ABSTRACT
The present study investigated whether changes in diet associated with an increased intake of micronutrients with antioxidant potential could affect reactive oxygen species (ROS) generation determined by EPR-spectroscopy. Out of initial 16 participants, 6 participants (28.3 ± 6.8 years) demonstrated a nutritional behavior below current national recommendations and were instructed to follow a mixed healthy diet for 2 weeks. ROS formation and nutrient intake were measured before and after the intervention and nutrient intake was quantified by nutritional protocols. The results indicated that ROS generation significantly decreased (p < .05). This was accompanied by a significantly increased intake of vitamin C (p < .05), vitamin E (p < .05), β-carotene (p < .01). This study showed that mixed healthy diet increased dietary intake of antioxidant vitamins and reduced ROS concentrations. The results indicated that EPR spectroscopy could be a useful tool to identify health-related effects of dietary regimens on ROS.
Introduction
Reactive oxygen species (ROS) is a collective term that describes chemical species that are formed upon incomplete reduction of oxygen.[Citation1,Citation2] These highly reactive molecules play a crucial role in human physiological and pathophysiological processes. Physiological concentrations of ROS promote muscular adaptations to exercise by activating anabolic signaling cascades.[Citation3] Furthermore, ROS are important for the amplification of immune responses[Citation4] or the regulation of cell adhesion and migration.[Citation5] However, supra-physiological levels of ROS have been demonstrated to modify proteins, DNA and lipids (oxidative stress) and initiate apoptosis.[Citation6] Consequently, an increased formation of ROS has shown to be associated with chronic inflammatory diseases such as coronary heart disease, osteoarthritis[Citation7] and rheumatoid arthritis[Citation8] as well as neurodegenerative diseases such as Parkinson’s and Alzheimer’s disease.[Citation6]
To counteract oxidative stress, antioxidant compounds are important to scavenge high ROS concentrations. The antioxidant system consists of enzymatic antioxidants such as superoxide dismutase (SOD), catalase (CAT), glutathione peroxidase (GPx) and thioredoxin (Trx) as well as the non-enzymatic antioxidants including vitamins and secondary plant compounds.[Citation9,Citation10] The enzymatic antioxidants can directly or indirectly break down ROS to keep the redox balance.[Citation11] Non-enzymatic antioxidants can neutralize pro-oxidants either indirectly by induction of anti-oxidative enzymes or directly by interfering with the propagation of lipid radicals or by interact directly with free radicals.[Citation10] Pathophysiological ROS concentrations can either result from an over proportional production of ROS or from an insufficient supply of antioxidants.
Studies have shown that dietary components such as vitamin C, vitamin E and carotenoids are involved in the antioxidant defense process.[Citation2] Obviously, besides supplementation, antioxidants are contained in many foodstuffs, particularly in fruits and vegetables and can thus be sufficiently ingested while eating a healthy mixed diet.[Citation12]
Previous studies examined the impact of healthy mixed diets on the ROS formation and showed that the increased intake of fruits and vegetables leads to an augmented intake of antioxidant vitamins and a reduced protein and lipid modification by ROS.[Citation13,Citation14] A direct alteration in ROS plasma concentrations have not been determined in these investigations. A preliminary study by Nemzer et al.[Citation15] investigated the beneficial effects of a quercetin-containing dietary supplement on pro-oxidant damage measuring ROS blood concentrations directly by ESR. However, the longer-term effects of altering nutritional habits should have a greater significance on health-related outcomes.[Citation16]
The aim of the present pilot study was to investigate the influence of a mixed diet rich in vitamins and antioxidants on the concentration of ROS in blood. In this context, the hypothesis of this study was that a higher vitamin- and micronutrient intake can be associated with a decreased ROS production. To the best of our knowledge, the present investigation was the first clinical study directly examining altered ROS production as the consequence of overall nutritional adjustments.
