ABSTRACT
Late-stage nitrogen (N) fertilization is an important crop management for improving rice production and also affects the quality of rice, whereas information on the influence of late N on structural and functional properties of indica rice starch is lacking. In this study, effects of various N rates (0, 60, and 120 kg ha−1 referred to as N0, N60 and N120, respectively) on structural and functional properties of grain starches from two rice cultivars (OM052 and Chaoyou1000) were investigated. Comparison of different N treatments showed that late N application exhibited strong influence on starch properties, and the sensitivity to N application varied with rice genotypes. Compared to non N rate, higher input of N (N60 for OM052, and N120 for Chaoyou1000) significantly reduced apparent amylose content (AAC), relative crystallinity (RC), gelatinization enthalpy, swelling power, solubility and degree of hydrolysis, but significantly increased the proportion of short chains with degree of polymerization 6–12 in amylopectin and gelatinization onset temperature. Further comparison and principal component analyses revealed that the variations in the starch functional properties were due mainly to the changes in RC. The solubility was positively associated with AAC. The present results can provide important information for understanding the environmental regulation of starch biosynthesis in rice grain.
Introduction
Rice (Oryza sativa L.) is an important cereal crop that plays a vital role in ensuring food security of many Asian countries. In irrigated rice production system, nitrogen (N) is essential for rice and usually is the most yield-limiting nutrient in rice production around the world.[Citation1] Since the 1960s, rice yield has increased markedly through the use of high-yield varieties and increased application of N fertilizer. In practice, to improve grain yield, farmers generally apply judicious rates of N before heading for panicle-promoting according to the growth of rice. Moreover, proper use of late-stage N fertilizer can also contribute a lot to improve the end-use quality of rice.
Rice quality is mainly related to its starch properties because starch constitutes about 90% of milled rice. In rice grain, starch consists of two main components, linear amylose and highly branched amylopectin, which together form insoluble semi-crystalline granules.[Citation2] The starch granules are packed in the form of semi-crystalline areas and amorphous regions in an alternating fashion, displaying as an internal lamellar structure. The crystalline regions of the lamellae are thought to be formed by double helices of amylopectin side chains packed laterally into a crystalline lattice.[Citation3] The amylopectin side chains can form two types of helices in starch granule. Helices that are packed in the short-range order are defined as the double-helical order, and helices that are packed in the long-range order are related to the packing of double helices forming crystallinity.[Citation4] Therefore, the fine structures of starch mainly include the proportion of amylose and amylopectin, amylopectin molecular structure, and crystalline structure of starch granule.
Gelatinization and hydrolysis properties of starch are generally considered as key functional properties that determine many applications of starch.[Citation5,Citation6] Gelatinization is an endothermic process that results in the disruption of molecular order within the starch. During the gelatinization process, granules absorb water and swell, the double-helical and crystalline structures are disrupted, which causes an increase in granule swelling and solubility. The gelatinization characteristics (including gelatinization transition temperature and gelatinization enthalpy) of starch can usually be measured by differential scanning calorimetry.[Citation6] Starch hydrolysis is involved in many biological and industrial processes.[Citation6] To analyze hydrolysis properties, starches are usually hydrolyzed by acid and two important amylolytic enzymes, α-amylase and amyloglucosidase.[Citation7,Citation8] The α-amylase is an endoamylase that cleaves the α-1,4 glycosidic bonds of amylose or the amylopectin chain at internal positions, while the amyloglucosidase is an exoamylase catalyzing the hydrolysis of both α-1,4 and α-1,6 glycosidic bonds at the branching point to release b-D-glucose residues of the polymer substrate.[Citation9] The functional properties of rice starch have been suggested to be influenced by its molecular and crystalline structures.[Citation8,Citation10,Citation11]
Structures and functional properties of rice starch depend on both genetic background and environmental factors during rice growth and development.[Citation12–14] With regard to the influence of N fertilization on starch properties, previous studies focused solely on japonica rice materials, and showed that late-stage N application may have marked effects on starch quality parameters.[Citation15–17] However, to our best knowledge, research on the influence of late N application on the structural and functional properties of indica rice starch has rarely been reported. Since the response to nitrogen, nitrate use efficiency and grain quality characteristics are very different between the japonica and indica rice subspecies,[Citation18–20] previous research results obtained from japonica rice may have limited values in guiding the production of indica rice with desired starch qualities. Indica rice starch is an essential commodity valued for its end uses in food, feed, medicine, and many other industries. Application of starch requires prior knowledge of its structural and functional properties. Therefore, the main objective of this study was to investigate the effects of varied levels of late-stage N fertilizer on the molecular structure (apparent amylose content and amylopectin chain length distribution), crystalline structure (X-ray diffraction pattern and relative crystallinity, and ordered structure of starch external region), as well as on the gelatinization and hydrolysis properties of indica rice starch.
