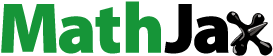
ABSTRACT
Drying kinetics and bioactive quality indices (total phenolics, flavonols, anthocyanins, and antioxidant activity) of fermented Merlot grape, cranberry, highbush blueberry, and wild lowbush blueberry pomace were evaluated. Thin layer drying experiments were performed at two loading densities (kg m−2) at 50, 60, and 70°C in a cabinet convection air dryer. Phenolics composition and antioxidant activity of both cabinet convection air dried pomace and freeze dried pomace were assessed. The effective moisture diffusivity (Deff) and activation energy (Ea) values were calculated for each pomace type subjected to cabinet convection air drying and experimental drying data from these experiments were modeled with the Newton/Lewis, Henderson-Pabis, and Page equations. This work showed that levels of phenolic compounds and antioxidant activity in pomace subjected to cabinet convection air drying at certain conditions were generally comparable to levels in freeze dried pomace. Results indicated that subjecting the berry pomace to shorter processing times upon cabinet convection air drying (half load density at 70°C) results in better bioactive quality retention. Thus this method could be used to generate dried berry pomace which could be a source of bioactive compounds with potential use as a value added product in the food industry and other industries.
Introduction
The efficient use of berry crops at all stages of production, particularly by reducing waste, is important to maximize profitability and ensure sustainable production as berry crops are generally high value and high input crops. Canadian winemakers, as well as producers of cranberry and blueberry juice, have expressed a desire to find quality use for juice by-products. Grapes, botanically classified as berries[Citation1] belong to the genus Vitis and different species and cultivars/varieties exist. Vitis vinifera species includes wine grapes of which Merlot is an example of a red cultivar/variety.[Citation2] For standard Merlot wine processing, Merlot grapes are typically fermented before pressing occurs .[Citation3] Key areas of wine grape production in Canada include: British Columbia, Ontario, Quebec and Nova Scotia .[Citation4] The number one planted grape in BC is Merlot which accounts for 1,585 acres or 641 hectares .[Citation5] Cranberry (Vaccinium macrocarpon) is an economically important crop in Canada with the key production areas in British Columbia and Quebec. The Canadian blueberry industry is made up of two distinct sectors: highbush blueberries (Vaccinium corymbosum), which are often referred to as cultivated blueberries and primarily grown in British Columbia, and lowbush blueberries (Vaccinium angustifolium), which are often referred to as wild blueberries and primarily grown in Quebec and Atlantic Canada .[Citation6]
Pomace is the major by-product of fruit processing operations such as winemaking and juicing, industries producing nearly 38.1 million metric tonnes (Mt) of fruit waste annually.[Citation7] The winemaking process yields about 20% grape pomace based on input berry mass,[Citation8] while cranberry and blueberry juice processing yields ~14-20% pomace.[Citation6,Citation9] Fruit pomaces are generally a good source of carbohydrates and other functionally important bioactive molecules, such as essential amino acids, fatty acids, dietary fibers, vitamins, minerals, and polyphenolics .[Citation10] Phenolics, which include flavonoids (such as anthocyanins and flavonols), stilbenes, tannins, and simple phenolic acids, are a major group of bioactive compounds present in fruits and fruit pomace. These phenolic compounds are of particular importance due to their human health benefits and their application in improvement of flavor, color and stability of food .[Citation8]
As the agriculture and agri-food sector is facing the increasing problem of waste and cost reduction of industrial processes there is opportunity to position pomace with high levels of bioactive compounds as a high value product. Uribe et al. [Citation11,Citation12] have promoted olive waste cake as a source of high value added bioproduct. Reque et al.[Citation13] and Tagliani et al. [Citation9] have positioned blueberry pomace, an industry by-product, as a valuable source of fiber with antioxidant capacity. Work performed by,Das et al. [Citation14] and Islam et al. [Citation15] have shown beneficial effects on intestinal health and immunity of including berry pomace as a broiler chicken feed additive., Juskiewicz et al. [Citation16] reported the effects of dietary dried fruit pomaces on turkey health while,Hassan et al. [Citation2] reviewed studies showing that grape pomace could be a promising feed additive as an antimicrobial alternative to antibiotic in livestock production.
Fruit pomace is a highly perishable material subject to spoilage due to its high moisture content, ~60-70% wet basis .[Citation8] Therefore drying is an important processing step in ensuring product stability in terms of shelf life and maintaining quality for utilization as a value added product. Previously, our work examining the bioactivity of berry pomace and its application as an antibiotic alternative in poultry feed utilized berry pomace that had been freeze dried to remove water and obtain a shelf stable product.[Citation14,Citation15] Freeze drying is an effective drying method as materials undergoing freeze drying are not exposed to high temperatures, minimizing destruction of any bioactive compounds present. Despite the advantages freeze drying offers on product quality, long drying times and high operating costs are significant disadvantages. Therefore freeze drying is not the ideal drying option for processing a cost-competitive product .[Citation8] Convective dehydration is an industrially relevant technology for the production of stabilized materials with maintained quality and reduced costs .[Citation11,Citation17–19]
Mathematical modeling of thin layer drying is required to control the drying process in terms of identifying suitable operating parameters for minimizing drying time and ensuring optimal product quality .[Citation11,Citation20,Citation21] Drying kinetics are generally evaluated experimentally by measuring the weight of a drying material as a function of time and drying curves may be represented by plots of moisture content versus time.[Citation21]
The present study was undertaken to determine the thin-layer drying kinetics of different berry pomace types (fermented Merlot grape, cranberry, highbush blueberry, and wild lowbush blueberry). The effective moisture diffusivity and activation energy for the drying process were calculated and the experimental drying data was fit to a selected model describing the drying curves. Further, an important aspect of this work was to assess both the bioactive quality (phenolics composition and antioxidant activity) of the different pomace types processed under different cabinet convection air drying conditions and the bioactive quality of the same pomace material subjected to freeze drying. The results of this study can be used in the development of industrially relevant value added products from berry pomace.
Materials and methods
Raw material
Four pomace types were examined in this work: fermented Merlot red grape (Vitis vinifera ‘Merlot’); cranberry (Vaccinium macrocarpon), highbush blueberry (Vaccinium corymbosum); and wild lowbush blueberry (Vaccinium angustifolium). The fermented Merlot grape pomace was collected from Township 7 Winery, Penticton, BC, September, 2018. The cranberry pomace and highbush blueberry pomace were received from Pacific Coast Fruit Products Ltd, Abbotsford, BC, March 2018. The wild lowbush blueberry pomace, which will be referred to lowbush blueberry pomace hereafter was obtained from Van Dyk’s Health Juice Products Ltd., Caledonia, NS, March 2019. All pomace materials were obtained from conventional production systems and all pomace types were frozen and stored at −20°C until subjected to drying experiments.
Freeze dried material
For each pomace type, subsamples were taken and dried in a freeze drier (Virtis, SP Industries, Warminster, PA). Pomace was dried at 150 mTorr vacuum at 25°C until dried. Freeze dried samples were milled for particle size reduction using an IKA mill (IKA Works, Inc., Wilmington, NC, USA) with a cutting mill attachment (2 mm screen). After drying and milling, all samples were packed in low-density polyethylene bags, sealed and stored at −20°C until analysis. These freeze dried samples were used to compare the effect of air drying treatments on phenolics and antioxidant activity.
