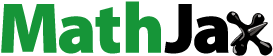
ABSTRACT
The core objective of the current study was to assess the effect of encapsulation on the viability and stability of probiotic bacteria under simulated gastrointestinal digestion and thermal conditions. Purposely, probiotics were encapsulated with hydrogel matrices (sodium alginate and carrageenan) using encapsulator. Furthermore, developed microbeads were characterized by X-ray diffraction (XRD) and Fourier Transform Infrared (FTIR) spectroscopy to elucidate the interaction between hydrogel matrices and probiotics. The viability of probiotics assessed by subjecting to simulated GIT and thermal conditions. Encapsulation exhibited a significant (p< .05) effect on the survival and stability of probiotics. Overall, a decreasing trend in viability of probiotics was observed in all treatments. A rapid log reduction was observed when free probiotic cells were stored at refrigeration temperature compared to encapsulated probiotic bacteria. Likewise, in vitro gastrointestinal assay, only 3 log while in case of non-encapsulated bacteria 6 log reduction was recorded. In short, the results of the viable count in the case of encapsulated cells were above recommended level (106 cfu/g) under thermal as well as in GIT conditions.
Introduction
Functional foods carrying probiotics have numerous claimed health benefits. The awareness on the functional foods has increased owing to their marketing demands globally. The Food and Agriculture Organization/World Health Organization [FAO/WHO] defined probiotic as “live microorganisms which when administered in adequate amounts (106–107 cfu/g) confer health benefit on the host”. Health Canada has recommended minimum (1 × 109 cfu/g) of probiotic containing food.[Citation1] Various functional dairy and nondairy foodstuffs are manufactured with incorporation of different probiotics.[Citation2] Probiotics refer to the wide range of microorganisms especially bacteria as well as yeast. Most widely studied group is lactic acid bacteria [LAB] and Lactobacillus acidophilus and bifidobacteria are considered to be the most important probiotics with beneficial possessions on the human gastro-intestinal [GI] pathway.[Citation3] All promising health benefits of probiotics are associated with their survivability and stability in carrier food and gastrointestinal conditions.
However, the viability and stability of these health promoting probiotics is questionable. In order to be effectual, the sufficient level of probiotics must retain in carrier food during storage and at the time of consumption. Several factors (extrinsic and extrinsic) affect the survival of probiotics, including oxygen, acidity in fermented products, pH, temperature, and production of different antimicrobial agents during processing. In dairy products, freezing injury and toxicity are the major hurdles in their survival during storage conditions.[Citation4]
The demand for probiotics in food and as supplements is increasing across the world. A rapid growth has been observed in the marketing of probiotics enriched food in Asia pacific.[Citation5] The consumption of probiotics enriched food is increasing due to their numerous health benefits.[Citation6] The storage, processing and other technological conditions reduce the level of probiotics in carrier food. The consumption of the product, containing probiotics don’t deliver the claimed benefits. The hostile conditions in stomach and intestine affect the survival of probiotics. The viability and the stability of probiotics are the major challenge for food manufacturer.[Citation7,Citation8] So, maintaining the viability of probiotics under stressed conditions and food products require a protection approach.[Citation9]
The various traditional methods or techniques (resistant strains, two step fermentation, addition of micronutrients, oxygen control, and stress adaptation) for the protection of probiotics are not effective in their target delivery.[Citation10] However, microencapsulation is gaining attention as it ensures the recommended level and target delivery of the core material. Encapsulation technique not only protects the probiotics from various harsh conditions, and it also maintains the therapeutic level of probiotics in food product.[Citation13] Microencapsulation of probiotics with compatible encapsulating material is the approach to protect live cells from adverse acidic conditions.[Citation15] Various encapsulating materials are Generally Recognized as Safe (GRAS) status are being used for encapsulation of probiotics on lab as well as industrial scale. The main type of materials includes proteins lipids polysaccharides and their derivatives.[Citation16] Sodium alginate and carrageenan are considered as effective encapsulating polymer among the hydrogel matrices. Sodium alginate is being used widely for encapsulation of probiotics due to its biocompatibility, versatility, heat, and acid resistance properties. Both, sodium alginate and carrageen wall materials are economical, nontoxic and easy to handle.[Citation17]
In this context, Lactobacillus acidophilus were encapsulated using two hydrogel matrices and the obtained beads were characterized using different instruments. The viability and stability of free and encapsulated cells were assessed by exposing the free and encapsulated probiotics under simulated technological and simulated GIT conditions.
Materials and methods
Procurement of material
Pure culture of Probiotics (L. acidophilus ATCC-4356) and encapsulating materials (sodium alginate and carrageenan) were obtained from National Institute of Food Science & Technology (NIFSAT), University of Agriculture, Faisalabad. Sodium alginate having ratio of mannuronic (M) to guluronic (G) units (M/G = 65/35) and molar weight (MW = 90–180 KDa) was used in this study. All general chemicals (Sigma–Aldrich GmbH, Sternheim, Germany) were purchased from scientific store.