Materials and methods
Plant materials
Two indica rice cultivars, OM052 and Chaoyou1000, were used in this study. OM052 is an inbred rice cultivar with high nitrogen use efficiency, while Chaoyou1000 is a hybrid rice cultivar famous for its super high yield (more than 15000 Kg ha−1). This experiment was carried out on the experimental farm of Anhui Academy of Agricultural Sciences, Hefei, P. R. China, in the normal planting season (from May to September) in 2016. Based on the application of equal compound fertilizer (200 kg ha−1) and urea (150 kg ha−1), three nitrogen rates (N0, 0 kg N ha−1; N60, 60 kg N ha−1; N120, 120 kg N ha−1) were employed at panicle heading stage, and each treatment was repeated three times. Plants were managed normally in cement pool cultivation, and the pools for N treatment were separated by bunds and plastic films. Growth management including prevention of diseases and insects was followed as other standard paddy fields during the course of experiments. After being air-dried and stored at room temperature for 3 months, the mature grains were used to isolate starch granules.
Preparation of native rice starches
Native starches were isolated from mature grains as previously described.[Citation21] Briefly, the brown rice was steeped in 0.2% NaOH for 2 days and homogenized in a mortar with a pestle. The homogenate was squeezed through five layers of cotton cloth and then filtered with 100-, 200-, and 400- mesh sieves, successively. The starch was washed with 0.2% NaOH and centrifuged at 3000 g for 10 min. The starch was washed with deionized water and centrifuged at 3000 g for 10 min. After removing the dirty layer on the top of starch precipitate, the starch was further washed with deionized water and dehydrated with anhydrous ethanol. Finally, the precipitated starch was dried under atmosphere, ground into powder, and passed through a 100-mesh sieve.
Measurements of protein content and apparent amylose content
Total protein was extracted using a total protein extraction Kit (BC3710, Solarbio, China) according to the manufacturer’s instructions, and the protein content was assayed according to the procedures of a protein assay Kit (Quick Start Bradford 1, Bio-Rad, USA). Apparent amylose content (AAC) was determined in accordance with the method reported by Teng et al.[Citation13]
Structural analysis of amylopectin
The amylopectin chain length distribution was analyzed by high-performance anion-exchange chromatography equipped with a pulsed amperometric detection (HPAEC-PAD) (ICS5000, Dionex, USA) according to the procedure of You et al.[Citation11] with some modifications. Briefly, 10 mg of rice starch was dispersed in 1.0 mL of 95% ethanol; then, the solution was mixed with 9.0 mL of deionized water and heated in boiling water with stirring for 60 min. The sample was cooled to room temperature and centrifuged at 4000 rpm for 10 min. The precipitate was then stirred into 2 mL of 50 mM sodium acetate buffer (pH 3.5) and 20 μL of isoamylase (1000 U/μL, I5284, Sigma) were added. The enzymatic reaction was carried out at 40°C for 24 hours using a ThermoMixer with continuous shaking (300 rpm). Enzyme activity was terminated by heating the mixture in a boiling water bath for 20 min, and an aliquot (400 μL) of the debranched sample was diluted with 2 mL of 150 mM NaOH. The sample was filtered (0.45 um nylon syringe filter) and injected 25 μL of the sample into the HPAEC-PAD system. The separation was obtained using a CarboPac PA1 column (4 × 250 mm, Dionex, USA) with a guard column and a gradient eluent with 150 mM NaOH and 500 mM sodium acetate in 150 mM NaOH at a flow rate of 0.5 mL/min. The peak areas corresponding to different chain lengths of amylopectin were analyzed using the PeakNet software (Dionex, USA).
X-ray diffraction analysis
X-ray diffraction (XRD) analysis of starch was performed with an XRD (D8, Bruker, Germany) according to the method of Teng et al.[Citation22] Before measurements, all the samples were stored in a desiccator where a saturated solution of NaCl maintained a constant humidity atmosphere (relative humidity = 75%) for a week. Water contents of the hydrated starch samples were calculated from the weight difference before and after drying at 130°C. The moisture contents of the hydrated starch samples were approximately 20%. The samples were exposed to the X-ray beam at 200 mA and 40 kV. The scanning region of the diffraction angle (2θ) was from 3 to 40 with a step size of 0.02 and a count time of 0.8 s. Relative crystallinity of starch was quantitatively calculated using software Origin 8.0 (Microcal, Northampton, MA).
Attenuated total reflectance Fourier transform infrared analysis
Ordered structure of starch external region was analyzed on a Varian 7000 Fourier transform infrared (FTIR) spectrometer with a deuterated sulfur triglycine peptide detector equipped with an attenuated total reflectance (ATR) single reflectance cell containing a germanium crystal (45° incidence-angle) (PIKE Technologies, USA) as previously described by Wei et al.[Citation21] with some modifications. Original spectra were corrected by subtraction of the baseline in the region from 1200 to 800 cm−1 before deconvolution was applied using Resolutions Pro. The assumed line shape was Lorentzian with a half-width of 19 cm−1 and a resolution enhancement factor of 1.9. Intensity measurements at 1047 and 1022 cm−1 were performed on the deconvoluted spectra by recording the height of the absorbance bands from the baseline using Adobe Photoshop 7.0 image software.
