ABSTRACT
Casein is an abundant, cheap, and easy to modify milk protein, which is useful as a wall material to encapsulate organic and inorganic substances that can be applied to protect, load, and deliver flavorings, phenolic compounds, drugs, or inorganic substances. There are many reviews that have explored casein itself as well as the configuration of micelle structures to load substances, but there is a lack of condensed information about the physicochemical properties of casein capsules and the factors that influence them, such as the type of casein, core material and the methods used to obtain the capsules and characterize them. The aim of this review was to identify trends among the different types of casein proteins that can be used as wall materials for encapsulation, the type of core substances that are encapsulated, the reported size, the encapsulation efficiency, and the characterization methods. It can be assumed that each modification of the casein micelle such as the type of casein, active substance, the proportion of substance, and methods used to encapsulate can produce different results in terms of size, morphology and stabilization. These characterization plays a key role in understanding the physicochemical properties of casein encapsulates. In this review the factors that affect the encapsulation with casein as wall material and the characterization methods are presented.
KEYWORDS:
INTRODUCTION
Casein is the principal milk protein, and calcium phosphate forms casein micelles .[1] Casein is a conglomerate of four proteins identified as α-casein (αs-1 and αs-2), β-casein and ĸ-casein, all of which have similar molecular weights of approximately 24 kDa but differ in their amino acid sequences and in their interactions with hydrophobic and hydrophilic substances .[Citation2,Citation3] Since 2014, casein has been proposed as a raw material for the formation of micro and nano capsules because of its high affinity for hydrophobic substances and is Generally Recognized as Safe (GRAS) substance [Citation4] .Casein has been used to encapsulate different substances in the food industry. Examples of lipophilic organic substances include: omega-3 fatty acids, [Citation5] β-carotene, [Citation1,Citation6,Citation7] fish oil, [Citation8] curcumin, [Citation9–12] vitamin A, vitamin D, [Citation13,Citation14], vanillin, [Citation15] eugenol, [Citation16] triclosan, [Citation17] thymol, [Citation18] celecoxib, [Citation19] emodin, [Citation20] sunflower oil, [Citation21] resveratrol, [Citation22,Citation23] hydroxycamptothecin, [Citation24] quercetin, [Citation9] blueberry anthocyanins, [Citation2] naringenin, [25] isoflavone, [Citation25] rutin[Citation4] and linoleic acid[Citation26]; and hydrophilic organic substances such as: vitamin C[Citation27] and phenolic compounds .[Citation28]
On the other hand, nanoparticles of inorganic substances have been encapsulated using caseins, such as iron oxide, [Citation29] calcium carbonate, [Citation30] zinc oxide, [Citation31] silver, gold and copper(II) oxide .[Citation32] Previous studies have discussed the interactions between caseins and core materials in the formation of the micelle structure during the process of encapsulation and their effects on the release of the core material, [Citation33] other studies have explored the use of milk proteins in general for the delivery of nutraceuticals, [Citation34] or the differences among types of casein as a wall material .[Citation35] This review aimed to compare the current state-of-the-art of nano-encapsulation techniques to assess the effects of the type of casein on the physicochemical characteristics, such as size, encapsulation efficiency and stability of the capsule. describes the heterogeneous works that were consulted to identify the factors that have been studied in the usage of casein to obtain micro and nano-particles with different active substances.
