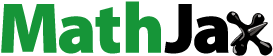
ABSTRACT
Arrowroot starch repeatedly ozonated for 10 and 30 cycles was mixed with 5 and 10 g palm oil/100 g starch to form a complex. The complex index increased as oil concentration or ozonation cycles increased. The increased complex due to ozonation could be linked to depolymerization or ring opening but not to the increase in the number of polar carboxyl groups, so more entry points to the amylose cavity became available for lipid inclusion. The properties of the ozonated starch mixed with palm oil were affected by the interplay between the effect of lipid–starch complex and carboxyl content. The effect of the lipid–starch complex prevailed over that of the carboxyl group at a palm oil concentration of 10 g palm oil/100 g starch.
Introduction
Starch plays an important role in food, textile, pharmaceutical and paper industries.[Citation1] Despite extensive studies on various starch sources, arrowroot as an alternative starch source has been rarely examined. Therefore, the properties of arrowroot starch should be investigated to enrich the existing information on various starch sources.
Native starch has several disadvantages when it is applied directly to food, so starch modification is required.[Citation2] Amongst starch modification methods, thermal and physical modifications have been commonly applied because they are regarded as safe ways to modify starch.[Citation3,Citation4] Chemical modification involving gaseous ozone may also be deemed as a safe method because of the lack of chemical residues and environmental wastes.[Citation5,Citation6] Most studies on starch ozonation have been carried out by using a suspension, such as those for cassava starch,[Citation7] potato starch[Citation8] and wheat starch.[Citation9] Starch modification with gaseous ozone is simpler than starch modification with a suspension because starch is modified under dry conditions. Therefore, no redrying processes after ozonation are required. Furthermore, gaseous ozone has been used to successfully modify the properties of banana starch and flour;[Citation10] corn, sago and tapioca starches;[Citation5] and pigeon pea, lima bean and jack bean starches.[Citation11] However, studying arrowroot starch modified with gaseous ozone is challenging and interesting.
The properties of arrowroot starch have been successfully modified using gaseous ozone in our laboratory.[Citation12] However, the properties of the ozonated arrowroot starch in the presence of palm oil remain unknown. In many food preparations, the addition of oil to starch is commonly applied as a part of ingredients. Numerous studies have revealed a complex formation between starch and lipid, and this formation affects pasting and functional properties,[Citation13,Citation14] granular morphology,[Citation15,Citation16] thermal properties, X-ray diffraction (XRD) characteristics and in vitro digestibility.[Citation15,Citation17]
Most studies on lipid–starch complexes have focused on native starch, but studies on modified starch have been rarely performed. Starch modification via ozonation increases the carboxyl content and reduces starch crystallinity.[Citation10,Citation18] Changes in the polarity and crystallinity of ozonated starch may alter the way starch interacts with lipids. As such, studying the complex formation between lipids and ozonated starch is interesting. Furthermore, palm oil is often used in the food industry because of its affordable and economical price. Therefore, palm oil as a lipid source is a realistic and applicable choice. Palm oil mainly contains lauric acid (±48%), myristic acid (±16%) and oleic acid (±15%); it also has palmitic acid (±8%) and linoleic acid (±2%).[Citation19]
This study aimed to reveal the interaction between ozonated starch and palm oil and the effect of this interaction on starch properties, such as color, functional and pasting properties. This study also examined the effect of palm oil concentration on these properties.
Materials and methods
Materials
The raw materials used in this study were native arrowroot starches (NAS), arrowroot starches ozonated for 10 (OAS10) and 30 (OAS30) cycles and palm oil. All chemicals were used directly without further purification. Arrowroot tubers (‘Creole’ cultivar) were harvested at the age of 10–11 months and obtained from farmers in Sragen, Central Java, Indonesia.
Extraction of arrowroot starch
Starches were extracted from the tubers in accordance with previously described methods.[Citation12] Unpeeled arrowroot tubers were washed, soaked and homogenated to form pulp (arrowroot tuber:water = 1:3.5). The pulp was then filtered twice by adding more water at the same ratio as the pulp. The filtrate was decanted for 24 hours, and water was replaced with fresh water every 6 hours. Starch was then dried in an oven at 50°C overnight, ground and sifted with a 100-mesh sieve. The starch content in the extract following oven drying was approximately 81.5%.
Starch ozonation
Native arrowroot starch (125 g) was placed in a 1 l cylindrical stainless container. Afterward, 13 ppm ozone was allowed to flow into the container for 5 min with a flow rate of 10 l/min. Starch was homogeneously exposed to ozone gas by rotating the container for 11 min. Rotation was stopped, and oxygen was flushed into the container for 1 min to remove any remaining gas in the container. Fresh ozone (13 ppm) was reflowed into the container for another 5 min, and the container was rerotated for another 11 min. The process was repeated for 10–30 cycles. Our preliminary study indicated that the ozone concentration dropped to 1% of the initial concentration within 11 min when the starch was ozonated. As such, ozonation should be terminated, and fresh ozone was used after flushing was conducted.