Differential scanning calorimetry analysis
Gelatinization properties of starch were investigated with the use of a differential scanning calorimetry (DSC) (200-F3, Netzsch, Germany) according to the method of Teng et al.[Citation22] Each sample (3 mg, dry starch basis) was precisely weighed and mixed with 3 times (by weight) deionized-distilled water (9 μL) in a DSC pan. The pans were then sealed, equilibrated for 4 h at room temperature (20°C), then heated from 20 to 120°C at a rate of 10°C/min. An empty pan was used as a control. Gelatinization onset temperature (To), peak temperature (Tp), conclusion temperature (Tc), transition temperature range (ΔT, Tc–To), and enthalpy (ΔH) values were measured from the DSC thermogram. The experiments were performed in triplicate.
Swelling power and solubility determination
The swelling power (g/g) and water solubility of starch were determined using 2% aqueous suspension of the starch according to the procedures of Cai et al.[Citation7] with some modifications. Briefly, 60 mg of dry starch was weighed into a pre-weighed centrifuge tube (10 mL). Then, the sample was mixed with 3.0 mL of double-distilled water and heated in a water bath at 95°C for 30 min with regular gentle inversions. After cooling for 30 min, the tube was centrifuged at 8000 g for 15 min, and the supernatant was removed and the sediment was weighed. The supernatant was dried by evaporation at 105°C to constant weight and weighed. The experiments were performed in triplicate. The swelling power and solubility (%) were calculated as follows:
Swelling power = (sediment weight)/(amount of dry starch-amount of dried supernatant),
Solubility (%) = (amount of dried supernatant×100)/amount of dry starch.
Hydrolysis degree determination
Starch was hydrolyzed by HCl, porcine pancreatic-amylase (PPA, Sigma A3176), and Aspergillus niger amyloglucosidase (AAG, Megazyme E-AMGDF) using the methods of Cai et al.[Citation7] with some modifications. For HCl hydrolysis, a total of 100 mg of starch was suspended in 10 mL of 2.2 M HCl, and hydrolysis was conducted in a ThermoMixer at 35°C with continuous shaking (800 rpm) for 4 days. For PPA hydrolysis, 50 mg of starch was suspended in 10 mL of enzyme solution (0.1 M phosphate sodium buffer, pH 6.9, 25 mM NaCl, 5 mM CaCl2, 0.02% NaN3, and 50 U PPA). For AAG hydrolysis, 50 mg of starch was suspended in 10 mL of enzyme solution (0.05 M acetate buffer, pH 4.5, and 5 U AAG). The enzymatic hydrolysis was conducted in a ThermoMixer with continuous shaking (800 rpm) at 37°C for PPA or at 55°C for AAG hydrolysis. After 12 h of hydrolysis, the starch slurry was quickly centrifuged (5000 × g) at 4°C for 5 min and the supernatant was used for measuring the soluble carbohydrates to quantify the hydrolysis degree with the anthrone–H2SO4 method. The experiments were repeated in triplicate.
Data analysis
All statistical analyses were performed using the SPSS 20.0 Statistical Software Program. Means were tested by least significant difference at P = .05 level (Duncan0.05). Principal component analysis (PCA) was performed to determine the relationship between different variables of starch property.
Results and discussion
Protein content and molecular structure of starch
In the present study, the late N fertilization exhibited a significant influence on the contents of total protein and amylose, while the effects varied with N rate and rice genotype (). For all the starch samples, milled rice protein content was lowest in the N0 treatment and highest under N120 rate, which was consistent with the increase of fertilizer application. For AAC, the increase of N application rate resulted in decreased AACs in both rice varieties, which was in agreement with previous findings.[Citation15,Citation23] At medium (N60) N rate, AAC of OM052 significantly decreased as compared to non (N0) N rate, but did not show significant decrease beyond N60 rate. For Chaoyou1000, slight differences in AAC were observed under N0 and N60 rates, and further increase in N application rate caused a significant decrease in AAC. This result suggested that Chaoyou1000 are relatively less sensitive to late N fertilization, which might be explained as that hybrid rice requires relatively higher N application than inbred rice. With regard to the influence of N on AAC, inconsistent results were reported in previous studies. For instance, Singh et al.[Citation23] and Yang et al.[Citation15] pointed that AAC decreased with increasing N fertilizer in low amylose rice cultivars, while an opposite trend was observed in rice materials with the Wxa allele as reported by Cao et al.[Citation16] The reasons for contradictory results from the present and previous studies might be due to the different genetic materials tested, since amylose biosynthesis in rice grain is a result of joint influence of genotype–environment interaction.[Citation12,Citation13]
Table 1. Protein content and molecular structure characteristics of rice starches fertilized with different nitrogen application ratesa.