Experimental cabinet convection air drying conditions
The drying behavior of the four different pomace types (fermented Merlot red grape, cranberry, high bush blueberry, and low bush wild blueberry) under different thin layer drying conditions (air drying temperatures and load density/bed thicknesses) were studied. The four different pomace types (fermented Merlot red grape, cranberry, highbush blueberry, and lowbush blueberry) were dried in a cabinet convection dryer (D-20 Commercial Food Dehydrator, TSM Products, Buffalo, NY, USA). The high speed fan setting was used. This corresponded to an air velocity of 0.366 ms−1 as determined with a thermo-anemometer probe (Model 275, Alnor, TSI Incorporated, Shoreview, MN). The air drying temperatures tested were 50, 60 and 70°C. The average relative humidity measured in the drying oven was low and ranged from 0–5% depending on drying conditions.
For the convection cabinet dryer experiments, water loss from the samples was determined by weighing the sample tray outside the drying chamber at 15 min intervals up to 180 min during the drying process. After 180 min, the samples were weighed at 30 min intervals. The drying experiment was stopped when mass remained constant for at least two readings and final moisture content (kg water kg−1 dry matter) was determined at this time point. All drying experiments were replicated four times. Dried samples were milled for particle size reduction using an Ika mill (IKA Works, Inc., Wilmington, NC, USA) with a cutting mill attachment (2 mm screen). After drying and milling all samples were packed in low-density polyethylene bags, sealed and stored at −20°C until analysis.
For completeness the bulk density, which equals the mass of particles in a material divided by the total volume occupied, of the pomace material before drying was determined. Poured bulk density, was measured by pouring material into a known volume, which creates a relatively loose structure, andthe mass was determined Also, tapped bulk density which in theory is the maximum bulk density that can be achieved without deformation of the particles, was determined. In practice, it is generally unrealistic to attain theoretical tapped bulk density, thus a method of tapping the sample in a standard experimental manner is typically used.[Citation22] Therefore this work obtained experimental measures of 1) poured bulk density and 2) tapped bulk density.
For poured bulk density determination, raw pomace at room temperature was poured into a 25 mL graduated cylinder and the mass was measured. For tapped bulk density, the pomace in the 25 ml graduated cylinder from the poured bulk density measurements was tapped with a 1 cm diameter glass rod 10 times and the compressed volume was determined. This procedure used pressure comparable to that used for preparing pomace in the trays for the drying experiments.
For the drying experiments, the pomace material was placed in the drying tray at a nominal load density/thickness of half-full or full. The length, width, and height/depth dimensions of the tray were 45.4, 52.4 and 0.8 cm, respectively. For the fermented Merlot grape pomace samples, the corresponding masses associated with the different filling conditions were 0.5 and 1 kg for half and full load density/thickness, respectively which corresponded to 2.1 and 4.2 kg m−2 load densities, respectively. For the cranberry pomace samples, the corresponding masses associated with the different filling conditions were 1 and 2 kg for half and full load density/thickness, respectively which corresponded to 4.2 and 8.4 kg m−2 load densities, respectively. For the highbush blueberry pomace samples, the corresponding masses associated with different filling conditions were 0.73 and 1.46 kg for half and full load density/thickness, respectively which corresponded to 3.06 and 6.14 kg m−2 load densities, respectively. For the lowbush blueberry pomace the corresponding masses associated with the different filling conditions were 0.75 and 1.5 kg for half and full load density/thickness which corresponded to 3.15 and 6.30 kg m−2 load densities, respectively. Actual pomace bed thickness was determined with a digital caliper (Mitutoyo, Aurora, IL, USA). The caliper was placed in the tray at 9 points in a 3 × 3 row configuration to calculate an average depth across the whole tray. The fermented Merlot grape pomace was essentially whole berries and the thickness levels for half and full load density were 5.3 and 8.1 mm, respectively. The cranberry, highbush blueberry and low bush blueberry pomace possessed broken berry pieces and the thickness levels for half load density were 3.99, 3.39, and 3.82 mm, respectively and the thickness levels for full load density were 8.32, 7.2, and 7.0 mm, respectively. As the pomace material was manually packed into the drying tray at thickness levels of half full and full, values were not exactly 4 and 8 mm, respectively.
Drying data analysis: theoretical considerations and modeling of drying data
There are important theoretical considerations with respect to drying kinetics analysis. Diffusion is noted to be the key mechanism during moisture transport and Fick’s second law of diffusion describes drying kinetics during the falling rate period .[Citation18] Drying of biological materials mostly occurs in the falling rate period and moisture transfer during drying is controlled by internal diffusion [Citation18]. Crank [Citation23] proposed a solution for determining the effective moisture diffusivity of an infinite slab using Fick’s second law and simplifying assumptions. These simplifying assumptions include: 1) moisture is initially distributed uniformly throughout the mass of a sample; 2) mass transfer is symmetric with respect to the center of the sample; 3) surface moisture content of the sample instantaneously reaches equilibrium with the condition of surrounding air; 4) resistance to the mass transfer at the surface is negligible compared to internal resistance of the sample; 5) mass transfer is by diffusion only; and 6) diffusion coefficient is constant and shrinkage is negligible.[Citation23,Citation24] The solution simplified for long drying times is:
where MR is the moisture ratio (dimensionless); Mt, M0 and Me are moisture content at time t of drying, initial moisture content and equilibrium moisture content (kg water/kg dry matter), respectively; t is the drying time (s), Deff is the effective diffusivity (m2s−1) and L is the half-slab thickness (half bed thickness, (m)). If drying occurs at only one surface of the slab, the thickness L in Equationequation 1a(1a)
(1a) must be substituted by 2 L,[Citation24] which was the case for these experiments. Moreover, the values of Me are relatively small compared to Mt and M0, therefore the error involved in the simplification is negligible and the moisture ratio can be simplified to MR = Mt/M0. Therefore:
Subsequently:
The effective moisture diffusivity can be calculated through EquationEquation 1c(1c)
(1c) by using the method of slopes. The effective diffusivities are typically determined by plotting experimental drying data in terms of ln (MR) versus time yielding EquationEquation 2
(Equation 2)
(Equation 2) .
Therefore a plot of ln MR versus time gives a straight line with a slope of: (π2⋅Deff)/(4L2) and the values of Deff at different temperatures can be obtained. The dependence of the Deff on the temperature is generally described by the Arrhenius equation (EquationEquation 3(3)
(3) ) [Citation18]:
Here, D0 is the pre-exponential factor of the Arrhenius equation in m2s−1, Ea is the activation energy in kJmol−1, R is the universal gas constant in kJmol−1·K−1 and T is drying temperature in °K. The activation energy can be determined from the method of slopes of the Arrhenius plot.
The slope of the line is (-Ea/R) and the intercept equals ln(D0). Experimental moisture ratio values versus drying time were fitted by means of three models widely used to model the drying kinetics of most foods, namely Newton/Lewis (EquationEquation 5a(5a)
(5a) ), Henderson-Pabis (EquationEquation 6a
(6a)
(6a) ), and Page (EquationEquation 7a
(7a)
(7a) ) .[Citation25]
The Newton/Lewis model was further linearized as:
The Henderson-Pabis model was further linearized as:
The Page model was further linearized as:
Where: a is Henderson-Pabis model coefficient (dimensionless constant), n is Page model coefficient (dimensionless constant), k is drying constant (s−1); t is time (s)
Statistical evaluation of the drying models
The literature notes several statistical test methods used to evaluate the performance of the models in fitting drying data. The coefficient of determination (R2), reduced chi-square (χ2) and root means square error (RMSE) are the most widely used [Citation18]. In accordance with,[Citation18] the best model describing thin layer drying of fruit pomace was chosen based on maximizing R2 and minimizing χ2 and RMSE values. The χ2 and RMSE values can be calculated as follows:
Where: MRexp,j and MRpre,i are experimental and predicted moisture ratios, respectively; N is the number of observations; and z is the number of constants in the model.
