citation88]
Microbiological content
Freezing prevents microbial growth and is, therefore, an excellent way to extend the shelf life of fruits and vegetables.[Citation89] and respectively illustrate the development of molds, bacteria, and yeast on the cabbage slices during the cold storage period at −18°C. The results indicated that the total number of bacteria that were present in all samples was below 3.2 × 102 CFU/g on day 0 (). However, the viable count was reduced by freezing and continued to decline during the frozen storage period.[Citation90] Hazen et al.[Citation91] discovered that MAB and yeast populations declined during the first three months of frozen storage while displaying a slight increase during the subsequent three months. The total bacteria in the cabbage slices reached levels of 5.1 × 108 and 8.5 × 104, 2.0 × 104 and 4.4 × 104 CFU/g, whereas yeast and molds reached 8.8 × 106 and 3.5 × 103, 1.0 × 103 and 2.9 × 103 CFU/g, on the 28th day for the control samples, as well as the samples exposed to −20°C quick-freezing, dry ice quick-freezing, and −60°C quick-freezing treatments, respectively. According to the microbiological indexes of vegetables stipulated in GB 4789.23, the total bacterial colonies ≤ 5.0 × 104 cfu/g, coliform colonies ≤ 1.5 mpn/g, and pathogenic bacteria should not be detected.[Citation92]
Table 4. Effects of different quick-frozen cryogenic preservation treatments on the total number of bacteria of cabbage slices
Table 5. Effects of different quick-frozen cryogenic preservation treatments on the total number of yeast and mold of cabbage slices
The results of this study indicated additional microbial inactivity during freezing, which can probably be ascribed to a higher vulnerability to sub-lethal damage due to being exposed to ClO2. When microorganisms are subjected to environmental stress such as freezing, some cells may exhibit no detrimental effects, some are destroyed, and some may experience a sub-lethal or metabolic injury. In a nonselective environment, injured cells repaired their damage, and then proceeded with growth and multiplication.[Citation93,Citation94] However, some psychrophilic bacteria and yeasts can grow at temperatures as low as −7.5 and −10°C, respectively.[Citation95] Furthermore, extracellular solute concentrations, intracellular solute toxicity, temperature shock, ice crystal production, and dehydration are all factors that have a destructive impact on specimens during the freezing process.[Citation86] The efficacy of cryogenic quick-freezing preservation on the growth of mesophilic aerobic bacteria, yeast, and mold, were observed in blueberries and shrimp products.[Citation95,Citation96] According to the investigation of Hazen et al.[Citation90], freezing extended the shelf life of blueberries by preventing microbial growth. A study conducted by Crowe et al.[Citation97] indicated a decline in the number of mesophilic aerobic bacteria, yeast and mold that were present in wild blueberries that remained either untreated or were exposed to 100 ppm free chlorine or 1 ppm zone, and fast-frozen at −30°C followed by a storage period of 12 months at −18°C. More importantly, freezing has an additional lethal effect on microorganisms related to temperature shock, the concentration of extracellular solutes, the toxicity of intracellular solutes, dehydration, and the formation of large ice crystals during freezing.
Sensory acceptability
The concept of sensory acceptability was used to comprehensively evaluate vegetables by appearance, taste, flavor, and intensity.[Citation40] Therefore, the effects of cryogenic quick-freezing preservation treatment on the sensory acceptability of cabbage slices were investigated. As shown in , freezing treatment postponed the emergence of surface deterioration, in comparison to the control samples. The sensory evaluation values of samples treated with color protection + blanching + −20°C quick-freezing, color protection + blanching + dry ice quick-freezing, and color protection + blanching + −60°C quick-freezing following storage at −18°C for 28 d, were 4.0, 6.4 and 5.2, respectively. The values for the rest of the treatments ranged between 2.4 and 5.3 and did not display significant differences. In addition, the samples treated with the dry ice quick-freezing method were still green, while the control samples only had a value of 1.0 and became unacceptable. The samples treated with the color protection + blanching method exhibited reduced sensory acceptability following storage at −18°C for 28 d, while the sensory evaluation of the samples was valued at 2.2.