Normalized chromatograms of amylopectin chain length distribution are presented in . For each rice cultivar, distinct differences in the chromatograms were observed under various N treatments, as expected. The HPAEC system used in this study was sensitive to resolve glucans with degree of polymerization (DP) ≤75. Amylopectin branch chains are categorized into four chain types: A chains (DP 6-12), B1 chains (DP 13-24), B2 chains (DP 25-36), and B3+ chains (DP ≥ 37) according to the range of chain length.[Citation24] The proportion of each chain type and average chain length of amylopectin are summarized in . In the present study, late N treatments significantly increased the proportion of short A chains (DP 6-12) in OM052 and Chaoyou1000 at N60 and N120, respectively. However, Yang et al.[Citation15] reported that higher input of late N might lower the percentage of short A chains (DP 6-12), which is inconsistent with our findings. This difference may come from different rice materials tested, since the materials investigated by Yang et al.[Citation15] were japonica but not indica rice. For short B1 chains (DP 13-24), no significant differences were detected between different N rates for OM052. While for Chaoyou1000, high input of N rate (N120) significantly increased the proportion of B1 chains. For long B2 chains (DP 25-36), no significant influence of N treatments was found for the tested rice. In addition, increase of N application (N60 for OM052, and N120 for Chaoyou1000) resulted in significant decreases in the amount of long B3+ chains (DP ≥ 37) and average chain length of amylopectin. In the current study, from the comparison between the three N rates, it appears that the variations in proportion of short chains (DP 6-12) were inversely associated with the changes in AAC (). In consistent with our findings, previous studies have suggested that rice starch with lower AAC is prone to have more amylopectin short branches.[Citation7,Citation10]
Crystalline structure of starch
The X-ray diffraction patterns of the fertilized starch samples are shown in . The tested starches all had strong diffraction peaks at about 15° and 23° 2θ and unresolved bimodal at around 17° and 18° 2θ, indicating that the diffraction pattern is all A-type. However, there existed differences in the peak positions among different N application treatments. Significant differences in relative crystallinity (RC) were observed with different N management rates (). Increase in N rate might lead to significant decrease in RC, and the variation trend in RC was in consistent with the changes in AAC and proportions of amylopectin short A chains (DP 6-12) under different N rates (). The exact nature of crystalline polymorphs should be attributed to the differences in proportions of amylose, short and long chains of amylopectin, and the interaction of double helices in starch granule.[Citation25] Chung et al.[Citation10] claimed that the presence of short chains (DP < 10) in amylopectin might result in a decrease in the stability of the double helix. You et al.[Citation11] also reported that the presence of short chains (DP 6-12) in amylopectin led to a decrease in crystallinity. Consistent with these reports, a negative association between RC and the proportion of DP 6-12 under various N rates was observed in this study (). In common sense, RC was suggested to be negatively correlated with amylose content among different rice genotypes.[Citation7,Citation10,Citation22] However, a positive association between RC and AAC was obtained in the present study (), strongly indicating that the changes in RC might not be due to the variations in AAC. Finally, based on our and previous results, it could be inferred that the molecular structure mechanism of the variations in RC caused by late N application is different from that resulted from genotypic differences. In future work, how the molecular structure of amylopectin affects the ordered structure of starch internal region needs to be further elucidated.
Table 2. Relative crystallinities and intensity ratios (1047/1022 cm−1) of rice starches fertilized with different nitrogen application ratesa.
Figure 2. X-ray diffraction patterns of rice starches affected by different nitrogen application rates. N0, non-nitrogen rate of 0 kg ha−1; N60, medium nitrogen rate of 60 kg ha−1; N120, high nitrogen rate of 120 kg ha−1
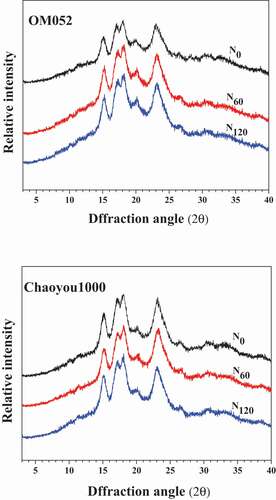
The deconvoluted ATR-FTIR spectra of rice starch samples are shown in . There were no significant differences in IR spectra among the tested starch samples. Amylopectin side chains can be arranged in two types of helices, short-range and long-range order, in starch granules.[Citation4] The intensity of absorbance at 1047 and 1022 cm−1 is sensitive to the changes in crystalline and amorphous structures near granule surface, respectively.[Citation26] The IR ratio of 1047/1022 cm−1 was used to assess the amount of ordered starch relative to amorphous starch as a convenient index of FTIR data.[Citation27,Citation28] The intensity ratios of 1047/1022 cm−1 were calculated as shown in . In this study, the tested rice starches showed significant differences in the ratio of 1047/1022 cm−1, and there existed genotypic differences in response to N treatments. The 1047/1022 cm−1 ratios of Chaoyou1000 under different N fertilizer rates followed the order N0 = N60 > N120, which was in agreement with the RC results. However, the trend of 1047/1022 cm−1 in OM052 was N0 < N60 = N120, which was in contrast with the RC.