Proximate composition
Initial moisture content of the different raw pomace types was determined using the method described by [Citation6]. Briefly, ~10-15 g pomace was placed into aluminum weighing dishes and placed in a vacuum oven at 70°C for 24 h. Freeze dried pomace was used to determine protein content, ash content, and lipid content, along with soluble dietary fiber and insoluble dietary fiber content of the initial pomace material using AOAC methods of analysis, AOAC 992.15, 923.03, 922.06, and 991.43, respectively [Citation26] The carbohydrate content and calorie content of the pomace materials was determined by difference. These analyses were performed in duplicate. Proximate composition was assessed only on initial pomace material that had been freeze dried using methods described by Ross et al. [Citation6] as the main scope of this work was to examine the effect of cabinet convection air drying conditions, in comparison to freeze drying, on bioactives content of berry pomace in terms of phenolics composition and antioxidant activity.
Phenolics composition
Total phenolics content of the freeze and air dried pomace material was assessed using the Folin Ciocalteu method while flavonol and anthocyanin levels were assessed using Glories method as described by Ross et al. [Citation6]. Extracts used for testing were obtained using a procedure based on the work of, Velioglu et al. [Citation27] as described by Ross et al. [Citation6] Briefly, an aliquot of 1.0 g sample (dried milled berry pomace) was weighed into a centrifuge tube and 20 mL of 70% MeOH was added to the tube [1: 20 ratio). Tubes were shaken for 30 minutes on an orbital shaker. The tubes were then centrifuged at ~10000 x g for 10 minutes. The resulting supernatant was vacuum filtered through Whatman No. 1 paper and collected. Extracts were stored at −20°C until analyzed
Measurement of total phenolics using the Folin-Ciocalteu colorimetric method was based on the procedure by Singleton and Rossi [Citation28], as described by Ross et al. [Citation6]. Quantification was determined using a standard curve for gallic acid in 70% methanol and measuring absorbance at 765 nm on a spectrophotometer [Cary 60, Agilent Technologies, Mississauga, ON]. Extracts were diluted with 70% methanol so values were within the standard curve range of 0–300 mg/L. The total phenolic content was expressed as mg gallic acid equivalent per 100 gram sample on a dry matter basis). This analysis was performed on the 4 replicates for each treatment.
Measurement of flavonols and anthocyanins using the Glories method was performed using a procedure based on the method of Harrison et al. [Citation29], as described by Ross et al. [Citation6]. Quercetin in methanol was used as the standard for quantification of flavonols in all pomace types. Cyanidin-3-glucoside in 70% methanol was used as the standard for quantification of anthocyanins in the cranberry and blueberry pomaces while malvidin-3-glucoside in 70% methanol was used as the standard for quantification of anthocyanins in the grape pomace. Quantification was determined using a standard curve for each standard and measuring absorbance at 360 and 520 nm on a spectrophotometer (Cary 60, Agilent Technologies, Mississauga, ON] for determination of flavonols and anthocyanins, respectively. The total amount of phenolic content was expressed as mg standard equivalent per 100 gram sample on a dry matter basis. These analyses were performed on the 4 replicates for each treatment.
Antioxidant activity
The antioxidant activity of the samples was investigated by the ABTS•+ radical scavenging assay and the Ferric Reducing Antioxidant Power [FRAP) assay as described by Ross et al. [Citation6]. Both of these assays used the 70% methanol extract samples obtained for determining phenolics. Extracts were diluted with 70% methanol to be within the standard curve range of the Trolox standard. For assessing ABTS•+ radical scavenging activity, absorbance at 734 nm was measured using a plate reader (SpectraMax M2, Molecular Devices, Sunnyvale, CA]. The results were expressed as μmol Trolox equivalents (TE) per 100 g sample on a dry matter basis. For (FRAP), absorbance at 593 nm was measured using a plate reader (SpectraMax M2, Molecular Devices, Sunnyvale, CA). The results were expressed as μmol Trolox equivalent (TE) per 100 g sample on a dry matter basis. These analyses were performed on the 4 replicates for each treatment.
Statistical analysis of data
The data, obtained from experimental replicates, were determined as means ± standard deviation. Further statistical analysis was conducted using SAS Institute Inc. Software, version 9.3 (SAS Institute, Cary, NC, USA. 2010). Data were subjected to analysis of variance (ANOVA) with replication using the SAS PROC GLM procedure. Least square (LS) means followed by Least Significant Difference (LSD) Fisher’s test at 5% significance level. were generated using the SAS PROC GLM procedure.
Results and discussion
Drying characteristics of pomace
For the drying experiments, load densities varied between the different pomace types due to their different density values. The poured bulk density values for the fermented Merlot grape, cranberry, highbush blueberry, and lowbush blueberry pomace were 380, 540, 520, and 510 kg m−3, respectively. For poured bulk density values, only the fermented Merlot pomace had a value significantly lower (p ≤ 0.05) than the other pomace types. The tapped bulk density values for the fermented Merlot grape, cranberry, highbush blueberry, and lowbush blueberry pomace were 740, 980, 850, and 900 kg m−3, respectively. For the tapped bulk density, the highbush blueberry and lowbush blueberry pomace samples did not have significantly different values (p ≤ 0.05). The cranberry pomace showed the highest tapped density while the fermented Merlot grape pomace showed the lowest bulk density (p ≤ 0.05). These results are reflected in the load density values and indicate why the fermented Merlot grape pomace had the lowest load density values at each fill condition, the cranberry pomace had the highest load density values at each fill condition, and the highbush and lowbush blueberry pomace had comparable load density values at each fill condition.
The initial moisture contents (wet basis) of the different pomace types were: 47.55% for the fermented Merlot grape pomace, 71.53% for the cranberry pomace, 53.6% for the highbush blueberry pomace, and 57.91% for the lowbush blueberry pomace (). also provides a summary of the proximate composition of the different pomace types, which was determined on freeze dried pomace to provide baseline information on the starting material. These results are presented on a dry matter basis. The fermented Merlot grape pomace showed the highest protein content (11.02 g 100 g−1) while the cranberry pomace showed the lowest protein content (4.55 g 100 g−1). Ash content was remarkably higher in the fermented Merlot grape pomace (7.9 g 100 g−1) compared to other pomace types (0.7–1.15 g 100 g−1). The high ash value observed in the fermented Merlot grape pomace was likely due to crystallization and precipitation of tartrates that occurs during the winemaking process.[Citation30] Soluble fiber content was nearly two times higher in the lowbush blueberry pomace (8.2 g 100 g−1) compared to the other pomace types (3.4–5.3 g 100 g−1). Insoluble fiber was lowest in the fermented Merlot grape pomace (49.1 g 100 g−1) compared to the other pomace types (52.2–57.7 g 100 g−1).
Table 1. Proximate composition of freeze dried Merlot grape, cranberry, highbush blueberry and wild lowbush blueberry pomaces
The changes in the experimental moisture ratio as a function of time for the various pomace types (fermented Merlot grape, cranberry, highbush blueberry, lowbush blueberry) at different air drying temperatures (50, 60, and 70°C) are presented in . The overall rate of drying was calculated as described by Shalini et al. [Citation19], using data from , as ratio of difference of initial and final moisture content and total drying time. Values reported are based on the average of the four drying experiments. As shown in , the fermented Merlot grape pomace samples dried at 50, 60 and 70°C and half load density exhibited: final moisture contents of 0.017, 0.017 and 0.017 kg water kg−1 dry matter basis (DM), respectively; drying times of 420, 270 and 180 min, respectively; and drying rates of 0.002, 0.003, and 0.005 kg water kg−1 DM min−1. Also shown in , the fermented Merlot grape pomace samples dried at 50, 60 and 70°C and full load density exhibited: final moisture contents of 0.054, 0.016 and 0.017 kg water kg−1 DM, respectively; drying times of 1395, 330 and 270 min, respectively; and drying rates of 0.0006, 0.003, and 0.003 kg water kg−1 DM min−1, respectively.