Culture activation
The activation of obtained probiotics was as described by Afzaal et al.[Citation20] by using de Man, Rogosa, and Sharpe (MRS) liquid medium (broth). The conditions were settled for optimum growth at 37°C 24 hours. The obtained growth of the probiotic was centrifuged, washed using sterile saline solution. The concentration of the target probiotics was adjusted at 1010 cfu/mL.
Preparation of beads of probiotics
The encapsulating of probiotics (L. acidophilus) was done by following method as described by Yeung et al.[Citation21] with slight amendment. Purposely, the solutions of hydrogel matrices (sodium alginate and carrageenan) were prepared with defined concentration (2.0% w/v). The prepared solutions were autoclaved at 121 °C (15 psi) for 15 min. The aseptic solutions of sodium alginate and carrageenan were mixed with 2 mL of 1010 cfu/mL probiotics obtained in activation process. The beads were prepared aseptically by using an encapsulator (B-390, Buchi-Switzerland) in standard operating conditions. Encapsulating materials liquors were injected into hardening solutions of calcium chloride (0.1 M) solution. Later on, the desired beads were acquired by filtration, washed by sterile deionized water. The acquired beads were stored in physiological saline solution at 4°C.
Characterization of beads
Morphological study of beads
The physical appearance of prepared microbeads was studied using Optical Microscope (SWIFT M7000D & 4000D) available at NIFSAT, University of Agriculture, Faisalabad, Pakistan. The results were recorded as described by Praepanitchai et al.[Citation22] Images were taken by the digital camera (Canon D-1200 Lens-75/300 mm). The carrageenan and alginate beads were vortexed before the examination. The size was measured using stage micrometer as previously described by Afzaal et al.,[Citation20] The size of about 100 beads were measured and average of each sample was considered for final demonstration of the results. Observations of size or diameter of the sodium and carrageenan microbeads were done by random selection of the beads.
Encapsulation efficiency (EE)
The efficiency of the encapsulation probiotic bacteria was investigated according to the method followed by Vaziri et al.,[Citation23] Microbeads of probiotics were fragmented using stomacher (Seward, UK) in buffer solution for 15 min, until all the entrapped probiotics released from the beads. Briefly, one-gram encapsulated probiotics from each sample was mixed with 9 ml of phosphate buffer solution. Subsequently, pour plate technique was used to calculate/enumerate the number of entrapped cells. The plates were incubated at 37°C and anaerobic conditions were ensured. The encapsulation efficiency was recorded using equation (given below) in terms of colony forming units/bead (cfu/bead).
where, N is the number of viable entrapped cells released from microbeads [Sodium alginate/Carrageenan], N0 is the number of free cells added to the biopolymer mixture [Sodium alginate/Carrageenan] prior to encapsulation.
FTIR analysis
Fourier Transform Infrared (FTIR) spectra were recorded as the method described by Ashwar et al.[Citation24] The FTIR (Brucker Tensor II; Resolution4 cm-1; Signal-to-Noise Ratio8000:1 to 45000:1;
Wavelength Range Near IR, Mid IR and Far IR spectral range) available at High Tech lab of Government College University, Faisalabad was used for characterization of alginate and carrageenan beads. The various peaks of the FTIR spectrum of sodium alginate and carrageen powder were interpreted.
X-ray diffraction [XRD] study
XRD study was carried out to characterize the sodium and carrageenan coated beads. The obtained pattern was recorded using the method of pour.[Citation25] The XRD available at high tech lab of Government College University, Faisalabad was used to characterize the encapsulating materials and the obtained beads.
In vitro assay
In-vitro assay was carried out to investigate the survival of free and encapsulated probiotic bacteria under simulated gastrointestinal conditions. For this purpose, simulated solutions were prepared by using analytical grade chemicals and aseptic conditions.
Survival of free and encapsulated probiotic bacteria (Lactobacillus acidophilus) in simulated gastric juice (SGJ)
Free and encapsulated beads of sodium alginate and carrageenan were subjected to simulated gastric fluid/juice. The tolerance of free and encapsulated probiotic bacteria was assessed by following Shafei et al.[Citation26, Citation27] with some modifications. The simulated gastric fluid was prepared by adding pepsin (3 g/L) in sterile sodium chloride solution. The pH of the prepared gastric juice was adjusted by using 0.1 N HCL. The pH of the solution was adjusted to about 2. The prepared solution was stored for the experiment. One gram of microbeads was suspended in a prepared simulated gastric solution. Free/unencapsulated and encapsulated probiotic bacteria were mixed with the gastric solution and were incubated (shaking incubator) at 37°C and 110 rpm. MRS agar was used to determine the number of viable bacteria by counting the cfu/g. The survivability of probiotic in free and encapsulated was recorded with a time interval of 0, 30, 60, 90, and 120 minutes. The results were recorded in triplicate.
