ABSTRACT
Pre-fermentation is crucial for black glutinous rice wine production. However, the dynamic changes occurring in metabolites during pre-fermentation remain unclear. Hence, multivariate analysis to investigate the relationship between metabolites and fermentation time was performed. Both PCA and OPLS-DA score plots showed a typical drift of metabolite profiles during fermentation for 0–60 h, whereas a reverse change occurred after 60 h. Of the 28 significantly different metabolites (SDMs) (VIP > 1 and p < .05) identified during pre-fermentation, 50% were enriched at 60 h including sugar, phenolic acid, and organic acid. This resulted in the highest levels of total phenolic and total acid. Pathway analysis based on SDMs indicated that the starch and sucrose metabolism, alanine, aspartate, and glutamate metabolism, and pentose phosphate pathway were most relevant to pre-fermentation (Pathway impact value > 0.1 and p < .05). The integrated metabolic pathway was generated to understand the transformation and accumulation of SDMs. Metabolite profiles at 0, 60, and 72 h were compared using S-plot. More sugar types were observed at 60 h than at 72 h. Moreover, succinic acid and 4-hydroxyphenylethanol, as metabolites of yeast, were significantly upregulated between 60 and 72 h. Therefore, 60 h is potentially optimal for pre-fermentation of black glutinous rice wine. This study rationalizes the pre-fermentation steps vital for black glutinous rice wine production.
Introduction
Glutinous rice (Oryza sativa var. glutinosa) has been used for rice wine fermentation in China for thousands of years due to its rich content of phenolic compounds and higher hydroxyl radical scavenging ability.[Citation1,Citation2] As one of the widely consumed traditional fermented alcoholic beverages in China, black glutinous rice wine has many potential medicinal properties, including antioxidant ability and a protective effect on the kidney.[Citation3,Citation4] This may be related to raw materials and fermentation. According to reports, black glutinous rice, a kind of grain resource used as both medicine and food, is rich in nutrients and shows remarkable antioxidative properties.[Citation5,Citation6] Studies in growing numbers have demonstrated that black glutinous rice has a variety of health benefits and pharmacological activities including preventing diabetic complications, inhibition of cholesterol absorption, combating chronic disease development and anti-aging of skin.[Citation7–Citation9] In addition, fermentation is not only an effective method to improve the nutritional, organoleptic, and functional properties of grain-based products,[Citation10] but is also a critical approach that decreases antinutritional factors and toxic compounds.[Citation11]
Typically, rice wine brewing can be carried out by soaking the rice, steaming, cooling, adding the starter, and fermenting.[Citation12] However, to be precise, the fermentation process includes pre- and post-fermentation steps, namely saccharification and alcoholic fermentation stages.[Citation13] As a key step in rice wine production, pre-fermentation allows the microorganisms (such as mold and yeast) in koji to grow and metabolize various enzymes rapidly.[Citation14] Under the action of these enzymes, macromolecules such as starch and proteins in the fermentation substrate can be degraded to monosaccharides and amino acids, thereby improving the sensory and nutritional properties of rice wine. Furthermore, the saccharification stage is necessary for alcoholic fermentation because yeast cannot directly utilize polysaccharides.[Citation15]
Recently, the use of metabolomic technology has become increasingly extensive to study metabolites in the fermentation process of fermented foods.[Citation16,Citation17] 1H-NMR, GC-MS, and LC-MS are some of the most widely used techniques in metabolomics.[Citation18,Citation19] However, MS-based techniques play an important role in detecting low-abundance metabolites compared to 1H-NMR.[Citation20] Metabolomics based on GC-MS has been successfully applied for the study of rice koji and rice wine, and has helped unravel the dynamic alterations in metabolite profiles as well as optimize the fermentation time.[Citation21,Citation22] However, little is known about the dynamic changes in metabolites during pre-fermentation of black glutinous rice wine. Hence, the main objective of the present study was to investigate the metabolite variations in black glutinous rice wine during pre-fermentation and to determine the optimal pre-fermentation time.
Materials and methods
Material and reagents
Z-20 Rhizopus was obtained from the Sichuan Institute of Wine Research, and black glutinous rice was provided by Yonghong Wine Industry Co., Ltd. (Guizhou, China). HPLC-grade methanol, chloroform, and pyridine were purchased from CNW Technologies (Duesseldorf, Germany) and Adamas Reagent Co., Ltd. (Shanghai, China), respectively. Folin-Ciocalteu reagent, adonitol, and n-proline (IS) were purchased from Sigma-Aldrich (Missouri, USA). BSTFA (with 1% TMCS, v/v) was purchased from Regis Technologies, Inc. (Chicago, USA). Saturated fatty acid methyl esters (FAMEs) were purchased from Dr. Ehrenstorfer (Augsburg, Germany). Trichloroacetic acid was purchased from Merck Millipore Co., Ltd. (Darmstadt, Germany). Sodium carbonate and tyrosine were purchased from Sinopharm Chemical Reagent Co., Ltd. (Shanghai, China). Methoxy amination hydrochloride was purchased from Tokey Chemical Industry Co., Ltd (Tokyo, Japan). All other chemicals and reagents used in this research were of analytical grade.
Sample preparations
Fermentation samples were prepared using black glutinous rice from the industry, three batches of black glutinous rice wine samples were produced. First, black glutinous rice (60 kg) was submerged in water (10 L) for 5 h. The water was then drained off, and the soaked rice was steamed for 20 min until the rice core disappeared and then cooled to 35°C with cold water. Lastly, the cooled rice was mixed with koji (6 kg), and fermented at 30°C for 72 h. The fermentation liquid was harvested every 12 h and stored at −80°C until analyzes. Notably, the mixture of rice and koji was collected in the unfermented samples (fermentation for 0 h) because there was no harvestable fermentation liquid.