Table 1. Extract of the type of capsules formed with casein, their main findings, and methods of characterization of recent literature
TYPES OF CASEINS
Casein is the main protein present in all types of milk. In fact, casein refers to four types denoted as αs1, αs2, β and ĸ that are fractions obtained separately by ion-exchange chromatography by changing the pH of elution .[Citation43] Molecular weight and composition are similar among caseins; however, they differ in their amino acid sequences, thus resulting in differences between their isoelectric points. In general, αs1 and β are the main caseins in terms of relative abundance, while αs2 and ĸ are of interest because of their sulfhydryl groups .[Citation43] Caseins, together, have an isoelectric point of pH 4.6 and expose their hydrophobic residues in alkaline aqueous solution, [Citation12] however at a calcium concentration of 6 mM at 30°C, these precipitates .[Citation43]
Casein micelles are globular colloids that carry calcium phosphate, and their sizes are heterogeneous between 50 and 500 nm .[Citation44] Furthermore, caseinates refer to commercial caseins that had been obtained from skimmed milk treated with acids, re-solubilized under alkaline conditions, dried, and are easily dissolved in water .[Citation6] Sodium caseinate, potassium caseinate and ammonium caseinate have similar physicochemical characteristics and can produce translucent, straw-colored and viscous solutions, whereas calcium caseinate solutions are turbid because they form larger aggregates .[Citation43] Finally, casein hydrolyzates are fractions of caseins and do not form capsules .[Citation45,Citation46]
CAPSULES OF CASEINS
Casein, casein α(s1 and s2), casein β, casein micelles, and caseinates have been used to obtain capsules for different purposes, principally for the protection and delivery of functional substances. shows the plotted data collected from recent literature on casein as a wall material, where each symbol is related to the type of casein used for encapsulation. Casein micelles and caseinates are the most extended forms of casein encapsulation owing to their ability to form suspensions and commercial availability. Capsules formed of casein micelles are the larger ones, which can be obtained using milk powder, skimmed milk, and commercial casein. It is possible that the authors used casein and casein micelles indistinctly, considering the possibility of taking casein micelles when milk (powder or skimmed) was used to obtain the capsules, or casein when casein powder was used as the wall material.
Figure 1. Capsule size and encapsulation efficiency reported by recent literature using different type of casein of encapsulation. Points are the main value and bars shows the minimum and maximum data reported
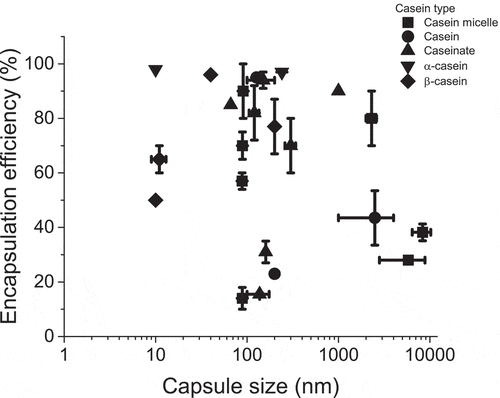
Nogueira[Citation28] used raw skimmed milk microfiltrated with a membrane pore size of 0.1 mm of pore size to obtain casein micelles, and a casein micelle suspension (4.0% w/w) was cross-linked with transglutaminase at pH 7 and mixed with phenolic extract and then dried by spray drying to obtain capsules of 3 to 25 μm of particle size; these capsules showed a significant reduction in the antioxidant activity of the phenolic compounds extracted from jaboticaba (Plinia jaboticaba). Yan[Citation27] used commercial casein suspended (10% w/w) and mixed dropwise with vitamin C. Then, the rennet solution homogenized with oil was added and the casein was emulsified at 6000 rpm, and later centrifuged to remove the oil; and the aqueous phase was dried using maltodextrin and Pluronic©. Vitamin C was loaded in 28.7%, and the casein micelles in aqueous solution were within the size range of 4 to 25 μm with an average of 14.8 μm, and the dried capsules ranged from 4 to 8 μm with an average of 5.8 μm. Yang[Citation20] used micro-filtrated skimmed milk treated with rennet solution, and it was spread dried and then the microcapsules dispersed in water (2 g/L) at 6.8 pH and emodin was dropwise, mixed and ultrasonicated, the dried capsules had a size of 5 μm and raisin-like shapes with a tendency to had larger size in function of emodin concentration, authors founded similarities in raisin-like shapes to coffee-casein complex[Citation47] with 10 μm of average size that can be attributed to casein state, micellar casein and spray dry conditions.
It is worth emphasizing that, in fact, the particle size is very heterogeneous across the cited studies, and not all the authors used the same methodology for particle size measurement, which has an additional impact on the comparison and interpretation of results. In general, when micellar casein is used to obtain capsules, the mean particle size is within the range of 3 to 10 μm. Moeller et al.[Citation14] used pasteurized raw cow’s milk to generate casein micelles. First, milk was micro-filtered and diafiltered three times. Then, it was diluted to 3.5% in water and cooled at 2°C; the pH was adjusted to 5.5 and vitamin D was added as the core material. Finally, the mixture was ultrafiltered and spray-dried. The loaded material was 87.9% of the capsule size and the particle diameter was 100 nm with little difference in variation size between each step of the process indicating a stable structure. Similar results were reported by the same authors, [Citation48] but they observed changes in structures between the casein micelles obtained from skimmed milk and those obtained from casein micelles from the washed retentate. Furthermore, under the same conditions, different lipophilic substances were encapsulated and it was found that the loading efficiency was higher for vitamin D and β-carotene (in the range of 80 to 97%) than for free docosahexaenoic acid (in the range of 13% to 51%), and docosahexaenoic acid bound to triglycerides was in the range of (5 to 9.17%), clearly demonstrating that the type of loaded substance itself is a factor in the loading efficiency. On the other hand, particle size distributions were similar among the different types of nanocapsules, which indicated that the method of encapsulation, the type of casein, and spray drying conditions define the morphology and particle size of nanocapsules around 90 nm, but also capsules within the ranges of 1.5–6.2 μm 6.7–36.2 μm.