Survival of free and encapsulated probiotic bacteria in simulated intestinal juice (SIJ)
The survival of probiotics is also important in the intestinal conditions after their passage through the stomach. The survivability of free and encapsulated probiotic bacteria was accessed by the method as described by Shafei et al. and Singh et al.[Citation26,Citation17] The tolerance of encapsulated bacteria (bead cells) to simulated intestinal transit conditions was determined by subjecting the free and encapsulated probiotic bacteria to the simulated intestinal solution. Briefly, bile salt (3 g/L) and pancreatic (10 g/L) were dissolved in phosphate buffer having pH (8.0). The pH (7.5) of the intestinal fluid was adjusted by using sterilized NaOH. One gram of microbeads was suspended in a prepared simulated gastric solution. MRS agar was used to determine the number of viable bacteria by counting the cfu. Both types of cells i.e unencapsulated/free and encapsulated were separately added to prepared simulated solutions and stored in shaking incubator at 37°C and 110 rpm. The viability/survival of free/unencapsulated and encapsulated suspending cells was calculated on pleating MRS agar with encoded interval of time (0, 30, 60, 90, and 120 minutes).
Thermal stability of free and encapsulated probiotics
Free and encapsulated probiotics were subjected to heat treatments by following method as previously described by Praepanitchai et al.[Citation22] with slight modifications. The viability and stability of free and encapsulated probiotics was assessed at 65°C with an interval of 0, 15, 30, and 45 minutes. Briefly, 1 g of both type of probiotic cells (encapsulated and free) were added in test tubes containing saline solution. Later on, the test tubes were incubated in water bath at defined temperature for different intervals of time. All samples were cooled to drop temperature. Enumeration of survived probiotics was done on MRS agar.
Viability of free and encapsulated cells under refrigerated conditions
Viability and stability under refrigeration was assessed by exposing the free and encapsulated probiotics to refrigeration temperature by adopting method of Araújo et al.[Citation12]. Briefly, the both type of cells were stored at 4°C and viability was enumerated with an interval of 0, 5, 10, and 15 days of storage.
Results and discussion
Characterization of beads
The probiotic bacteria were encapsulated with sodium alginates and carrageenan. Encapsulated probiotics were characterized using a microscope and pictures were collected (). The morphological analysis, immediately after encapsulation was carried out. The results exhibited that cells appeared rod-shaped and the blank beads coated with alginate were morphological similar to beads containing probiotics. The findings are also corroborate with the results as reported by Mirzaei et al.[Citation10]. All prepared beads shrank to about 1 mm. The mean or average size of microbead size of sodium alginate beads were 716 µm, while for carrageen it was 733 µm. The size of the microcapsule is affected by the concentration and nature of the polymer used for encapsulation of probiotics. The hydration capacity is an important factor in controlling the diameter of the capsule [Citation11]. Coarse and aggregated surfaces were observed from both alginate and carrageenan microbeads. Alginate beads (with and without containing probiotics) were similar in shape. It was observed that the surface of all samples was severely dried after dehydration. Surface cavities were also observed in both alginate and carrageenan beads. Cells were not in original shape as they shrank after dehydration. It was observed that the carrageenan microbeads were larger in size as compared to sodium alginate beads. The increase in size could be due to chemical structure and concentrations of the solutions used for encapsulation of probiotics. Characterization of microbeads was done in a study[Citation32] who reported that the spheres shape microbeads are easier to produce and use. Ashwar et al.[Citation24] reported in a study that the smooth and spherical hydrogel beads formed while dropping the alginate alone and alginate with various concentrations of soy protein isolate (SPI). Furthermore, the gelation of the two biopolymeric mixtures (sodium alginate and SPI) is likely to occur via electrostatic complexion and the Ca2+ ions neutralize electrostatic repulsion and form salt bridges between the biopolymeric networks. The structure of outer wall provides an efficient protective barrier to diffusion toward the core of the capsules, the entrapped rod-shaped probiotic cells are clearly observed inside the membranes of the hydrogel beads.
Encapsulation efficiency
Two different encapsulating materials (sodium alginate and carrageenan) exhibited different encapsulation efficiency. Microbeads with wall material sodium alginate showed efficiency (98%) as compared to carrageen (96%). The number of entrapped cells after in case of sodium alginate and carrageenan were 8.54 ± .03 and 8.21 ± .06, respectively. The type of hydrogel matrices andmethod of encapsulation directly affects the encapsulation efficiency. In most of the studies carried out by different scientists proved that sodium alginate is more effective encapsulating polymer in terms of encapsulation efficacy and protection. The results showed that required cell number was entrapped by both type of materials. However, a greater number of cells were entrapped in sodium alginate matrix as compared to carrageenan. The results of the present study agreed with Donthidi et al.[Citation33] who reported higher encapsulation efficiency in case of sodium alginate-starch coating and efficiency varied owing to unfavorable factors. An effective intake of the product containing probiotics is required for colonizing of the probiotics in the colon. Encapsulation with alginate and whey protein enhanced the survival of probiotics.[Citation7]