Figure 5. Effects of different quick-frozen cryogenic preservation treatments on sensory acceptability of Purple Cabbage Slices during storage (■, Control; ●, Color protecting+blanching; ▲, Color protecting + blanching + −20°C quick-frozen + stored at −18°C; ▼, Color protecting + blanching + dry ice quick-frozen + stored at −18°C; ◆, Color protecting + blanching + −60°C, quick-frozen + stored at −18°C)
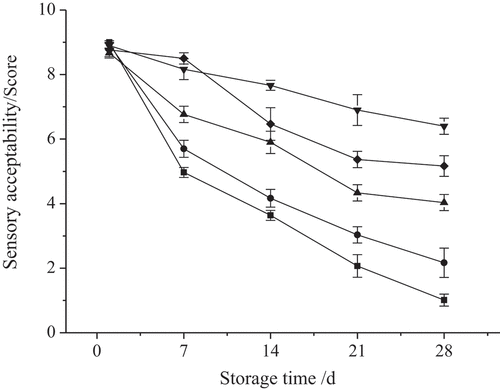
Compared to unstored samples, the color changes for all the frozen cabbage slices were negligible. However, at the conclusion of the storage period, the frozen samples remained green. At lower storage temperatures, the color may be stabilized by the formation of metal-chlorophyll compounds, but the chlorophyll content does not provide a reliable prediction of color retention.[Citation28] Research by Guillermo Petzold et al.[Citation25] indicated that a texture evaluation of cooked beans showed an insignificant decline in samples that were minimally blanched for 120 s, and a significant deterioration in samples that were over-blanched for 180 s compared to unblanched beans. Therefore, the quality of frozen food more closely resembled that of its fresh counterparts since the diameter of the ice crystals declined in conjunction with increased freezing rates.[Citation98] Specifically, the integrity of the protein network was preserved by low storage temperatures such as −35°C and −40°C and the formation of numerous small ice crystals was promoted.[Citation99] These results could be attributed to improved crystal growth rates and enhanced nucleation, as well as the significant antifungal activity of cinnamaldehyde.[Citation100] However, although most of the microorganisms that are responsible for spoilage are unable to multiply and grow to cause pathogenicity when frozen, they remain dormant. Compared to thermal processing, freezing remains an ideal technique to preserve food since it produces a product exhibiting optimal physicochemical, sensory, and microbiological properties.[Citation101]
Conclusion
In conclusion, the quick-freezing treatment combined with cryogenic preservation can maintain the quality of cabbage slices, and control the progressing of decay. The cabbage slices subjected to color protection + blanching + dry ice quick-freezing + stored at −18°C treatment display the lowest number of changes in the texture values and sensory acceptability, while the chlorophyll, VC, and TA content exhibit minimal changes. The combined treatment substantially impedes the degradation of both total sugar and reducing sugar during the specific storage period. Microbial analysis indicates that the color protection + blanching + dry ice quick-freezing + stored at −18°C treatment is effective in hindering the growth of bacteria, yeast, and mold. In addition, compared to other processed specimens, the samples that are quick-frozen using dry ice display an optimum surface microstructure. This study shows that the application of the dry ice quick-freezing method followed by storage at −18°C is an excellent technique for the maintenance of the quality and microbiological safety of cabbage slices.
Additional information
Funding
References
- Zhang, X. L.; Su, Y. B.; Liu, Y. M.; Fang, Z. Y.; Yang, L. M.; Zhuang, M.; Zhang, Y. Y.; Li, Z. S.; Lv, H. H. Genetic Analysis and QTL Mapping of Traits Related to Head Shape Incabbage (Brassica Oleracea Var. Capitata L.). Sci. Hortic. 2016, 207, 82–88.
- Nosek, M.; Surówka, E.; Cebula, S.; Libik, A.; Goraj, S.; Kornas, A.; Miszalski, Z. Distribution Pattern of Certain Antioxidants in White Cabbage Heads (Brassica Oleracea L. Var. Capitata F. alba). Acta Physiol. Plant. 2011, 33, 2125–2134. DOI: 10.1007/s11738-011-0752-6.
- Singh, B. K.; Sharma, S. R.; Singh, B. Combining Ability for Superoxide Dismutase, Peroxidase and Catalase Enzymes in Cabbage Head (Brassica Oleracea Var. Capitata L.). Sci. Hortic. 2009, 122, 195–199. DOI: 10.1016/j.scienta.2009.05.012.
- Maggio, A.; De Pascales, S.; Ruggiero, C.; Barbieri, G. Physiological Response of Field-grown Cabbage to Salinity and Drought Stress. Eur. J. Agron. 2005, 23, 57–67. DOI: 10.1016/j.eja.2004.09.004.
- Khattab, H.;. The Defense Mechanism of Cabbage Plant against Phloem-sucking Aphid (Brevicoryne brassicae). Aust. J. Basic Appl. Sci. 2007, 1, 56–62.
- Xing, Y.; Li, X.; Xu, Q.; Yun, J.; Lu, Y.; Tang, Y. Effects of Chitosan Coating Enriched with Cinnamon Oil on Qualitative Properties of Sweet Pepper (Capsicum Annuum L.). Food Chem. 2011, 124, 1443–1450. DOI: 10.1016/j.foodchem.2010.07.105.
- Darfouroduro, S. A.; Buchner, D. M.; Andrade, J. E.; Grigsby-Toussaint, D. S. A Comparative Study of Fruit and Vegetable Consumption and Physical Activity among Adolescents in 49 Low-And-Middle-Income Countries. Sci. Rep. 2018, 8, 1623. DOI: 10.1038/s41598-018-19956-0.
