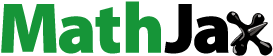
ABSTRACT
The downy rose-myrtle berry is regarded as a healthy food due to its high content of anthocyanin and other antioxidant phytochemicals. The berries are sometimes dried, ground into a powder and used as a natural food coloring agent or functional food additive. This study aimed to determine the physicochemical properties and anthocyanin bioaccessibility of superfine ground downy rose-myrtle powders. Compared with shear pulverized coarse particles, the superfine ground particles of berry powders had decreased bulk density and fluidity while improved water-holding capacity and solubility. In addition, the amounts of polyphenols and anthocyanins released from the superfine powders were significantly higher than those from coarse powders during in vitro gastrointestinal digestion. These results indicate that after superfine grinding treatment, downy rose-myrtle powder could serve as a potential resource of natural bioactive compounds for food and nutraceutical applications.
Introduction
Downy rose-myrtle, scientifically named Rhodomyrtus tomentosa (Ait.) Hassk, is a well-known edible and medicinal plant, and is mainly found in south-east Asian countries, such as Thailand, Japan, and China.[Citation1] The juicy downy rose-myrtle berry turns dark purple when ripe, and is historically regarded as a healthy food that has been used for making traditional wine, jams and beverages. Anthocyanin pigments confer the purple color and are considered the active components of downy rose-myrtle.[Citation2] These pigments are located in the vacuole of plant cells, and are released following cell rupture during eating or processing. Generally, bioaccessibility is defined by the fraction of bioactive substance that is released from the food matrix after digestion and solubilized in the gut lumen for uptake across the intestinal mucosa.[Citation3] The cell wall breakage ratio is a key factor limiting anthocyanin bioaccessibility during food digestion, since the approximate diameter of a plant cell ranges from 10 to 50 μm,[Citation4] which is too small to be broken by chewing and grinding with teeth. Anthocyanins remaining within intact cells are inaccessible during upper gut digestion.[Citation5]
Superfine grinding is a new technology that can reduce the particle size of food ingredients to 0.01–25 μm. Compared with traditional mechanically ground particle materials, superfine food powder has outstanding characteristics including high solubility, dispersion, adsorption, chemical reactivity, and fluidity.[Citation6–Citation9] This is because superfine grinding can increase the particle surface area and break down cell walls. Ni et al. found that superfine grinding markedly increases the extraction of polyphenols from green tea, leading to more potent free radical scavenging capacity.[Citation10] Tang et al. found that the antioxidant activity of red grape pomace powder increases with decreasing particle size after superfine grinding.[Citation7] Superfine grinding can break the plant cell wall and promote the release of active components from the food matrix.[Citation9] Therefore, it is interesting to study the physicochemical and morphological properties of superfine powder derived from downy rose-myrtle and its bioaccessible anthocyanin as a functional ingredient.
In vitro gastrointestinal digestion procedures have been extensively used to assess the bioaccessibility of food constituents because they are rapid, safe and do not have the same ethical restrictions as in vivo approaches. The in vitro gastrointestinal digestion method has already been used for the study of triturated foods rich in anthocyanins such as chokeberry,[Citation11] mulberry,[Citation12] pomegranate,[Citation13] and red cabbage.[Citation14] There were a mass of controversial findings about the effects of in vitro gastrointestinal digestion on the bioaccessibility and stability of anthocyanin in the previous research. The degree of pulverization may impact the anthocyanin release from food matrix. Till date, little is known about the anthocyanin bioaccessibility and antioxidant activity of super finely ground food powder during digestion.
In this study, we compared the morphological and physicochemical properties of superfine powders and coarse particles derived from downy rose-myrtle. In addition, the digestibility of phenolics and anthocyanins, as well as the antioxidant activity of digested compounds of downy rose-myrtle powder were determined using an in vitro gastrointestinal digestion model.