Fourier transform infrared spectroscopy (FTIR) analysis
In case of alginates, the major peaks appeared at 3346.25 cm−1 related to O-H vibrational stretching. That could be related to intra, intermolecular bonding and free hydroxyl groups. A higher and broader area for O-H stretching was noticed in alginate beads in the presence of Ca2+ ions. Peak was also shifted to a higher wavenumber at 3354.96 cm−1 in the presence of calcium. The peak appeared at 1623.54 cm−1 indicted the carboxylic [COO-] asymmetric stretching. This peak was shifted to a higher wavenumber in the presence of Ca2+ ions, the intensity of the peak was also lowered as well. The peak at 1421.2 cm−1 is representative for symmetric stretching of carboxylic [COO-] groups in the polymer backbone. FTIR spectrum of powder and beads are presented in (). Like alginates, this polymer undergoes gelation in the presence of potassium or calcium ions.[Citation34] The peaks at wavenumber 3215.23 cm−1 were observed for O-H stretching vibrations. Alginates are polyampholytic polysaccharides are also known as polyelectrolytes. Alginates have the ability to cross-link or making hydrogels in the presence of mono or polyvalent metallic cations, typically calcium or potassium. The carrageenas are sulfated polysaccharides obtained from red seaweeds. The interaction of divalent cations enhances the mechanical and chemical integrity and stability resulting in their suitability for encapsulating various bioactive compounds, such as antioxidants, drugs, prebiotics, or probiotics. FTIR attains infrared spectra of compounds, and is a robust and simple technique to elucidate the chemical structure of the polymer of interest, and it helps in estimating the interaction between two or more components of interest, i.e., the encapsulant (polymer) and active ingredient. Beads presented relatively more intense peak for the stretch at the same wavenumber. The changed peak intensity might be an indication of changing crystallinity and bonding. Paşcalău et al.[Citation35] observed ester sulfate stretch at lower wavenumber. The peak at 1026.44 cm−1 is representing absorption for glycosidic linkage. The carrageenan beads presented a shift for this group toward higher wavenumber though retained similar intensity. The beads presented relatively broader areas compared to alginate powder. Minor peaks appeared at 912.3 cm−1 and 856.7 cm−1 are reported for 3,6-anhydro-galactose and secondary sulfate [CO-S] at C4 in galactose structure. These absorption peaks are more intense in the case of beads suggesting structural organization favored in the presence of calcium ions
X-ray diffraction analysis
The powdered alginates presented three crystalline peaks in diffract gram, i.e., 2θ = 14.0°, 22.8°, and 37°. This countered variation might be due to the difference in the ratio of guluronic and mannuronic acid residues in the alginate samples. In the case of carrageenan, the very mild diffraction peaks were noticed at 2θ = 19.8° and 30.5°. Beads prepared with a mixture of carrageenan and alginates resulted in a very broad peak at 2θ = 31.1°. In the presence of Ca2+ ions, the peak intensity improved at 13.8°. It suggests that the presence of calcium favor the structural organization of alginates by enhancing the linkages between sulfate groups and precipitated the peak intensity. Afzaal et al.[Citation36] found that the presence of divalent calcium crystallinity was enhanced in the cellulose/alginates-based membranes. However, the second peak in alginate beads remained almost similar to powdered (). The results are in line with.[Citation37] The diffraction peaks in beads did not present much change compared to powdered carrageenan. However, an additional peak with very minor intensity was noticed at 2θ = 34.2°. This might be due to the changes in the structural organization of carrageenan nucleating the associations. It was reported that extraction and purification have a great influence on the crystallinity of the carrageenan
Figure 3. X Ray diffraction study of Alginate powder (a), Alginate beads, (b) Carrageenan powder (c), and carrageenan microbeads (d)
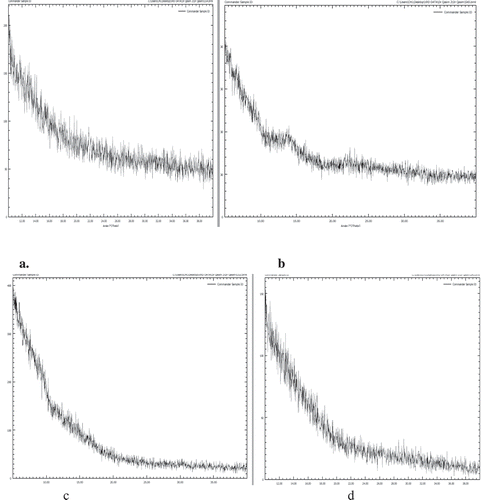
Stability of the encapsulated probiotic bacteria in simulated gastric juice
The viability and the stability of free and encapsulated probiotics was assessed in simulated conditions. The results showed an instant decrease in the case of free cells in contrast to the cells encapsulated with sodium alginate and carrageenan (). The cell viability in case of free cells decreased from 10.33 logcfu/g to 3.11 log cfu/g over an exposure of 120 minutes. While in case of encapsulation with sodium alginate decreased from 10.46 log cfu/g to 6.26 log cfu/g. Similarly, the viability of probiotics in case of carrageenan decreased from 10.45 log cfu/g to 6.05 log cfu/g over defined interval of time. Cell viability in stomach and intestinal conditions is important in order to get the desired benefits of probiotics. The results of the present study are in accordance with the results of Shi et al.[Citation8] who demonstrated that use of polymer for encapsulation of probiotics protects and maintain the desired viability of probiotics under acidic environment. The encapsulation of the cells with hydrogel materials improve the viability and stability in low pH medium. The survival rate of probiotics in case of sodium alginate was higher as compared to carrageenan. Poor survival in case of unencapsulated cells was recorded. The results demonstrated that encapsulation provides protection to probiotics in simulated gastric conditions. The results are also in line with the study of Afzaal et al.[Citation20] who reported a poor survival of free probiotics.