Sodium caseinate is the result of adding NaOH to acid casein; it can form protein-ligand complexes and casein micelles .[Citation37] Sodium caseinates are soluble in water and have a lower moisture content, and higher ash content than casein .[Citation43] To form capsules with sodium caseinate is sufficient to form dispersions in the range from 2 to 5% (w/w) in water, and the core substance is added, mixed, and then acidified to a pH of 6. The solution can then be centrifuged, spray-dried[Citation6] or lyophilized .[Citation4] Some modifications to this process have been made, but the formation of capsules with sodium caseinate is faster and more feasible than using casein from microfiltrated skimmed milk or casein fractions. Sodium caseinates form capsules in the size range of 60–200 nm, and at higher phosphate concentrations, there is an increase in the number of larger particles to form aggregates that can reach 26 μm in size .[Citation13] The encapsulation efficiency tends to increase at higher amounts of core substance in the mixture and at higher lipophilic affinity; for example, when β-carotene is loaded from 2 to 5%, the encapsulation efficiency increases from 27 to 35%, [Citation6] a loaded substance such as α-tocopherol reaches 92% in encapsulation efficiency and only 40 to 60% if the core substance is resveratrol .[Citation37] Malekhosseini[Citation38] found that efficiency encapsulation is higher at a pH lower than 6.7, for capsules of 67–129 nm sodium caseinate capsules loaded with epigallocatechin gallate and folic acid.
β-casein with a molecular mass of 24 kDa and an amphiphilic primary structure, it has been used to encapsulate substances, being the only casein that shows temperature and concentration-dependent self-assembling behavior .[Citation3] β-casein is usually acquired with a purity of 90% and solutions higher than the critical micellar concentration at 6.8 pH are needed. To form the capsules, a β-casein dispersion (1 to 2% w/w) was mixed with MgCl2, ethylene glycol, tetra-acetic acid, and NaCl, and the core substance was celecoxib, which was added dropwise to the protein dispersion and the solution was lyophilized to obtain capsules with a particle size of 16 nm, which did not change significantly before or after lyophilization and rehydration .[Citation19] A similar size, in the range of 6 to 11 nm on average, was obtained by quenching cis and trans resveratrol with an encapsulation efficiency dependent on the concentration of resveratrol used in the dropwise step to a solution of β-casein at pH 7.4 .[Citation22] Capsules of β-casein tend to have sizes of less than 300 nm, but particle sizes can be affected by pH, NaCl concentration and the addition of Genipin as a crosslinking agent, resulting in a population of particles ranging in size from 4 nm to 10 μm to encapsulate naringenin .[Citation36] Remarks from these studies are that the capsules obtained with β-casein can reach the smallest particle size in comparison to other casein particles and that the encapsulation efficiency and average particle size are dependent on the amount of loaded substance.
There is not enough data about capsules obtained from α-casein; however, due to the similarities with β-casein, it is possible that their behavior is similar. Dezhamapanah[Citation39] compared α and β casein in the encapsulation of dipyridamole and it was found that the binding interactions were similar. Interestingly, a study on the design of non-viral vectors for gene delivery, proposed a combination of α-casein and chitosan to prepare nanoparticles for this purpose. These were prepared by combining the DNA with the casein solution and adding it to a chitosan solution (95% deacetylated) in an acetate buffer under vigorous agitation. The particles successfully adsorbed DNA and were highly stable. Moreover, the transfection rates of COS-7 cells were positive after a 72 h incubation period, especially with larger particle sizes (741 ± 33 nm) corresponding to a 3.5:1 nanoparticles-to-DNA ratio. Even though effectiveness must be improved, the authors consider the afore mentioned system to be potentially useful for more advanced applications, which include DNA vaccines and gene therapy that highly utilize these delivery tools .[Citation49]
Although, there are no significant differences in the encapsulation process, there are many possibilities for the encapsulation of substances with casein starting with the type of casein, the pH required for capsule formation and the core substance, as well as the addition of substances such as phosphates that induce the formation of aggregates that increase the particle size, or the use of caseinates or β-casein to assess particle size smaller than 200 nm.