Materials and methods
The study was designed as a monocentric, prospective, pilot trial (within-subject design) conducted at the University of Freiburg, Germany. In total, dietary patterns of 16 healthy subjects (18 to 50 years) were included. Dietary behavior was assessed using a three-day nutritional protocol and the results were then compared with the nutritional guidelines (D-A-CH recommendations) of the German Society of Nutrition.[Citation17] The sample size was determined by a power calculation based on the data of a previous trial examining the beneficial effect of an antioxidant supplement on ROS concentrations.[Citation15] Nutrient intake was quantified by evaluating the recorded foods using Nutriguide © (Nutri-Science GmbH, Hausach, Germany). Subjects were included if they did not reach the respective recommendations for micronutrients with antioxidant potential (). A total of six participants (28.3 ± 6.8 years) were chosen to change their diet regimen since their diet demonstrated non-conformity according to the D-A-CH recommendations[Citation18] (). Unstable weight and eating behavior were defined as the exclusion criteria. In addition, reported cardiovascular, metabolic or renal diseases led to an exclusion of the screened participants.
Table 1. D-A-CH Reference values for antioxidant nutrients[Citation17].
This study follows the principles of the Declaration of Helsinki. Following written informed consent, included participants were advised to implement a healthy mixed diet according to the D-A-CH recommendations[Citation18] for two weeks: (i) Enjoy food diversity, (ii) Vegetables and fruit – take ‘5 a day’, (iii) Favor whole-grain foods, (iii) Carefully choose animal-based foods, (iv) Avoid processed food, (v) Choose health-promoting fats, (vi) Reduce sugar and salt intake, (vii) Water is the best choice, (viii) Prepare carefully cooked dishes, (ix) Mindful eating and enjoying, and (x) Watch your weight and stay active.
If allergies or food intolerances existed, the respective foods were not consumed following consultation with the principal study investigator. Subjects were asked to complete a nutrition protocol during the intervention on a daily basis. With special reference to antioxidant vitamins E, C and β-carotene, all protocols were analyzed using Nutriguide© (Nutri-Science GmbH, Hausach, Germany).
For pretest and posttest, participants were instructed to arrive at the laboratory in the morning at the same time and were told to consume the same foods and liquids the evening prior to both examinations. Screening was started with a medical history questionnaire to ensure the inclusion criteria were met. In addition, they were asked to complete the Freiburg Questionnaire of physical activity.[Citation19]
Total ROS formation in capillary blood was measured at the screening time, after one week of the baseline diet (pretest) and after two weeks of the nutritional intervention following an overnight fast (posttest) (), since the ROS productions can be associated with postprandial glucose concentrations[Citation20] and can be consequently influenced by food intake. The ROS formation rate was measured by electron paramagnetic resonance (EPR) spectroscopy. For the examination of ROS formation under in vivo condition at 37°C, a Bench Top EPR spectrometer E-SCAN system from Bruker (Karlsruhe, Germany) was connected with a temperature controller BIO-III.[Citation21]
For measuring ROS concentration 7.5 µL of oxygen-sensitive label (NOX 15.1–5 mmol/L) were transferred into Safe-Lock Tubes (0.5 mL, Eppendorf, Wesseling-Berzdorf, Germany).[Citation22] Subsequently, 7.5 µL of capillary blood was collected from the participants’ index finger and mixed with the oxygen label. For detection and quantification of ROS in the blood samples, 15 μL of a Krebs-HEPES buffer diluted spin probe 1-hydroxy-3-methoxycarbonyl-2,2,5,5-tetramethylpyrrolidine (CMH, 400 µmol/l) was added.[Citation23] After transferring the mixture into a capillary tube (Hirschmann., Eberstadt, Germany) the ROS formation rate was measured with the following settings: Center field: g = 2.011, sweep width: 60 G, frequency = 9.76 GHz, power = 20.97 mW, gain:1*103, modulation amplitude: 1.06 G, sweep time: 5.24s, number of scans: 10. The increase in EPR amplitude equates to the production of ROS. To calculate the ROS concentration of the blood samples the detected amplitude of the standard solution was used. Prior to each measuring sequence a quality control was performed using a standard solution, which contains a defined ROS concentration of 10 μM.