Analysis of pasting properties and formation of starch–lipid complexes
Pasting properties were measured using a rapid Visco analyzer (RVA-SM2; Warriewood Australia) in accordance with previously described methods.[Citation20] Palm oil was weighed accurately (5 and 10 g/100 g starch) and placed in a canister. Then, 25 ml of distilled water and 3.5 g of starch (NAS, OAS10, OAS30) were added. The mixture was agitated by raising and lowering the plastic paddle through the canister 10 times before the canister was inserted into the instrument. Viscosity was recorded with the following temperature profile: holding at 50°C for 1 min, heating from 50°C to 95°C for 3.7 min, holding at 95°C for 2.5 min and cooling down to 50°C for 3.8 min. The resulting gel was maintained at 50°C for 2 min with rapid stirring at 960 rpm for the first 10 s to disperse the starch sample and then with a constant paddle rotating speed of 160 rpm throughout the entire analysis. The total run time for each sample was 13 min. The following parameters were recorded: peak viscosity (PV), hold viscosity (HV), final viscosity (FV), breakdown, setback and pasting temperature. All the measurements were reported as the pasting properties. The final resulting pasta (starch–lipid complex) was dried at 40°C overnight and ground to fine powder.[Citation21]
Complex index of gelatinized starch–palm oil mixtures
The complex index (CI) of starch was determined using the method of Gilbert and Spragg[Citation22] with slight modifications. Starch paste (5.0 g) was removed from the RVA canister immediately after the completion of the profile and mixed with 25 ml of distilled water at 50°C in a 50 ml capped tube. The tube was homogenized by vortexing for 2 min, and 100 μl of the resulting dispersion was mixed with 15 ml of distilled water. Then, 2 ml of iodine solution (2.0% KI and 1.3% of I2 in distilled water) was added. The absorbance at 690 nm was measured. Pastes that contained only starch were used as a reference. Tests were performed within 60 min to avoid starch retrogradation, and CI was calculated using the following equation:
Swelling volume and solubility
Swelling volume and solubility were measured by suspending 0.35 g (db) of arrowroot starch in 12.5 ml of water in a centrifuge tube. The suspension was mixed by vortexing for 30 s and then placed in a water bath at 92.5°C for 30 min. The samples were cooled in iced water for 1 min and centrifuged at 3500 rpm for 30 min. The volume of the supernatant was measured, and solubility was determined from the supernatant previously dried in a hot air oven. The swelling volume and solubility were expressed in Equations (1) and (2)[Citation23]:
Freeze–thaw stability
Freeze–thaw stability was determined in accordance with a previously reported method.[Citation3] In brief, 5% of starch (w/v dry basis) was suspended in water, heated at 95°C for 30 min under constant mild agitation and cooled to room temperature in an iced shaking water bath. Then, 20 g of paste was taken, placed in a centrifuge tube and subjected to a freeze–thaw cycle by storing at 4°C for 24 hours, freezing at −15°C for 48 hours, thawing at 25°C for 3 hours and centrifuging at 3500 rpm for 15 min. The supernatant removed from the gel was weighed. The extent of syneresis was expressed as the percentage of separated liquid per total weight of the sample in the centrifuge tube.
Gel strength
Gel characterization was carried out using the paste of the lipid–starch complex formed from the RVA. The resulting paste was poured into a pipe container (2.5 cm in diameter, 3 cm in height) at room temperature for 1 hour to form a gel. The container was covered with aluminum foil and stored in a refrigerator (4°C) for 24 hours. A texture analyzer (TA-XT2) was used to measure gel strength.[Citation4]
Lightness analysis
The color of the starches was analyzed using a spectrophotometer (CM-5 model; Konica Minolta Co., Osaka, Japan). The color parameters L*, a* and b* are indices reflecting lightness, redness/greenness and yellowness/blueness, respectively. In this experiment, the spectrophotometer was calibrated against a standard white tile (L* = 97.63, a* = 0.78 and b* = 0.25), where Δa, ΔL and Δb represent the deviations of the individual values from the respective values of native arrowroot starch.
∆E (difference in coloration), C (chroma) and ∆H* (hue angle or variation in color) were assessed using EquationEquations (3)(3)
(3) –(Equation6
(6)
(6) ):
X-ray diffraction (XRD)
Starches were analyzed through powder XRD by using an X-ray diffractometer (PANalytical X’Pert PRO seri PW3040/x0) with a Cu Ka value of 1.54 radiation with a range of 3°–50° for 2 hours at a voltage of 40 kV and a filament current of 30 mA. Relative crystallinity was calculated by using the Sigma plot programme in accordance with previously reported methods.[Citation10]
Statistical analysis
Data were collected in triplicate and analyzed with SPSS by using ANOVA followed by post hoc Duncan’s test.
Results and discussion
Complex index (CI)
The extent of lipid–starch complex formation was expressed as CI (). Two palm oil concentrations (5 and 10 g palm oil/100 g starch) were applied to reveal the effect of different oil concentrations on the interaction with ozonated arrowroot starch at different cycles (10 and 30 cycles). Native starch was used as the control to further understand the effect of ozonation.
Figure 1. Complex index of starch ozonated for different cycles mixed with different palm oil concentration (5 and 10 g/100 g starch). Different letters in the compared values denote significant difference at p ≤ .05.