Gelatinization properties
Differential scanning calorimetry was used to measure the thermal properties of rice starches. Gelatinization onset, peak, and conclusion temperatures (To, Tp, Tc), gelatinization transition temperature range (ΔT, Tc–To), and gelatinization enthalpy (ΔH) are summarized in . As expected, the fertilized starch samples showed significant differences in gelatinization property parameters, and the responses to N treatments were different between the two rice cultivars. Starches from OM052 treated with N60 and N120 rates had significantly higher values of To compared with the N0 samples, while only excessive N rate (N120) caused a significant increase in To of starch samples from Chaoyou1000. Further, comparison analysis revealed that To was positively associated with RC and negatively related to AAC, which is in keeping with previous reports.[Citation22] For Tp and Tc, starch samples of each cultivar stabilized for all of the N treatments. For ΔT, late N application showed significant influence on Chaoyou1000, while had slight effect on OM052. For ΔH, significant changes in the fertilized starches were observed when the amount of fertilizer N added to a high rate (N60 for OM052 and N120 for Chaoyou1000), which was consistent with the RC results (). According to Tester and Morrison,[Citation29] ΔH reflects the overall crystallinity (quantity and amount of starch crystallites) of amylopectin. Gunaratne and Hoover[Citation30] postulated that ΔH represents the loss of double-helical order in crystalline and non-crystalline regions. Therefore, in this study, the variations in ΔH could be partly due to the differences in double helices in crystalline regions caused by different N treatments ().
Table 3. Thermal and swelling properties of rice starches fertilized with different nitrogen application ratesa.
Swelling power and solubility
When starch is heated in excess water the crystalline structure is disrupted, and water molecules become linked to the exposed amylose and amylopectin, causing an increase in granule swelling and solubility.[Citation6] In this study, the application of late N exerted significant influence on swelling property parameters (). Application of N fertilizer exerted significant and negative influence on swelling property parameters as the N fertilizer rate increased beyond a certain level (N60 for OM052, and N120 for Chaoyou1000). Swelling and solubility behaviors have been suggested to be mainly influenced by amylose content, amylopectin molecular structure and crystalline structure.[Citation6,Citation7,Citation22] Previous studies showed that swelling power is positively correlated with RC but negatively correlated with amylose content.[Citation7,Citation22] In this study, the decreased swelling powers and solubilities of starches were clearly associated with the reduced AACs and relative crystallinities (). Thus, it can be inferred that the changes in swelling power caused by late N should be attributed to the variations in RC but not AAC. For normal rice starch granules, during the swelling process, the main component leached from the granules is amylose.[Citation31] Therefore, it is not difficult to understand that solubility decreased with AAC in the starch samples of OM052 and Chaoyou1000 ().
Hydrolysis properties
In food and nonfood industries, the application of starch requires the disruption of starch granules. Enzyme hydrolysis of starch is involved in many industrial processes, such as malting, fermentation, glucose syrup, and bioethanol production, while acid hydrolysis is widely used to produce thin boiling starches for use in food, paper, textile, and other industries.[Citation32] Therefore, it is useful to investigate the hydrolysis properties for starch application. In this study, the fertilized starch samples were subjected to 12 h of hydrolysis by PPA and AAG, and 4 days of hydrolysis by HCl (). The present results showed that Late N application had significant influence on hydrolysis degrees of these rice starches. Under N60 rate, hydrolysis degrees of native starches from OM052 markedly decreased from 65.19 to 62.65% for PPA, from 60.65 to 57.31% for AAG, and from 63.45 to 59.09% for HCl. For Chaoyou1000, when N fertilizer was enhanced to a high (N120) rate, significant decreases were observed in the degrees of hydrolysis by PPA, AAG, and HCl from 69.26 to 64.48%, 65.34 to 58.41%, and 66.34 to 59.83%, respectively. Increase in N fertilizer rate could lead to significant decrease in hydrolysis degree, which was consistent with the variations in AAC, RC, and amylopectin short chains (). Susceptibility of starch to PPA, AAG, and HCl attack is influenced by many factors such as amylose content, amylopectin molecular structure, crystalline structure, granule size and integrity, and structural in homogeneities.[Citation7,Citation8,Citation33] Generally, it is suggested that the rate of starch hydrolysis by amylase is positively correlated with the proportion of DP 6-12 in amylopectin and degree of crystallinity but is inversely related to amylose content and the proportion of amylopectin long branch chains (DP > 24).[Citation7,Citation8,Citation34,Citation35] In the current study, however, the results showed that lowing AAC or increasing short A chains (DP 6-12) did not increase hydrolysis degree for each rice material (). Therefore, the reason that rice starch samples treated with higher rates of N exhibited lower hydrolysis degrees might be due to its relatively lower degrees of RC.
Table 4. Hydrolysis degrees of rice starches fertilized with different nitrogen application ratesa.