Stability of the encapsulated probiotic in simulated intestinal Juice
A rapid reduction in free cells was observed as compared to the encapsulated probiotics in simulated intestinal fluid with pH 7.5 (). The encapsulation of the cells with either sodium alginate or carrageenan had statistically significant effect (p < .05) on cell survival. The cell viability in case of free cells when exposed to high pH decreased from initial viable number of 9.66 log cfu/g to 2.85 log cfu/g over an exposure of 120 minutes. While in case of encapsulation with sodium alginate t decreased from 8.14 log cfu/g to 6.84 log cfu/g. In case of encapsulation of with carrageenan, it decreased from 9.53 log cfu/g to 6.63 log cfu/g. Cell viability in stomach and intestinal conditions is important in order to get the desired benefits of probiotics. The intestinal solution with high directly affects the release of the encapsulated cells. Encapsulating polymer at high pH solution, starts to dissolve that cause a rapid release of cells from the beads in intestinal sector.[Citation14,Citation38] The survival rate of probiotics in case of sodium alginate was higher as compared to carrageenan. Poor survival in case of unencapsulated cells was recorded. The results demonstrated that encapsulation provides protection to probiotics in simulated intestinal conditions.
Stability of free and encapsulated bacteria under thermal conditions
The survival of probiotics is very important when subjected to heat treatments. Most of the liquid food like milk and juices are passed through high heat treatment process (pasteurization, ultra-high temperature) for pathogenic destruction. When product is exposed for heat treatment the survival and the stability of probiotics become questionable. Encapsulation ensures the survival of probiotics under thermal conditions as reported by Teoh et al.[Citation39] The results of the present study indicated that high temperature negatively affected the survival of probiotics (). On the other hand, encapsulated probiotics cells showed better survival during exposure to a 65°C for defined interval of time. Encapsulation of probiotics caused a low heat transfer from surrounding, that maintains the viability during exposure to high temperature [Citation18].Furthermore, no significant difference regarding the type of encapsulating material was observed. The results of the current study are in accordance with the findings of Kim et al.[Citation19] who evaluated the survival of free and encapsulated probiotics under different heat treatment process with and without product. The viability of probiotics at pasteurization and other heat-treatment process is very important to get the claimed benefits of the probiotics. Encapsulation is an effective approach to maintain the minimum recommended level (106–107 cfu/g) of probiotics in functional foods [Citation40].
Stability of free and encapsulated bacteria under refrigeration conditions (4°C)
The viability of free and encapsulated probiotics is affected by low temperature. Low temperature interferes with enzymatic activity of the probiotic cell and make it difficult for nutrient uptake. The results indicated that encapsulation has a key role in increasing the stability of the probiotic bacteria at low temperature (). The result of the present study are in accordance with Nualkaekul et al.[Citation41] who reported that encapsulation ensure the viability in carrier food during storage at 4°C. L. plantarum encapsulated with pectin showed better viability compared to free cells under refrigeration conditions over 38 days of storage.[Citation42] In another study conducted by Çabuk and Harsa[Citation43] demonstrated that probiotics (L. acidophilus) encapsulated using encapsulating materials (whey protein and pullulan) showed low log reduction as compared to free cells over a period of 4 weeks of storage at refrigeration temperature (4°C). Similarly, the viability of encapsulated probiotics was found to be more than 9log cfu/g at 5°C over 6 months of storage.[Citation28, Citation29, Citation30, Citation31] The results of the present study are also correlated with the studies of Mokhtari et al.[Citation44] who also studied the storage stability of probiotics and reported that encapsulation is an effective approach to enhance the survival of probiotics under stressed conditions.
Conclusion
The findings of the study indicated that microencapsulation has a key role in improving the viability and stability of probiotics in stressed processing as well as in vitro digestion conditions. Sodium alginate and carrageenan are the effective polymer for ensuring the survival and target delivery of probiotics. The alginate polymer as encapsulating material has an edge over carrageenan owing to better cell release and pH filed response. Additionally, sodium alginate has provided better protection in thermal as well under simulated gastrointestinal conditions gastric. Microencapsulation ensured the endorsed level of probiotics under detrimental conditions for health benefits.
List of abbreviation
GIT | = | Simulated gastrointestinal |
SALG | = | Sodium Alginate |
CAG | = | Carageenan |
EE | = | encapsulation Efficiency |
MRS | = | De Man, Rogosa and Sharpe agar |
Acknowledgments
The authors extend their appreciation to the Deanship of Scientific Research at King Saud University for funding this research work through research group no. RG-1441-405. The authors are also thankful to Government College University Faisalabad and NIFSAT for providing technical support and laboratory facilities during research work. No funding has been provided by any agency for paper publication. This article does not contain any studies with human participants or animals performed by any of the authors. For this type of study, formal consent is not required. Authors declare that they have no conflict of interest.