Statistical analysis
SPSS statistics software (IBM SPSS Statistics for Windows, Version 24.0. Armonk, NY: IBM Corp.) was used for all statistical analyses. Since the variable data of all the groups showed normal distribution according to the Kolmogorov–Smirnoff (KS)-test, the significance of changes in nutrition and ROS formation during the intervention period within groups was analyzed with the paired sample t-test. Furthermore, a one-sample student’s T-test was performed to check whether the nutrients intake at baseline and during the intervention differed statistically significant from the D-A-CH reference values. All the tests in the descriptive analysis were performed as two-sided tests and the significance level was set at α = 0.05. Additionally, effect sizes were calculated from differences in means between groups at the end of the investigation divided by the pooled standard deviation (Cohen’s d). All data are presented as mean ± standard deviation (SD) in tables and mean ± standard error (SE) in figures.
Results
All participants completed the trial and were included in the statistical analysis. No adverse events were noted, and no pathological findings were observed in the routine anamnesis. Baseline data of participants are summarized in . At baseline, the one-sample student’s T-test revealed significantly lower daily intake of β-carotene (2.72 ± 1.57 mg) in comparison with D-A-CH reference values of men (6.0 mg; p = .004) and women (4.8 mg; p = .023). Furthermore, the participants daily intake of vitamin C (76.59 ± 26.70 mg vs. 110 mg; p = .028) and vitamin E (8.54 ± 3.37 mg vs. 15 mg; p = .006) was significantly lower than the recommendations for men.
Table 2. Baseline data for anamnestic parameters and outcomes.
The data of the nutritional protocols showed a significantly increased intake of β-carotene (9.46 ± 4.78 mg; p < .01); vitamin C (136.42 ± 87.42 mg; p < .05) and vitamin E (6.24 ± 1.24 mg; p < .05) during the intervention compared with baseline (). With respect to the D-A-CH recommendations for men and women, participants had a significantly higher intake in β-carotene (12.18 ± 3.5 mg; p < .01) and vitamin C (213.02 ± 83.68 mg; p < .05) during the intervention period. In contrast to baseline, the intake of vitamin E (14.78 ± 3.37 mg) was not significantly different from reference values of 12 and 15 mg per day. Consequently, participants reached at least the recommended amount of the respective micronutrients per day.
Figure 3. Changes of nutrient intake in mg/day (mean ± SE) from baseline to intervention period (* = p < .05; ** = p < .01).
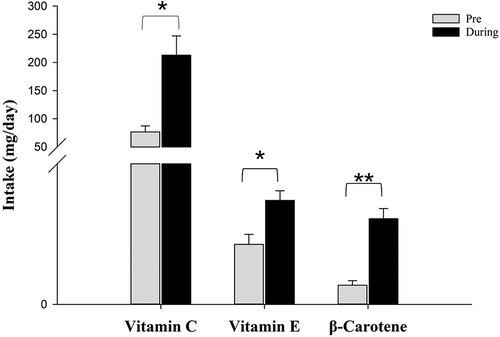
shows the comparison of daily dietary intake between the baseline and intervention period. The intake of energy, alcohol and sodium showed significant decreases. In contrast, the intake of dietary fibers significantly increased. The results of the paired sample t-test revealed a statistically significant decrease in total ROS concentration (−0.36 ± 0.27 µM/min, p = .022) at the end of the two-week intervention period in comparison with the pretest values (). With the baseline value as reference, the impact of a healthy mixed diet was also reflected by large effect sizes of d = 3.478 (β-carotene), d = 1.838 (vitamin E) and d = 2.197 (vitamin C) after a two week intake of a healthy mixed diet.
Table 3. Changes in daily dietary intake during the baseline and intervention period. Statistical differences were analyzed using paired t-test.
Discussion
The current investigation demonstrated that the adherence to a guideline-matched healthy mixed diet led to a significantly increased intake of the antioxidant micronutrients β-carotene, vitamin E and vitamin C and a concomitant reduction in ROS production. The current state of research suggests that some micronutrients as well as polyphenols have an antioxidant effect and thus a protective impact against increased ROS formation.[Citation2] This study revealed a statistically significant decrease in ROS formation after the intervention, which can at least partly be explained by an elevated supply of β-carotene, vitamin C and vitamin E. Nevertheless, the effect of other micronutrients including secondary plant products is likely to have a beneficial effect as well – however, this cannot by quantified by the current design.