Materials and methods
Materials
Fresh mature fruits of downy rose-myrtle were obtained in September 2016 from a local market in Shaoguan, Guangdong Province, China. HPLC certified syringe filters, formic acid, and acetonitrile were purchased from Dikma Technologies Inc. (Beijing, China). The standard of cyanidin-3-glucoside was provided by Polyphenol AS (Sandnes, Norway). Folin-Ciocalteu’s reagent was obtained from Sinopharm Chemical (Shanghai, China). The enzymes used for in vitro digestion were supplied by Sigma Aldrich (St Louis, MO, USA).
Sample preparation
The downy rose-myrtle berries were put in a forced convection oven and incubated at 60°C for 8 h and then dried in a chamber under a vacuum with heated air at 60°C until the moisture content reached less than 8%. The moisture content was determined by weighing the sample after drying at 105°C for 24 h. The dried fruits were milled into raw material powders by a DLF18 disc mill (Dingli Medical Instrument, Wenzhou, China). Some of the raw material powders were screened through sieves with bore diameters <96 μm, 96–120 μm and 120–180 μm to separate granulates to yield three different particle sizes of coarse powders (CP), denoted as CP96, CP120, and CP180, respectively. The remaining RMPs were re-milled at 20,000 r/min for 3 min with a YF2-2 micronizer (Yongli Pharmaceutical Machinery, Ruian, China) to obtain superfine powder.
Particle size and specific surface area measurements
The particle size distribution and specific surface area were measured using a LS-POP9 laser diffraction particle size analyzer (Omec Instruments, Zhuhai, China) at room temperature with deionized water as the solvent. Particle size distribution was characterized by the median diameter (D50) and span factor [(D90 – D10)/D50], where D10, D50, and D90 represent 10%, 50% and 90% cumulative percentiles of particles, respectively.[Citation15]
Scanning electron microscope (SEM)
Downy rose-myrtle powder (DRMP) samples were spread on a conductive adhesive carbon tape that was pasted on a sample stub. The morphological characteristics of different sized DRMPs were determined using a Hitachi TM3030 model SEM (Hitachi, Japan) at 15 kV accelerated voltage and 10–15 mm working distance.
Color difference analysis
The color of different sized DRMPs was determined using a WR-10 automatic chromameter (Wave Optoelectronics Technology Co., Ltd, Shenzhen, China). The color values represented whiteness/darkness (L/-L), redness/greenness (a/-a) and yellowness/blueness (b/-b).[Citation16]
Bulk density
The bulk density of a powder is the ratio of the mass of an untapped powder sample to its volume including pores and interparticle voids. 10.0 g powder samples were poured into a 50-mL volumetric cylinder using a funnel and the volume of the powder was taken. The bulk density of the downy rose-myrtle powder was calculated using the following formula:
Angle of repose and slide
Angle of repose is the tan inverse of the angle between the height of the powder pile and the radius of the base of the conical pile. The angle of repose of downy rose-myrtle powder was measured using a funnel method.[Citation6] First, a funnel was vertically fixed above the graph paper so that the distance of the paper from the outlet of the funnel (H) was 4 cm. Then, four types of downy rose-myrtle powders were poured into the funnel till the tip of the powder cone touched the outlet of the funnel. The radius (R) of the cone was measured for each type of powder. Results were only considered valid when a symmetrical cone of powder was formed. The angle of repose (°) was calculated as follows:
The slide angle was determined as previously described.[Citation8] Briefly, 3.0 g powder samples with different sizes were poured on a glass plate with a length (L) of 150 mm and a width of 100 mm. The top of the glass plate was gradually lifted until the surface of the downy rose-myrtle powder began to slide. The vertical distance (H) between the top of the inclined glass plate and the horizontal was estimated to calculate the angle of slide using the following formula:
Water-holding capacity (WHC)
WHC represents the ability of a powder to absorb and retain bound, hydrodynamic, capillary, and physically entrapped water against gravity. The WHC of downy rose-myrtle powder was determined as previously described with some modifications.[Citation6] First, 2.0 g powder was weighed in pre-weighed 50 mL plastic centrifuge tube. Then, the sample was dispersed in 40 mL distilled water and placed in a water bath at 37°C for 10 min. The mixture was centrifuged at 5000 r/min for 15 min. The supernatant liquid was carefully removed and the centrifuge tubes with the powders were weighed again. WHC (g water/g powder) was calculated as: WHC = total water mass/dry powder mass.