Figure 1. Experimental and predicted (Page model) moisture ratio of fermented Merlot grape pomace dried at 50, 60, and 70°C at half and full load density conditions
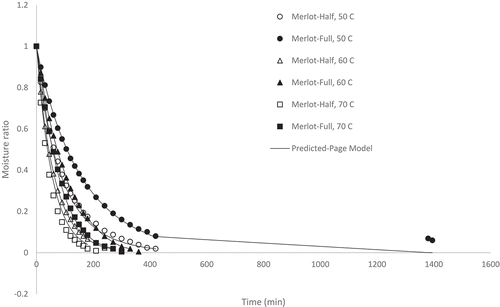
Figure 2. Experimental and predicted (Page model) moisture ratio of cranberry pomace dried at 50, 60, and 70°C at half and full load density conditions
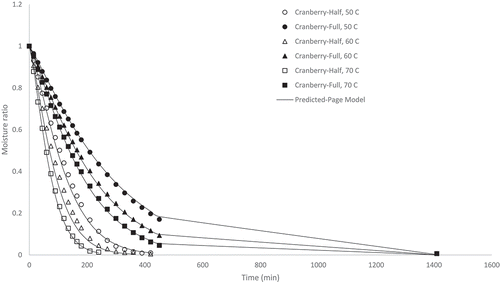
Figure 3. Experimental and predicted (Page model) moisture ratio of highbush blueberry pomace dried at 50, 60, and 70°C at half and full load density conditions
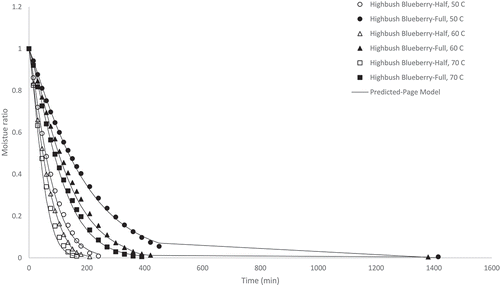
Figure 4. Experimental and predicted (Page model) moisture ratio of wild low bush blueberry pomace dried at 50, 60, and 70°C at half and full load density conditions
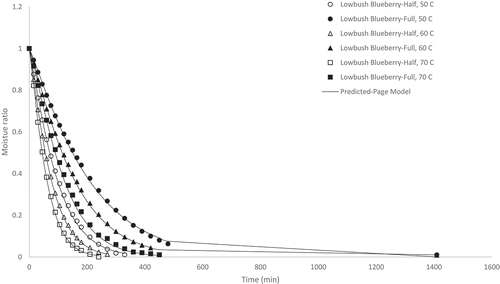
The cranberry pomace samples dried at half load density at 50, 60 and 70°C possessed final moisture contents of 0.023, 0.033, and 0.033 kg water kg−1 DM; drying times of 420, 360, and 240 min, respectively; and drying rates of 0.006, 0.007, and 0.01 kg water kg−1 DM min−1 (). Also seen in , the cranberry pomace samples dried at 50, 60 and 70°C and full load density exhibited: final moisture contents of 0.015, 0.015 and 0.015 kg water kg−1 DM, respectively; drying times of 1410, 1410 and 1410 min, respectively; and drying rates of 0.002, 0.002, and 0.002 kg water kg−1 DM min−1, respectively.
As shown in , the highbush blueberry pomace samples at 50, 60 and 70°C and half load density exhibited: final moisture contents of 0.01, 0.007 and 0.01 kg water kg−1 DM, respectively; drying times of 240, 210 and 165 min, respectively; and drying rates of 0.005, 0.006, and 0.007 kg water kg−1 DM min−1, respectively. Also seen in , the highbush blueberry pomace samples at 50, 60 and 70°C and full load density exhibited: final moisture contents of 0.006, 0.005 and 0.007 kg water kg−1 DM, respectively; drying times of 1410, 1380 and 390 min, respectively; and drying rates of 0.0008, 0.0008 and 0.003 kg water kg−1 DM min−1, respectively.
The lowbush blueberry pomace samples dried at 50, 60 and 70°C and half load density possessed final moisture contents of 0.014, 0.018 and 0.007 kg water kg−1 DM, drying times of 330, 270 and 240 min, respectively; and drying rates of 0.004, 0.005 and 0.006 kg water kg−1 DM min−1, respectively (). Also shown in , the lowbush blueberry pomace samples dried at 50, 60 and 70°C and full load density exhibited final moisture contents of 0.0014, 0.012 and 0.012 kg water kg−1 DM, respectively; drying times of 1410, 1410 and 450 min, respectively; and drying rates of 0.001, 0.001 and 0.003 kg water kg−1 DM min−1, respectively.
Overall, the results from indicate that drying took place during the falling rate period as the moisture ratio decreased continuously with temperature following a decreasing exponential pattern .[Citation17] This was expected for an agricultural material [Citation24] such as pomace. [Citation31] Generally, drying time was a function of air drying temperature and load density with drying conditions of half load density and higher temperature showing shorter drying times/higher drying rates. The effect of temperature on drying time was expected as it is well known that the energy of water molecules is increased at higher temperatures and evaporation occurs more quickly.[Citation32] Within each pomace type, material with lower load densities/thickness levels dried faster due to the reduced distance the moisture needed to migrate and the increased surface area exposed for the given volume of the material.[Citation18] However, it should be noted that the cranberry pomace dried at the full load density condition did not show a shorter drying time at higher drying temperature unlike the other pomace types which all exhibited the shortest drying time when dried at 70°C, although the moisture ratio during the first 8 hours of drying was lower at higher temperatures as expected. The cranberry pomace exhibited the highest tapped density and consequently the highest load density tested during these drying experiments, which required longer drying times. Due to experimental constraints, mass measurements could only be taken in intervals during the eight hour work day. The longer drying times of 1380 and 1410 min noted in this work represents a 23 and 23.5 h drying time, respectively. It is possible that equilibrium moisture content and the drying endpoint was reached at a time between 8 and 23.5 h. If mass measurements were taken in intervals between 8 and 23.5 h it is likely shorter drying times at higher temperatures would have been detected. Nevertheless, these results indicate that the cranberry pomace required longer drying time compared to the other pomace types at full load density and 70°C temperature. The drying behavior of the highbush blueberry and lowbush blueberry were comparable, which was not unexpected due to their similar load densities.
Evaluation of mathematical models representing drying curves
Several mathematical models including the Lewis-Newton, Henderson-Pabis, and Page were used to evaluate the best model for representing experimental fruit pomace drying data. These are semi-theoretical models derived from Fick’s second law of diffusion. Mathematical consideration of drying kinetics helps to ensure efficiency and cost effectiveness of drying processes and promotes product quality.[Citation33] shows statistical test values (R2, RMSE and χ2) for the three selected models. It was determined that the Page model gave a better fit to experimental data with highest R2 > 0.993–0.999, lowest RMSE < 0.00032 and lowest χ2 < 0.014, depending on pomace type, load, and drying temperature; therefore, the Page model provided a satisfactory description of the drying characteristics of fruit pomace for a whole range of drying temperatures, pomace types and drying load densities. This result is in agreement with other work examining the drying characteristics of biological material such as okra, eggplant slices, carrot pomace, and papaya slices [Citation17,Citation18,Citation31,Citation32] which found the Page model best described experimental data. For the Page model, the calculated k values ranged from 0.0000119 to 0.005 s−1 and the n values ranged from 0.884 to 1.357 depending on pomace type, load and drying temperature. shows the experimental and predicted (Page model) moisture ratio of fruit pomace types at half load density and full drying load density drying conditions.