Khan et al., [Citation24] Alvarez-Suarez et al.[Citation13] and Dragsted et al.[Citation25] investigated the effects on oxidative stress in their studies using indirect ROS measurement methods. Daily intake of blackcurrant juice, [Citation24] strawberries, [Citation13] or natural fruits and vegetables[Citation25] resulted in a significant increase in vitamin C concentration. According to the authors, these results are associated with an increased antioxidant status, which in turn has a positive effect on oxidative stress. In the investigations of Khan et al., [Citation24] only a minimal increase in vitamin E could be detected in the group that consumed blackcurrant juice with a high dose of antioxidants and polyphenols. Regarding the study by Dragsted et al., [Citation25] only the supplement group (vitamins and minerals) could achieve a significantly enhanced vitamin E intake. Dragsted et al.[Citation25] investigated the effects of increased fruit and vegetable consumption on the β-carotene concentration and also noted a significant increase.
This assumption is now confirmed by Alvarez-Suarez et al., [Citation13] who investigated the association between increased bioavailability of vitamin C and increased plasma antioxidant capacity. Vitamin C acts as a strong inhibitor of LDL oxidation.[Citation26] The association between increased vitamin C concentration and reduced lipid peroxidation was confirmed by a decrease in MDA and 8-isoprostane. Furthermore, the 8-OHdG lower DNA damage was detected by Alvarez-Suarez et al. .[Citation13] A similar result was reported by Khan et al., [Citation24] who investigated the oxidative stress marker F2-isoprostane in their study following vitamin C and polyphenols. They observed a significant correlation between an increased plasma vitamin C concentration and a reduced F2-isoprostane concentration and consequently a reduced lipid peroxidation. Díaz-Rubio et al.[Citation14] investigated to what extent the daily consumption of 200 ml (= 2000 μmol Trolox units) of an antioxidant-rich juice for 8 weeks affects the oxidative status and metabolites in healthy adults. Díaz-Rubio et al. concluded that the significantly increased antioxidant capacity, as well as the significantly reduced lipid peroxidation resulted from the increased intake of polyphenols and vitamin C.[Citation14] In addition, levels of endogenous antioxidants significantly increased from pre to post-intervention levels. These findings support the above mentioned hypothesis that the modified oxidative status is mainly caused by the enhanced intake of polyphenols and vitamin C. In contrast, Dragsted et al.[Citation25] did not show an improved antioxidant capacity and lipid peroxidation despite an increased intake of vitamin C and β-carotene. Furthermore, Dragsted et al.[Citation25] confirmed a negative effect on protein oxidation, since high vitamin C concentrations can exert a pro-oxidative effect on lysine.
A review by Traber & Aktinson[Citation27] confirmed the antioxidant effects of increased vitamin E concentrations. Consequently, it can be suggested that the effects of vitamins on antioxidant status are highly dependent on the study design. It should also be critically considered that a large number of previous studies have worked with indirect measuring methods. Thus, the change in the ROS generation can be interpreted only through modifications in lipids, DNA or proteins, whereas the present work applied direct ROS measurements using EPR.
According to the evaluation of the nutritional protocols the significantly increased vitamin C concentration resulted from an increased intake of vegetables such as red pepper, broccoli or potatoes and fruits such as kiwi, oranges or apples.[Citation18] The daily intake of 213.02 (± 83.68) mg vitamin C was higher than the recommended dietary allowance (RDA) of 95–110 mg per day considered necessary by the German Society of Nutrition.[Citation28] In contrast to the German Society of Nutrition, the Dietary Guidelines for American and Canadian recommend a daily intake of 75 mg for women (except pregnancy and lactation) and 95 mg for men over the age of 19.[Citation29] The significantly higher vitamin E intake of 14.78 (± 3.37) mg per day during the intervention period was in accordance with the recommended vitamin E reference values published by the German Society of Nutrition. Based on the nutritional protocols the increased consumption of certain oils, such as sunflower and olive oil, as well as nuts was responsible for the higher vitamin E intake.[Citation30] The Canadian and American Nutrition organizations agree with the German Society of Nutrition that a daily intake of 15 mg of vitamin E meeting the currently established RDA for most adults.[Citation29] During the intervention, the β-carotene intake of 12.18 (± 3.51) mg per day was twice as high as the nutritional requirements of 4.8–6 mg according to the German Society of Nutrition or 5.2–6 mg according to Canadian and American Nutrition organizations.[Citation29] Fruits and vegetables such as carrots, iceberg lettuce, spinach, broccoli, red peppers and tomatoes and fruits such as mango or apricots were the main sources of β-carotene during the intervention.[Citation31]
Compared with the imbalanced diet during the baseline period, the mixed healthy diet change showed variations in intake of other nutrients such as alcohol, sodium and fiber, in addition to vitamins. Previous studies have proved that alcohol can promote ROS production and oxidative stress, thereby enhancing peroxidation of lipids, protein and DNA.[Citation32] Recently, sodium has been demonstrated that can increase NADPH oxidase-derived oxidative stress independently by Brett.J.Wong .[Citation33] In contrast, dietary fiber could reduced levels of oxidative stress in few studies.[Citation34,Citation35] Therefore, the reduced intake of alcohol and sodium[Citation36] and increased intake of fiber may have boosted the antioxidant effect in this experiment.