Water solubility index (WSI)
This parameter was measured according to the method reported by Zhao et al. with some modifications.[Citation6] 1.000 g (W1) sample was measured and dispersed in tubes with 50 mL of distilled water. The mixture was incubated at 37°C in a shaking water bath for 30 min at a speed of 100 r/min. Then, the tubes were centrifuged at 5000 r/min for 15 min. The clear supernatant solution was drained off by evaporation, and the samples were dried at 105°C and weighed (W2). The WSI was calculated as: WSI (%) = W2/W1 × 100%.
In vitro simulated gastrointestinal digestion
The digestion procedure mimicking the physiological situation of the stomach and small intestine was carried out as previously described with some modifications.[Citation17] 40 mL distilled water was added to 5.0 g downy rose-myrtle powder. To simulate gastric digestion, 60,000 units pepsin (EC 2.4.23.1) was added to the samples, and the pH was adjusted to 2 with 1 M HCl (final incubation volume 50 mL). The mixture was incubated with gently shaking at a 37°C for 2 h in the absence of light. After post-gastric digestion, aliquots of 10 mL samples were taken and frozen for analysis of anthocyanins and phenolics. The remainder was diluted with 30 mL water adjusted to pH 7.4 with 1 M NaHCO3. Then, a stock solution of pancreatin from porcine pancreas (final trypsin activity 100 U/mL) and bile salts containing a mixture of sodium cholate and sodium deoxycholate (final concentration of bile acid 10 mM) were added for a final volume of 80 mL. The resulting mixtures were incubated for an additional 2 h at 37°C. After pancreatic digestion, the aliquots of the digested samples were rapidly acidified to pH 2.0 and stored at −80°C until use.
Determination of total polyphenols and anthocyanins
One gram of different sized downy rose-myrtle powders was dispersed in 16 mL of 70% aqueous methanol or the same amount of distilled water and put into centrifuge tubes, placed in a water bath at 37°C and shaken for 4 h. The extracts were then centrifuged at 5000 g for 10 min, and supernatants were collected. The obtained methanolic and aqueous extracts, as well as supernatants of the gastric and pancreatic digestion samples, were assayed for total polyphenol and anthocyanin content. Results obtained for methanolic extracts were assumed to be 100% of polyphenols or anthocyanins contained in the downy rose-myrtle powders. The bioaccessibility of polyphenols and anthocyanins, defined as the fraction of an external dose released from their matrix in the gastrointestinal tract,[Citation18] was calculated as follows:
where AD is the amount of polyphenols or anthocyanins present in the digesta post gastric and pancreatic digestion, and AE is the amount of polyphenols or anthocyanins extracted from downy rose-myrtle powders using methanol as extractant. Total phenolics content was determined by the Folin–Ciocalteu method.[Citation19] An aliquot of 0.5 mL of sample was mixed with 2.5 mL of Folin-Ciocalteu’s reagent (FCR; 1:10 dilution), and incubated at 50°C for 30 min to allow for the FCR to react completely with the oxidizable phenolates. To destroy the residual reagent, 2 mL of Na2CO3 (20% solution in water) was added. The absorbances were measured at 760 nm using a spectrophotometer. Quantification was done using a standard curve of gallic acid and expressed as milligrams of catechin equivalents per 100 g of powder.