References
- Hill, C.; Guarner, F.; Reid, G.; Gibson, G.-R.; Merenstein, D.-J.; Pot., B.; Calder, P.-C. Expert Consensus Document: The Int. Scientific Association for Probiotics and Prebiotics Consensus Statement on the Scope and Appropriate Use of the Term Probiotic. Nat. Rev. Gastroenterol. Hepatol. 2014, 11(8), 506.
- Hashemi, M.; Gheisari, H.-R.; Shekarforoush, S. Preparation and Evaluation of Low‐calorie Functional Ice Cream Containing Inulin, Lactulose and Bifidobacterium Lactis. Int. J. Dairy Technol. 2015, 68(2), 183–189. DOI: 10.1111/1471-0307.12173.
- Burgain, J.; Gaiani, C.; Linder, M.; Scher, J. Encapsulation of Probiotic Living Cells: From Laboratory Scale to Industrial Applications. J. Food Eng. 2011, 104(4), 467–483.
- Champagne, C.-P.; da Cruz, A.-G.-M. Daga Strategies to Improve the Functionality of Probiotics in Supplements and Foods Current. Opin. Food Sci. 2018, 22, 160–166. DOI: 10.1016/j.cofs.2018.04.008.
- Aryana, K.-J.; Olson, D.-W. A 100-year Review: Yogurt and Other Cultured Dairy Products. J Dairy sci. 2017, 100(12), 9987–10013.
- Kanmani, P.; Kumar, R.-S.; Yuvaraj, N.; Paari, K.-A.; Pattukumar, V.; Arul, V. Cryopreservation and Microencapsulation of a Probiotic in Alginate-chitosan Capsules Improves Survival in Simulated Gastrointestinal Conditions. Biotechnol. Bioprocess Eng. 2011, 16(6), 1106–1114. DOI: 10.1007/s12257-011-0068-9.
- Chotiko, A.; Sathivel, S. Development of a Combined Low-methoxyl-pectin and Rice-bran-extract Delivery System to Improve the Viability of Lactobacillus Plantarum under Acid and Bile Conditions. LWT-Food Sci. Technol. 2016, 66, 420–427. DOI: 10.1016/j.lwt.2015.10.058.
- Shi, L.-E.; Li, Z.-H.; Li, D.-T.; Xu, M.; Chen, H.-Y.; Zhang, Z.-L.; Tang, Z.-X. Encapsulation of Probiotic Lactobacillus Bulgaricus in Alginate–milk Microspheres and Evaluation of the Survival in Simulated Gastrointestinal Conditions. J. Food Eng. 2013, 117(1), 99–104. DOI: 10.1016/j.jfoodeng.2013.02.012.
- Terpou, A.; Papadaki, A.; Lappa, I. K.; Kachrimanidou, V.; Bosnea, L. A.; Kopsahelis, N. Probiotics in Food Systems: Significance and Emerging Strategies Towards Improved Viability and Delivery of Enhanced Beneficial Value. Nutrients. 2019, 11(7), 1591. DOI: 10.3390/nu11071591.
- Mirzaei, H.; Pourjafar, H.; Homayouni, A. Effect of Calcium Alginate and Resistant Starch Microencapsulation on the Survival Rate of Lactobacillus Acidophilus La5 and Sensory Properties in Iranian White Brined Cheese. Food Chem. 2012, 132(4), 1966–1970. DOI: 10.1016/j.foodchem.2011.12.033.
- Boudou, T.; Crouzier, T.; Ren, K.; Blin, G.; Picart, C. Multiple Functionalities of Polyelectrolyte Multilayer Films: New Biomedical Applications. Adv.Mate. 2010, 22(4), 441–467.
- de Araújo Etchepare, M.; Raddatz, G. C.; de Moraes Flores, É. M.; Zepka, L. Q.; Jacob-Lopes, E.; Barin, J. S.; Ferreira Grosso, C. R.; de Menezes, C. R. Effect of Resistant Starch and Chitosan on Survival of Lactobacillus Acidophilus Microencapsulated with Sodium Alginate. LWT - Food Science and Technology. 2016, 65, 511–517. DOI: 10.1016/j.lwt.2015.08.039.
- Zanjani, M. A. K.; Ehsani, M. R.; Ghiassi Tarzi, B.; Sharifan, A. Promoting Lactobacillus Casei and Bifidobacterium Adolescentis Survival by Microencapsulation with Different Starches and Chitosan and Poly L‐lysine Coatings in Ice Cream. J. Food Process. Preserv. 2018, 42(1), e13318. DOI: 10.1111/jfpp.13318.
- Ahmad, M.; Mudgil, P.; Maqsood, S. Camel Whey Protein Microparticles for Safe and Efficient Delivery of Novel Camel Milk Derived Probiotics. LWT. 2019, 108, 81–88. DOI: 10.1016/j.lwt.2019.03.008.
- Yeung, T.-W.; Arroyo-Maya, I.-J.; McClements, D.-J.; Sela, D.-A. Microencapsulation of Probiotics in Hydrogel Particles: Enhancing Lactococcus Lactis Subsp. Cremoris LM0230 Viability Using Calcium Alginate Beads. Food Funct. 2016, 7(4), 1797–1804. DOI: 10.1039/C5FO00801H.