Relationship between the structural and functional properties
In this study, a PCA analysis was performed to visualize the relationships between different starch properties of the fertilized starch samples. Based on factor analysis, the two first principal factors which accounted for 94.42% of the variance in all variables were used for drawing the PCA loading plot (). The values in the PCA loading plot indicate the contribution of each measured starch property to the factors. By employing the PCA loading plot, gelatinization temperatures (To, Tp, Tc), ΔH and SP were found to be located in the same direction with RC. Besides, it was also observed that RC is located closely and oppositely with the degree of hydrolysis by PPA, AAG and HCl. These results indicate that RC might play an important role in regulating starch functional properties under various N treatments. The variables of amylopectin short B1 chains (DP 13-24) and gelatinization temperatures To located closely in group, indicating positive correlations between them. Similarly, Nakamura et al.[Citation36] reported that higher proportion of DP 13-24 could contribute to higher values of gelatinization temperatures. The variables of AAC and solubility are located in the same direction, which was in accordance with previous reports that AAC is positively correlated with solubility.[Citation7,Citation22] Moreover, the PCA results showed that the proportion of amylopectin short A chains (DP 6-12) and protein content had little correlations with other starch properties.
Figure 4. Principal component analysis of the variation in structural and functional properties of rice starches from different rice cultivars. A, B1, B2 and B3+ are the proportions of amylopectin branch chains of DP 6-12, DP 13-24, DP 25-36, and DP ≥ 37, respectively; ACL, average chain length of amylopectin; AAC, apparent amylose content; PC, protein content; RC, relative crystallinity; IR, intensity ratio of 1047/1022 cm−1; To, onset temperature; Tp, peak temperature; Tc, conclusion temperature; ΔT, gelatinization range (Tc–To); ΔH, enthalpy of gelatinization; SP, swelling power
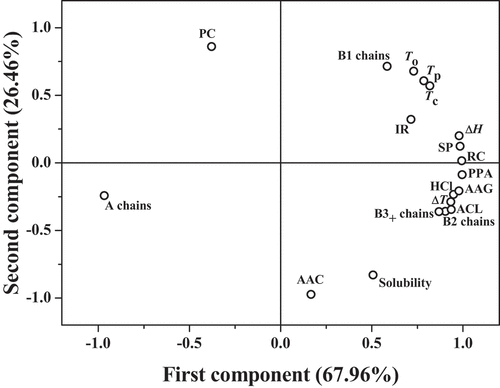
Conclusion
The application of late-stage N significantly affected the structural and functional properties of starches from two indica rice cultivars. In the present study, the response of starch properties to late N application was genotype-dependent. Increase in N rate might lead to significant reductions in AAC, RC, gelatinization enthalpy, swelling power, solubility and degree of hydrolysis, and cause significant increases in the proportion of amylopectin short A chains (DP 6-12) and gelatinization onset temperature. Further comparison and principal component analyses of the relations between different rice starch properties provided a better understanding of how the late N fertilization affects starch functional properties through the influence of molecular and crystalline structures of rice starch. It could be concluded that gelatinization enthalpy, swelling power, and the degree of hydrolysis were mainly determined by the degree of crystallinity as influenced by late N fertilization. The above results may provide valuable information for the regulation of the end-use quality of rice starch.
Correction Statement
This article has been republished with minor changes. These changes do not impact the academic content of the article.
Additional information
Funding
References
- Samonte, S. O.; Wilson, L. T.; Medley, J. C.; Pinson, S. R. M.; Mc-Clung, A. M.; Lales, J. S. Nitrogen Utilization Efficiency: Relationships with Grain Yield, Grain Protein, and Yield-related Traits in Rice. Agron. J. 2006, 98, 168–176. DOI: https://doi.org/10.2134/agronj2005.0180.
- Zeeman, S. C.; Kossmann, J.; Smith, A. M. Starch: Its Metabolism, Evolution, and Biotechnological Modification in Plants. Annu. Rev. Plant Biol. 2010, 61, 209–234. DOI: https://doi.org/10.1146/annurev-arplant-042809-112301.
- Blazek, J.; Gilbert, E. P. Application of Small-angle X-ray and Neutron Scattering Techniques to the Characterisation of Starch Structure: A Review. Carbohydr. Polym. 2011, 85, 281–293. DOI: https://doi.org/10.1016/j.carbpol.2011.02.041.
- Atichokudomchai, N.; Varavinit, S.; Chinachoti, P. A. A Study of Ordered Structure in Acid-modified Tapioca Starch by 13C CP/MAS Solid-state NMR. Carbohydr. Polym. 2004, 58, 383–389. DOI: https://doi.org/10.1016/j.carbpol.2004.07.017.
- Tawil, G.; Viksø-Nielsen, A.; Rolland-Sabaté, A.; Colonna, P.; Buléon, A. In Depth Study of a New Highly Efficient Raw Starch Hydrolyzing A-amylase from Rhizomucor Sp. Biomacromolecules. 2011, 12, 34–42. DOI: https://doi.org/10.1021/bm100913z.
- Wani, A. A.; Singh, P.; Shah, M. A.; Schweiggert-Weisz, U.; Gul, K.; Wani, I. A. Rice Starch Diversity: Effects on Structural, Morphological, Thermal, and Physicochemical Properties–a Review. Compr. Rev. Food Sci. Food Saf. 2012, 11, 417–436.
- Cai, J.; Man, J.; Huang, J.; Liu, Q.; Wei, W.; Wei, C. Relationship between Structure and Functional Properties of Normalrice Starches with Different Amylose Contents. Carbohydr. Polym. 2015, 125, 35–44. DOI: https://doi.org/10.1016/j.carbpol.2015.02.067.