The anthocyanin profiles of nondigested or digested samples were analyzed by a Shimadzu 20A HPLC System equipped with a UV-Vis detector and a Diamonsil C18 column (250 mm × 4.6 mm; 5 μm). All samples before injection were passed through a 0.45 μm filter. The binary mobile phase consisted of water/formic acid (95:5, v/v) (solvent A) and acetonitrile, according to our previous study.[Citation2] The system was run with a flow rate of 1 mL/min and under the following gradient program: 0 min: 85% A + 15% B, 5 min: 75% A + 25% B, 15 min: 65% A + 35% B, 20 min: 55% A + 45% B, and 30 min: 50% A + 50% B. Anthocyanins were detected at 520 nm and their content was expressed as cyanidin 3-glucoside equivalents.[Citation20]
ABTS radical cation scavenging activity
Experiments were performed according to a procedure described in our previous study.[Citation21] ABTS radical cation was produced by mixing the 7 mM ABTS solution with 2.45 mM potassium persulfate aqueous solution and leaving the mixture to stand for 12–16 h in the dark at room temperature before use. The stock solution was diluted with phosphate buffer to the absorbance of 0.70 ± 0.05 at 734 nm. Each sample solution (0.1 mL) was added to 1.9 mL of diluted ABTS+ solution and incubated at 37°C for 5 min before the absorption was measured at 734 nm. In the blank, 0.1 mL distilled water was used instead of the sample. Trolox (6-hydroxy-2,5,7,8-tetramethylchromane-2-carboxylic acid) was used as a standard and results were expressed in mmol Trolox equivalents (TE)/g of powder.
Statistical analyses
All data are expressed as ‘mean ± standard deviation’ from at least three replicates. Statistical comparisons were made using one-way analysis of variance (ANOVA) followed by Tukey–Kramer post hoc test for multiple comparisons. Statistically significant difference was defined as P< .05. All analyses were performed with the Statistical Package for the Social Sciences software (version 19.0, SPSS Inc., Chicago, IL).
Results and discussion
Micromeritic properties of downy rose-myrtle powder
The particle size of the superfine downy rose-myrtle powder ranged from 0.79 to 41.57 μm, with a median particle size of 16.41 μm (). The particle size distributions of the coarsely ground powder fractions of CP180, CP120, and CP96 were much wider, ranging from 1.52 to 218.94 μm, with median particle sizes of 86.93, 73.19 and 46.56 μm, respectively. In addition, the superfine powder had a significantly narrower particle size distribution (span factor = 2.20) than the coarsely ground downy rose-myrtle powders. Thus, compared to the traditional shear pulverization grinding process, superfine grinding brings much more energy with higher reduction efficiency, making the samples easy to reduce to sub-micron size powders.
Table 1. Micromeritic and color properties of downy rose-myrtle powder
The specific surface area values of the different particle-sized powders ranged from 1.82 to 3.14 m2/g. These parameters varied significantly between the downy rose-myrtle particles with different sizes (P < .05). Furthermore, the specific surface area increased as the particle size decreased. These results indicate that the surface parameters of downy rose-myrtle powders are directly related to the projected size of the corresponding fruit granules.
Morphology of downy rose-myrtle powder
SEM images at 600 times magnification were obtained for the four downy rose-myrtle powder sizes (), and the SEM results are highly consistent with the particle size analysis data. After superfine grinding, the particle size was significantly reduced and uniformity was greatly improved. Partial aggregation was observed in the superfine downy rose-myrtle powder, which may attribute to its high specific surface area and electrostatic interaction.[Citation22]
Color difference of downy rose-myrtle powders
In addition to particle size, color is an important quality character for food powders. A previous study by Hu et al. indicated that particle size has a significant effect on the color characteristics of green tea powder.[Citation10] The effect of superfine grinding on the color properties of downy rose-myrtle powder is shown in . The results show that the values of lightness, redness, and yellowness of the superfine ground powder were significantly higher than those of CP180 and CP120 (P < 0.05). Thus, superfine grinding can yield downy rose-myrtle powders with bright red and yellow colors.