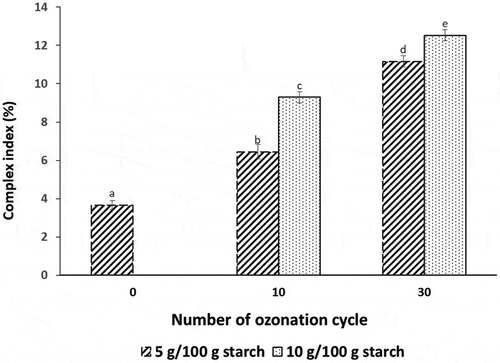
The result showed that the CI increased as the palm oil concentration increased. In a starch–lipid complex inclusion model, the aliphatic part of lipids is located inside the helical cavity of amylose, whilst the polar group of fatty acids remains outside the entrance of the helical cavity because of steric and electrostatic repulsions.[Citation24] Amylose can accommodate two fatty acids whose polar part is located at the end of each helix. Long amylose chains may even interact with more than two fatty acids, but these chains are distorted to accept polar groups of fatty acids. In the present study, the CI increased as the lipid content increased because more lipids could form a complex with starch.
Palm oil forms a complex with starch.[Citation17] Our finding was in agreement with previous studies.[Citation14,Citation25] An optimum concentration of lipid is needed to form a maximal complex with starch, but the CI tends to remain unchanged or decrease above this concentration because of the preference of lipids to self-associate.[Citation20] In the present study, the lipid concentrations were obviously in the range of acceptable concentrations, given that the CI increased as the lipid concentration increased.
In terms of ozonation, starch pretreatment with ozonation increased the degree of complex formation as indicated by the increase in the CI of native starch from 3.66% to about 6.44% and 11.17% when starch was pretreated with ozonation for 10 and 30 cycles, respectively. A similar trend of the effect of ozonation was also observed when 10 g palm oil/100 g starch was used. Ozonation increases carboxyl contents and reduces starch crystallinity.[Citation12] Ozonation also results in amylose and amylopectin depolymerization.[Citation6]
The change in the functional group from a hydroxyl group to a carboxyl group following ozonation may lead to an increase in starch polarity. In this study, this change was interesting because the degree of complex formation increased as the carboxyl content increased; that is, polarity increased. In another study, the number of starch–lipid complexes increased as lipid hydrophobicity increased; this result suggests that hydrophobic interaction plays a pivotal role[Citation20] in complex formation. Therefore, the increase in CI as starch polarity increased was unexpected.
After ozonation, the polarity of a single amylose helix changed because of carboxyl formation. As such, inserting the aliphatic chains of lipids into a helical cavity of amylose became less favorable, and the CI would decrease. Regardless of theory, the opposite observation in the present study could be explained by several possible reasons. For instance, interactions between starch and lipids could be different from that shown in the inclusion model. Godet et al.[Citation24] hypothesized that aliphatic chains are located outside amylose helices in a crystal unit cell during starch–lipid interactions. Another possibility is that carboxyl formation is accompanied with the ring opening of a glucose chain.[Citation6] This opening may create another entrance to the amylose cavity so that amylose can accommodate more lipids. Furthermore, amylose and amylopectin depolymerization following ozonation simply results in the formation of more starch polymers for lipid inclusion as more entry points to the cavity become available.
Reduction in crystallinity may not play any role in the increase in CIs. A highly amorphous region is likely favorable to complex formation between lipids and starches because this region may provide a more accessible area for lipids to come in contact with starch polymers.[Citation26] However, this hypothesis was unlikely the underlying mechanism because complex formation in this study was triggered by heating the mixture of palm oil and starch in the RVA canister until 95°C so that starch mostly experienced gelatinization (amorphous state). Therefore, the extent of amorphous region during gelatinization might not differ.
Functional and color properties
The effect of starch pretreatment via ozonation prior to palm oil addition on functional and color properties is presented in . The role of palm oil in these properties was also revealed by comparing different amounts of lipids added to starch. Palm oil addition and pretreatment via ozonation affected SV, solubility, gel strength and freeze–thaw stability. Color properties, such as lightness, ΔE and ΔH, were also linked to palm oil addition and ozonation pretreatment.
Table 1. Functional and color properties of ozonated arrowroot starch in the presence of palm oil.
Effect of palm oil
The swelling volume of arrowroot starch decreased as the palm oil concentration increased. The swelling volume of starch ozonated for 10 cycles (OAS10-PO5) was 7.27%, which decreased to approximately 5.74% when 10 g palm oil/100 g starch was added. The swelling volume of the starch ozonated for 30 cycles (OAS30-PO5) also decreased from 9.87% to 5.04% when the oil concentration increased to 10 g palm oil/100 g starch.