Physicochemical analysis
To evaluate the physicochemical characteristics of black glutinous rice wine, 10 g or 10 mL of samples were extracted with 90 mL of distilled water at 120 rpm and 30°C for 1 h. pH was measured using a pH meter (Thermo, USA). Total acid contents were measured by titrating the samples with 0.1 N NaOH solution to a pH value of 8.4. The amino-type nitrogen contents were estimated by adding 36% formaldehyde solution (20 mL) to the titrated samples, followed by sample titration to pH 8.4 using 0.1 N NaOH solution. The total phenolic contents in each sample were determined using the Folin-Ciocalteu method. Briefly, 100 µL of Folin-Ciocalteu reagent (0.2 N) and 20 µL of sample aqueous extract were added in a 96-well plate and incubated at 25 ± 2°C for 6 min. Then, 30 µL of 20% (w/v) sodium carbonate (Na2CO3) was added to the mixture. After incubation for 30 min, the absorbance of the mixture was recorded at 760 nm by a multiscan spectrum (SpectraMax 190, Molecular Devices, USA). The total phenolic contents of the extracts were presented as gallic acid equivalent (GE) concentrations (ppm) per milligram.
Determination of enzymatic activities
The enzymatic activity assays include β-glucosidase, protease, cellulase, and pectinase. β-glucosidase and protease activities were conducted according to the protocols described by Seo et al. (2018).[Citation23] Firstly, 10 g or 10 mL of the harvested samples were extracted with 90 mL of distilled water at 120 rpm and 30°C for 1 h in a shaking incubator. Secondly, the mixtures were centrifuged for 5 min at 5000 rpm, 4°C. Finally, the supernatants were passed through 0.2-µm PTFE filters, and the filtered were used to evaluate enzymatic activities. Cellulase and pectinase activities were assessed using the method described by Lin et al. (2016).[Citation24] Briefly, 10 g or 10 mL samples were homogenized in 75 mL of 0.1 M sodium acetate or citrate buffer solution. The mixtures were centrifuged for 10 min at 5900 × g, 4°C, and the supernatants were used for enzymatic activity assays.
Metabolites extraction
All samples were pulverized to extract metabolites after lyophilization for 48 h. Samples of 60 ± 1 mg, extraction liquid of 480 µL (VMethanol: VH2O = 3:1), and internal standard of 20 µL (adonitol, 0.5 mg/mL stock in dH2O) were added into 2-mL EP tubes, and vortex mixed for 30 s. The mixture was homogenized in a ball mill for 4 min at 45 Hz and treated with ultrasound for 5 min (incubated in ice water). The centrifugal process was then conducted at 12,000 rpm and 4°C for 15 min; the supernatant (400 µL) was transferred to a fresh 2 mL GC/MS glass vial. Besides, 40 µL supernatant from each sample was collected as the QC sample. After the supernatant was completely dried in a vacuum concentrator (without heating), 80 µL of methoxy amination hydrochloride was added and incubated for 30 min at 80°C. Next, 100 µL of the BSTFA regent (1% TMCS, v/v) was added to the sample aliquots and incubated for 1.5 h at 70°C. Finally, 10 µL FAMEs (in chloroform) was added to the QC sample when cooling to room temperature. All samples were analyzed by a gas chromatography system coupled with a Pegasus HT time-of-flight mass spectrometer (GC-TOF-MS).
GC-TOF-MS analysis
GC-TOF-MS analysis was performed using an Agilent 7890 gas chromatography system (Agilent, Atlanta, GA) coupled with a Pegasus HT time-of-flight mass spectrometer (LECO Corporation, St. Joseph, MI, USA). The system utilized a DB-5MS capillary column coated with 5% diphenyl cross-linked with 95% dimethylpolysiloxane (30 m × 250 µm inner diameter, 0.25 µm film thickness; J&W Scientific, Folsom, CA, USA). A 1-µL aliquot of the analyte was injected in the splitless mode. Helium was used as the carrier gas, the front inlet purge flow was 3 mL/min, and the gas flow rate through the column was 1 mL/min. The initial temperature was kept at 50°C for 1 min, then raised to 310°C at a rate of 10°C/min, and kept for 8 min at 310°C. The injection, transfer line, and ion source temperatures were 280, 280, and 250°C, respectively. The energy was −70 eV in the electron impact mode. Mass spectrometry data were acquired in the full-scan mode with an m/z range of 50–500 at a rate of 20 spectra per second after a solvent delay of 6.27 min.
Data analysis
Chroma TOF 4.3X software from LECO Corporation and LECO-Fiehn Rtx5 database were used for raw peaks exacting, data baseline filtering and calibration of the baseline, peak alignment, deconvolution analysis, peak identification, and integration of the peak area.[Citation25] Both mass spectrum match and retention index match were considered in the metabolite identification. Peaks detected in < 50% of QC samples or RSD > 30% in QC samples were removed.[Citation26] The data including the compound name, peak area, retention time, similarity to metabolites in the database, and mass (m/z) were ultimately imported into Microsoft Excel.
Statistical analysis
SIMCA 14.1 software (Umetrics, Umea, Sweden) was used for multivariate statistical analysis. To clearly understand the changes in metabolite composition during fermentation, principal component analysis (PCA) and orthogonal partial least squares-discriminant analysis (OPLS-DA) were used to analyze the metabolic patterns. Based on the OPLS-DA model, the variable importance in the projection (VIP) values of metabolites were calculated. Meanwhile, significant differences among metabolites (p < .05) were tested by one-way analysis of variance using SPSS 22.0 statistical software (Chicago, USA). MetaboAnalyst, a free and web-based tool with a high-quality KEGG metabolic pathway, was used for pathway analysis (http://www.metaboanalyst.ca).