Basic physical properties of downy rose-myrtle powder
We next characterized some physical properties of downy rose-myrtle powder with different sizes (). The bulk density of the superfine ground powder was significantly lower than that of the other powders (P < 0.05). This suggests that the superfine ground power has larger interparticle voids, which would offer a larger contact surface with the surroundings. Bulk density can also help determine whether a raw material should be mixed or a final product is packed in a predetermined container. As with other powders of the same weight, the finer downy rose-myrtle powder should be packed in a relatively large container.
Table 2. Physical properties of downy rose-myrtle powder
The change in the fluidity of the downy rose-myrtle powder can be shown through the angles of repose and slide, which were found to increase dramatically with a decreasing particle size (). The superfine powder displayed the highest repose and slide angles. As the angle of repose and slide increased, the fluidity of the particle worsened. This result is inconsistent with the results of super finely ground ginger and pomegranate peel powders.[Citation6,Citation8] However, Zhao et al. showed that the fluidity of red grape pomace powders increased with increasing particle size.[Citation7] Similarly, this could be explained by the fact that the finer downy rose-myrtle powder is easily agglomerated due to its oil content or absorbance of water from the air. The aggregates tended to arrange in a cone with an angle that was much higher than expected. As a result, the fluidity of the finer powder decreased.
The different particle-sized powders had different WHC and WSI. Compared with the coarse downy rose-myrtle particles, the superfine powder possessed higher WHC and WSI (). This may be due to improved surface properties of the superfine powders, such as an increase in surface area and surface energy.[Citation9] In addition, the hydrophilic groups in the cellulose and hemicellulose of the downy rose-myrtle may have been exposed, resulting in easy integration with water and ultimately increasing the WHC and WSI values. The results indicate that the finer downy rose-myrtle powder could be dispersed better in water than coarser particles and may increase the solubility of water-soluble ingredients. The dispersibility and dissolution of the superfine powder were exceptional and the functional components increased after superfine grinding, which could be applied in food processing as a new value-added product with health-promoting benefits.
Release of polyphenols and anthocyanins from downy rose-myrtle powder before and after gastrointestinal digestion
Absorption of phenolic compounds is dependent on release from the food matrix.[Citation23] To understand the potential bioaccessibility of phenolic compounds in downy rose-myrtle powder after digestion, the release of polyphenols () and anthocyanins () was measured. Polyphenols and anthocyanins can both be extracted efficiently using methanol, so the amounts of polyphenols and anthocyanins extracted by methanol were considered to be 100%.[Citation13] Aqueous extracts were also measured to assess the bioaccessibility of these phytochemicals in the digestive system. In the non-digested aqueous extracts of the coarse powders, the polyphenol content varied from 514 ± 16 to 570 ± 12 mg GAE/100 g, while in the superfine powder, the polyphenol content significantly increased to 662 ± 21 mg GAE/100 g. Similarly, the amount of anthocyanins released from the coarse powders was lower than that released from the superfine powders. After gastric digestion, both the released polyphenol and anthocyanin content increased markedly, which is consistent with previous studies.[Citation13,Citation24] During the gastric phase, pepsin digestion and low pH favored the inseparable phenolic compounds, which could be released by diffusion from the food matrix to the aqueous phase due to reduced ionic interactions.
Table 3. The release of polyphenols (mg GAE/100 g powder) from downy rose-myrtle powders before and after in vitro gastrointestinal digestion
Table 4. The release of anthocyanins (mg CGE/100 g powder) from downy rose-myrtle powders before and after in vitro gastrointestinal digestion
Compare with polyphenols release after gastric digestion, pancreatic treatment of the coarse powders significantly enhanced the amounts of polyphenols released, but this parameter of the superfine powder was unchanged (). However, transition of samples from the acidic gastric (pH 2.0) to the mild alkaline intestinal environment (pH 7.4) caused a slight decrease in the amounts of anthocyanins relative to the initial samples (). The phenolic compounds of the downy rose-myrtle powders were found to be more stable to gastrointestinal digestion than anthocyanin, and losses were greater during pancreatic digestion than gastric digestion. Losses of anthocyanins during pancreatic digestion were likely due to an increase in pH.[Citation25] Overall, we found that the bioaccessibility of either polyphenols or anthocyanins bioaccessibility of the superfine powder was higher than that of the other powders (all P < 0.05; ).