The presence of palm oil forming a complex with amylose likely decreased the ability of starch to swell. This decrease might be related to the reduced capacity of starch to absorb water because of the presence of oil, thereby limiting the swelling volume. A similar finding was observed when stearic acid was added to corn starch.[Citation27] Lipid may also exist as an insoluble layer preventing water from diffusing within the starch region.[Citation28]
Solubility and gel strength decreased as palm oil concentration increased. The solubility of OAS10-PO5 decreased from 7.73% to approximately 6.96% when a high oil concentration was added. A similar trend was identified in OAS30-PO5. Our finding was in line with some other studies involving myristic fatty acid, palmitic fatty acid and stearic fatty acid added to different starches.[Citation25,Citation29,Citation30]
A decrease in solubility suggested that the presence of oil reduced amylose leaching possibly because of the interaction between oil and amylose forming a complex with a high molecular weight; as a result, the release of amylose from starch granules was retarded. The reduced amylose leaching could also be attributed to the formation of an insoluble oil layer surrounding starch.[Citation28]
In terms of gel strength reduction, the presence of oil undermined the starch polymer network in the gel system. A similar finding was reported in another study involving stearic acid.[Citation13] The presence of oil forming a complex with starch is apparently not favorable to gel strengthening. Gel formation is dependent on long-term starch retrogradation because of the formation of double-helix amylopectin and short-term retrogradation due to amylose.[Citation31] Complex formation between amylose and palm oil may prevent the formation of double helices, junction zones and gel network during storage. The complex prevents or retards the interaction between starch molecules during recrystallization, leading to the formation of a weak gel.[Citation13,Citation32,Citation33]
Freeze–thaw stability measured by the extent of syneresis indicated that an increase in oil addition increased syneresis, particularly in starch ozonated for 30 cycles. This finding suggested that the presence of oil decreased the stability of starch against retrogradation. The mixture with palm oil also affected the color properties of arrowroot starch. Color is an important factor of food commodities because it plays a role in increasing attractiveness; that is, it serves as an identification of food and quality attribute inherent in food commodities. Complex formation due to the addition of 5 g of palm oil to 100 g of native starch decreased lightness from 97.2 to 78.73, whilst ∆E and ∆H were 18.05 and 0.17, respectively. ∆E was so high that the color of NAS-PO5 was obviously distinguishable from that of native starch in the absence of palm oil. The effect of increasing palm oil concentration on ∆E and ∆H in ozonated starch either for 10 or 30 cycles was relatively insignificant, suggesting that the maximum effect of palm oil on color properties was observed in 5 g/100 g starch.
Effect of ozonation pretreatment
The swelling volume of arrowroot starch subjected to ozonation pretreatment increased when 5 g palm oil/100 g starch was added. In another study, the swelling volumes of banana starch and flour ozonated at 100 and 200 ppm also increase.[Citation10] Similar findings have been observed in corn starch and wheat starch.[Citation5,Citation34] The increased swelling volume of starch after ozonation might be attributed to the increase in the number of carboxylic functional groups because of ozonation, thereby increasing the ability of starch to absorb more water. However, ozonation was ineffective in increasing the swelling volume when starch was mixed with 10 g palm oil/100 g starch. This ineffectiveness could be explained by the reducing effect of palm oil on the swelling volume as explained previously. Therefore, at high palm oil concentrations, the effect of swelling volume reduction due to oil addition seemed to counteract the influence of swelling volume increased by ozonation.
Ozonation also increased solubility, gel strength and freeze–thaw stability. Our finding was in agreement with that in ozonated banana starch.[Citation10] However, this increase was important in the presence of 5 g palm oil/100 g starch. The increase in the number of ozonation cycles did not affect solubility, gel strength and freeze–thaw stability at 10 g palm oil/100 g starch. The reducing effect of palm oil seemingly outpowered the increasing effect of ozonation. The decreasing effect of 10 g palm oil/100 g starch was so large that the syneresis of starch ozonated for 30 cycles increased to 14.25%. The role of palm oil in reducing the effect of ozonation might be further explored by comparing the effect of ozonation in the absence of oil with that in the presence of oil. A previous study reported that the ozonation of banana starch can reduce syneresis from 26.9% to 0.004%.[Citation10] In the present study, the reduction reached only as much as 10.99% in the presence of 5 g palm oil/100 g starch, and this value was not as remarkable as that in a previous study.
Ozonation and palm oil addition led to an increase in lipid–starch complex formation. However, the effect of ozonation on functional properties was opposite of the effect of palm oil addition. For instance, the freeze–thaw stability was increased by ozonation at 5 g palm oil/100 g starch but was decreased by oil addition despite the same trend in increasing the complex formation. This difference might be attributed to the way through which starch–lipid complexes were formed because oil addition was different from ozonation, which increased amylose polarity; hence, a different effect on functional properties was observed. However, this hypothesis remains unclear and requires further proof. Furthermore, the increase in carboxyl after ozonation is more likely the underlying mechanism. Ozonation increases not only carboxyl contents but also freeze–thaw stability, gel strength, solubility and swelling volume of starch in the absence of lipids.[Citation10] In the present study, the increase in functional properties after ozonation was predominantly due to the change in carboxyl content, not the lipid–starch complex formation, particularly up to 5 g palm oil/100 g starch addition. Indeed, the lipid complex tends to nullify the effect of carboxyl content as observed in ozonated starch in the presence of a high lipid concentration (10%).
The effect of ozonation on color properties could be determined by comparing the samples of NAS-PO5, OAS10-PO5 and OAS30-PO5. Lightness increased as the number of cycles of ozonation increase possibly because of the role of ozone, which oxidizes the pigment component in arrowroot starch; as a result, the sample was lighter. The difference in color as observed in ∆E decreased when the sample was oxidized by ozone, suggesting that its color was closer to that of native starch. Ozonation was ineffective in the presence of high lipid concentrations, i.e. 10 g palm oil/100 g starch. Similar to the effect of lipids on functional properties, the reducing effect of lipids seemingly nullified the increasing effect of ozonation.