- Barrett, D. M.; Garcia, E. L.; Russell, G. F.; Ramirez, E.; Shirazi, A. Blanchtime and Cultivar Effects on Quality of Frozen and Stored Corn and Broccoli. J. Food Sci. 2000, 65, 534–540. DOI: 10.1111/jfds.2000.65.issue-3.
- Zambounis, A.; Obeidant, I. N.; Tsaftaris, A. Cloning of Cu/Zn SOD Gene in Pepper for Stress Tolerance. Acta Hortic. 2002, 579, 101–105. DOI: 10.17660/ActaHortic.2002.579.13.
- Yiu, J. C.; Tseng, M. J. Manipulation of Superoxide Dismutase and Catalase to Enhance Sulfur Dioxide Tolerance in Transgenic Chinese Cabbage. Acta Hortic. 2005, 692, 91–99. DOI: 10.17660/ActaHortic.2005.692.11.
- Xu, Q. L.; Xing, Y. G.; Che, Z. M.; Guan, T. W.; Zhang, L.; Bai, Y. M.; Gong, L. Effect of Chitosan Coating and Oil Fumigation on the Microbiological and Quality Safety of Fresh-cut Pear. J. Food Safety. 2013, 33, 179–189. DOI: 10.1111/jfs.12038.
- Petersen, O. H.; Spat, A.; Verkhratsky, A. Introduction: Reactive Oxygen Species in Health and Disease. Philos. T. R. Soc. B. 2005, 360, 2197–2199. DOI: 10.1098/rstb.2005.1776.
- Ganthavorn, C.; Powers, J. R. Changes in Peroxidase Activity, Hexanal, Ascorbic Acid and Free Sulfhydryl in Blanched Asparagus during Frozen Storage. J. Food Sci. 1988, 53, 1403–1405. DOI: 10.1111/jfds.1988.53.issue-5.
- Canet, W.; Alvarez, M. D.; Fernandez, C.; Tortosa, M. E. The Effect of Sample Temperature on Instrumental and Sensory Properties of Mashed Potato Products. Int. J. Food Sci. Tech. 2005, 40, 481–493. DOI: 10.1111/ifs.2005.40.issue-5.
- Petzold, G.; Aguilera, J. M. Ice Morphology: Fundamentals and Technological Applications in Foods. Food Biophys. 2009, 4, 378–396. DOI: 10.1007/s11483-009-9136-5.
- Pita-Calvo, C.; Guerra-Rodríguez, E.; Saraiva, J. A.; Aubourg, S. P.; Vázquez, M. Effect of High-pressure Processing Pretreatment on the Physical Properties and Colour Assessment of Frozen European Hake (Merluccius merluccius) during Long Term Storage. Food Res. Int. 2018, 112, 233–240. DOI: 10.1016/j.foodres.2018.06.042.
- Xin, C.; Nie, L.; Chen, H.; Li, J.; Li, B. Effect of Degree of Substitution of Carboxymethyl Cellulose Sodium on the State of Water, Rheological and Baking Performance of Frozen Bread Dough. Food Hydrocolloid. 2018, 80, 8–14. DOI: 10.1016/j.foodhyd.2018.01.030.
- Parisi, S.;. The World of Foods and Beverages Today. Online Video Course, Learning; The Economist Group, 750 Third Ave NY, NY, 10017, 2016.
- Lisiewska, Z.; Kmiecik, W. Effect of Storage Period and Temperature on the Chemical Composition and Organoleptic Quality of Frozen Tomato Cubes. Food Chem. 2000, 70, 167–173. DOI: 10.1016/S0956-7135(99)00110-3.
- Cai, C.; Miao, H.; Qian, H.; Yao, L.; Wang, B.; Wang, Q. Effects of Industrial Pre-Freezing Processing and Freezing Handling on Glucosinolates and Antioxidant Attributes in Broccoli Florets. Food Chem. 2016, 210, 451–456. DOI: 10.1016/j.foodchem.2016.04.140.
- Hawkins, L. A.;. The Effect of Low-Temperature Storage and Freezing on Fruits and Vegetables. Am. J. Bot. 1922, 9, 551–556. DOI: 10.1002/j.1537-2197.1922.tb05694.x.
- Tano, K.; Mathias, K.; Oulé, D.; Lencki, G.; Arul, R. W. Comparative Evaluation of the Effect of Storage Temperature Fluctuation on Modified Atmosphere Packages of Selected Fruit and Vegetables. Postharvest Biol. Tec. 2007, 46, 212–221. DOI: 10.1016/j.postharvbio.2007.05.008.
- Salunkhe, D. K.; Wu, M. T.; Rahman, A. R. Developments in Technology of Storage and Handling of Fresh Fruits and Vegetables. Crit. Rev. Food Sci. 1974, 5, 15–54.
- Gorny, J. R.; Hess-Pierce, B.; Cifuentes, R. A.; Kader, A. A. Quality Changes in Fresh-cut Pear Slices as Affected by Controlled Atmospheres and Chemical Preservatives. Postharvest Biol. Tec. 2002, 24, 271–278. DOI: 10.1016/S0925-5214(01)00139-9.