Influence of in vitro digestion on antioxidant capacity
The health benefits of downy rose-myrtle berries are partly attributed to their antioxidant activity. The antioxidant capacities of the initial and digested samples were evaluated with the ABTS free radical scavenging method, which is widely used to evaluate the free radical scavenging capacities of natural compounds. The radical scavenging activities of the aqueous downy rose-myrtle powder extract were found to be significantly lower than those of the methanolic extract (). Among all tested aqueous extracts, the superfine powder extract exhibited the strongest ABTS free radical scavenging activity, while the crude powder extract with the largest particle size showed the lowest antiradical activity. After the gastric phase of digestion, although there was no change in the order of the activities, the activities of all powder digesta significantly increased. After the intestinal phase of digestion, the antiradical activities of the crude powder samples were further increased, while those of the superfine powder remained unchanged. Interestingly, a high positive correlation was also observed between the antiradical activity and the total polyphenolic content (r = 0.9316, P < 0.01) or the total anthocyanin content (r = 0.8956, P < 0.01) of the downy rose-myrtle powder extracts. A possible explanation for this is that anthocyanins and phenolic compounds may directly contribute to the antioxidant capacity of the digestion samples.[Citation26]
Conclusion
Our research dried and ground downy rose-myrtle berries into superfine powder form with a median particle size of 16.41 μm. Compare with coarsely ground powders, the superfine powders have many significant characteristics including narrow and uniform particle size distribution, strong specific surface energy, remarkable dispersibility and solubility, polyphenol and anthocyanin bioaccessibility, and ABTS radical scavenging activity. These findings indicate that superfine grinding has a great potential to improve the physicochemical and functional properties of downy rose-myrtle powders, which is helpful to promote the overall quality and healthy effect of foods containing downy rose-myrtle powders.
Acknowledgments
Conceptualization, H.G.; methodology, D.Z.; formal analysis, X.G. and Y.L.; data curation, Y.L.; original draft preparation, X.G. and H.G.; review and editing, L.Z. and D.C.; supervision, H.G.; funding acquisition, H.G. The authors declare no conflict of interest.
Additional information
Funding
References
- Jeong, D.; Yang, W. S.; Yang, Y.; Nam, G.; Kim, J. H.; Yoon, D. H.; Noh, H. J.; Lee, S.; Kim, T. W.; Sung, G.-H., et al. In Vitro and In Vivo Anti-inflammatory Effect of Rhodomyrtus Tomentosa Methanol Extract. J. Ethnopharmacol. 2013, 146, 205–213. DOI: 10.1016/j.jep.2012.12.034.
- Liu, G.; Guo, H.; Sun, Y. Optimization of the Extraction of Anthocyanins from the Fruit Skin of Rhodomyrtus Tomentosa (Ait.) Hassk and Identification of Anthocyanins in the Extract Using High-performance Liquid Chromatography-electrospray Ionization-mass Spectrometry (HPLC-ESI-MS). Int. J. Mol. Sci. 2012, 13, 6292–6302. DOI: 10.3390/ijms13056292.
- Amagloh, F. K.; Atuna, R. A.; McBride, R.; Carey, E. E.; Christides, T. Nutrient and Total Polyphenol Contents of Dark Green Leafy Vegetables, and Estimation of Their Iron Bioaccessibility Using the In Vitro digestion/Caco-2 Cell Model. Foods. 2017, 6, 54. DOI: 10.3390/foods6070054.