METHODOLOGIES OF ENCAPSULATION
Homogenization and coacervation
Generally, obtaining casein capsules relies on the physicochemical interactions between the loaded substance and casein. However, some steps can be performed with the aim to increase their interactions, such as homogenization or coacervation. Homogenization is effective at ultra-high pressure which forces a fluid with a larger particle size to pass through a homogenizing valve under pressure by small orifice; the outlet fluid has a smaller particle size than the inlet fluid .[Citation50] Homogenization induces disruption and reassociation of casein micelles that sometimes increase micelle size and changes in the viscosity of the fluids .[Citation51] Fluids are subjected to turbulence, cavitation, high pressure, shear stress, and high temperature .[Citation17] Homogenization is not indispensable to form casein micelles, however, Ye & Harte[Citation52] clearly demonstrated that homogenization reduces particle size and precipitation of casein micelles-hydroxypopyl cellulose.
On the other hand, coacervation is a promising technique to encapsulate substances modifying the medium in which polymers are dispersed. There are simple and complex coacervation techniques, they depend on how many polymers are involved in the process that basically consists of equilibrating the electrostatic charges around the core (active substance) .[Citation53] Coacervation refers to the technique in which phase separation occurs when oppositely charged polyelectrolytes are electrically balanced in aqueous solutions producing microcapsules that are water-insoluble, depending on a set of conditions such as pH, charge density on the polymers, colloid concentration, ionic strength of the medium, and temperature .[Citation6] It is possible to mix casein, with a coacervating agent; a thickener, and a plasticizer, to promote acylation and thus, a structural change of casein to obtain a homogeneous coacervate .[Citation54] Coacervation with casein includes the addition of natural polymers and crosslinking agents and does not eliminate homogenization. Santinho et al.[Citation55] prepared a coacervation solution with lactate, hydroxypropyl cellulose, and soy lecithin mixed with casein, and it was clearly demonstrated that the active substance (acetaminophen) was entrapped by the casein. Bayomi et al.[Citation56] proposed the used of chitosan in an acid medium that contains the active compound that precipitates with casein in a basic medium, furthermore, formaldehyde was used as a crosslinking agent.
After promoting interaction between casein and hydrophobic substances, homogenization was achieved at 6000 rpm at 45°C and macromolecular mixtures were acidified by adding HCl from pH 10.5 to pH 2 under magnetic stirring, then gum tragacanth under basic medium was added to achieve electrical equivalence point, and finally, genipin was added to rigidize the structure via amide bonds .[Citation7] In general, complex coacervation with casein involves the adding of other polymers to induce the entrapment of core material, and the final addition of a crosslinking agent. In general, caseins are soluble in 3 to 5% w/v in distilled water at pH in the range of 6.8 to 7.8, and at lower pH casein tends to precipitate because of the electrostatic charge of caseins at different pH values. Once casein is completely soluble and dispersed, the core material is added. The core material could be soluble in ethanol, acetone, or oil and then added to the casein solution. Some authors use homogenization at high pressure, [Citation57,Citation58] while others use ultrasonication, [Citation20,Citation23] or simply magnetic stirring .[Citation11]
Precipitation, spray dryness and centrifugation
Once casein has encapsulated an active substance, separation of the medium is necessary for both preservation and characterization. If casein encapsulates precipitate, a separation via membrane or even decantation could be enough to separate it from the medium, otherwise spray dryness is the most helpful method to separate an aqueous medium. It can be expected that casein obtained by precipitation is larger than that obtained by spray dryness; however, there is no clear evidence on which dry methodology is related with to particle size: when precipitation is used the particle size ranges from 125 nm[] to 500 μm, [Citation29] meanwhile in the use of spray drying the particle size ranges from 100 nm[Citation40] to 25 μm[Citation59]; those differences can be explained in terms of multifactorial effects, since the type of the casein, the proportion casein/core, the pH of the medium, the solvent ionic strength, the active material, the use of co-polymers; in the spray-drying process, affects the nozzle type, pressure, velocity, viscosity and solid content .[Citation60,Citation61] In freeze-drying was identified that ultrasound decreases the size of the particle from 1.25 μm to 1.1 μm but further evidence is needed[Citation62]; and for simple evaporation, homogenization decreases the size of the particle from 15 μm to 3.6 μm .[Citation17] Centrifugation has been also used to separate the medium from casein capsules with a particle size among 40 to 721 nm, [Citation48] it could be expected that factors like time, pH, centrifugation method, ionic strength, g-force are going to affect the particle size of casein capsules separated by centrifugation, but we did not find specific evidence .[Citation63] From the technological point of view, an ideal capsule is the one that has improved characteristics of chemical stability and controlled release under specific conditions[Citation64] and are not characteristics necessarily related to aspects like particle size, size distribution, or morphology of the encapsulates. More specific reviews will be done in the future for every system of casein-core material.