- Petzold, G.; Caro, M.; Moreno, J. Influence of Blanching, Freezing and Frozen Storage on Physicochemical Properties of Broad Beans (Vicia Faba L). Int. J. Refrig. 2014, 40, 429–434. DOI: 10.1016/j.ijrefrig.2013.05.007.
- Liu, F.; Wang, F.; Wang, Z.; Luo, X.; Gu, Z. Numerical Simulation on Flat Finned-tube Condenser of Diffusion Absorption Refrigerator. Refrig. Air Conditioning. 2014, 14, 44–47.
- Liang, D.; Lin, F.; Yang, G.; Yue, X.; Zhang, Q.; Zhang, Z.; Chen, H. Advantages of Immersion Freezing for Quality Preservation of Litchi Fruit during Frozen Storage. LWT-Food Sci. Technol. 2015, 60, 948–956. DOI: 10.1016/j.lwt.2014.10.034.
- Bulut, M.; Bayer, Ö.; Kırtıl, E.; Bayındırlı, A. Effect of Freezing Rate and Storage on the Texture and Quality Parameters of Strawberry and Green Bean Frozen in Home Type Freezer. Int. J. Refrig. 2018, 88, 360–369. DOI: 10.1016/j.ijrefrig.2018.02.030.
- Kaale, L. D.; Eikevik, T. M.; Rustad, T.; Nordtvedt, T. S. Changes in Water Holding Capacity and Drip Loss of Atlantic Salmon (Salmo salar) Muscle during Superchilled Storage. LWT-Food Sci. Technol. 2014, 55, 528–535. DOI: 10.1016/j.lwt.2013.10.021.
- Pralhad, T.; Rajendrakumar, K. Study of Freeze-dried Quercetin-cyclodextrin Binary Systems by DSC, FT-IR, X-ray Diffraction and SEM Analysis. J. Pharmaceut. Biomed. 2004, 34, 333–339. DOI: 10.1016/S0731-7085(03)00529-6.
- Ganatsios, V.; Terpou, A.; Gialleli, A.-I.; Kanellaki, M.; Bekatorou, A.; Koutinas, A. A. A Ready-to-use Freeze-dried Juice and Immobilized Yeast Mixture for Low Temperature Sour Cherry (Prunus cerasus) Wine Making. Food Bioprod. Process. 2019, 117, 373–379. DOI: 10.1016/j.fbp.2019.08.009.
- Albanese, D.; Russo, L.; Cinquanta, L.; Brasiello, A.; Di Matteo, M. Physicaland Chemical Changes in Minimally Processed Green Asparagus during Cold-storage. Food Chem. 2007, 101, 274–280. DOI: 10.1016/j.foodchem.2006.01.048.
- Duthie, S. J.; Duthie, G. G.; Russell, W. R.; Kyle, J. A. M.; Macdiarmid, J. I.; Rungapamestry, V.; Stephen, S.; Baeza, C. M.; Kaniewska, J. J.; Shaw, L.; et al. Effect of Increasing Fruit and Vegetable Intake by Dietary Intervention on Nutritional Biomarkers and Attitudes to Dietary Change: A Randomised Trial. Eur. J. Nutr. 2018, 57, 1855–1872. DOI: 10.1007/s00394-017-1469-0.
- Xing, Y.; Lin, H.; Cao, D.; Xu, Q.; Han, W.; Wang, R.; Che, Z.; Li, X. Effect of Chitosan Coating with Cinnamon Oil on the Quality and Physiological Attributes of China Jujube Fruits. Biomed Res. Int. 2015, Article ID 83515, 10 pages.
- Xing, Y.; Xu, Q.; Lin, H.; Li, X.; Zhang, D.; Yang, X.; Meng, Y.; Li, X.; Liu, X. Preparation, Properties and in Vivo Antimicrobial Activity in Yacon Roots of Microencapsulation Containing Cinnamon Oil. Mater. Technol. 2016, 31, 40–46. DOI: 10.1080/10667857.2016.1160502.
- Ranganna, S.;. Handbook of Analysis and Quality Control for Fruit and Vegetable Products; Tata Mc Graw-Hill Publishing Co: New Delhi, 1986; pp 838–865.
- Birch, D.; Memery, J.; Johns, N.; Musarskaya, M. Stimulating UK Adolescents’ Seafood Consumption. J. Int. Food Agribusiness Marketing. 2018, 30, 1–9. DOI: 10.1080/08974438.2017.1382423.
- Rojas-Graü, M. A.; Tapia, M. S.; Martín-Belloso, O. Using Polysaccharide- Based Edible Coatings to Maintain Quality of Fresh-cut Fuji Apples. Lebensm. Wiss. Technol. 2008, 41, 139–147. DOI: 10.1016/j.lwt.2007.01.009.