Results and discussion
Dynamic changes in physicochemical properties
Physicochemical properties are important indicators used to evaluate the degree of fermentation. shows the differences in properties among samples with different fermentation times. In contrast with the trend of total acid contents, pH value was continuously decreased (panels A and B of ). Previous studies have proved that higher levels of amino acids in rice wine are mainly obtained from the hydrolysis of proteins in raw materials and koji.[Citation27] The trend of amino-type nitrogen contents was first increased and then decreased along with the prolonging of fermentation time (); this was probably because some of the amino acids were consumed by yeast for the biosynthesis of enzymes and structural proteins.[Citation21] Polyphenols of rice wine are mainly derived from their plant materials, and rice wines made from different raw materials are significantly different in total phenolic contents.[Citation1] In , the total phenolic contents were first increased and then decreased, and reached a peak after 60 h of fermentation. Saharan et al. (2017)[Citation28] reported a similar trend during cereal fermentation and they suggested that the decrease in total phenolic contents probably caused by degradation. In addition, the reduction of total phenolic contents also might be related to the utilization of phenolic compounds by microorganisms.[Citation29]
Dynamic changes in enzymatic activities
The enzymatic activities of β-glucoamylase, protease, pectinase, and cellulase are shown in . Overall, the activity of these enzymes increased along with the prolonging of fermentation time, as also observed by other authors.[Citation22,Citation30] Enhancement of enzymatic activity helps to break down the starch, protein and fat in the substrate into small molecules and to change the metabolite profiles found in fermented foods.[Citation31]
As one of the most important enzymes in the saccharification stage of rice wine, β-glucosidase produces glucose by destruction of glucosidic linkages in polysaccharides.[Citation32] Regarding proteases, several studies have demonstrated the positive correlation between amino acid levels and protease activity.[Citation30] Therefore, the increase of amino-type nitrogen contents was related to protease activity (). Cellulase is a complex enzyme with high yield and activity that could degrade stubborn cellulase effectively and generate oligosaccharides of different lengths.[Citation33] Cellulose in the substrate can be completely degraded to glucose when cellulase works synergistically with other enzymes.[Citation34] For example, pectinase and cellulase are available to degrade pectin and cellulose in the plant cell wall, and promote the release of carbohydrates, esters, vitamins, and proteins. Moreover, enhancement of total phenolic contents () could be associated with an increase in the activity of pectinase, cellulase, and β-glucosidase.[Citation28,Citation35] Therefore, enzymatic activity is essential for the quality and sensory properties of black glutinous rice wine.
Multivariate analysis of black glutinous rice wine
Multivariate analysis based on GC-TOF-MS datasets was conducted to investigate the metabolite differences associated with fermentation time. The principal component analysis (PCA) score plot () showed a total variance of 50.3% (PC1, 36.3%; PC2, 14.0%) with plot satisfaction values for the X variables of 0.667 (R2X) and a prediction accuracy of 0.336 (Q2), indicating that the PCA model has a poor fitting and predictability.[Citation36] To maximize the difference between samples and enhance the fitting and predictability, the OPLS-DA model was used to eliminate the noncorrelated variation in X variables to Y variables or for variability in X that is orthogonal to Y.[Citation37] Compared to the PCA model, improved fitting (R2X = 0.865) and predictability (Q2 = 0.669) were observed in the OPLS-DA model (). In addition to fermentation for 72 h, metabolites profiles were distributed according to fermentation time on both PCA and OPLS-DA score plots. More specifically, metabolite profiles were distributed along PC1 (from the left to the right) and OPLS1 (from the right to the left), which indicated that continuous metabolic changes were occurred during fermentation from 0 to 60 h. Hence, fermentation for 60 h may be a key turning point in the production of black glutinous rice wine.
Identification of significantly different metabolites
Generally, significantly different metabolites (SDMs) were determined based on the variable importance in projection (VIP > 1.0) values and p-value (p < .05).[Citation38] In total, 28 SDMs were screened, including 12 sugars and sugar alcohols, 7 organic acids, 4 amino acids, 3 phenolic acids, and 2 fatty acids. All the relevant information about SDMs is shown in the Supplementary Table S1 and Figure S1. A heatmap was drawn based on the relative contents to reveal the pairwise correlation between metabolites and fermentation time. As shown in , SDMs can be roughly divided into four parts (I-IV) with an increase in fermentation time. Six SDMs in part I were enriched before fermentation and decreased significantly after fermentation for 24 h, indicating that these metabolites primarily originated from black glutinous rice. Part II contains 5 SDMs, and their contents were not the lowest before fermentation and reached the highest after fermentation for 36 or 48 h. This showed that they derived from both raw material and fermentation. The SDMs in parts III and IV may be primarily derived from microbial metabolism due to their lower concentrations compared to those of parts I and II before fermentation.
Figure 4. The relative content of SDMs in black glutinous rice wine at different fermentation times. The relative contents are represented as log10-transformed peak areas. Red and green symbolize higher and lower relative content, respectively
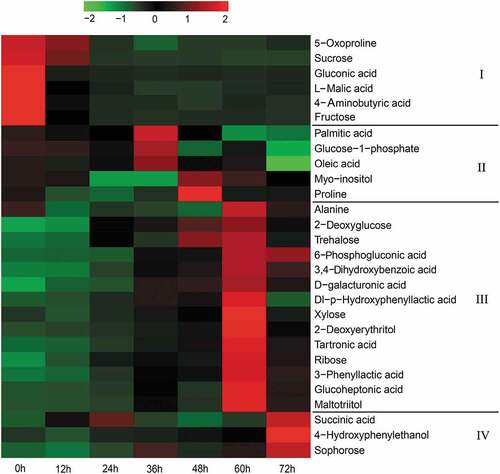