METHODS OF CHARACTERIZATION OF CASEIN CAPSULES
Techniques to Assess Particle Size and Morphology
Characterization of casein capsules is key to identify the physicochemical profile to establish alternatives to improve their performance for a specific purpose. The current tendency of research is focused on reducing the particle size of casein capsules, because, theoretically, a higher proportion surface/volume will improve the stability of the suspensions, but also, it is possible to obtain the contrary effect due to the enhancement of the Van der Waals attractive forces[Citation65]; either way to know particle size is important to classify at least among casein capsules according to their size that is expected to be among 50 and 600 nm with average in 150 nm .[Citation6] However, the particle size of casein capsules depends also on the technique used to determine it.
For example, scanning electron microscopy (SEM) and field emission scanning electron microscopy (FESEM) are used to obtain micrographs of the capsules, to obtain information on the shape and size as well as the roughness or smoothness of the capsules, and, as it can be seen in a) an b), it is possible to identify various capsule morphologies that have been reported, they are agglomerated and form a network or spherical particles with wrinkles. SEM is useful to identify the morphology of the casein obtained and to know if the particles were aggregated, but it is important to consider that manipulation of the sample and the sputtering with gold can also affect the quality of the image obtained.
Figure 2. SEM and AFM images of casein capsules taken from a), [Citation66, 76] b) and c) [Citation11] [Citation59] and TEM images of casein capsules taken from d)[Citation76],e)[11] and f) , [Citation38] [][] [Citation6]
![Figure 2. SEM and AFM images of casein capsules taken from a), [Citation66, 76] b) and c) [Citation11] [Citation59] and TEM images of casein capsules taken from d)[Citation76],e)[11] and f) , [Citation38] [][] [Citation6]](/cms/asset/2c8d122d-7ab6-4755-bd6f-2bddfd197db0/ljfp_a_1953069_f0002_oc.jpg)
Transmission electron microscopy uses an electron beam to interact with the sample, providing information at the atomic scale, such as the crystal structure and granularity, however, protein capsules, such as casein, do not scatter the electron beam efficiently, and thus, capsules require metal coating or staining procedures[Citation67] Also, the sample must be dried before analysis, which may cause changes in the size and agglomeration .[Citation68] TEM can be performed if the capsules obtained are on the nanoscale and, it is useful to observe with more detail how capsules were formed, [Citation15] some observations have been described, for example, that the spherical casein nanoparticles have serrated edges indicating the protruding of ĸ-casein fraction, [Citation38] and that indeed, casein is a cluster with randomly grown branches with fractal characteristics with stelliform geometry, and each cluster is comprised by a number of small cylindrical units, [Citation6] a description that is closer to the structure of a protein with multiple domains, in which core substance had to be absorbed into specific domains of the polymeric chains of the casein, either way, particles can be measured and geometry can be assumed as spheric.
Atomic force microscopy (AFM) relies on tapping a particle with the force of a cantilever, so resolution may be limited with flexible structures, such as casein capsules, which can move under the force of the microscope .[Citation69] AFM has been used to measure the particle size and topography of the obtained material[Citation18,Citation40,Citation41]; however, the information obtained is not well understood, but it is possible to use different probes to characterize the mechanical[Citation70] and electrical characteristics of casein capsules.