- Lin, L.; Cai, C.; Gilber, R. G.; Li, E.; Wang, J.; Wei, C. Relationships between Amylopectin Molecular Structures and Functional Properties of Different-sized Fractions of Normal and High-amylose Maize Starches. Food Hydrocolloids. 2016, 52, 359–368. DOI: https://doi.org/10.1016/j.foodhyd.2015.07.019.
- van der Maarel, M. J. E. C.; van der Veen, B.; Uitdehaag, J. C. M.; Leemhuis, H.; Dijkhuizen, L. Properties and Applications of Starch-converting Enzymes of the A-amylase Family. J. Biotechnol. 2002, 49, 137–155. DOI: https://doi.org/10.1016/S0168-1656(01)00407-2.
- Chung, H. J.; Liu, Q.; Lee, L.; Wei, D. Z. Relationship between the Structure, Physicochemical Properties and in Vitro Digestibility of Rice Starches with Different Amylose Contents. Food Hydrocolloids. 2011, 25, 968–975. DOI: https://doi.org/10.1016/j.foodhyd.2010.09.011.
- You, S. Y.; Oh, S. K.; Kim, H. S.; Chung, H. J. Influence of Molecular Structure on Physicochemical Properties and Digestibility of Normal Rice Starches. Int. J. Biol. Macromol. 2015, 77, 375–382. DOI: https://doi.org/10.1016/j.ijbiomac.2015.02.054.
- Bao, J. S.; Kong, X. L.; Xie, J. K.; Xu, L. J. Analysis of Genotypic and Environmental Effects on Rice Starch. 1. Apparent Amylose Content, Pasting Viscosity, and Gel Texture. J. Agric. Food Chem. 2004, 52, 6010–6016. DOI: https://doi.org/10.1021/jf049234i.
- Teng, B.; Zeng, R.; Wang, Y.; Liu, Z.; Zhang, Z.; Zhu, H.; Ding, X.; Li, W.; Zhang, G. Detection of Allelic Variation at the Wx Locus with Single-segment Substitution Lines in Rice (Oryza Sativa L.). Mol. Breed. 2012, 30, 583–595. DOI: https://doi.org/10.1007/s11032-011-9647-x.
- Fujita, N.;. Starch Biosynthesis in Rice Endosperm. AGri Biosci. Monogr. 2014, 4, 1–18. DOI: https://doi.org/10.5047/agbm.2014.00401.0001.
- Yang, X.; Bi, J.; Gilbert, R. G.; Li, G.; Liu, Z.; Wang, S.; Ding, Y. Amylopectin Chain Length Distribution in Grains of Japonica Rice as Affected by Nitrogen Fertilizer and Genotype. J. Cereal Sci. 2016, 71, 230–238. DOI: https://doi.org/10.1016/j.jcs.2016.09.003.
- Cao, X. M.; Sun, H. Y.; Wang, C. G.; Ren, X. J.; Liu, H. F.; Zhang, Z. J. Effects of Late-stage Nitrogen Fertilizer Application on the Starch Structure and Cooking Quality of Rice. J. Sci. Food Agric. 2018, 98, 2332–2340. DOI: https://doi.org/10.1002/jsfa.8723.
- Tang, S.; Zhang, H.; Liu, W.; Dou, Z.; Zhou, Q.; Chen, W.; Ding, Y. Nitrogen Fertilizer at Heading Stage Effectively Compensates for the Deterioration of Rice Quality by Affecting the Starch-related Properties under Elevated Temperatures. Food Chem. 2019, 277, 455–462. DOI: https://doi.org/10.1016/j.foodchem.2018.10.137.
- Umemoto, T.; Yano, M.; Satoh, H.; Shomura, A.; Nakamura, Y. Mapping of a Gene Responsible for the Difference in Amylopectin Structure between Japonica-type and Indica-type Rice Varieties. Theor. Appl. Genet. 2002, 104, 1–8. DOI: https://doi.org/10.1007/s001220200000.
- Kang, H. J.; Hwang, I. K.; Kim, K. S.; Choi, H. C. Comparison of the Physicochemical Properties and Ultrastructure of Japonica and Indica Rice Grains. J. Agric. Food Chem. 2006, 54, 4833–4838.
- Hu, B.; Wang, W.; Ou, S.; Tang, J.; Li, H.; Che, R.; Zhang, Z.; Chai, X.; Wang, H.; Wang, Y.;; et al. Variation in NRT1.1B Contributes to Nitrate-use Divergence between Rice Subspecies. Nat. Genet. 2015, 47, 834–838. DOI: https://doi.org/10.1038/ng.3337.
- Wei, C. X.; Xu, B.; Qin, F. L.; Yu, H. G.; Chen, C.; Meng, X. L.; Zhu, L.; Wang, Y.; Gu, M.; Liu, Q. C-type Starch from High-amylose Rice Resistant Starch Granules Modified by Antisense RNA Inhibition of Starch Branching Enzyme. J. Agric. Food Chem. 2010, 58(12), 7383–7388.