- Praepanitchai, O.-A.; Noomhorm, A.; Anal, A.-K. Survival and Behavior of Encapsulated Probiotics (Lactobacillus Plantarum) in Calcium-Alginate-Soy Protein Isolate-Based Hydrogel Beads in Different Processing Conditions (Ph and Temperature) and in Pasteurized Mango Juice. Biomed Res. Int. 2019. DOI: 10.1155/2019/9768152.
- Singh, M.; Sharma, D.; Chauhan, R.; Goel, G. Skimmed Milk-based Encapsulation for Enhanced Stability and Viability of Lactobacillus Gastricus BTM 7 under Simulated Gastrointestinal Conditions. Probiotics Antimicrob. Proteins. 2019, 11(3), 850–856. DOI: 10.1007/s12602-018-9472-1.
- Ding, W. K.; Shah, N. P. Survival of Free and Microencapsulated Probiotic Bacteria in Orange and Apple Juices. Int. Food Res. J. 2008, 15(2), 219–232.
- Kim, S. J.; Cho, S. Y.; Kim, S. H.; Song, O. J.; Shin, I. S.; Cha, D. S.; Park, H. J. Effect of Microencapsulation on Viability and Other Characteristics in Lactobacillus Acidophilus ATCC 43121. LWT Food Sci. Technol. 2008, 41(3), 493–500. DOI: 10.1016/j.lwt.2007.03.025.
- Brinques, G. B.; Ayub, M. A. Z. Effect of Microencapsulation on Survival of Lactobacillus Plantarum in Simulated Gastrointestinal Conditions, Refrigeration, and Yogurt. J. Food Eng. 2011, 103(2), 123–128. DOI: 10.1016/j.jfoodeng.2010.10.006.
- Ashwar, B.-A.; Gani, A.; Gani, A.; Shah, A.; Masoodi, F.-A. Production of RS4 from Rice Starch and Its Utilization as an Encapsulating Agent for Targeted Delivery of Probiotics. Food Chem. 2018, 239, 287–294. DOI: 10.1016/j.foodchem.2017.06.110.
- Pour, -M.-M.; Saberi-Riseh, R.; Mohammadinejad, R.; Hosseini, A. Investigating the Formulation of Alginate-gelatin Encapsulated Pseudomonas Fluorescens (VUPF5 and T17-4 Strains) for Controlling Fusarium Solani on Potato. Int. J. Biol. Macromol. 2019, 133, 603–613. DOI: 10.1016/j.ijbiomac.2019.04.071.
- El-Shafei, K.; Elshaghabee, -F.-M.-F.; El-Sayed, H.-S.; Kassem, J.-M. Assessment the Viability Properties of Lactobacillus Casei Strain Using Labneh as a Carrier. Acta Scientiarum Polonorum Technologia Alimentaria. 2018, 17(3), 267–276.
- Moghaddas Kia, E.; Ghasempour, Z.; Ghanbari, S.; Pirmohammadi, R.; Ehsani, A. Development of Probiotic Yogurt by Incorporation of Milk Protein Concentrate (MPC) And Microencapsulated Lactobacillus Paracasei in Gellan-caseinate Mixture. Br. Food J. 2018, 120(7), 1516–1528. DOI: 10.1108/BFJ-12-2017-0668.
- Horwitz, W.; Chichilo, P.; Reynolds, H. Official Methods of Analysis of the Association of Official Analytical Chemists. Off Methods Anal Assoc Off Anal Chem. 1970.
- Karimi, R.; Mortazavian, A.-M.; Amiri-Rigi, A. Selective Enumeration of Probiotic Microorganisms in Cheese. Food Microbiol. 2012, 29(1), 1–9. DOI: 10.1016/j.fm.2011.08.008.
- Akgun, A.; Yazici, F.; Gulec, H.-A. The Combined Effect of Probiotic Cultures and Incubation Final pH on the Quality of Buffalo Milk Yogurt during Cold Storage. Food Sci. Nutr. 2018, 6(2), 492–502. DOI: 10.1002/fsn3.580.
- Pradeep Prasanna, P.-H.; Charalampopoulos, D. Encapsulation in an Alginate–goats’ Milk–inulin Matrix Improves Survival of Probiotic Bifidobacterium in Simulated Gastrointestinal Conditions and Goats’ Milk Yoghurt. Int. J. Dairy Technol. 2019, 72(1), 132–141. DOI: 10.1111/1471-0307.12568.
- Volić, M.; Pajić-Lijaković, I.; Djordjević, V.; Knežević-Jugović, Z.; Pećinar, I.; Stevanović-Dajić, Z.; Bugarski, B. Alginate/soy Protein System for Essential Oil Encapsulation with Intestinal Delivery. Carbohydr. Polym. 2018, 200, 15–24. DOI: 10.1016/j.carbpol.2018.07.033.
- Dias, C.-O.; de Almeida, J.-D.-S.-O.; Pinto, -S.-S.; de Oliveira Santana, F.-C.; Verruck, S.; Müller, C.-M.-O.; Amboni, R.-D.-D.-M.-C. Development and Physico-chemical Characterization of Microencapsulated Bifidobacteria in Passion Fruit Juice: A Functional Non-dairy Product for Probiotic Delivery. Food Biosci. 2018, 24, 26–36. DOI: 10.1016/j.fbio.2018.05.006.