Table 2. Kinetic parameters of drying models as affected by pomace type, load density, and temperature
Drying kinetic analysis: effective moisture diffusivity and activation energy
As shown in , within pomace type, effective moisture diffusivity (Deff) values increased with a drying temperature increase between 50 and 70°C [p ≤ 0.05) which was in agreement with the work of [Citation31]. Generally within pomace type, Deff values were higher at higher load densities (p ≤ 0.05], which was in agreement with the work of Sairam [Citation32]. In this work, Deff values ranged from 2.43 to 14.0 × 10−10 m2 s−1 depending on pomace type, load density, and drying temperature. These Deff values are in the general range of 10 −11 to 10−9 m2 s−1 reported for food materials.[Citation17] Some Deff values noted in the literature for thin layer drying include: ~5 x 10−10 to 2.4 × 10−9 for tomatoes,[Citation25] ~0.9 x 10−10 to 9 × 10−10 for eggplant,[Citation18] ~ 4 × 10−10 to 1.3 × 10−9 for okra,[Citation17] ~ 2.7 × 10−9 to 4.6 × 10−9 for carrot pomace,[Citation31] and ~ 6.6 × 10−10 to 13.9 × 10−10 for olive pomace.[Citation34] Differences between Deff values for the different pomace types at common drying conditions were examined. There were no differences in Deff values for pomaces dried at half load density and 50 and 60°C. However, the fermented Merlot pomace showed the highest Deff values when dried at half load density and 70°C. For the pomaces dried at full load, cranberry pomace showed the lowest Deff values at all drying temperatures. This result is in agreement with the longer drying times associated with the cranberry pomace dried at full load density at each drying temperature. The fermented Merlot grape pomace showed the highest Deff values when dried at full load density at 60 and 70°C but showed values comparable to the highbush and lowbush blueberry pomaces when dried at 50°C. It is possible that the lower tapped density value of the fermented Merlot pomace allowed for higher Deff values at the higher drying temperatures at full load density drying condition.
Table 3. Effective moisture coefficient and activation energy as affected by pomace type, load density, and temperature
The influence of temperature on effective moisture diffusivity is described using an Arrhenius type relationship. The activation energy (Ea) is the energy barrier that must be overcome to activate moisture diffusion and the diffusivity constant (Do) equivalent to the diffusivity at infinitely high temperature. [Citation11] Thus, for this work the natural logarithm of Deff as a function of the reciprocal of the absolute temperature shows a linear relationship or an Arrhenius type relationship with R2 ranging from 0.821 to 0.998 depending on pomace type, load, and drying temperature. Values of D0 and Ea calculated from the linear regression ranged from 3.0 × 10−7 m2 s−1 to 0.0033 and 18.69 to 48.22 kJ mol−1, respectively depending on pomace type and loading level. The Ea values are in agreement with those reported in the literature for biological materials; which include: ~23 kg mol−1 for carrot pomace[Citation31] and ~26-38 kg mol−1 for olive pomace.[Citation35] Only the highbush blueberry and lowbush blueberry pomaces showed an increase in Ea with an increase in load density. It has been shown in the literature that Ea typically increases as load density increases.[Citation32,Citation35] Load density did not affect the Ea of the fermented Merlot grape pomace and the cranberry pomaces, which was unexpected but may be explained by the fact that the diffusion model assumes that transport occurs in one direction from the inside to the outer surface of the product, which is an assumption that is valid for thin layer. However, for thicker layers it is possible that some side diffusion may occur, which might potentially improve moisture removal from the product.[Citation35] It is possible that the full load density conditions of the thicker bed levels of the fermented Merlot grape pomace (8.1 mm) and cranberry pomace [8.3 mm) promoted diffusion and made the process less affected by drying temperature and subsequently Ea was not impacted by load density as for the highbush blueberry and wild lowbush blueberry with slightly lower bed thickness values, 6.36 and 7.00 mm, respectively. The fermented Merlot grape pomace also showed the highest Ea values which may have been a result of its physical structure. The grape pomace was comprised of whole grape berries while the other pomace types were comprised of broken berry pieces; the intact skin of the grape pomace may have impeded further moisture removal and resulted in higher Ea values.
Phenolic compounds and antioxidant activity
A key aspect of this work was to assess the phenolics composition and antioxidant activity of the different pomace types processed under different cabinet convection air drying conditions and the bioactive quality of the same pomace material subjected to freeze drying. Statistical analysis, ANOVA followed by Fisher’s LSD test, provided an indication of differences between samples. There was variation within sample replicates which understandably impacted significant difference results. The LSD test was an appropriate multiple comparison post-hoc test as opposed to Dunnnet’s test as the goal was to compare levels of phenolic compounds and antioxidant activity in cabinet convection air dried samples and freeze dried samples. The work,Citation36compared the physicochemical and nutraceutical properties of tomato powder processed with air drying and freeze drying and used comparable statistical analysis.
shows the phenolic content and antioxidant activity levels of fermented Merlot grape pomace dried under different conditions. For the fermented Merlot grape pomace (), freeze dried pomace exhibited total phenolics levels that were not significantly different than the pomace dried at 70°C at the half and full load density drying conditions (p ≤ 0.05). Levels of total phenolics ranged from ~3421-4236 mg gallic acid equivalents (eq.) 100 g−1 dry matter (DM). These values are in agreement with those reported in the literature for Merlot grape skins, 2644 mg gallic acid eq. 100 g−1 DM.[Citation37] For flavonols drying type and condition had no effect on levels in the fermented Merlot grape pomace (p ≤ 0.05), which ranged from ~110-130 mg quercetin eq. 100 g−1 DM.
Table 4. Fermented Merlot grape pomace phenolic content and antioxidant activity: Comparison of freeze drying and cabinet convection air drying methods
For anthocyanins, freeze dried fermented Merlot pomace showed significantly (p ≤ 0.05) higher levels (~295 mg malvidin 3-glucoside eq. 100 g−1 DM) than fermented Merlot pomace treated at all cabinet convection air drying conditions, which showed comparable levels ~166-189 mg malvidin 3-glucoside eq. 100 g−1 DM. A value of 243 mg malvidin 3-glucoside eq. 100 g−1 DM has been reported in the literature for Merlot grape skins .[Citation37] For antioxidant levels (assessed with ABTS), freeze dried fermented Merlot grape pomace showed the highest levels (~31221 µmol Trolox Equivalent (TE) 100 g−1 DM). Fermented Merlot grape pomace dried at the half load density at 50, 60, and 70°C showed higher antioxidant levels than pomace treated at the full load density and 60°C drying condition (p ≤ 0.05). For antioxidant levels (assessed with FRAP), freeze dried Merlot grape pomace again showed the highest levels (~20192 µmol TE 100 g−1 DM).
shows that for the cranberry pomace there was no effect of drying type or conditions on total phenolics content, flavonol levels and antioxidant activity levels (p ≤ 0.05). Total phenolics values were reported to be ~1355-1517 mg gallic acid eq. 100 g−1 DM. Flavonol levels were reported to be ~286-303 mg quercetin eq. 100 g−1 DM. Antioxidant activity levels were ~8933-9610 µmol TE 100 g−1 DM (ABTS) and ~6717-7080 µmol TE 100 g−1 DM (FRAP).