In the pre-fermentation stage of brewing rice wine, enzymatic hydrolysis and saccharification of rice starch are the main biochemical reactions.[Citation13] Therefore, sugars and sugar alcohols were the most abundant metabolites in this study, they contribute to the sweetness of black glutinous rice wine. During fermentation, sugars are mostly consumed as the main carbon sources, providing energy for the growth of microorganisms.[Citation31] Polysaccharides cannot be directly absorbed or utilized, and need to be degraded into monosaccharides by certain microbial enzymes, such as α-amylase, β-amylase, and β-glucosidase.[Citation39] As shown in , sucrose was mostly exhausted after 24 h of fermentation, which was consistent with a sharp increase in β-glucosidase activity observed during this period (). As the fermentation progressed, the levels of xylose, ribose, 2-deoxyglucose, and trehalose reached the maximum at 60 h of fermentation. These monosaccharides can be absorbed and utilized by yeast. Trehalose, the second most abundant disaccharide in Korean rice wine (Makgeolli), not only acts as a reserve carbohydrate but also enhances the resistance of the cells to adverse conditions.[Citation40] Sugar alcohols can be derived from plant material and microbial fermentation.[Citation41] For example, xylose can be converted to xylitol by fungi through NADPH-consuming reactions.[Citation42] However, highly concentrated sugar alcohols are usually present in small amounts in alcoholic beverages.[Citation21]
Organic acids are called “wine skeletons” due to their contribution to flavor and taste.[Citation43] The organic acid in the wine can originate from raw materials and/or microbial metabolism. Seo et al. (2016)[Citation21] suggested that the key organic acids (lactic, malic, and succinic acids) in Makgeolli are primarily produced by yeast metabolism. However, in the present study, L-malic acid appeared to originate mainly from black glutinous rice. Consistent with the results depicted in , most of the organic acids such as 6-phosphogluconic, D-galacturonic, tartaric, and glucoheptonic acids were found to be enriched at 60 h of fermentation, resulting in the highest total acid content. Succinic acid is an important flavor component in Japanese rice wine, and its formation comprises the following two pathways: 1) alpha-ketoglutaric acid oxidation through the TCA cycle and 2) reduction of fumarate under anaerobic conditions.[Citation44] In addition, succinic acid has been identified as a taste active ingredient in fermented foods due to its lower taste threshold.[Citation45]
Amino acids play a significant role in the flavor and sensory quality of rice wine. A total of four amino acids were determined as SDMs in the present study. A functional ingredient widely found in Chinese rice wine is 4-Aminobutyric acid (GABA), and it is usually converted from glutamate by glutamate decarboxylase.[Citation46] GABA has been confirmed to possess several physiological activities, such as neurotransmission, antianxiety, and improving brain function and long-term memory.[Citation47] According to Gong et al. (2017)[Citation48], the increase of GABA in rice wine mainly occurs in the post-fermentation stage. Pyroglutamate, also known as 5-oxoproline, is formed by cyclization of glutamic acid or glutamine. A previous study has proved that high concentration of 5-oxoproline exerts a negative effect on the aroma of wine.[Citation49] Proline and alanine are the two main amino acids in Shaoxing rice wine.[Citation50] In addition to contributing to sweetness, proline and alanine have been confirmed to exert multiple physiological effects, including preventing apoptosis and scavenging free radicals.[Citation51,Citation52]
Phenolic compounds in rice wine are mainly derived from plant material that are associated with the color, aroma, and sensory quality of the product.[Citation1] Que et al. (2006)[Citation53] compared the antioxidant activity of five Chinese rice wines and found a strongly positive correlation between antioxidant activity and total phenolic content. Additionally, the wine prepared from Nuomi (glutinous rice) showed the highest antioxidant activity. In the present study, three polyphenols, namely, 3,4-dihydroxybenzoic, dl-p-hydroxyphenyl lactic, and 3-phenyllactic acids, reached a peak after 60 h of fermentation (), and this result was in accordance with the dynamics of the total polyphenol content (). Among these polyphenols, 3,4-dihydroxybenzoic acid is widely present in rice wine and other plant-derived beverages and can prevent the transformation from urea/citrulline to ethyl carbamate, which is one of the most harmful carcinogenic compounds found in rice wine.[Citation54]
Fatty acids are important for the flavor and taste of Chinese rice wine.[Citation55] According to Xiao et al. (2015)[Citation56], most fatty acids in rice wine are released or produced by yeast during the fermentation process; however, some originate from raw materials. Here, palmitic and oleic acids were found to be derived from both black glutinous rice and fermentation (). Palmitic acid is one of the most abundant fatty acids in Makgeolli[Citation40], and it plays a crucial role in early human development.[Citation57] Oleic acid is a common monounsaturated fatty acid present in rice wine. It has the potential to affect the growth of yeast, thereby influencing the flavor and taste of rice wine.[Citation58]
Integrated analysis of metabolic pathways
Metabolic pathway analysis of SDMs was performed using MetaboAnalyst 4.0. In total, 18 metabolic pathways were obtained by inputting the compound name of SDMs into the KEGG database. Among all the metabolic pathways, starch and sucrose metabolism, alanine, aspartate and glutamate metabolism, and pentose phosphate pathway were the most related to pre-fermentation of black glutinous rice wine (pathway impact > 0.1 and p < .05) (Supplementary Table S2 and Figure S2).
Fermentation of rice wine usually begins with the degradation of polysaccharides (). The sucrose content was the highest in unfermented samples and decreased significantly within 24 h of fermentation. The reason may be that enhanced β-glucoamylase activity accelerated the transformation of starch and sucrose metabolic pathway and the production of fructose and glucose.[Citation59] Subsequently, fructose was involved in the inositol phosphate metabolism pathway and resulted in increased in myo-inositol levels. Simultaneously, glucose-1-phosphate was produced by phosphorylation of glucose and was converted by the glycolysis/gluconeogenesis pathway to glucose-6-phosphate, which then entered the pentose phosphate pathway. In this pathway, glucose-6-phosphate and gluconic acid were transformed to 6-phosphategluconic acid and ultimately generated ribose. Therefore, the level of 6-phosphategluconic acid and ribose increased as the gluconic acid level decreased. As the glycolysis/gluconeogenesis pathway progressed, glucose was finally transformed into pyruvate, which participated in the TCA cycle by acetyl-CoA and produced various organic acids. Among them, 2-oxoglutaric acid can be converted to proline and 4-aminobutyric acid by the alanine, aspartic acid, and glutamic acid metabolic, whereas 4-aminobutyric acid eventually generated succinic acid. Then, succinic acid was further transformed into dl-p-hydroxyphenyl lactic acid and 4-hydroxyphenylethanol.