From another perspective, using the self-reported dietary protocols to assess the intake of the antioxidant nutrients is flawed. It is assumed that the data of the vitamins taken from the nutritional records do not represent the actual plasma concentration since misreporting is a major concern to research on relations between diet and health.[Citation37] Furthermore, the bioavailability of the nutrients cannot be concluded from the nutritional protocols for the absorption underlies individual differences.[Citation38] In addition, it is possible that the extremely high vitamin C concentration leads to a pro-oxidative effect.[Citation25] One subject showed small changes in ROS concentration and a high vitamin C intake, at the same time. For this reason, to obtain more reasonable results, further studies should also examine the plasma concentrations of respective vitamins.
Neverthless, the study has several limitations. In this study, ROS concentrations with EPR were directly measured. However, a combination of direct and indirect measurement methods could have clarified to what extent the higher initial ROS concentrations were associated with oxidative damage and in how far this has also been improved by the dietary changes.[Citation39] Since a healthy diet is often associated with an improved health status, [Citation40–Citation42] the relationship between ROS concentration and metabolic risk factors such as cholesterol levels, HbA1c or blood pressure would be interesting. To describe and quantify this complex system, Halliwell and Gutteridge[Citation43] recommend using two or more ROS measuring methods to facilitate the interpretation of the results. Considering the charge of different spin traps only joint application of a series of probes could provide the identification of different sources of cellular radicals.[Citation44] However, in this trial only CMH was used to “lable” the radicals. Furthermore, there are no reference values for the ROS concentrations directly determined by ESR. Although our results show significant difference in ROS generation and intake of micronutrients, including six participants might not have been enough to detect other potential influence. Meanwhile, all participants were relatively young people so our result may not necessarily be valid for elderly or patients suffering from chronic conditions.
Conclusion
This study confirmed that following a healthy mixed diet, ROS concentration was significantly reduced, presumably as a consequence of an increased intake of nutrients with antioxidant capacity. Although some studies have also shown beneficial effects of dietary supplements on pro-oxidative damage, the long-term effects of altering nutritional habits should have a greater significance on health-related outcomes. Measuring ROS concentrations in blood by EPR spectroscopy, rather than by oxidative stress markers, could be a useful tool to identify positive, health-related effects of different dietary regimens. This, however, needs to be verified in larger trials involving more subjects and lasting for a longer period.
Acknowledgments
We would like to thank all of the participants. ZZ, CB, DZ and CC as well as DK designed the study. ZZ and CB were responsible for data acquisition. All authors read and approved the final version of the manuscript. The authors declare no conflict of interest.
References
- Valko, M.; Leibfritz, D.; Moncol, J.; Cronin, M. T. D.; Mazur, M.; Telser, J. Free Radicals and Antioxidants in Normal Physiological Functions and Human Disease. Int. J. Biochem. Cell Biol. 2007, 39, 44–84. DOI: 10.1016/j.biocel.2006.07.001.
- Wojcik, M.; Burzynska-Pedziwiatr, I.; Wozniak, L. A Review of Natural and Synthetic Antioxidants Important for Health and Longevity. Curr. Med. Chem. 2010, 17(28), 3262–3288. DOI: 10.2174/092986710792231950.
- Barbieri, E.; Sestili, P. Reactive Oxygen Species in Skeletal Muscle Signaling. J. Signal Transduction. 2012, 2012, 1–17. Doi:10.1155/2012/982794.