- Donthidi, A. R.; Tester, R. F.; Aidoo, K. E. Effect of Lecithin and Starch on Alginate-encapsulated Probiotic Bacteria. J. Microencapsulation. 2010, 27(1), 67–77.
- Huo, W.; Xie, G.; Zhang, W.; Wang, W.; Shan, J.; Liu, H.; Zhou, X. Preparation of a Novel Chitosan-microcapsules/starch Blend Film and the Study of Its Drug-release Mechanism. Int. J. Biol. Macromol. 2016, 87, 114–122. DOI: 10.1016/j.ijbiomac.2016.02.049.
- Rao, -K.-K.; Naidu, B.-V.-K.; Subha, M.-C.-S.; Sairam, M.; Aminabhavi, T.-M. Novel Chitosan-based pH-sensitive Interpenetrating Network Microgels for the Controlled Release of Cefadroxil. Carbohydr. Polym. 2006, 66(3), 333–344.
- Paşcalău, V.; Popescu, V.; Popescu, G.-L.; Dudescu, M.-C.; Borodi, G.; Dinescu, A.; Paul, M. The Alginate/k-carrageenan Ratio’s Influence on the Properties of the Cross-linked Composite Films. J. Alloys Compd. 2012, 536, 418–423. DOI: 10.1016/j.jallcom.2011.12.026.
- Afzaal, M.; Saeed, F.; Arshad, M.-U.; Nadeem, M.-T.; Saeed, M.; Tufail, T. The Effect of Encapsulation on the Stability of Probiotic Bacteria in Ice Cream and Simulated Gastrointestinal Conditions. Probiotics and antimicrobial proteins, 11(4), 1348-1354.
- Nualkaekul, S.; Lenton, D.; Cook, M. T.; Khutoryanskiy, V. V.; Charalampopoulos, D. Chitosan Coated Alginate Beads for the Survival of Microencapsulated Lactobacillus Plantarum in Pomegranate Juice. Carbohydr. Polym. 2012, 90(3), 1281–1287.
- Teoh, P. L.; Mirhosseini, S. H.; Mustafa, S.; Manap, M. Y. A. Tolerance of Free and Encapsulated Probiotics Towards Heat Treatment and High Sodium Concentration. J Food Agric Environ. 2011, 9(1), 69–73.
- Çabuk, B.; Harsa, Ş. T. Protection of Lactobacillus Acidophilus NRRL-B 4495 under in Vitro Gastrointestinal Conditions with Whey Protein/pullulan Microcapsules. J. Biosci. Bioeng. 2015, 120(6), 650–656. DOI: 10.1016/j.jbiosc.2015.04.014.
- Mokhtari, S.; Khomeiri, M.; Jafari, S. M.; Maghsoudlou, Y.; Ghorbani, M. Descriptive Analysis of Bacterial Profile, Physicochemical and Sensory Characteristics of Grape Juice Containing Saccharomyces Cerevisiae Cell Wall-coated Probiotic Microcapsules during Storage. Int. J. Food Sci. Technol. 2017, 52(4), 1042–1048. DOI: 10.1111/ijfs.13370.
- Nualkaekul, S., & Charalampopoulos, D. (2011). Survival of Lactobacillus plantarum in model solutions and fruit juices. International Journal of Food Microbiology, 146(2), 111–117 doi:10.1016/j.ijfoodmicro.2011.01.040
- Rodrigues, D.; Sousa, S.; Rocha-Santos, T.; Silva, J.; Lobo, J. S.; Costa, P.; Amaral, M.; Pintado, M.; Gomes, A.; Malcata, F. Influence of L-cysteine, Oxygen and Relative Humidity upon Survival Throughout Storage of Probiotic Bacteria in Whey Protein-based Microcapsules. Int. Dairy J. 2011, 21(11), 869–876. DOI: 10.1016/j.idairyj.2011.05.005.
- Yasmin, I.; Saeed, M.; Pasha, I.; Zia, M. A. Development of Whey Protein Concentrate-pectin-alginate Based Delivery System to Improve Survival of B. Longum BL-05 in Simulated Gastrointestinal Conditions. Probiotics Antimicrob. Proteins. 2019, 11(2), 413–426. DOI: 10.1007/s12602-018-9407-x.
- Huq, T.; Fraschini, C.; Khan, A.; Riedl, B.; Bouchard, J.; Lacroix, M. Alginate Based Nanocomposite for Microencapsulation of Probiotic: Effect of Cellulose Nanocrystal (CNC) and Lecithin. Carbohydr. Polym. 2017, 168, 61–69.
- Zeashan, M.; Afzaal, M.; Saeed, F.; Ahmed, A.; Tufail, T.; Ahmed, A.; Anjum, F. M. Survival and Behavior of Free and Encapsulated Probiotic Bacteria under Simulated Human Gastrointestinal and Technological Conditions. Food Sci. Nutr. 2020. DOI: 10.1002/fsn3.1531.