Figure 5. Integrated metabolic pathway of black glutinous rice wine during pre-fermentation. Beneath each metabolite, the color on a white-to-red gradient indicates the fold change with respect to unfermented black glutinous rice, white and red represent the minimum and maximum values, respectively. The SDMs (VIP > 1 and p < .05) screened by the OPLS-DA model are shown in red, and the intermediates are shown in black
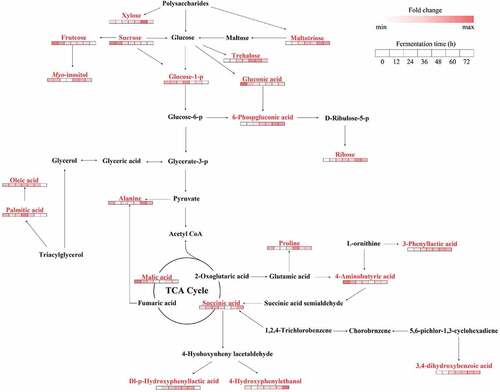
Optimization analysis of fermentation time
To further optimize the pre-fermentation time of black glutinous rice wine, the OPLS-DA model was used to compare and analyze the metabolite profiles at 0, 60, and 72 h of fermentation. The samples with different fermentation times can be clearly distinguished due to well-fitting and predictability (higher R2 and Q2) of score plots (panels A, C, and E of ). Therefore, the fermentation time has an essential influence on the composition of metabolites in black glutinous rice wine. The S-plot allows us to identify significantly different metabolites in the samples. As shown in panels B and D of , the levels of various metabolites were significantly reduced, especially fructose, sucrose, glucose-1-phosphate, and L-malic acid. Also, more sugars were observed after fermentation for 60 h including xylose, ribose, 2-deoxyglucose, trehalose, raffinose, and maltotriose (). However, within the next 12 h, various metabolites such as xylose were significantly downregulated, and only 1,5-anhydroglucitol, succinic acid, and 4-hydroxyphenylethanol were significantly upregulated (). According to previous reports, succinic acid is the predominant organic acid produced by yeast during the alcoholic fermentation stage, and 4-hydroxyphenylethanol is also derived from yeast synthesis.[Citation60,Citation61] Hence, black glutinous rice wine may have entered the post-fermentation stage after fermentation for 60 h.
Figure 6. The OPLS-DA score plot (A, C, E) and S-plot (B, D, F) for black glutinous rice wine at 0 h, 60 h, and 72 h of fermentation obtained by GC-TOF-MS. Significantly different metabolites were labeled in the S-plot as blue for downregulated, red for upregulated, and green for no significant change
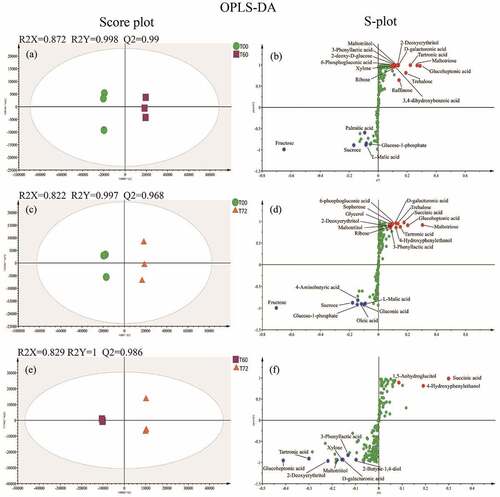
Conclusion
As summarized in present study, metabolomics analysis based on GC-TOF-MS technology is a powerful tool for the optimization of fermentation time. Regarding to the physicochemical property, the enzymatic activity, and the metabolite profile, the black glutinous rice wines with diverse fermentation time were significantly different. Among all the 7 time points, 60 h and 72 h were characterized by the optimum physicochemical property and enzymatic activity, respectively. However, both PCA and OPLS-DA results showed that better metabolite profile was observed at 60 h rather than at 72 h, especially more types and levels of sugar. This result was also supported by S-plot, which indicates that the ultimate goal of pre-fermentation for accumulation sugars was accomplished properly. Thus, we concluded that 60 h was an optimal time for black glutinous rice wine fermentation.
Supplemental Material
Download MS Word (2.4 MB)Supplementary material
Supplemental data for this article can be accessed on the publisher’s website.
Additional information
Funding
References
- Cai, H. Y.; Zhang, Q.; Shen, L. Z.; Luo, J.; Zhu, R.; Mao, J.; Zhao, M.; Cai, C. Phenolic Profile and Antioxidant Activity of Chinese Rice Wine Fermented with Different Rice Materials and Starters. LWT–Food Sci. Technol. 2019, 111, 226–234. DOI: 10.1016/j.lwt.2019.05.003.
- Rao, S.; Yang, Z.; Gao, L.; Song, Y.; Fang, W. M. Effect of Rice Varieties on Antioxidant Activity of Chinese Rice Wine. China Brewing. 2014, 33(8), 75–79.
- Su, W.; Qi, Q.; Zhao, X.; MU, Y. C.; Qiu, S. Y.; Bai, W. D. Variation and Biological Activity of Polyphenols during Brewing Process of Black Glutinous Rice Wine. Guizhou Agric. Sci. 2017, 45(08), 112–117.
- Qi, Q.; Li, J. W.; Mu, Y. C.; Su, W. Experimental Study on the Effect of Black Glutinous Rice Health Wine on Tonifying Kidney in Mice. J. Biobased Mater. Bioenergy. 2018, 12(1), 148–152. DOI: 10.1166/jbmb.2018.1752.
- Kim, J. Y.; Seo, W. D.; Park, D. S.; Jang, K. C.; Choi, K. J.; Kim, S. Y.; Oh, S. H.; Ra, J. E.; Yi, G.; Park, S. K.; et al. Comparative Studies on Major Nutritional Components of Black Waxy Rice with Giant Embryos and Its Rice Bran. Food Sci. Biotechnol. 2013, 22(1), 121–128. DOI: 10.1007/s10068-013-0057-1.
- Phonsakhan, W.; Kong-Ngern, K. A Comparative Proteomic Study of White and Black Glutinous Rice Leaves. Electron. J. Biotechnol. 2015, 18(1), 29–34. DOI: 10.1016/j.ejbt.2014.11.005.
- Yawadio, R.; Tanimori, S.; Morita, N. Identification of Phenolic Compounds Isolated from Pigmented Rices and Their Aldose Reductase Inhibitory Activities. Food Chem. 2007, 101(4), 1616–1625. DOI: 10.1016/j.foodchem.2006.04.016.