Role of lipid and ozonation in starch recrystallization
In this experiment, the mixture of arrowroot starch and palm oil was gelatinised using the RVA. As such, oil formed a complex with starch in the gelatinised form, that is, the amorphous phase. During the storage of gelatinised starch prior to XRD, recrystallisation occurred. shows the recrystallisation of arrowroot starch in the presence of palm oil and following ozonation pretreatment. Recrystallisation increased as the palm oil concentration increased as observed in OAS10-PO5; in particular, it increased from 6.38% to 7.09% in OAS10-PO10. A similar trend was observed in starch ozonated for 30 cycles. This consistent finding indicated that lipids increased starch recrystallisation probably because of the lipid–starch complex. Lipids form a Vh-type crystal,[Citation35] which might contribute to the increase in recrystallisation in the present study. In comparison with the result on freeze–thaw stability, the increase in recrystallisation caused more water to be expelled from the gel system. Consequently, syneresis increased. Therefore, the increase in recrystallisation was consistent with the decrease in the stability of starch against retrogradation.
Figure 2. XRD diffractograms of NAS-PO5; OAS10-PO5; OAS10-PO10; OAS30-PO5; OAS30-PO10 and degree of crystallinity. NAS-PO5 = Native arrowroot starch mixed with palm oil 5 g/100 g starch; OAS10-PO5 and OAS10-PO10 = Arrowroot starch ozonated 10 cycles mixed with respectively palm oil 5 g and 10 g/100 g starch; OAS30-PO5, OAS30-PO10 = Arrowroot starch ozonated 30 cycles mixed with palm oil 5 g and 10 g/100 g starch.
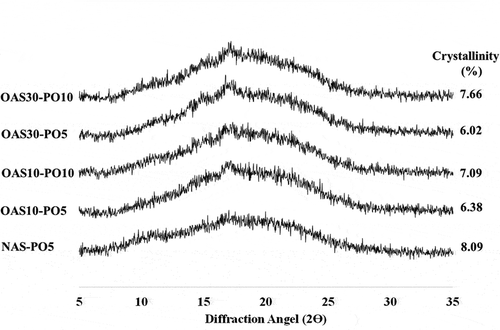
Ozonation decreased the degree of recrystallisation. Native starch (NAS-PO5) displayed 8.92% crystallinity. When starch was pretreated with ozonation either for 10 or 30 cycles, recrystallisation decreased with 6.38% and 6.02% crystallinities, respectively. Therefore, the increase in the ozonation cycle decreased recrystallisation. However, a different trend was noted when ozonated starch was mixed with 10 g palm oil/100 g starch as observed in the comparison of the crystallinities of OAS10-PO10 and OAS30-PO10. This different trend in the effect of ozonation could be explained by the effect of oil on recrystallisation. Similar to the effect of palm oil on functional properties, the reducing effect of palm oil at 10 g palm oil/100 g starch apparently outpowered the increasing effect of ozonation. Further comparison with freeze–thaw stability suggested that the finding on recrystallisation was consistent with the trend in syneresis. At 10 g palm oil/100 g starch, an increase in recrystallisation was accompanied with an increase in syneresis.
Pasting properties
The typical pasting curves of ozonated arrowroot starches in the presence of 5 and 10 g palm oil/100 g starch are shown in and . Neither ozonation nor palm oil addition affected the pasting temperature of the starch. A previous study on ozonated banana starch in the absence of oil also revealed that pasting temperature remains unchanged after ozonation.[Citation10] This finding suggests that neither carboxyl nor starch–lipid complex is sufficient for altering the pasting temperature of the starch. Similarly, final and breakdown viscosities were relatively unchanged by ozonation or palm oil addition. Our finding on the effect of ozonation on final and breakdown viscosities was in line with a previous study.[Citation10]
Table 2. Pasting properties of ozonated arrowroot starches in the presence of palm oil.
Figure 3. Typical pasting curves of native arrowroot starch along with their corresponding ozonated forms. NAS-PO5 = Native arrowroot starch mixed with palm oil 5 g/100 g starch; OAS10-PO5 and OAS10-PO10 = arrowroot starch ozonated 10 cycles mixed with respectively palm oil 5 g and 10 g/100 g starch; OAS30-PO5, OAS30-PO10 = Arrowroot starch ozonated 30 cycles mixed with palm oil 5 g and 10 g/100 g starch.