Table 5. Cranberry pomace phenolic content and antioxidant activity: Comparison of freeze drying and cabinet convection air drying methods
For anthocyanins content, only the cranberry pomace dried at the full load density and 70°C drying condition (~107 mg cyanidin-3-glucoside eq. 100 g−1 DM) showed lower levels (p ≤ 0.05) than the freeze dried cranberry pomace samples [~147 mg cyanidin-3-glucoside eq. 100 g−1 DM). All other cranberry samples dried in the cabinet convection air drying at other conditions showed comparable levels ~111-120 mg cyanidin-3-glucoside eq. 100 g−1 DM. Overall the total phenolics, flavonols, anthocyanins, and antioxidant activity levels reported for the cranberry pomace were only slightly lower than reported by Ross et al. [Citation6], yet values were lower. These differences were likely due to environmental and growing location effects. The cranberry pomace studied in our current work was sourced from British Columbia while the pomace studied in the work of Ross et al. [Citation6], was sourced from Quebec. The variation in total phenolics, flavonols, anthocyanins, and antioxidant activity levels were not unexpected as production area and climate have been reported as variables affecting the phenolic composition of fruit [Citation38]. Further the pomace examined in the work of Ross et al. [Citation6], originated from berries that were produced organically. It has been reported that organically grown fruit can show higher levels of sugars, total phenolics, total anthocyanins, and antioxidant activity than fruit grown conventionally/non-organically.[Citation39]
For the highbush blueberry pomace (), drying type and condition had no effect on total phenolics content (p ≤ 0.5) which were reported to be ~1835-2052 mg gallic acid eq. 100 g−1 DM. These values are in agreement with the total phenolics levels reported by [Citation40], for highbush blueberry pomace (~2880-3800 mg chlorogenic acid eq/100 g DM (converted from fresh basis assuming 15% dry matter]. For flavonols, high bush blueberry pomace dried at the full load density and 70°C drying condition showed significantly lower levels (~376 mg quercetin eq. 100 g−1 DM) than the freeze dried high bush blueberry pomace (~421 mg quercetin eq. 100 g−1 DM). High bush blueberry pomace treated at all other cabinet convection air drying conditions showed comparable levels (392–426 mg quercetin eq. 100 g−1 DM).
Table 6. Highbush blueberry pomace phenolic content and antioxidant activity: Comparison of freeze drying and cabinet convection air drying methods
For anthocyanins, highbush blueberry pomace dried at half load density at 50°C drying condition (~1360 mg cyanidin-3-glucoside eq. 100 g−1 DM) showed similar levels (p ≤ 0.05) to the freeze dried highbush blueberry samples [1412 mg cyanidin-3-glucoside eq. 100 g−1 DM). These values are in agreement with the anthocyanin levels reported by [Citation40], for highbush blueberry pomace (~1266-1513 mg cyaniding-3-glucoside eq/100 g DM (converted from fresh basis assuming 15% dry matter]. All other highbush blueberry pomace samples dried with cabinet convection air drying, except the highbush blueberry pomace dried at half load and 50°C, showed comparable levels of anthocyanins which were lower than anthocyanin levels in the freeze dried highbush blueberry pomace, with the lowest values for the full load at higher temperature. For antioxidant activity levels, highbush blueberry pomace treated at all cabinet convection air drying conditions, except for full load at 70°C, showed similar levels to the freeze dried samples (p ≤ 0.05) which were reported to be ~13594-14628 µmol TE 100 g−1 DM. (ABTS) and ~ 11774–12629 µmol TE 100 g−1 DM (FRAP). Antioxidant values were not affected by cabinet convection air drying conditions, with the exception of full load at the highest temperature.
For the lowbush blueberry pomace (), drying type and condition had no effect on total phenolics content (p ≤ 0.05) with values ranging from ~ 1776–2082 mg gallic acid eq. 100 g−1 DM. These values are in the same order of magnitude as those reported by Ross et al. [Citation6] which reported values ~3113 mg gallic acid eq. 100 g−1 DM. Again these differences may be due to geographical and environmental effects[Citation38] as the lowbush blueberry pomace studied in our current work was sourced from Nova Scotia while the lowbush blueberry pomace studied in the work of Ross et al. [Citation6], was sourced from Quebec.
Table 7. Wild lowbush blueberry pomace phenolic content and antioxidant activity: Comparison of freeze drying and cabinet convection air drying methods
Lowbush blueberry pomace dried at the full load density regardless of drying temperature showed lower flavonol levels (p ≤ 0.05, ~275-284 mg gallic acid eq. 100 g−1 DM) compared to the freeze dried pomace while lowbush blueberry pomace treated at the half load density and 50, 60 and 70°C cabinet convection air drying conditions showed flavonol levels similar to freeze dried low bush wild blueberry pomace (p ≤ 0.05 ~ 309–335 mg quercetin/100 g DM).
For anthocyanins, lowbush blueberry pomace treated with freeze drying and half load density at 70°C exhibited the highest levels (p ≤ 0.05, ~1513-1587 mg cyanidin-3-glucoside eq. 100 g−1 DM) while pomace dried at full load density showed the lowest levels (p ≤ 0.05, ~1135-1317 mg cyanidin-3-glucoside eq. 100 g−1 DM).
For antioxidant activity levels, assessed with the ABTS method, freeze dried lowbush blueberry pomace and lowbush blueberry pomace treated at the half load density and at 50, 60 and 70°C cabinet convection air drying conditions showed the highest levels (p ≤ 0.05, ~13489-14829 µmol TE 100 g−1 DM). For antioxidant activity levels assessed with FRAP, freeze dried lowbush blueberry pomace (~12611 µmol TE 100 g−1 DM) and lowbush blueberry pomace treated at the half load density at 70°C (~12051 µmol TE 100 g−1 DM) showed comparable levels (p ≤ 0.05).
In summary with respect to cabinet convection air drying, total phenolics levels for each pomace type was comparable to levels observed in corresponding freeze dried pomace although levels measured in fermented Merlot grape pomace were statistically lower. Flavonol levels in fermented Merlot grape pomace and cranberry pomace were not affected by cabinet convection air drying in comparison to levels measured in corresponding freeze dried pomace. Only the high bush blueberry pomace dried at full load density at 70°C and lowbush blueberry pomaces dried at full load density at all temperatures showed lower flavonol levels compared to corresponding freeze dried pomace.
Anthocyanin levels were all significantly lower in cabinet convection air dried pomaces compared to freeze dried samples. For the cranberry pomace though, the anthocyanin level was only significantly lower with drying at full load density at 70°C compared to freeze dried pomace. For antioxidant activity levels, only cabinet air convection drying of cranberry was not significantly different than freeze dried sample.
Overall, for all pomace types and air drying temperatures, flavonols, anthocyanins and antioxidant activity were higher or similar for half load than full load trials. This trend was more apparent at higher temperatures and for low bush wild blueberry. Drying temperature (50, 60 and 70°C) generally did not result in significant differences in flavonols, anthocyanins and antioxidant activity at half load in all pomace types. Considering drying time decreases with higher temperatures, air drying at 70°C and half load density would allow efficient drying while maintaining phenolics and antioxidant activity.