- Yao, S. L.; Xu, Y.; Zhang, Y. Y.; Lu, Y. H.; Rice, B. Anthocyanins Induce Inhibition of Cholesterol Absorption in Vitro. Food Funct. 2013, 4(11), 1602–1608. DOI: 10.1039/C3FO60196J.
- Phetpornpaisan, P.; Tippayawat, P.; Jay, M.; Sutthanut, K. A Local Thai Cultivar Glutinous Black Rice Bran: A Source of Functional Compounds in Immunomodulation, Cell Viability and Collagen Synthesis, and Matrix Metalloproteinase-2 and −9 Inhibition. J. Funct. Foods. 2014, 7, 650–661. DOI: 10.1016/j.jff.2013.12.020.
- Ahmed, I. A. M.; Al-Juhaimi, F. Y.; Bekhit, E. D. A. Fermentation of Grains[M]. Ref. Module Food Sci. 2018. DOI: 10.1016/B978-0-08-100596-5.21657-0.
- Wood, B. J. B.;. Fermentation: Origins and Applications. Encycl. Food Grains. 2016, 176–182. DOI: 10.1016/b978-0-12-394437-5.00135-2.
- Cai, H.; Zhang, T.; Zhang, Q.; Luo, J.; Cai, C.; Mao, J. Microbial Diversity and Chemical Analysis of the Starters Used in Traditional Chinese Sweet Rice Wine. Food Microbiol. 2018, 73, 319–326. DOI: 10.1016/j.fm.2018.02.002.
- Zhu, Y. B.; Zhang, J. H.; Shi, Z. P.; Mao, Z. G. Optimization of Operating Conditions in Rice Heat Blast Process for Chinese Rice Wine Production by Combinational Utilization of Neural Network and Genetic Algorithms. J. Inst. Brew. 2004, 110(2), 117–123. DOI: 10.1002/j.2050-0416.2004.tb00190.x.
- Suganuma, T.; Fujita, K.; Kitahara, K. Some Distinguishable Properties between Acid-Stable and Neutral Types of α-Amylases from Acid-Producing Koji. J. Biosci. Bioeng. 2007, 104(5), 353–362. DOI: 10.1263/jbb.104.353.
- Ferdouse, J.; Yamamoto, Y.; Taguchi, S.; Yoshizaki, Y.; Takamine, K.; Kitagaki, H. Glycosylceramide Modifies the Flavor and Metabolic Characteristics of Sake Yeast. Peer J. 2018, 6(9), 4768–4784. DOI: 10.7717/peerj.4768.
- Wu, Z.; Mao, Y.; Zhang, X.; Weng, P. Symbolic Metabolite Analysis of Pickled Wax Gourd in Eastern China by 1H-NMR Spectroscopy and Multivariate Data. Int. J. Food Prop. 2015, 19(9), 2052–2062. DOI: 10.1080/10942912.2015.1099044.
- Park, S. E.; Yoo, S. A.; Seo, S. H.; Lee, K. I.; Na, C. S.; Son, H. S. GC-MS Based Metabolomics Approach of Kimchi for the Understanding of Lactobacillus Plantarum Fermentation Characteristics. LWT–Food Sci. Technol. 2016, 68, 313–321. DOI: 10.1016/j.lwt.2015.12.046.
- Alañón, M. E.; Pérez-Coello, M. S.; Marina, M. L. Wine Science in the Metabolomics Era. TrAC, Trends Anal. Chem. 2015, 74, 1–20. DOI: 10.1016/j.trac.2015.05.006.
- More, T.; Roychoudhury, S.; Gollapalli, K.; Patel, S. K.; Gowda, H.; Chaudhury, K.; Rapole, S. Metabolomics and Its Integration with Systems Biology: PSI 2014 Conference Panel Discussion Report. J. Proteomics. 2015, 127, 73–79. DOI: 10.1016/j.jprot.2015.04.024.
- Scano, P.; Murgia, A.; Pirisi, F. M.; Caboni, P. A Gas Chromatography-mass Spectrometry-based Metabolomic Approach for the Characterization of Goat Milk Compared with Cow Milk. J. Dairy Sci. 2014, 97(10), 6057–6066. DOI: 10.3168/jds.2014-8247.
- Seo, S. H.; Park, S. E.; Yoo, S. A.; Lee, K. I.; Na, C. S.; Son, H. S. Metabolite Profiling of Makgeolli for the Understanding of Yeast Fermentation Characteristics during Fermentation and Aging. Process Biochem. 2016, 51(10), 1363–1373. DOI: 10.1016/j.procbio.2016.08.005.
- Lee, D. E.; Lee, S.; Singh, D.; Jang, E. S.; Shin, H. W.; Moon, B. S.; Lee, C. H. Time-resolved Comparative Metabolomes For, Koji, Fermentation with Brown-, White-, and Giant Embryo-rice. Food Chem. 2017, 231, 258–266. DOI: 10.1016/j.foodchem.2017.03.119.
- Seo, H. S.; Lee, S.; Singh, D.; Shin, H. W.; Cho, S. A.; Lee, C. H. Untargeted Metabolite Profiling for Koji-fermentative Bioprocess Unravels the Effects of Varying Substrate Types and Microbial Inoculation. Food Chem. 2018, 266, 161–169. DOI: 10.1016/j.foodchem.2018.05.048.
- Lin, W.; Song, J.; Hu, W.; Miao, J.; Gao, X. Relationship between Extracellular Cellulase, Pectinase and Xylanase Activity of Isolated\r, Aspergillus Oryzae\r, Strains Grown on Koji and the Umami-Tasting Amino Acid Content of Soy Sauce. Food Biotechnol. 2016, 30(4), 278–291. DOI: 10.1080/08905436.2016.1244768.
- Kind, T.; Wohlgemuth, G.; Lee, D. Y.; Lu, Y.; Palazoglu, M.; Shahbaz, S.; FiehnLib:, F. O.; Spectral, M. And Retention Index Libraries for Metabolomics Based on Quadrupole and Time-of-flight Gas Chromatography/Mass Spectrometry. Anal. Chem. 2009, 81(24), 10038–10048. DOI: 10.1021/ac9019522.