- Chen, Y.; Zhou, Z.; Min, W. Mitochondria, Oxidative Stress and Innate Immunity. Front. Physiol. 2018, 9, 1487. DOI: 10.3389/fphys.2018.01487.
- Schröder, K.;. NADPH Oxidases in Redox Regulation of Cell Adhesion and Migration. Antioxid. Redox Signaling. 2014, 20, 2043–2058. DOI: 10.1089/ars.2013.5633.
- Singh, A.; Kukreti, R.; Saso, L.; Kukreti, S. Oxidative Stress: A Key Modulator in Neurodegenerative Diseases. Molecules. 2019, 24(8), 1583. DOI: 10.3390/molecules24081583.
- Lepetsos, P.; Papavassiliou, A. G. ROS/oxidative Stress Signaling in Osteoarthritis. Biochim. Biophys. Acta Mol. Basis Dis.. 2016, 1862, 576–591. Doi:10.1016/j.bbadis.2016.01.003.
- Phull, A. R.; Nasir, B.; Haq I, U.; Kim, S. J. Oxidative Stress, Consequences and ROS Mediated Cellular Signaling in Rheumatoid Arthritis. Chem.-Biol. Interact. 2018, 281, 121–136. DOI: 10.1016/j.cbi.2017.12.024.
- Waring, W. S.; Convery, A.; Mishra, V.; Shenkin, A.; Webb, D. J.; Maxwell, S. R. J. Uric Acid Reduces Exercise-induced Oxidative Stress in Healthy Adults. Clin. Sci. 2003, 105(4), 425–430. DOI: 10.1042/CS20030149.
- He, L.; He, T.; Farrar, S.; Ji, L.; Liu, T.; Ma, X. Antioxidants Maintain Cellular Redox Homeostasis by Elimination of Reactive Oxygen Species. Cell. Physiol. Biochem. 2017, 44(2), 532–553. DOI: 10.1159/000485089.
- Christofidou-Solomidou, M.; Muzykantov, V. R. Antioxidant Strategies in Respiratory Medicine. Treat. Respir. Med.. 2006, 5(1), 47–78.
- Kaliora, A. C.; Dedoussis, G. V. Z.; Schmidt, H. Dietary Antioxidants in Preventing Atherogenesis. Atherosclerosis. 2006, 187(1), 1–17. DOI: 10.1016/j.atherosclerosis.2005.11.001.
- Alvarez-Suarez, J. M.; Giampieri, F.; Tulipani, S.; Casoli, T.; Di Stefano, G.; González-Paramás, A. M.; Santos-Buelga, C.; Busco, F.; Quiles, J. L.; Cordero, M. D.; et al. One-month Strawberry-rich Anthocyanin Supplementation Ameliorates Cardiovascular Risk, Oxidative Stress Markers and Platelet Activation in Humans. J. Nutr. Biochem. 2014, 25(3), 289–294.
- Díaz-Rubio, M. E.; Pérez-Jiménez, J.; MÁ, M.-B.; Álvarez, I.; Saura-Calixto, F. Regular Consumption of an Antioxidant-rich Juice Improves Oxidative Status and Causes Metabolome Changes in Healthy Adults. Plant Foods Hum. Nutr. 2015, 70(1), 9–14. DOI: 10.1007/s11130-014-0455-4.
- Nemzer, B. V.; Fink, N.; Fink, B. New Insights on Effects of a Dietary Supplement on Oxidative and Nitrosative Stress in Humans. Food Sci. Nutr. 2014, 2(6), 828–839. DOI: 10.1002/fsn3.178.
- Hahn, A.; Ströhle, A.; Wolters, M. Ernährung. Physiologische Grundlagen, Prävention, Therapie; Wissenschaftliche Verlagsgesellschaft: Stuttgart, 2016.
- Referenzwerte für die Nährstoffyufuhr [Reference values for nutrient intake] [Internet]. Germany: Deutsche Gesellschaft für Ernährung e.V. [German Nutrition Society]; 2019. http://www.dge.de/wissenschaft/referenzwerte/ (accessed April 20, 2019)
- 10 Regeln der DGE [10 rules of the DGE] [Internet]. Germany: Deutsche Gesellschaft für Ernährung e.V. [German Nutrition Society]; 2018. https://www.dge.de/index.php?id=52 (accessed April 15, 2019).