- Xing, Y.; Li, X.; Yun, J.; Lu, Y. Extending the Shelf-life of Fresh-cut Lotus Root with Anti Browning Agents,cinnamon Oil Fumigation, and Moderate Vacuum Packaging (MVP). J. Food Process Eng. 2012b, 35, 505–521. DOI: 10.1111/j.1745-4530.2010.00604.x.
- Chien, P. J.; Sheu, F.; Yang, F. H. Effect of Edible Chitosan on Quality and Shelf Life of Sliced Mango Fruit. J. Food Eng. 2007, 78, 225–229. DOI: 10.1016/j.jfoodeng.2005.09.022.
- Sanz, P. D.; Elvira, C.; Martinob, M.; Zaritzkyb, N.; Oteroa, L.; Carrascoa, J. A. Freezing Rate Simulation as an Aid to Reducing Crystallization Damage in Foods. Meat Sci. 1999, 52, 275–278. DOI: 10.1016/S0309-1740(99)00002-9.
- Sun, D.; Li, B. Microstructural Change of Potato Tissues Frozen Byultrasound-assisted Immersion Freezing. J. Food Eng. 2003, 57, 337–345. DOI: 10.1016/S0260-8774(02)00354-0.
- Duun, A. S.; Rustad, T. Quality of Superchilled Vacuum Packed Atlantic salmon(Salmo salar) Fillets Stored at −1.4 And −3.6 °C. Food Chem. 2008, 106, 122–131. DOI: 10.1016/j.foodchem.2007.05.051.
- Olivera, D. F.; Salvadori, V. O. Effect of Freezing Rate in Textural and Rheological Characteristics of Frozen Cooked Organic Pasta. J. Food Eng. 2009, 90, 271–276. DOI: 10.1016/j.jfoodeng.2008.06.041.
- Delgado, A. E.; Zheng, L. Y.; Sun, D. W. Influence of Ultrasound on Freezing Rate of Immersion-frozen Apples. Food Bioprocess. Tech. 2009, 2, 263–270. DOI: 10.1007/s11947-008-0111-9.
- Holzwarth, M.; Korhummel, S.; Carle, R.; Kammerer, D. R. Evaluation of the Effects of Different Freezing and Thawing Methods on Color, Polyphenol and Ascorbic Acid Retention in Strawberries (Fragaria Ananassa duch.). Food Res. Int. 2012, 48, 241–248. DOI: 10.1016/j.foodres.2012.04.004.
- Yu, S.; Ma, Y.; Zheng, X.; Liu, X.; Sun, D. W. Impacts of Low and Ultra-low Temperature Freezing on Retrogradation Properties of Rice Amylopectin during Storage. Food Bioprocess. Tech. 2012, 5, 391–400. DOI: 10.1007/s11947-011-0526-6.
- Ferreira, A.; Canet, W.; Alvarez, M. D.; Tortosa, M. E. Freezing, Thawing and Cooking Effects on Quality Profile Assessment of Green Beans (Cv. win). Eur. Food Res. Technol. 2006, 223, 433–445. DOI: 10.1007/s00217-005-0217-5.
- Jia, R.; Jiang, Q.; Kanda, M.; Tokiwa, J.; Nakazawa, N.; Osako, K.; Okazaki, E. Effects of Heating Processes on Changes in Ice Crystal Formation, Water Holding Capacity, and Physical Properties of Surimi Gels during Frozen Storage. Food Hydrocolloid. 2019, 90, 254–265. DOI: 10.1016/j.foodhyd.2018.12.029.
- Martino, M. N.; Otero, L.; Sanz, P. D.; Zaritzky, N. E. Size and Location of Ice Crystals in Pork Frozen by High-pressure-assisted Freezing as Compared to Classical Methods. Meat Sci. 1998, 50, 303–313. DOI: 10.1016/S0309-1740(98)00038-2.
- Neri, L.; Hernando, I.; Pérez-Munuera, I.; Sacchetti, G.; Mastrocola, D.; Pittia, P. Mechanical Properties and Microstructure of Frozen Carrots during Storage as Affected by Blanching in Water and Sugar Solutions. Food Chem. 2014, 144, 65–73. DOI: 10.1016/j.foodchem.2013.07.123.
- Roy, S. S.; Taylor, T. A.; Kramer, H. L. Textural and Ultrastructural Changes in Carrot Tissue as Affected by Blanching and Freezing. J. Food Sci. 2001, 66, 176–180. DOI: 10.1111/j.1365-2621.2001.tb15602.x.
- Charoenrein, S.; Ireechathammawong, N. Undercooling Associated with Slow Freezing and Its Influence on the Microstructure and Properties of Rice Starch Gels. J. Food Gng. 2010, 100, 310–314. DOI: 10.1016/j.jfoodeng.2010.04.014.
- Seetapan, N.; Limparyoon, N.; Gamonpilas, C.; Methacanon, P.; Fuongfuchat, A. Effect of Cryogenic Freezing on Textural Properties and Microstructure of Rice flour/tapioca Starch Blend Gel. J. Food Eng. 2015, 151, 51–59. DOI: 10.1016/j.jfoodeng.2014.11.025.