Dynamic light scattering (DLS) measures the hydrodynamic diameter of particles in a suspension by intensity fluctuations on scattered light, thus gives a fundamentally different measure of particle size in comparison to the previously mentioned microscopies. Moreover, since biological molecules interact in complex ways with their environment, produce wider molecular disturbances and translation which can dramatically affect the motion of the particles under Brownian diffusion and agglomeration. However, in comparison to microscopies, DLS provides a wider structural analysis overview of the sample, since the technique allows scanning of millions of capsules at once[Citation67] and, moreover, it is less time consuming and expensive technique. Static light scattering (SLS) measures the time-averaged intensity of scattered light that allows calculation of the mass of a molecule .[Citation66] So DLS and SLS not only determine the size or mass of the particles, but also provide information on the particle size distribution and are sensible for detecting particles with multiple orders of magnitude. By these techniques, it can be detected the amount and distribution of particles of different sizes and these can be expressed with a simple average and standard deviation, but also with either the D-value related with the cumulative distribution and span; or by the moment-ratio notation expressed in the form dp,q that serves to know the weight in proportion among the particles of different size. However, these techniques also have some limitations depending on the sample, for instance, stability and morphology problems after dilutions; the fact that measurements are by volume in comparison to microscopy, which is by number; or that has partial resolution with polydisperse samples .[Citation68] Another important fact of DLS to consider is that it assumes a sphere diameter with the same translational diffusion coefficient as the sample, and thus, measurement results cannot be compared to microscopy or to other light techniques, for example, laser diffraction (LD) or X-ray sedimentation, since measurements are not equivalent. LD particle size analysis measures a hard spherical diameter that is a sphere with the same cross-sectional area as the same diffraction pattern of the sample. X-ray sedimentation uses Stokes’ Law and Lambert-Beer Law principles to assess particle sizes .[Citation71] Thus, the main consideration here is that each technique is unique and using one or another for size particle analysis requires several considerations. Also, techniques are useful but not interchangeable due to the differences in the methodology, treatment of the instrument signals and the angle and length of the measurement .[Citation69] DLS examples are presented in a, where naringenin was encapsulated on β-casein and it is observed an increase in the particle size at higher proportion of naringenin.
Figure 3. Excerpts based on typical DLS of capsules of a) casein- vitamin D2, [Citation56] , DLS of β-casein with different proportions of naringenin[Citation72];b) FTIR of casein encapsulating metformin[Citation73], casein[Citation7]; c)DRX of casein capsules with β-caroten [7]; and d) Raman spectroscopy of casein compared with pectin[Citation74] [Citation7]
![Figure 3. Excerpts based on typical DLS of capsules of a) casein- vitamin D2, [Citation56] , DLS of β-casein with different proportions of naringenin[Citation72];b) FTIR of casein encapsulating metformin[Citation73], casein[Citation7]; c)DRX of casein capsules with β-caroten [7]; and d) Raman spectroscopy of casein compared with pectin[Citation74] [Citation7]](/cms/asset/18e8b74c-0bba-435b-8880-49d0a2e307f8/ljfp_a_1953069_f0003_oc.jpg)
Chemical interaction: FTIR, RAMAN, Z-Potential, UV-Vis, TGA, DSC, XPS
Z-potential measurement is a common technique for describing the surface charge of casein capsules, [Citation75] is measured in aqueous suspensions and is dependent on pH and for casein has been measured at pH around 6 and 6.6 giving a Z-potential between −14 mV[Citation58] to −31 mV[Citation41]; the more negative the Z-potential, the better the electrostatic repulsion among the particles, resulting in a more stable suspension.
XPS is one of the most sensitive and accurate surface analysis techniques in which it can be detected different atom oxidation states and their proportions .[Citation76] X-ray (EDX) studies have been performed to confirm the presence of inorganic materials encapsulated by casein, such as Fe3O4, [Citation28] CaFe2O4, [Citation74] Ag, Au and CuO .[Citation31]
Fourier-transform infrared spectroscopy (FTIR) has been used to understand the chemical interactions between casein and the loaded substance. On and , it is shown the typical FTIR of casein, it has an absorption band near 3300 cm−1 related to O-H stretching band and a at 2925–2960 cm−1 due to stretching of CH3 and CH2, the signal at 1655 cm−1 is due to C = O stretch or amide I band and 1532–1536 cm−1 to N-H bending or amide II, [Citation12,Citation20,Citation23] once the absorption bands of casein are evaluated their spectral shifting are attributed to hydrophobic interactions[Citation39] but there is no clear evidence if spectral shifting is common with casein encapsulation; however, no shifting is regularly observed; characteristic bands of loaded substances tend to disappear; some authors have proposed that a reduction in the stretching and bending of loaded substance bonds is due to the transformation from the crystalline to amorphous state, [Citation23] but this assumption does not fit with the observed XRD in which loaded substance is identified with peaks related to a crystalline state. Confocal Raman microscopy could have more advantages in the study of casein microparticles than FTIR because it is possible to analyze the distribution of components in spatial dimension, [Citation77] furthermore, on , it is demonstrated that Raman spectroscopy is sensible in differentiation between two biopolymers like casein and pectin.