Finally, as pomace is a by-product of fruit processing operations, it is likely that the fruit material had been exposed to oxygen and light which is known to degrade phenolics compounds and lower antioxidant activity levels[Citation41] during and post-processing. Initial phenolic retention may be maximized by incorporating cabinet air drying at the fruit processing location and subjecting the fruit pomace to shorter processing times, as this may further offset losses of bioactives in comparison to freeze drying, which often must happen at a central location.
Conclusion
Drying kinetics and bioactive quality indices (levels of total phenolics, flavonols, anthocyanins, and antioxidant activity) of fermented Merlot grape, cranberry, high bush blueberry, and lowbush blueberry pomace were evaluated. Thin layer drying of berry pomaces at 50, 60, and 70°C followed falling rate period for each load density examined. Drying time decreased with temperature and increased with load density. The effective moisture diffusivity (Deff) generally increased with drying temperature and load density. Deff values ranged from 2.43 to 14.0 × 10−10 m2 s−1 depending of pomace type, load density, and drying temperature. The activation energy (Ea) was calculated for each pomace type and ranged from 18.69 to 48.22 kJ mol−1 depending on pomace type and loading level. Out of the mathematical models tested, the Page model fit the experimental data the best. In terms of maintaining levels of phenolic compounds and antioxidant activity upon cabinet convection air drying, this work showed that levels of phenolic compounds and antioxidant activity in pomace subjected to cabinet convection air drying were generally comparable to levels in freeze dried pomace. Specifically, phenolics and flavonols were well preserved across all pomace types, particularly at the half load density. Antioxidants followed a similar pattern, except in the fermented Merlot samples, where they were consistently lower than freeze dried levels. Anthocyanins were the most challenging compound to maintain during cabinet convection air drying. If the goal is to maximize specific compound types, attention to the particular conditions for each type of pomace is required. However, in general, it appears that subjecting the berry pomace to shorter processing times using cabinet convection air drying (half load density at 70°C) maximizes bioactive quality retention. Therefore the recommended drying conditions, for all berry pomace types, based on the data from the drying kinetics work along with the phenolics and antioxidant activity, is half load density at 70°C. From an efficiency (time and energy) consideration, the shorter drying time is desirable. Finally, these results indicated that dried berry pomace could be a source of bioactive compounds with potential use as an additive for the food industry or other industries.
Acknowledgments
The authors are grateful to Agriculture and Agri-Food Canada for financial support of this research project (#2574). We acknowledge Manon Gentes and David Godfrey for their technical assistance. We are also grateful to Township 7 Winery for donating the fermented Merlot grape pomace used in this study. The authors declare that the research was conducted in the absence of any commercial or financial relationships that could be construed as a potential conflict of interest.
Additional information
Funding
References
- Rogiers, S. Y.; Coetzee, Z. A.; Walker, R. R.; Deloire, A.; Tyerman., S. D. Potassium in the Grape (Vitis Vinifera L.) Berry: Transport and Function. Front. Plant Sci. 2017, 8, 1629. DOI: 10.3389/fpls.2017.01629.
- Hassan, Y. I.; Kosir, V.; Yin, X.; Ross, K.; Diarra, M. S. Grape Pomace as A Promising Antimicrobial Alternative in Feed: A Critical Review. J. Agric. Food Chem. 2019, 67(35), 9705–9718. DOI: 10.1021/acs.jafc.9b02861.
- McDermott, M. 2018. Personal Communication, September, 2018.
- Statistics Canada. From the Vine to the Glass: Canada’s Grape and Wine Industry. [Online]. 2009. https://www150.statcan.gc.ca/n1/pub/11-621-m/11-621-m2006049-eng.htm [accessed Jun 25, 2020]
- Wines of Canada. Grape Varieties in British Columbia.[Online]. 2019. www.winesofcanada.com/grapes-bcvar.html. [accessed Jul 21, 2020]
- Ross, K. A.; Ehret, D.; Godfrey, D.; Fukumoto., L.; Diarra, M. Characterization of Pilot Scale Processed Canadian Organic Cranberry (Vaccinium Macrocarpon) and Blueberry (Vaccinium Angustifolium) Juice Pressing Residues and Phenolic-enriched Extractives. Int. J. Fruit Sci. 2017, 17(2), 202–232. DOI: 10.1080/15538362.2017.1285264.
- Djilas, S.; Canadanovic-Brunet, J.; Cetkovic, G. By-products of Fruits Processing as a Source of Phytochemicals. Chem. Ind. Chem. Eng. Q. . 2009, 15(4), 191–202. DOI: 10.2298/CICEQ0904191D.
- Planinić, M.; Aliakbarian, B.; Perego, P.; Greganic, P.; Tomas, K.; Bucić-Kojić, A. Influence of Temperature and Drying Time on Extraction Yield of Phenolic Compounds from Grape Pomace Variety “Portogizac”. Chem. Biochem. Eng. Q. 2015, 29, 343–350. DOI: 10.15255/CABEQ.2015.2278.
- Tagliani, C.; Perez, C.; Curutchet, A.; Arcia, P.; Cozzano, S. Blueberry Pomace, Valorization of an Industry By-product Source of Fibre with Antioxidant Capacity. Food Sci. Technol. . 2019, 39(3), 644–651. Epub June 06, 2019. DOI: 10.1590/fst.00318.
- Islam, M. R.; Hassan, R. Y. I.; Das, Q.; Lepp, D.; Hernandez, M.; Godfrey, D. V.; Orban, S.; Ross, K.; Delaquis, P.; Diarra, M. S. Dietary Organic Cranberry Pomace Influences Multiple Blood Biochemical Parameters and Cecal Microbiota in Pasture-raised Broiler Chickens. J. Funct. Foods. 2020, 72, 104053. DOI: 10.1016/j.jff.2020.104053.
- Uribe, E.; Lemus-Mondaca, R.; Pasten, A.; Astudillo, S.; Vega-Galvez, A.; Puento-Díaz, L.; Di Scala, K. Dehydrated Olive-waste Cake as a Source of High Value-added Bioproduct: Drying Kinetics, Physicochemical Properties, and Bioactive Compounds. Chilean J. Agric. Res. 2014, 74(3), 293–301.
- Uribe., E.; Pasten, A.; Lemus‐Mondaca, R.; Vega‐Gálvez, A.; Quispe‐Fuentes, I.; Ortiz, J.; Di Scala, K. Comparison of Chemical Composition, Bioactive Compounds and Antioxidant Activity of Three Olive‐waste Cakes. J. Food Biochem. 2015, 39(2), 189–198. DOI: 10.1111/jfbc.12120.
- Reque, P. M.; Steffens, R. S.; Martins Da Silva, A.; Jablonski, A.; Flores, S. H.; Rios, A. O.; Vogt de Jong, E. Characterization of Blueberry Fruits (Vaccinium Spp.) And Derived Products. Food Sci. Technol. Campinas. 2014, 34(4), 773–779. DOI: 10.1590/1678-457X.6470.
- Das, Q.; Islam, M. R.; Dion, L.; Tang, J.; Yin, X.; Mats, L.; Liu, H.; Ross, K.; Kennes, Y. M.; Yacini, H.; et al. Gut Microbiota, Blood Metabolites, and Spleen Immunity in Broiler Chickens Fed Berry Pomaces and Phenolic-enriched Extractives. Front. Vet. Sci. 2020, 7, 150. DOI: 10.3389/fvets.2020.00150.