- Lee, S.; Cornillons, P.; Kim, Y. R. J. F. S. Spatial Investigation of the Non-Frozen Water Distribution in Frozen Foods Using NMR SPRITE. J. Food Sci. 2002, 67, 2251–2255. DOI: 10.1111/jfds.2002.67.issue-6.
- Chassagne-Berces, S.; Fonseca, F.; Citeau, M.; Marin, M. Freezing Protocol Effect on Quality Properties of Fruit Tissue according to the Fruit, the Variety and the Stage of Maturity. LWT-Food Sci. Technol. 2010, 43, 0–1449. DOI: 10.1016/j.lwt.2010.04.004.
- Mazur, P.;. Freezing of Living Cells: Mechanisms and Implications. Am. J. Physiolo. Cell Ph. 1984, 247, 125–142. DOI: 10.1152/ajpcell.1984.247.3.C125.
- Ando, H.; Kajiwara, K.; Oshita, S.; Suzuki, T. The Effect of Osmotic Dehydrofreezing on the Role of the Cell Membrane in Carrot Texture Softening after Freeze-thawing. J. Food Eng. 2012, 108, 473–479. DOI: 10.1016/j.jfoodeng.2011.08.013.
- Alvarez-Jubete, L.; Valverde, J.; Patras, A.; Maria, A.; Marcos, M. B. Assessing the Impact of High-Pressure Processing on Selected Physical and Biochemical Attributes of White Cabbage. Food Bioprocess. Tech. 2014, 7, 682–692. DOI: 10.1007/s11947-013-1060-5.
- Xing, Y.; Xu, Q.; Che, Z.; Li, X.; Li, W. Effects of Chitosan-oil Coating on Blue Mold Disease and Quality Attributes of Jujube Fruits. Food Funct. 2011, 2, 466–474. DOI: 10.1039/c1fo10073d.
- Martínez, S.; Pérez, N.; Carballo, J.; Franco, I. Effect of Blanching Methods and Frozen Storage on Some Quality Parameters of Turnip Greens (“grelos”). LWT-Food Sci. Technol. 2013, 51, 383–392. DOI: 10.1016/j.lwt.2012.09.020.
- Bahçeci, K. S.; Serpen, A.; Gökmen, V.; Acar, J. Study of Lipoxygenase and Peroxidase as Indicator Enzymes in Green Beans: Change of Enzyme Activity, Ascorbic Acid and Chlorophylls during Frozen Storage. J. Food Eng. 2005, 66, 187–192. DOI: 10.1016/j.jfoodeng.2004.03.004.
- Lisiewska, Z.; Kmiecik, W.; S1upski, J. Contents of Chlorophylls and Carotenoids in Frozen Dill: Effect of Usable Part and Pre-treatment on the Content of Chlorophylls and Carotenoids in Frozen Dill (Anethum graveolensL.), Depending on the Time and Temperature of Storage. Food Chem. 2004, 84, 511–518. DOI: 10.1016/S0308-8146(03)00265-6.
- Murcia, M. A.; López-Ayerra, B.; Martínez-Tomé, M.; García-Carmona, F. Effect of Industrial Processing on Chlorophyll Content of Broccoli. J. Sci. Food Agr. 2000, 80, 1447–1451. DOI: 10.1002/1097-0010(200008)80:10<1447::AID-JSFA670>3.0.CO;2-X.
- Muftugil, N.;. Effect of Blanching and Refrigerated Storagetemperature on the Quality of Frozen Broad Beans. Int. J. Refrig. 1985, 8, 236–239. DOI: 10.1016/0140-7007(85)90122-7.
- Kmiecik, W.; Lisiewska, Z.; Slupski, J.; Gebczynski, P. The Effect of Pre-treatment, Temperature and Length of Frozen Storage on the Retention of Chlorophylls in Frozen Brassicas. Acta Sci. Pol. Technol. Aliment. 2008, 7, 21–34.
- Oruña-Concha, M. J.; Gonza lez-Castro, M. J.; Lopez-Hernandez, J.; Simal- Lozano, J. Effects of Freezing on the Pigment Content in Green Beans and Padròn Peppers. Z. Lebensm. Unters. Forsch. A. 1997, 205, 148–152. DOI: 10.1007/s002170050143.
- Elke, A.; Belitz, H.-D.; Grosch, W. Schieberle: Food Chemistry, Third Edition. Anal. Bioanal. Chem. 2005, 382, 10–11. DOI: 10.1007/s00216-004-3036-9.
- Alhamdan, A.; Hassan, B.; Alkahtani, H.; Abdelkarim, D.; Younis, M. Freezing of Fresh Barhi Dates for Quality Preservation during Frozen Storage. Saudi J. Biol. Sci. 2016, 25, 1552–1561. DOI: 10.1016/j.sjbs.2016.02.003.