- Frey, I.; Berg, A.; Grathwohl, D.; Keul, J. [Freiburg Questionnaire of Physical Activity–development, Evaluation and Application]. Soz Praventivmed. 1999, 44(2), 55–64. DOI: 10.1007/BF01667127.
- O’Keefe, J. H.; Gheewala, N. M.; O’Keefe, J. O. Dietary Strategies for Improving Post-Prandial Glucose, Lipids, Inflammation, and Cardiovascular Health. J. Am. Coll. Cardiol. 2008, 51, 249–255. Doi:10.1016/j.jacc.2007.10.016.
- Dikalov, S. I.; Li, W.; Mehranpour, P.; Wang, S. S.; Zafari, A. M. Production of Extracellular Superoxide by Human Lymphoblast Cell Lines: Comparison of Electron Spin Resonance Techniques and Cytochrome C Reduction Assay. Biochem Pharmacol. 2007, 73(7), 972–980. DOI: 10.1016/j.bcp.2006.12.012.
- Mariappan, N.; Elks, C. M.; Fink, B.; Francis, J. TNF-induced Mitochondrial Damage: A Link between Mitochondrial Complex I Activity and Left Ventricular Dysfunction. Free Radic. Biol. Med. 2009, 46(4), 462–470. DOI: 10.1016/j.freeradbiomed.2008.10.049.
- Mrakic-Sposta, S.; Gussoni, M.; Montorsi, M.; Porcelli, S.; Vezzoli, A. Assessment of a Standardized ROS Production Profile in Humans by Electron Paramagnetic Resonance. Oxid. Med. Cell. Longev. 2012, 2012, 1–10. DOI: 10.1155/2012/973927.
- Khan, F.; Ray, S.; Craigie, A. M.; Kennedy, G.; Hill, A.; Barton, K. L.; Broughton, J.; Belch, J. J. F. Lowering of Oxidative Stress Improves Endothelial Function in Healthy Subjects with Habitually Low Intake of Fruit and Vegetables: A Randomized Controlled Trial of Antioxidant- and Polyphenol-rich Blackcurrant Juice. Free Radical Biol. Med. 2014, 72, 232–237. DOI: 10.1016/j.freeradbiomed.2014.04.006.
- Dragsted, L. O.; Pedersen, A.; Hermetter, A.; Basu, S.; Hansen, M.; Haren, G. R.; Kall, M.; Breinholt, V.; Castenmiller, J. J.; Stagsted, J.; et al. The 6-a-day Study: Effects of Fruit and Vegetables on Markers of Oxidative Stress and Antioxidative Defense in Healthy Nonsmokers. Am. J. Clin. Nutr. 2004, 79(6), 1060–1072.
- Zhang, P. Y.; Xu, X.; Li, X. C. Cardiovascular Diseases: Oxidative Damage and Antioxidant Protection. Eur. Rev. Med. Pharmacol. Sci. 2014, 18(20), 3091–3096.
- Traber, M. G.; Atkinson, J. Vitamin E, Antioxidant and Nothing More. Free Radical Biol. Med. 2007, 43, 4–15. DOI: 10.1016/j.freeradbiomed.2007.03.024.
- Bechthold, A.;. New Reference Values for Vitamin C Intake. Ann. Nutr. Metab. 2015, 67, 13–20. DOI: 10.1159/000434757.
- Institute of Medicine (US) Panel on Dietary Antioxidants and Related Compounds. Dietary Reference Intakes for Vitamin C, Vitamin E, Selenium, and Carotenoids; National Academies Press (US): Washington (DC), 2000.
- Reboul, E.;. Vitamin E Bioavailability: Mechanisms of Intestinal Absorption in the Spotlight. Antioxidants. 2017, 6(4), 95. DOI: 10.3390/antiox6040095.
- Grune, T.; Lietz, G.; Palou, A.; Ross, A. C.; Stahl, W.; Tang, G.; Thurnham, D.; Yin, S.-A.; Biesalski, H. K. β-Carotene Is an Important Vitamin A Source for Humans. J. Nutr. 2010, 140(12), 2268–2285.