- Teng, B.; Zhang, Y.; Du, S.; Wu, J.; Li, Z.; Luo, Z.; Yang, J. Crystalline, Thermal and Swelling Properties of Starches from Single-segment Substitution Lines with Different Wx Alleles in Rice (Oryza Sativa L.). J. Sci. Food Agric. 2017, 97, 108–114. DOI: https://doi.org/10.1002/jsfa.7693.
- Singh, N.; Pala, N.; Mahajan, G.; Singh, S.; Shevkani, K. Rice Grain and Starch Properties: Effects of Nitrogen Fertilizer Application. Carbohydr. Polym. 2011, 86, 219–225. DOI: https://doi.org/10.1016/j.carbpol.2011.04.039.
- Hanashiro, I.; Abe, J.; Hizukuri, S. A Periodic Distribution of the Chain Length of Amylopectin as Revealed by High-performance Anion-exchange Chromatography. Carbohydr. Res. 1996, 283, 151–159. DOI: https://doi.org/10.1016/0008-6215(95)00408-4.
- Singh, J.; Kaur, L.; McCarthy, O. J. Factors Influencing the Physicochemical, Morphological, Thermal and Rheological Properties of Some Chemically Modified Starches for Food Applications: A Review. Food Hydrocolloids. 2007, 21, 1–22. DOI: https://doi.org/10.1016/j.foodhyd.2006.02.006.
- Van Soest, J. J. G.; Tournois, H.; de Wit, D.; Vliegenthart, J. F. G. Short-range Structure in (Partially) Crystalline Potato Starch Determined with Attenuated Total Reflectance Fourier-transform IR Spectroscopy. Carbohydr. Res. 1995, 279, 201–214. DOI: https://doi.org/10.1016/0008-6215(95)00270-7.
- Sevenou, O.; Hill, S. E.; Farhat, I. A.; Mitchell, J. R. Organization of the External Region of the Starch Granule as Determined by Infrared Spectroscopy. Int. J. Biol. Macromol. 2002, 31, 79–85. DOI: https://doi.org/10.1016/S0141-8130(02)00067-3.
- Warren, F. J.; Royall, P. G.; Gaisford, S.; Butterworth, P. J.; Ellis, P. R. Binding Interactions of α-amylase with Starch Granules: The Influence of Supramolecular Structure and Surface Area. Carbohydr. Polym. 2011, 86, 1038–1047. DOI: https://doi.org/10.1016/j.carbpol.2011.05.062.
- Tester, R. F.; Morrison, W. R. Swelling and Gelatinization of Cereal Starches I. Effects of Amylopectin, Amylose and Lipids. Cereal Chem. 1990, 67, 551–557.
- Gunaratne, A.; Hoover, R. Effect of Heat–moisture Treatment on the Structure and Physicochemical Properties of Tuber and Root Starches. Carbohydr. Polym. 2002, 49, 425–437. DOI: https://doi.org/10.1016/S0144-8617(01)00354-X.
- Mandala, I. G.; Bayas, E. Xanthan Effect on Swelling, Solubility and Viscosity of Wheat Starch Dispersions. Food Hydrocolloids. 2004, 18, 191–201. DOI: https://doi.org/10.1016/S0268-005X(03)00064-X.
- Rohwer, R. G.; Klem, R. E. Chapter XVII - Acid-Modified Starch: production and uses. Starch: Chemistry and Technology (2nd Edition), (Academic Press, San Diego, 1984), pp. 529–541
- Blazek, J.; Gilbert, E. P. Effect of Enzymatic Hydrolysis on Native Starch Granule Structure. Biomacromolecules. 2010, 11, 3275–3289. DOI: https://doi.org/10.1021/bm101124t.
- Li, J. H.; Vasanthan, T.; Hoover, R.; Rossnagel, B. G. Starch from Hull-less Barley: V. In-vitro Susceptibility of Waxy, Normal, and High-amylose Starches Towards Hydrolysis by Alpha-amylases and Amyloglucosidase. Food Chem. 2004, 84, 621–632. DOI: https://doi.org/10.1016/S0308-8146(03)00287-5.
- Teng, B.; Zhang, C.; Zhang, Y.; Du, S.; Xi, M.; Song, F.; Ni, J.; Luo, Z.; Ni, D. Effects of Different Wx Alleles on Amylopectin Molecular Structure and Enzymatic Hydrolysis Properties of Rice Starch. Int. J. Food Prop. 2018, 21, 2772–2784. DOI: https://doi.org/10.1080/10942912.2018.1561464.
- Nakamura, Y.; Sakurai, A.; Inaba, Y.; Kimura, K.; Iwasawa, N.; Nagamine, T. The Fine Structure of Amylopectin in Endosperm from Asian Cultivated Rice Can Be Largely Classified into Two Classes. Starch. 2002, 54, 117–131. DOI: https://doi.org/10.1002/1521-379X(200204)54:3/4<117::AID-STAR117>3.0.CO;2-2.