- Carrillo, C.; Buvé, C.; Panozzo, A.; Grauwet, T.; Hendrickx, M. Role of Structural Barriers in the In Vitro Bioaccessibility of Anthocyanins in Comparison with Carotenoids. Food Chem. 2017, 227, 271–279. DOI: 10.1016/j.foodchem.2017.01.062.
- Yang, M.; Koo, S. I.; Song, W. O.; Chun, O. K. Food Matrix Affecting Anthocyanin Bioavailability: Review. Curr. Med. Chem. 2011, 18, 291–300. DOI: 10.2174/092986711794088380.
- Zhao, X.; Yang, Z.; Gai, G.; Yang, Y. Effect of Superfine Grinding on Properties of Ginger Powder. J. Food Eng. 2009, 91, 217–222. DOI: 10.1016/j.jfoodeng.2008.08.024.
- Zhao, X.; Zhu, H.; Zhang, G.; Tang, W. Effect of Superfine Grinding on the Physicochemical Properties and Antioxidant Activity of Red Grape Pomace Powders. Powder Technol. 2015, 286, 838–844. DOI: 10.1016/j.powtec.2015.09.025.
- Zhong, C.; Zu, Y.; Zhao, X.; Li, Y.; Ge, Y.; Wu, W.; Zhang, Y.; Li, Y.; Guo, D. Effect of Superfine Grinding on Physicochemical and Antioxidant Properties of Pomegranate Peel. Int. J. Food Sci. Tech. 2016, 51, 212–221. DOI: 10.1111/ijfs.12982.
- Jiang, L.; Xu, Q. X.; Qiao, M.; Ma, F. F.; Thakur, K.; Wei, Z. J. Effect of Superfine Grinding on Properties of Vaccinium Bracteatum Thunb Leaves Powder. Food Sci. Biotechnol. 2017, 26, 1571–1578. DOI: 10.1007/s10068-017-0126-y.
- Hu, J.; Chen, Y.; Ni, D. Effect of Superfine Grinding on Quality and Antioxidant Property of Fine Green Tea Powders. LWT - Food Sci. Technol. 2012, 45, 8–12. DOI: 10.1016/j.lwt.2011.08.002.
- Bermudezsoto, M.; Tomasbarberan, F.; Garciaconesa, M. Stability of Polyphenols in Chokeberry (Aronia Melanocarpa) Subjected to In Vitro Gastric and Pancreatic Digestion. Food Chem. 2007, 102, 865–874. DOI: 10.1016/j.foodchem.2006.06.025.
- Liang, L.; Wu, X.; Zhao, T.; Zhao, J.; Li, F.; Zou, Y.; Mao, G.; Yang, L. In Vitro Bioaccessibility and Antioxidant Activity of Anthocyanins from Mulberry (Morus Atropurpurea Roxb.) Following Simulated Gastro-intestinal Digestion. Food Res. Int. 2012, 46, 76–82. DOI: 10.1016/j.foodres.2011.11.024.
- Sengul, H.; Surek, E.; Nilufer-Erdil, D. Investigating the Effects of Food Matrix and Food Components on Bioaccessibility of Pomegranate (Punica Granatum) Phenolics and Anthocyanins Using an In-vitro Gastrointestinal Digestion Model. Food Res. Int. 2014, 62, 1069–1079. DOI: 10.1016/j.foodres.2014.05.055.
- Podsędek, A.; Redzynia, M.; Klewicka, E.; Koziołkiewicz, M. Matrix Effects on the Stability and Antioxidant Activity of Red Cabbage Anthocyanins under Simulated Gastrointestinal Digestion. Biomed Res. Int. 2014, 2014, 1–11. DOI: 10.1155/2014/365738.
- Xiao, W.; Zhang, Y.; Fan, C.; Han, L. A Method for Producing Superfine Black Tea Powder with Enhanced Infusion and Dispersion Property. Food Chem. 2017, 214, 242–247. DOI: 10.1016/j.foodchem.2016.07.096.