- Bilbao-Sainz, C.; Sinrod, A.; Powell-Palm, M.; Dao, L.; Takeoka, G.; Williams, T.; Wood, D.; Ukpai, G.; Aruda, J.; Bridges, D. F.; et al. Preservation of Sweet Cherry by Isochoric (Constant volume) Freezing. Innov. Food Sci. Emerg. Technol. 2018, 52, 108–115. DOI: 10.1016/j.ifset.2018.10.016.
- Hunter, K. J.; Fletcher, J. M. The Antioxidant Activity and Composition of Fresh Frozen, Jarred and Canned Vegetables. Innov. Food Sci. Emerg. Technol. 2002, 3, 399–406. DOI: 10.1016/S1466-8564(02)00048-6.
- Tosun, B.; Yücecan, S. Influence of Commercial Freezing and Storage on Vitamin C Content of Some Vegetables. Int. J. Food Sci. Tech. 2008, 43, 316–321. DOI: 10.1111/j.1365-2621.2006.01436.x.
- Serpen, A.; Gökmen, V.; Bahçeci, K. S.; Acar, J. Reversible Degradation Kinetics of Vitamin C in Peas during Frozen Storage. Eur. Food Res. Technol. 2007, 224, 749–753. DOI: 10.1007/s00217-006-0369-y.
- Gonçalves, E. M.; Abreu, M.; Brandão, T. R. S.; Silva, C. L. M. Degradation Kinetics of Colour, Vitamin C and Drip Loss in Frozen Broccoli (Brassica Oleracea L. Ssp. italica) during Storage at Isothermal and Non-isothermal Conditions. Int. J. Refrig. 2011a, 34, 2136–2144. DOI: 10.1016/j.ijrefrig.2011.06.006.
- Gonçalves, E. M.; Pinheiro, J.; Abreu, M.; Brandão, T. R. S.; Silva, C. L. M. Kinetics of Quality Changes of Pumpkin (Curcurbita Maxima L.) Stored under Isothermal and Non-isothermal Frozen Conditions. J. Food Eng. 2011b, 106, 40–47. DOI: 10.1016/j.jfoodeng.2011.04.004.
- Martins, R. C.; Silva, C. L. M. Frozen Green Beans (Phaseolus Vulgaris L.) Quality Profile Evaluation during Home Storage. J. Food Eng. 2004, 64, 481–488. DOI: 10.1016/j.jfoodeng.2003.11.015.
- Abbasi, N. A.; Iqbal, Z.; Maqbool, M.; Hafiz, I. A. Postharvest Quality of Mango (Mangifera indicaL.) Fruits as Affected by Coating. Pak. J. Bot. 2009, 41, 343–357.
- Sheu, S. C.; Chen, A. O. Lipoxygenase as Blanching Index for Frozen Vegetable Soybeans. J. Food Sci. 1991, 56, 448–451. DOI: 10.1111/j.1365-2621.1991.tb05300.x.
- Zhao, J.; Li, H.; Xi, W.; An, W.; Niu, L.; Cao, Y.; Wang, H.; Wang, Y.; Yin, Y. Changes in Sugars and Organic Acids in Wolf Berry (Lpeiumn Barbara L.) Fruit during Development and Maturation. Food Chem. 2015, 173, 718–724. DOI: 10.1016/j.foodchem.2014.10.082.
- Martins, R. C.; Silva, C. L. M. Kinetics of Frozen Stored Green Bean (Phaseolus Vulgaris L.) Quality Changes: Texture, Vitamin C, Reducing Sugars, and Starch. J. Food Sci. 2010, 68, 2232–2237. DOI: 10.1111/j.1365-2621.2003.tb05752.x.
- Deng, Y.; Wu, Y.; Li, Y. F. Effects of High O2 Levels on Post-harvest Quality and Shelf Life of Table Grapes during Long-term Storage. Eur. Food Res. Technol. 2005, 221, 392–397. DOI: 10.1007/s00217-005-1186-4.
- Yang, H.; Wang, Y.; Lai, S.; An, H.; Li, Y.; Chen, F. Application of Atomic Force Microscopy as a Nanotechnology Tool in Food Science. J. Food Sci. 2007a, 72, 65–75. DOI: 10.1111/jfds.2007.72.issue-4.
- Xu, Z.; Guo, Y.; Ding, S.; An, K.; Wang, Z. Freezing by Immersion in Liquid CO2, at Variable Pressure: Response Surface Analysis of the Application to Carrot Slices Freezing. Innov. Food Sci. Emerg. 2014, 22, 167–174. DOI: 10.1016/j.ifset.2013.06.005.
- Van Buggenhout, S.; Tran, T. T.; Sila, D.; Smout, C.; Hendrickx, M. Influence of Pectin Conversions Combined with High Pressure Shift Freezing on the Texture of Frozen Carrots. Commu. Agric. Appl. Biol. Sci. 2004, 69, 289–292.