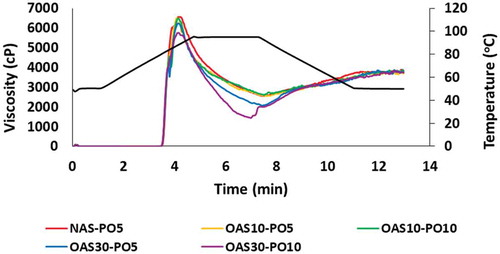
The effect of palm oil or ozonation on peak viscosity was observed particularly in 30 cycles or 10 g palm oil/100 g starch addition. No effect of 10-cycle ozonation on peak viscosity was observed in the presence of 5 g palm oil/100 g starch (OAS10-PO5) compared with that on its native form (NAS-PO5). However, ozonation for 30 cycles decreased the peak viscosity to 6283 cP. A similar trend was found in the presence of 10 g palm oil/100 g starch in which the peak viscosity of 10-cycle ozonated starch was approximately 6609 cP that decreased to 5790 cP after 30 cycles. The addition of oil also significantly decreased the peak viscosity when 30-cycle ozonation was applied. The reduction of peak viscosity due to lipids is also found in another study on bambara starch, fufu flour and other starches.[Citation13,Citation14,Citation21,Citation27,Citation36–Citation39]
The hold viscosity decreased from 2539 cP to 1974 cP, especially in 30-cycle ozonation compared with that of the native one in the presence of 5 g palm oil/100 g starch. Viscosity also decreased from 2568 cP in the 10-cycle ozonated starch to 1364 cP in the 30-cycle ozonated starch in the presence of 10 g palm oil/100 g starch. Cahyana et al.[Citation10] observed an increase in the hold viscosity of starch after ozonation in the absence of lipids, but this finding was not in line with the present results. Setback similarly increased as the number of ozonation cycles increased, particularly in 30 cycles. This increase was attributed to the decrease in the hold viscosity. In general, pasting properties, particularly pasting temperature, final viscosity and breakdown of starch, are not relatively affected by ozonation and presence of lipids. High lipid concentrations (at least 10 g palm oil/100 g starch) and extensive ozonation (30 cycles) were required to cause an effect on certain pasting properties, such as peak, hold and setback viscosities.
Previous studies reported that the ozonation of starch in the absence of oil increases the peak and hold viscosities of banana or arrowroot starch, and this increase may be related to the increase in starch polarity due to a high carboxyl content. The opposite result in this study in the presence of palm oil could be linked to several factors. An increase in the number of ozonation cycles and the concentration of palm oil increased the CI of starch. This increase was consistent with the decrease in peak and hold viscosities. The higher the CI was, the lower the peak and hold viscosities would be. Therefore, the lipid–starch complex might play a role in reducing peak and hold viscosities.
Ozonation resulted in not only a high carboxyl content but also a high CI. High CIs might be related to several factors, not to the high carboxyl content, as explained in the previous section. This finding indicated that ozonated starch mixed with lipid exhibits two opposite effects; that is, an increase occurred because of carboxyl contents, whereas a decrease was observed because of starch complex formation. As such, high palm oil concentrations and extensive ozonation (at least 30 cycles) were required to achieve low peak and hold viscosities.
Conclusion
Starch–lipid complex formation increased as the number of ozonation cycles or the concentration of palm oil increased. An increase in the CI after ozonation might be attributed to more available entrances to the amylose cavity because of the depolymerization or ring opening of the glucose chain in amylose rather than the high polarity of the carboxyl group. However, ozonation and palm oil differently modulated the functional properties of starch. The effect of ozonation, particularly with 5 g palm oil/100 g starch concentration, on functional properties was opposite to the effect of palm oil. Ozonation increased the carboxyl content, freeze–thaw stability, gel strength, solubility and swelling volume, but the opposite was observed with palm oil. The increase in the functional properties after ozonation was seemingly due to the predominant contribution of carboxyl content rather than that of the lipid–starch complex formation, particularly up to 5 g palm oil/100 g starch addition. Indeed, the lipid–starch complex tended to counteract the effect of carboxyl contents, as observed in the ozonated starch in the presence of high lipid concentrations (10 g palm oil/100 g starch). Furthermore, the response to the freeze–thaw stability after ozonation and palm oil addition was related to the degree of crystallinity during recrystallization. Recrystallization was increased by lipids but was decreased by ozonation. A similar observation was found in color properties. Palm oil in native starch decreased lightness and changed color as indicated by ∆E and ∆H. Ozonation increased the lightness of starch probably due to pigment oxidation. However, the effect of ozonation was ineffective in the presence of a high lipid concentration, i.e. 10 g palm oil/100 g starch. Pasting properties were relatively unchanged by palm oil and ozonation. High palm oil concentrations (at least at 10 g palm oil/100 g starch) and extensive ozonation (30 cycles) were required to affect certain pasting properties. At least two driving factors, namely, carboxyl content and lipid–starch complex, were involved when ozonated starch was mixed with palm oil. The effect of carboxyl content counteracted the effect of the lipid–starch complex. Starch properties, such as functional and pasting properties, which were affected by both factors, required certain lipid concentrations or ozonation to have a sufficient effect on the lipid–starch complex compared with the opposite effect of the carboxyl group.
Acknowledgments
This work was supported by financial sponsorship from Lembaga Pengelola Dana Pendidikan (LPDP), Indonesia.
References
- Lawal, O.; Adebowale, K. O. Physicochemical Characteristics and Thermal Properties of Chemically Modified Jack Bean (Canavalia ensiformis) Starch. Carbohydr. Polym. 2005, 60(3), 331–341. DOI: 10.1016/j.carbpol.2005.01.011.
- Jyothi, A. N.; Sajeev, S.; Sreekumar, J. N. Hydrothermal Modifications of Tropical Tuber Starches. 1. Effect of Heat-Moisture Treatment on the Physicochemical, Rheological and Gelatinization Characteristics. Starch - Stärke 2010, 62(1), 28–40. DOI: 10.1002/star.200900191.
- Cahyana, Y.; Wijaya, E.; Halimah, T. S.; Marta, H.; Suryadi, E.; Kurniati, D. The Effect of Different Thermal Modifications on Slowly Digestible Starch and Physicochemical Properties of Green Banana Flour (Musa acuminata Colla). Food Chem. 2019, 274, 274–280. DOI: 10.1016/j.foodchem.2018.09.004.