- Su, G.; Zhu, S.; Xu, M.; Ramaswamy, H. S.; Lin, Y.; Yu, Y. Pressure Degradation Kinetics of Anthocyanin Pigment and Visual Color of Chinese Bayberry Juice. Int. J. Food Prop. 2015, 19, 443–453. DOI: 10.1080/10942912.2015.1038562.
- Minekus, M.; Alminger, M.; Alvito, P.; Ballance, S.; Bohn, T.; Bourlieu, C.; Carrière, F.; Boutrou, R.; Corredig, M.; Dupont, D., et al. A Standardised Static In Vitro Digestion Method Suitable for Food – An International Consensus. Food Funct. 2014, 5, 1113. DOI: 10.1039/c3fo60702j.
- D’Antuono, I.; Garbetta, A.; Linsalata, V.; Minervini, F.; Cardinali, A. Polyphenols from Artichoke Heads (Cynara Cardunculus (L.) Subsp. Scolymus Hayek): In Vitro Bio-accessibility, Intestinal Uptake and Bioavailability. Food Funct. 2015, 6, 1268–1277. DOI: 10.1039/c5fo00137d.
- Fang, Z.; Bhandari, B. Effect of Spray Drying and Storage on the Stability of Bayberry Polyphenols. Food Chem. 2011, 129, 1139–1147. DOI: 10.1016/j.foodchem.2011.05.093.
- Lee, S. G.; Vance, T. M.; Nam, T.-G.; Kim, D.-O.; Koo, S. I.; Chun, O. K. Evaluation of pH Differential and HPLC Methods Expressed as Cyanidin-3-glucoside Equivalent for Measuring the Total Anthocyanin Contents of Berries. J. Food Meas. Charact. 2016, 10, 562–568. DOI: 10.1007/s11694-016-9337-9.
- Liu, Y.; Chen, F.; Guo, H. Optimization of Bayberry Juice Spray Drying Process Using Response Surface Methodology. Food Sci. Biotechnol. 2017, 26, 1235–1244. DOI: 10.1007/s10068-017-0169-0.
- Zhang, H.; Chen, L.; Yang, T.; Liu, Q.; Chen, X.; Zhang, Y.; Shu, G.; Li, J. Production of Superfine Green Tea Powder from Processing Wastes: Characterization of Chemical Composition and Exploration of Antimicrobial Potential against Ralstonia Solanacearum. LWT - Food Sci. Technol. 2019, 104, 142–147. DOI: 10.1016/j.lwt.2019.01.032.
- Scholz S, Willimson G. Interactions Affecting the Bioavailability of Dietary Polyphenols in Vivo. Int. J. Vitam. Nutr. Res. 2007, 77, 224–235. DOI: 10.1024/0300-9831.77.3.224.
- Blancas-Benitez, F. J.; Mercado-Mercado, G.; Quirós-Sauceda, A. E.; Montalvo-González, E.; González-Aguilar, G. A.; Sáyago-Ayerdi, S. G. Bioaccessibility of Polyphenols Associated with Dietary Fiber and In Vitro Kinetics Release of Polyphenols in Mexican ‘Ataulfo’ Mango (Mangifera Indica L.) By-Products. Food Funct. 2015, 6, 859–868. DOI: 10.1039/c4fo00982g.
- Jamei, R.; Babaloo, F. Stability of Blueberry (Cornus Mas – Yulyush) Anthocyanin Pigment under pH and Co-pigment Treatments. Int. J. Food Prop. 2016, 20, 2128–2133. DOI: 10.1080/10942912.2016.1233116.
- Guofang, X.; Xiaoyan, X.; Xiaoli, Z.; Yongling, L.; Zhibing, Z. Changes in Phenolic Profiles and Antioxidant Activity in Rabbiteye Blueberries during Ripening. Int. J. Food Prop. 2019, 22, 320–329. DOI: 10.1080/10942912.2019.1580718.