- Dunn, W. B.; Broadhurst, D.; Begley, P.; Zelena, E.; Francis-McIntyre, S.; Anderson, N.; Goodacre, R. Procedures for Large-scale Metabolic Profiling of Serum and Plasma Using Gas Chromatography and Liquid Chromatography Coupled to Mass Spectrometry. Nat. Protoc. 2011, 6(7), 1060–1083. DOI: 10.1038/nprot.2011.335.
- Shen, F.; Niu, X.; Yang, D.; Ying, Y.; Li, B.; Zhu, G.; Wu, J. Determination of Amino Acids in Chinese Rice Wine by Fourier Transform Near-infrared Spectroscopy. J. Agric. Food Chem. 2010, 58, 9809–9816. DOI: 10.1021/jf1017912.
- Saharan, P.; Sadh, P. K.; Singh Duhan, J. Comparative Assessment of Effect of Fermentation on Phenolics, Flavanoids and Free Radical Scavenging Activity of Commonly Used Cereals. Biocatal. Agric. Biotechnol. 2017, 12, 236–240. DOI: 10.1016/j.bcab.2017.10.013.
- Wang, J.; Cao, F.; Su, E.; Wu, C.; Zhao, C.; Ying, R. Improving Flavonoid Extraction from Ginkgo Biloba Leaves by Pre-fermentation Processing. J. Agric. Food Chem. 2013, 61(24), 5783–5791. DOI: 10.1021/jf400712n.
- Lee, S.; Lee, D. E.; Singh, D.; Lee, C. H. Metabolomics Reveal Optimal Grain Preprocessing (Milling) toward Rice Koji Fermentation. J. Agric. Food Chem. 2018, 66(11), 2694–2703. DOI: 10.1021/acs.jafc.7b05131.
- Lee, D.; Lee, S.; Jang, E.; Shin, H.; Moon, B.; Lee, C. Metabolomic Profiles of Aspergillus Oryzae and Bacillus Amyloliquefaciens during Rice Koji Fermentation. Molecules. 2016, 21(6), 773–787. DOI: 10.3390/molecules21060773.
- Zhang, Y. C.; Lee, J. H.; Vodovotz, Y.; Schwartz, S. J. Changes in Distribution of Isoflavones and β-Glucosidase Activity during Soy Bread Proofing and Baking. Cereal. Chem. 2004, 81, 741–745. DOI: 10.1094/CCHEM.2004.81.6.741.
- Bayer, E. A.; Lamed, R. The Cellulose Paradox: Pollutant Par Excellence And/or a Reclaimable Natural Resource? Biodegradation. 1992, 3(2–3), 171–188. DOI: 10.1007/BF00129082.
- Reese, E. T.; Siu, R. G. H.; Levinson, H. S. The Biological Degradation of Soluble Cellulose Derivatives and Its Relationship to the Mechanism of Cellulose Hydrolysis. J. Bacteriol. 1950, 59(4), 485–497. DOI: 10.1016/0021-9169(50)90025-0.
- Castilho, L. R.; Medronho, R. A.; Alves, T. L. M. Production and Extraction of Pectinases Obtained by Solid State Fermentation of Agroindustrial Residues with Aspergillus Niger. Bioresour. Technol. 2000, 71(1), 45–50. DOI: 10.1016/S0960-8524(99)00058-9.
- Szeto, S. S.; Reinke, S. N.; Sykes, B. D.; Lemire, B. D. Mutations in the Saccharomyces Cerevisiae Succinate Dehydrogenase Result in Distinct Metabolic Phenotypes Revealed through 1H NMR-based Metabolic Footprinting. J. Proteome Res. 2010, 9(12), 6729–6739. DOI: 10.1021/pr100880y.
- Sun, X. M.; Yu, X. P.; Liu, Y.; Xu, L.; Di, D. L.; Bootstrap, C. Uninformative Variable Elimination: Chemometric Identification of Metabonomic Biomarkers by Nonparametric Analysis of Discriminant Partial Least Squares. Chemom. Intell. Lab. Syst. 2012, 115, 37–43. DOI: 10.1016/j.chemolab.2012.04.006.
- Hao, R. J.; Du, X. D.; Yang, C. Y.; Deng, Y. W.; Zheng, Z.; Wang, Q. H. Integrated Application of Transcriptomics and Metabolomics Provides Insights into Unsynchronized Growth in Pearl Oyster Pinctada Fucata Martensii. Sci. Total Environ. 2019, 666, 46–56. DOI: 10.1016/j.scitotenv.2019.02.221.
- Gänzle Michael, G.;. Enzymatic and Bacterial Conversions during Sourdough Fermentation. Food Microbiol. 2014, 37, 2–10. DOI: 10.1016/j.fm.2013.04.007.
- Son, E. Y.; Lee, S. M.; Kim, M.; Seo, J. A.; Kim, Y. S. Comparison of Volatile and Non-volatile Metabolites in Rice Wine Fermented by Koji Inoculated with Saccharomycopsis Fibuligera and Aspergillus Oryzae. Food Res. Int. 2018, 109, 596–605. DOI: 10.1016/j.foodres.2018.05.008.
- Godswill, A. C.;. Sugar Alcohols: Chemistry, Production, Health Concerns and Nutritional Importance of Mannitol, Sorbitol, Xylitol, and Erythritol. Int. J. Adv. Res. 2017, 3, 31–66.
- Fernandes, S.; Murray, P. G. Metabolic Engineering for Improved Microbial Pentose Fermentation. Bioeng. Bugs. 2010, 1, 424–428. DOI: 10.4161/bbug.1.6.12724.
- Zhang, X. K.; Lan, Y. B.; Zhu, B. Q.; Xiang, X. F.; Duan, C. Q.; Shi, Y. Changes in Monosaccharides, Organic Acids and Amino Acids during Cabernet Sauvignon Wine Ageing Based on a Simultaneous Analysis Using Gas Chromatography-Mass Spectrometry. J. Sci. Food Agric. 2017, 98(1), 104–112. DOI: 10.1002/jsfa.8444.