- Islam., M. R.; Lepp, D.; Godfrey, D. V.; Orban, S.; Ross, K.; Delaquis, P.; Moussa, D. Effects of Wild Blueberry (Vaccinium Angustifolium) Pomace Feeding on Gut Microbiota and Blood Metabolites in Free-range Pastured Broiler Chickens. Poult. Sci. 2019, 98(9), 3739–3755. DOI: 10.3382/ps/pez062.
- Juskiewicz, J.; Jankowski, J.; Kosmala, M.; Zdunczyk, Z.; Slominski, B. A.; Zdunczyk, P. The Effects of Dietary Dried Fruit Pomaces on Growth Performance and Gastrointestinal Biochemistry of Turkey Poults. J. Anim. Physiol. Anim. Nutr. 2016, 100(5), 967–976. DOI: 10.1111/jpn.12415.
- Doymaz, I.;. Drying Characteristics and Kinetics of Okra. J. Food Eng. 2005, 69, 275–279.
- Doymaz, I.; Gol, E. Convective Drying Characteristics of Eggplant Slices. J. Food Process Eng. 2011, 34(4), 1234–1252. DOI: 10.1111/j.1745-4530.2009.00426.x.
- Shilani, R.; Gupta, D. K.; Singh, A. Drying Kinetics of Apple Pomace Cake. J. Food Sci. Technol. 2009, 46(5), 477–479.
- Ertekin, C.; Yaldiz, O. Drying of Eggplant and Selection of a Suitable Thin Layer Drying Model. J. Food Eng. 2004, 63(3), 349–359. DOI: 10.1016/j.jfoodeng.2003.08.007.
- Fudholi, A.; Othman, M. Y.; Ruslan, M. H.; Yahya, M.; Zaharim, A.; Sopian, K. The Effects of Drying Air Temperature and Humidity on the Drying Kinetics of Seaweed. In Recent Research in Geography, Geology, Energy, Environment and Biomedicine, Corfu, Greece, 2011; pp 129–133.
- Sreerama, Y. N.; Sashikala, V. B.; Pratape, V. M. Expansion Prop-erties and Ultrastructure of Legumes: Effect of Chemical and En-zyme Pre-treatments. LWT – Food Sci. Technol. 2009, 42(1), 44–49. DOI: 10.1016/j.lwt.2008.07.005.
- Crank, J.;. The Mathematics of Diffusion; Claredon Press: Oxford, UK, 1975.
- Yagcioglu, A.; Demir, V.; Gunhan, T. Effective Moisture Diffusivity Estimation from Drying Data. Tarim Makinalari Bilimi Dergisi. 2007, 3(4), 249–265.
- Purkayastha, M. D.; Nath, A.; Deka, B. C.; Mahanta, C. L. Thin Layer Drying of Tomato Slices. J. Food Sci. Technol. 2013, 50(4), 642–653. DOI: 10.1007/s13197-011-0397-x.
- Association of Official Analytical Chemists International-AOAC. 2005. Official methods of analysis (18th ed.). AOAC International, Gaithersburg, MD, USA.
- Velioglu, Y. S.; Mazza, G.; Gao, L.; Oomah, B. D. Antioxidant Activity and Total Phenolics in Selected Fruits, Vegetables, and Grain Products. J. Agric. Food Chem. 1998, 46(10), 4113–4117. DOI: 10.1021/jf9801973.
- Singleton, V. L.; Rossi, J. A. Colorimetry of Total Phenolics with Phosphomolybdic–phosphotungstic Acid Reagents. Am. J. Enol. Viticult. 1965, 16, 144–158.
- Harrison, J. E.; Oomah, B. D.; Diarra, M. S.; Ibarra-Alvarado, C. Bioactivities of Pilot Scale Extracted Cranberry Juice and Pomace. J. Food Process. Pres. 2013, 37(4), 356–365. DOI: 10.1111/j.1745-4549.2011.00655.x.
- Marchiani, R.; Bertolino, M.; Ghirardello, D.; McSweeney, P. L., .; Zeppa, G. Physicochemical and Nutritional Qualities of Grape Pomace Powder-fortified Semi-hard Cheeses. J. Food Sci. Technol. 2016, 53(3), 1585–1596. DOI: 10.1007/s13197-015-2105-8.
- Kumar, N.; Sarkar, B. C.; Sharma, H. K. Mathematical Modelling of Thin Layer Hot Air Dyring of Carrot Pomace. J. Food Sci. Technol. 2012, 49(1), 33–41. DOI: 10.1007/s13197-011-0266-7.
- Sairam, N.; Mahanti, N.; Edukondalu, L.; Kumar, G. Effect of Slice Thickness on Drying Kinetics of Papaya Using Food Dehydrator. Int. J. Agric. Environ. Biotechnol. 2017, 10(6), 749. DOI: 10.5958/2230-732X.2017.00092.4.
- Onwude, D.; Norhashila, H.; Rimfiel, J.; Nazmi, N.; Khalina., A. Modeling the Thin-layer Drying of Fruits and Vegetables: A Review. Compr. Rev. Food Sci. Food Saf. 2016, 15(3), 599–618. DOI: 10.1111/1541-4337.12196.
- Milczarek, R.; Dai, A.; Otonic, C.; McHugh, T. Effect of Shrinkage on Isothermal Drying Behavior of 2-phase Olive Mill Waste. J. Food Eng. 2011, 103(4), 434–441. DOI: 10.1016/j.jfoodeng.2010.11.013.
- Meziane, S.; Mesbahi, N. Determination of Moisture Diffusivity and Activation Energy in Thin Layer Drying of Olive Pomace. Int. J. Food Eng. 2012, 8(3), Article 34. DOI: 10.1515/1556-3758.2648.
- Farooq, S.; Rather, S. A.; Gull, A.; Ganai, S. A.; Masoodi, F. A.; Wani, S. M.; Ganaie, T. A. Physicochemical and Nutraceutical Properties of Tomato Powder as Affected by Pretreatments, Drying Methods, and Storage Period. Int. J. Food Prop. 2020, 23(1), 797–808. DOI: 10.1080/10942912.2020.1758716.
- Xia, L.; Xu, C.; Huang, K.; Lu, J.; Zhang, Y. Evaluation of Phenolic Compounds, Antioxidant and Antiproliferative Activities of 31 Grape Cultivars with Different Genotypes Article ID e12626. J. Food Biochem. 2019, 43(6), e12626. DOI: 10.1111/jfbc.12626.
- Xu, C.; Zhang, Y.; Zhu, L.; Huang, Y.; Lu, J. Influence of Growing Season on Phenolic Compounds and Antioxidant Properties of Grape Berries from Vines Grown in Subtropical Climate. J. Agric. Food Chem. 2011, 59(4), 1078–1086. DOI: 10.1021/jf104157z.
- Wang, S. Y.; Chen, C.-T.; Sciarappa, W.; Wang, C. Y.; Camp, M. J. Fruit Quality, Antioxidant Capacity, and Flavonoid Content of Organically and Conventionally Grown Blueberries. J. Agric. Food Chem. 2008, 56(14), 5788–5794. DOI: 10.1021/jf703775r.
- Ribera, A. E.; Reyes-Díaz, M.; Alberdi, M.; Zuñiga, G. E.; Mora, M. L. Antioxidant Compounds in Skin and Pulp of Fruits Change among Genotypes and Maturity Stages in Highbush Blueberry (Vaccinium Corymbosum L.) Grown in Southern Chile. J. Soil. Sci. Plant Nutr. 2010, 10(4), 509–536. DOI: 10.4067/S0718-95162010000200010.
- Issaoui, M.; Delgado, A. M.; Iommi, C.; Chammem, N. Polyphenols and the Mediterranean Diet; Springer: Cham, Switzerland, 2020.