Termogravimetric analysis (TGA) is useful to evaluate thermostability of the capsules and it is possible to identify the protection of the casein capsule if decomposition temperature is increased, [Citation15] through differential scanning calorimetry (DSC) can be identified exothermic peaks of casein at 57.1°C and glass transition at 2.3°C, [Citation78] furthermore, the oxidation process can be studied by changing the inlet gas. Together TGA-DSC thermal stability and thermal transitions can be identified for the casein capsules. Finally, UV-Vis spectrophotometry is used to measure the concentration and presence of loaded substances through the Lambert-Beer equation, either to measure the encapsulation efficiency or the kinetics of delivery in the liquid phase.
Crystalline structure
When crystalline structures are present in the casein capsules, diffraction patterns can be observed in the selected area electron diffraction (SAED) and X-ray diffraction (XRD) .[Citation31] Diffraction is attributed to the crystal structure of the materials; casein has an amorphous structure and the X-ray diffraction (XRD) of casein does not show any diffraction pattern (); however, peaks at 2θ = 31° and 45° are observable when treated with NaOH, [Citation4] and some loaded substance can be identified with wide peaks, which is useful for identifying the presence of the loaded substances, [Citation7] and the peaks are well defined when the loaded material is crystalline .[Citation31] Finally, to summarize the way in which some characterization methods may affect, the summarize why there may be differences in the results and interpretations of the casein capsules that were analyzed in this review.
Table 2. Factors of some characterization methods that affects the results and interpretation of casein capsules
CONCLUSION
Casein is a milk protein comprised of four subtypes αs1-, αs2-, β- and κ-casein, whose functional properties differ and solubility is highly dependent on pH. This literature review has highlighted the application of casein as a useful material for the encapsulation of various substances with heterogeneous physicochemical properties, allowing for protection against degradation of the substance, maintaining fragrance, designing control release under specific conditions, providing textures to mixtures of components, and many other useful properties. Among the main advantages of caseins are not only their low cost or high abundance but also stability, biocompatibility, and biodegradability and even is reported the self-assembling properties of these proteins; therefore, are applications of caseins in encapsulation of big interest to the food and pharmaceutical industries. Several encapsulation methods have been described and compared to provide strategies for designing and improving capsules for potential new applications, based on their ease of production, range of size and dispersion of the capsules, amount of core substance, and the active ingredient that is encapsulated. It is a remark to identify the use and application of the characterization techniques as the size, structure, and chemical interactions determine the stability and processability. In the review, is observed that advantages and disadvantages of size measurement techniques depend on the nature of the size, mass, shape, and mechanical properties of the capsules, but also, the size resolution of the instrument, and the theoretical principle behind any measurement. Chemical interactions should be characterized to measure stability, composition, concentration, leaking or degradation, polymorphism aggregation, among others. These changes may affect the final performance and activity of structures and must be understood and controlled to adequately exploit the properties of the casein protein encapsulates in the food and pharmaceutical fields. Consequently, this manuscript provides recommendations for future works to better understand which techniques to apply and what factors can influence the particle and structural instrumental analysis.
Acknowledgments
We want to thank CONACYT for the economic support through SNI. No funding was received to assist with the preparation of this manuscript.
References
- Moeller, H.; Martin, D.; Schrader, K.; Hoffmann, W.; Lorenzen, P. C. Native Casein Micelles as Nanocarriers for β-carotene: PH-and Temperature-induced Opening of the Micellar Structure. Int. J. Food Sci. Technol. 2017, 1–9. DOI: https://doi.org/10.1111/ijfs.13387..
- Lang, Y.; Gao, H.; Tian, J.; Shu, C.; Sun, R.; Li, B.; Meng, X. Protective Effects of α-casein or β-casein on the Stability and Antioxidant Capacity of Blueberry Anthocyanins and Their Interaction Mechanism. LWT - Food Sci. Technol. 2019, 115, 108434. DOI: https://doi.org/10.1016/j.lwt.2019.108434..