- Wu, D.; Cederbaum, A. I. Alcohol, Oxidative Stress, and Free Radical Damage. Alcohol Res. Health. 2003, 27(4), 277–284.
- Wong, B. J.;. Dietary Sodium and Oxidative Stress Impair Cutaneous Microvascular Function Independent of Blood Pressure. Am. J. Physiol. 2019, 317(1), 60–62. DOI: 10.1152/ajpheart.00309.2019.
- Diniz, Y. S.; Cicogna, A. C.; Padovani, C. R.; Silva, M. D. P.; Faine, L. A.; Galhardi, C. M.; Rodrigues, H. G.; Novelli, E. L. B. Dietary Restriction and Fibre Supplementation: Oxidative Stress and Metabolic Shifting for Cardiac Health. Can. J. Physiol. Pharmacol. 2003, 81(11), 1042–1048.
- Sánchez, D.; Quiñones, M.; Moulay, L.; Muguerza, B.; Miguel, M.; Aleixandre, A. Soluble Fiber-enriched Diets Improve Inflammation and Oxidative Stress Biomarkers in Zucker Fatty Rats. Pharmacol. Res. 2011, 64(1), 31–35. DOI: 10.1016/j.phrs.2011.02.005.
- Kitiyakara, C.; Chabrashvili, T.; Chen, Y.; Blau, J.; Karber, A.; Aslam, S. Salt Intake, Oxidative Stress, and Renal Expression of NADPH Oxidase and Superoxide Dismutase. J. Am. Soc. Nephrol. 2003, 14(11), 2775–2782.
- Mendez, M. A.; Popkin, B. M.; Buckland, G.; Schroder, H.; Amiano, P.; Barricarte, A.; Huerta, J.-M.; Quirós, J. R.; Sánchez, M.-J.; González, C. A.; et al. Alternative Methods of Accounting for Underreporting and Overreporting When Measuring Dietary Intake-obesity Relations. Am. J. Epidemiol. 2011, 173(4), 448–458.
- Galan, P.; Viteri, F. E.; Bertrais, S.; Czernichow, S.; Faure, H.; Arnaud, J.; Ruffieux, D.; Chenal, S.; Arnault, N.; Favier, A.; et al. Serum Concentrations of β-carotenE, Vitamins C and E, Zinc and Selenium are Influenced by Sex, AgE, Diet, Smoking Status, Alcohol Consumption and Corpulence in a General French Adult Population. Eur. J. Clin. Nutr. 2005, 59(10), 1181–1190.
- Poljšak, B.; Jamnik, P. Methodology for Oxidative State Detection in Biological Systems. In Handbook of Free Radicals: Formation, Types and Effects; Kozyrev, D., Sutsky, V., Eds.; Nova Science Publishers, Inc.: New York (NY), 2010; pp 421–448.
- Swain, J. F.; McCarron, P. B.; Hamilton, E. F.; Sacks, F. M.; Appel, L. J. Characteristics of the Diet Patterns Tested in the Optimal Macronutrient Intake Trial to Prevent Heart Disease (Omniheart): Options for a Heart-Healthy Diet. J. Am. Diet. Assoc. 2008, 108(2), 257–265. DOI: 10.1016/j.jada.2007.10.040.
- Hu, F. B.;. Diet and Lifestyle Influences on Risk of Coronary Heart Disease. Curr. Atherosclerosis Rep. 2009, 11(4), 257–263. DOI: 10.1007/s11883-009-0040-8.
- Giugliano, D.; Ceriello, A.; Esposito, K. The Effects of Diet on Inflammation. Emphasis on the Metabolic Syndrome. J. Am. Coll. Cardiol. 2006, 48(4), 677–685. DOI: 10.1016/j.jacc.2006.03.052.
- Halliwell, B.; Gutteridge, J. M. C. Free Radicals in Biology and Medicine; Oxford University Press: New York (NY), 2015.
- Dikalov, S. I.; Kirilyuk, I. A.; Voinov, M.; Grigor’Ev, I. A. EPR Detection of Cellular and Mitochondrial Superoxide Using Cyclic Hydroxylamines. Free Radic. Res. 2011, 45(4), 417–430. DOI: 10.3109/10715762.2010.540242.