- Grayson, A. L.; King, D. A.; Shackelford, S. D.; Koohmaraie, M.; Wheeler, T. L. Freezing and Thawing or Freezing, Thawing, and Aging Effects on Beef Tenderness. J. Anim. Sci. 2014, 92, 2735–2740. DOI: 10.2527/jas.2014-7613.
- Lin, H. T.; Xi, Y. F.; Chen, S. J. Post Harvest Softening Physiological Mechanism of Huang Hua Pear Fruit. Sci. Agric. Sin. 2003, 36, 349–352.
- Lohani, S.; Trivedi, P. K.; Nath, P. Changes in Activities of Cell Wall Hydro-lases during Ethylene-induced Ripening in Banana: Effect of 1-MCP, ABA and IAA. Post Harvest Biol. Tec. 2004, 31, 119–126. DOI: 10.1016/j.postharvbio.2003.08.001.
- Li, Z.; Liu, Y.; Cai, N. Effect of CaO Hydration and Carbonation on the Hydrogen Production from Sorption Enhanced Water Gas Shift Reaction. Int. J. Hydrogen Energ. 2012, 37, 11227–11236. DOI: 10.1016/j.ijhydene.2012.04.160.
- Zaritzky, N.;. Physical-chemical Principles in Freezing. In Handbook of Frozen Food Processing and Packaging; Sun, D., Ed.., Taylor and Francis group edn. Boca Raton, FL: CRC Press. 2005, pp 32.
- Livanainen, E.; Martikainen, P. J.; Katila, M. L. Effect of Freezing of Water Samples on Viable Counts of Environmental Mycobacteria. Lett. Appl. Microbiol. 2010, 21, 257–260. DOI: 10.1111/j.1472-765X.1995.tb01055.x.
- Hazen, R. A.; Bushway, A. A.; Davis-Dentici, K. Evaluation of the Microbiological Quality of IQF Processed Maine Wild Blueberries: An “In Plant study”. Int. J. Fruit Sci. 2001, 1, 47–59.
- National Standards of the People's Republic of China, GB4789.23: Microbiological Examination of Food Hygiene-Examination of Cold dish and Bean Products. In Ministry of Health of the People's Republic of China, Standardization Administration of the People's Republic of China; Beijing, China, 2003, 165–168.
- Calcott, P. H.; MacLeod, R. A. Survival of Escherichia Coli from Freeze-thaw Damage: A Theoretical and Practical Study. Can. J. Microbiol. 1974, 20, 671–681. DOI: 10.1139/m74-103.
- Vasafi, P. S.; Hamdami, N.; Keramat, J. Quality and Microbial Stability of Part-baked ‘Barbari Bread’ during Freezing Storage. LWT-Food Sci. Tech. 2019, 104, 173–179. DOI: 10.1016/j.lwt.2019.01.033.
- Zhang, L.; Yan, Z.; Hanson, E. J.; Ryser, E. T. Efficacy of Chlorine Dioxide Gas and Freezing Rate on the Microbiological Quality of Frozen Blueberries. Food Control. 2015, 47, 114–119. DOI: 10.1016/j.foodcont.2014.06.008.
- Abd-El-Aziz, N. A.; Moharram, Y. G. Microbiological Quality of Imported Frozen Shrimp in Egypt. AOAS. 2016, 61, 35–40.
- Crowe, K. M.; Bushway, A.; Davis-Deotici, K. Impact of Postharvest Treatmeats, Chlorine and Ozone Coupled with Low-temperature Frozen Storage on the Aotimicrobial Quality of Lowbush Blueberries (Vaccinium angustifoium). LWT-Food Sci. Technol. 2012, 47, 213–215. DOI: 10.1016/j.lwt.2011.12.026.
- Chevalier, D.; Bail, A. L.; Ghoul, M. Freezing and Ice Crystals Formed in a Cylindrical Food Model: Part I. Freezing at Atmospheric Pressure. J. Food Eng. 2000, 46, 277–285. DOI: 10.1016/S0260-8774(00)00089-3.
- Meziani, S.; Ioannou, I.; Jasniewski, J.; Belhaj, N.; Muller, J. M.; Ghoul, M.; Desobry, S. Effects of Freezing Treatments on the Fermentative Activity and Gluten Network Integrity of Sweet Dough. LWT-Food Sci. Technol. 2012, 46, 118–126. DOI: 10.1016/j.lwt.2011.10.017.
- Yi, J.; Kerr, W. L. Combined Effects of Freezing Rate, Storage Temperature and Time on Bread Dough and Baking Properties. LWT-Food Sci. Technol. 2009b, 42, 1474–1483. DOI: 10.1016/j.lwt.2009.05.017.
- Al-Bulushi, I. M.; Kasapis, S.; Dykes, G. A.; Al-Waili, H.; Guizani, N.; Al-Oufi, H. Effect of Frozen Storage on the Characteristics of a Developed and Commercial Fish Sausage. J. Food Sci. Technol-Mysore. 2011, 50, 1158–1164. DOI: 10.1007/s13197-011-0441-x.