- Marta, H.; Cahyana, Y.; Arifin, H.; Khairani, L. Comparing the Effect of Four Different Thermal Modifications on Physicochemical and Pasting Properties of Breadfruit (Artocarpus altilis) Starch. Int. Food Res. J. 2019, 26(1), 269–276.
- Chan, H. T.; Bhat, R.; Karim, A. A. Physicochemical and Functional Properties of Ozone-Oxidized Starch. J. Agric. Food. Chem. 2009, 57(13), 5965–5970. DOI: 10.1021/jf9008789.
- Vanier, N.; Halal, S.; Dias, Á.; Zavareze, E. Molecular Structure, Functionality and Applications of Oxidized Starches: A Review. Food Chem. 2016, 221. DOI: 10.1016/j.foodchem.2016.10.138.
- Matta Junior, M.; Castanha, N.; Boralli Prudente Dos Anjos, C.; Augusto, P.; Sarmento, B. S. Ozone Technology as an Alternative to Fermentative Processes to Improve the Oven-Expansion Properties of Cassava Starch. Food Res. Int. 2019, 123, 56–63. DOI: 10.1016/j.foodres.2019.04.050.
- Castanha, N.; Matta Junior, M. D. D.; Augusto, P. E. D. Potato Starch Modification Using the Ozone Technology. Food Hydrocoll. 2017, 66, 343–356. DOI: 10.1016/j.foodhyd.2016.12.001.
- Çatal, H.; İbanoğlu, Ş. Effect of Aqueous Ozonation on the Pasting, Flow and Gelatinization Properties of Wheat Starch. LWT - Food Sci. Technol. 2014, 59(1), 577–582. DOI: 10.1016/j.lwt.2014.04.025.
- Cahyana, Y.; Titipanillah, R.; Mardawati, E.; Sukarminah, E.; Rialita, T.; Andoyo, R.; Djali, M.; Hanidah, -I.-I.; Setiasih, I. S.; Handarini, K. Non-Starch Contents Affect the Susceptibility of Banana Starch and Flour to Ozonation. J. Food Sci. Technol. 2018, 55(5), 1726–1733. DOI: 10.1007/s13197-018-3085-2.
- Oladebeye, A. O.; Oshodi, A. A.; Amoo, I. A.; Karim, A. A. Gaseous Ozonation of Pigeon Pea, Lima Bean, and Jack Bean Starches: Functional, Thermal, and Molecular Properties. Starch‐Stärke 2018, 70(11–12), 1700367. DOI: 10.1002/star.201700367.
- Handarini, K.; Hamdani, J. S.; Cahyana, Y.; Setiasih, I. S. Gaseous Ozonation at Low Concentration Modifies Functional, Pasting, and Thermal Properties of Arrowroot Starch (Maranta Arundinaceae). Starch - Stärke 2020, 72(5–6), 1900106. DOI: 10.1002/star.201900106.
- D’Silva, T. V.; Taylor, J. R.; Emmambux, M. N. Enhancement of the Pasting Properties of Teff and Maize Starches through Wet–Heat Processing with Added Stearic Acid. J. Cereal Sci. 2011, 53(2), 192–197. DOI: 10.1016/j.jcs.2010.12.002.
- Oyeyinka, S. A.; Singh, S.; Venter, S. L.; Amonsou, E. O. Effect of Lipid Types on Complexation and Some Physicochemical Properties of Bambara Groundnut Starch. Starch‐Stärke 2017, 69(3–4), 1600158. DOI: 10.1002/star.201600158.
- Chang, F.; He, X.; Fu, X.; Huang, Q.; Jane, J.-L. Effects of Heat Treatment and Moisture Contents on Interactions between Lauric Acid and Starch Granules. J. Agric. Food. Chem. 2014, 62(31), 7862–7868. DOI: 10.1021/jf501606w1.
- Alvarez-Ramirez, J.; Vernon-Carter, E.; Carrillo-Navas, H.; Meraz, M. Effects of Cooking Temperature and Time on the Color, Morphology, Crystallinity, Thermal Properties, Starch-Lipid Complexes Formation and Rheological Properties of Roux. LWT Food Sci. Technol. 2018, 91, 203–212. DOI: 10.1016/j.lwt.2018.01.038.
- Farooq, A. M.; Dhital, S.; Li, C.; Zhang, B.; Huang, Q. Effects of Palm Oil on Structural and in Vitro Digestion Properties of Cooked Rice Starches. Int. J. Biol. Macromol. 2018, 107, 1080–1085. DOI: 10.1016/j.ijbiomac.2017.09.089.
- Klein, B.; Vanier, N. L.; Moomand, K.; Pinto, V. Z.; Colussi, R.; da Rosa Zavareze, E.; Dias, A. R. G. Ozone Oxidation of Cassava Starch in Aqueous Solution at Different Ph. Food Chem. 2014, 155, 167–173. DOI: 10.1016/j.foodchem.2014.01.058.
- Hossain, M. Applications of Palm Oil and Palm Kernel Oils in Different Food Products of Bangladesh. Daffodil Int. Univ. J. Sci. Technol. 2013, 8(2), 33–38.