- Arikawa, Y.; Kuroyanagi, T.; Shimosaka, M.; Muratsubaki, H.; Enomoto, K.; Kodaira, R.; Okazaki, M. Effect of Gene Disruptions of the TCA Cycle on Production of Succinic Acid in Saccharomyces Cerevisiae. J. Biosci. Bioeng. 1999, 87(1), 28–36. DOI: 10.1016/s1389-1723(99)80004-8.
- Zhang, J.; Yi, Y.; Pan, D. D.; Zhou, G. H.; Wang, Y.; Dang, Y. L.; Cao, J. X. 1 H NMR-based Metabolomics Profiling and Taste of Boneless Dry-cured Hams during Processing. Food Res. Int. 2019, 122, 114–122. DOI: 10.1016/j.foodres.2019.04.005.
- Liu, T.; Zhou, Y.; Zhu, Y.; Song, M.; Li, B. B.; Shi, Y.; Gong, J. Study of the Rapid Detection of γ-Aminobutyric Acid in Rice Wine Based on Chemometrics Using near Infrared Spectroscopy. J. Food Sci. Technol. 2015, 52, 5347–5351. DOI: 10.1007/s13197-014-1576-3.
- Gibbs, M. E.; Bowser, D. N. Astrocytes and Interneurons in Memory Processing in the Chick Hippocampus: Roles for G-Coupled Protein Receptors, GABA(B) and mGluR1. Neurochem. Res. 2009, 34(10), 1712–1720. DOI: 10.1007/s11064-009-9980-1.
- Gong, J.; Huang, J.; Xiao, G.; You, Y.; Yuan, H.; Chen, F.; Li, B. Determination of γ-Aminobutyric Acid in Chinese Rice Wines and Its Evolution during Fermentation. J. Inst. Brew. 2017, 123(3), 417–422. DOI: 10.1002/jib.431.
- Pfeiffer, P.; König, H. Pyroglutamic Acid: A Novel Compound in Wines. In Biology of Microorganisms on Grapes, in Must and in Wine, Springer-Verlag Berlin Heidelberg. 2009; pp 233–240. DOI: 10.1007/978-3-540-85463-0_12.
- Chen, L.; Liu, S.; Tang, Y.; Han, X.; Zhou, Z.; Zou, H.; Mao, J. Changes in Flavor Components during Fermentation Process of Mechanically Produced Shaoxing Rice Wine. Food Sci. 2018, 39(14), 221–228. DOI: 10.7506/spkx1002-6630-201814033.
- Lee, D. E.; Shin, G. R.; Lee, S.; Jang, E. S.; Shin, H. W.; Moon, B. S.; Lee, C. H. Metabolomics Reveal that Amino Acids are the Main Contributors to Antioxidant Activity in Wheat and Rice Gochujangs (Korean Fermented Red Pepper Paste). Food Res. Int. 2016, 87, 10–17. DOI: 10.1016/j.foodres.2016.06.015.
- Lee, S. Y.; Lee, S.; Lee, S.; Oh, J. Y.; Jeon, E. J.; Ryu, H. S.; Lee, C. H. Primary and Secondary Metabolite Profiling of Doenjang, a Fermented Soybean Paste during Industrial Processing. Food Chem. 2017, 165, 157–166. DOI: 10.1016/j.foodchem.2014.05.089.
- Que, F.; Mao, L.; Pan, X. Antioxidant Activities of Five Chinese Rice Wines and the Involvement of Phenolic Compounds. Food Res. Int. 2006, 39(5), 0–587. DOI: 10.1016/j.foodres.2005.12.001.
- Zhou, W. Y.; Fang, R. S.; Chen, Q. H. Effect of Gallic and Protocatechuic Acids on the Metabolism of Ethyl Carbamate in Chinese Yellow Rice Wine Brewing. Food Chem. 2017, 233, 174–181. DOI: 10.1016/j.foodchem.2017.04.113.
- Cao, Y.; Xie, G.; Wu, C.; Lu, J. A Study on Characteristic Flavor Compounds in Traditional Chinese Rice Wine-Guyue Longshan Rice Wine. J. Inst. Brew. 2010, 116(2), 182–189. DOI: 10.1002/j.2050-0416.2010.tb00416.x.
- Xiao, Z.; Xin, D.; Zhu, J.; Yu, H. Classification of Chinese Rice Wine according to Geographic Origin and Wine Age Based on Chemometric Methods and SBSE-TD-GC-MS Analysis of Volatile Compounds. Food Sci. Technol. Res. 2015, 21(3), 371–380. DOI: 10.3136/fstr.21.371.
- Innis, S. M.;. Palmitic Acid in Early Human Development. Crit. Rev. Food Sci. Nutr. 2015, 56(12), 1952–1959. DOI: 10.1080/10408398.2015.1018045.
- Kang, B. S.; Lee, J. E.; Park, H. J. Electronic Tongue-Based Discrimination of Korean Rice Wines (Makgeolli) Including Prediction of Sensory Evaluation and Instrumental Measurements. Food Chem. 2014, 151, 317–323. DOI: 10.1016/j.foodchem.2013.11.084.
- Chi, Z.; Chi, Z.; Liu, G.; Zhang, T. Saccharomycopsis Fibuligera and Its Applications in Biotechnology. Biotechnol. Adv. 2009, 27(4), 423–431. DOI: 10.1016/j.biotechadv.2009.03.003.
- Futagami, T.; Mori, K.; Yamashita, A.; Wada, S.; Kajiwara, Y.; Takashita, H.; Omori, T.; Takegawa, K.; Tashiro, K.; Kuhara, S.; et al. Genome Sequence of the White Koji Mold Aspergillus Kawachii IFO 4308, Used for Brewing the Japanese Distilled Spirit Shochu. Eukaryotic Cell. 2011, 10(11), 1586–1587. DOI: 10.1128/EC.05224-11.
- Jackson, R. S.;. Chemical Constituents of Grapes and Wine. In Wine Science; Taylor, S. L., Ed.; Academic Press: San Diego, 2000; pp 232–271.