- Tang, M. C.; Copeland, L. Analysis of Complexes between Lipids and Wheat Starch. Carbohydr. Polym. 2007, 67(1), 80–85. DOI: 10.1016/j.carbpol.2006.04.016.
- Chang, F.; He, X.; Huang, Q. Effect of Lauric Acid on the V-Amylose Complex Distribution and Properties of Swelled Normal Cornstarch Granules. J. Cereal Sci. 2013, 58(1), 89–95. DOI: 10.1016/j.jcs.2013.03.016.
- Gilbert, G.; Spragg, S. Iodometric Determination of Amylose: Methods in Carbohydrate Chemistry Starch; Academic Press: New York, 1964; Vol. 4, pp 168–169.
- Marta, H.; Cahyana, Y.; Djali, M.; Arcot, J.; Tensiska, T. A Comparative Study on the Physicochemical and Pasting Properties of Starch and Flour from Different Banana (Musa Spp.) Cultivars Grown in Indonesia. Int. J. Food Prop. 2019, 22(1), 1562–1575. DOI: 10.1080/10942912.2019.1657447.
- Godet, M. C.; Buléon, A.; Tran, V.; Colona, P. Structural Features of Fatty Acid-Amylose Complexes. Carbohydr. Polym. 1993, 21, 91–95. DOI: 10.1016/0144-8617(93)90003-M.
- Kaur, K.; Singh, N. Amylose-Lipid Complex Formation during Cooking of Rice Flour. Food Chem. 2000, 71, 511–517. DOI: 10.1016/S0308-8146(00)00202-8.
- Chung, H.-J.; Hoover, R.; Liu, Q. The Impact of Single and Dual Hydrothermal Modifications on the Molecular Structure and Physicochemical Properties of Normal Corn Starch. Int. J. Biol. Macromol. 2009, 44(2), 203–210. DOI: 10.1016/j.ijbiomac.2008.12.007.
- Raphaelides, S. N.; Georgiadis, N. Effect of Fatty Acids on the Rheological Behaviour of Maize Starch Dispersions during Heating. Carbohydr. Polym. 2006, 65(1), 81–92. DOI: 10.1016/j.carbpol.2005.12.028.
- Van Lonkhuysen, H.; Blankestijn, J. Influence of Monoglycerides on the Gelatinization and Enzymatic Breakdown of Wheat and Cassava Starch. Starch‐Stärke 1976, 28(7), 227–233. DOI: 10.1002/star.19760280705.
- Hibi, Y. Effect of Lipids on the Viscoelastic Properties of Rice Starch Gel. Starch 1994, 46, 44–48.
- Singh, N.; Cairns, P.; Morris, V.; Smith, A. C. Physical Properties of Extruded Wheat Starch-Additive Mixtures. Cereal Chem. 1998, 75, 325–330. DOI: 10.1094/CCHEM.1998.75.3.325.
- Lii, C. Y.; Lai, V. M. F.; Shen, M. C. Changes in Retrogradation Properties of Rice Starches with Amylose Content and Molecular Properties. Cereal Chem. 2004, 81(3), 392–398. DOI: 10.1094/CCHEM.2004.81.3.392.
- Gudmundsson, M. Retrogradation of Starch and the Role of Its Components. Thermochim. Acta 1994, 246(2), 329–341. DOI: 10.1016/0040-6031(94)80100-2.
- Richardson, G.; Kidman, S.; Langton, M.; Hermansson, A.-M. Differences in Amylose Aggregation and Starch Gel Formation with Emulsifiers. Carbohydr. Polym. 2004, 58(1), 7–13. DOI: 10.1016/j.carbpol.2004.06.013.
- Sandhu, H. P. S.; Manthey, F. A.; Simsek, S. Ozone Gas Affects Physical and Chemical Properties of Wheat (Triticum Aestivum L.) Starch. Carbohydr. Polym. 2012, 87(2), 1261–1268. DOI: 10.1016/j.carbpol.2011.09.003.
- Putseys, J.; Lamberts, L.; Delcour, J. Amylose-Inclusion Complexes: Formation, Identity and Physico-Chemical Properties. J. Cereal Sci. 2010, 51(3), 238–247. DOI: 10.1016/j.jcs.2010.01.011.
- Sanni, L.; Kosoko, S.; Adebowale, A.; Adeoye, R. The Influence of Palm Oil and Chemical Modification on the Pasting and Sensory Properties of Fufu Flour. Int. J. Food Prop. 2004, 7(2), 229–237. DOI: 10.1081/JFP-120026059.
- Copeland, L.; Blazek, J.; Salman, H.; Tang, M. C. Form and Functionality of Starch. Food Hydrocoll. 2009, 23(6), 1527–1534. DOI: 10.1016/j.foodhyd.2008.09.016.
- Guraya, H. S.; Kadan, R. S.; Champagne, E. T. Effect of Rice Starch‐Lipid Complexes on in Vitro Digestibility, Complexing Index, and Viscosity. Cereal Chem. 1997, 74(5), 561–565. DOI: 10.1094/CCHEM.1997.74.5.561.
- Wokadala, O. C.; Ray, S. S.; Emmambux, M. N. Occurrence of Amylose–Lipid Complexes in Teff and Maize Starch Biphasic Pastes. Carbohydr. Polym. 2012, 90(1), 616–622. DOI: 10.1016/j.carbpol.2012.05.086.