- Romano, D.; Bonacucina, G.; Cespi, M.; Bonazza, F.; Filippo, G.; Pucciarelli, S.; Polzonetti, V.; Attarian, L.; Polidori, P.; Vincenzetti, S. A Comparison among β -caseins Purified from Milk of Different Species: Self- Assembling Behaviour and Immunogenicity Potential. Colloids Surf. B Biointerfaces. 2019, 173, 210–216. DOI: https://doi.org/10.1016/j.colsurfb.2018.09.079..
- Rashidinejad, A.; Loveday, S. M.; Jameson, G. B.; Hindmarsh, J. P. Rutin-casein Co-precipitates as Potential Delivery Vehicles for Flavonoid Rutin. Food Hydrocoll. 2019, 96, 451–462. DOI: https://doi.org/10.1016/j.foodhyd.2019.05.032..
- Mora-Gutierrez, A.; Attaie, R.; Kirven, J. M.; Jr, H. M. F. Cross-linking of Bovine and Caprine Caseins by Microbial Transglutaminase and Their Use as Microencapsulating Agents for N −3 Fatty Acids. Int. J. Food Sci. Technol. 2014, 49, 1530–1543. DOI: https://doi.org/10.1111/ijfs.12450..
- Jarunglumlert, T.; Nakagawa, K.; Adachi, S. Influence of Aggregate Structure of Casein on the Encapsulation Efficiency of B-carotene Entrapped via Hydrophobic Interaction. Food Struct. 2015, 5, 42–50. DOI: https://doi.org/10.1016/j.foostr.2015.05.001..
- Jain, A.; Thakur, D.; Ghoshal, G.; Katare, O. P.; Shivhare, U. S. Characterization of Microcapsulated β-Carotene Formed by Complex Coacervation Using Casein & Gum Tragacanth. Int. J. Biol. Macromol. 2016, 87, 101–113. DOI: https://doi.org/10.1016/j.ijbiomac.2016.01.117..
- Li, J.; Xiong, S.; Wang, F.; Regenstein, J. M.; Liu, R. Optimization of Microencapsulation of Fish Oil with Gum Arabic/Casein/Beta-Cyclodextrin Mixtures by Spray Drying. J. Food Sci. 2015, 80, 1445–1452. DOI: https://doi.org/10.1111/1750-3841.12928..
- Ghayour, N.; Mohammad, S.; Hosseini, H.; Eskandari, M. H.; Esteghlal, S.; Nekoei, A.; Gahruie, H. H.; Tatar, M.; Naghibalhossaini, F. Nanoencapsulation of Quercetin and Curcumin in Casein-based Delivery Systems. Food Hydrocoll. 2018, 87, 349–403. DOI: https://doi.org/10.1016/j.foodhyd.2018.08.031..
- Isabel, M.; Neves, L.; Desobry-banon, S.; Tuler, I.; Desobry, S.; Petit, J. Encapsulation of Curcumin in Milk Powders by Spray-drying: Physicochemistry, Rehydration Properties, and Stability during Storage. Powder Technol. 2019, 345, 601–607. DOI: https://doi.org/10.1016/j.powtec.2019.01.049..
- Purushothaman, B. K.; M.h. S,; Maheswari, P. U.; Sheri, K. M. M. Magnetic Assisted Curcumin Drug Delivery Using Folate Receptor Targeted Hybrid Casein-calcium Ferrite Nanocarrier. J. Drug Deliv. Sci. Technol. 2019, 52, 509–520. DOI: https://doi.org/10.1016/j.jddst.2019.05.010.
- Xu, G.; Li, L.; Bao, X.; Yao, P. Curcumin, Casein and Soy Polysaccharide Ternary Complex Nanoparticles for Enhanced Dispersibility, Stability and Oral Bioavailability of Curcumin. Food Biosci. 2020, 35, 100569. DOI: https://doi.org/10.1016/j.fbio.2020.100569.
- Loewen, A.; Chan, B.; Li-chan, E. C. Y. Optimization of Vitamins A and D3 Loading in Re-assembled Casein Micelles and Effect of Loading on Stability of Vitamin D3 during Storage. Food Chem. 2018, 240, 472–481. DOI: https://doi.org/10.1016/j.foodchem.2017.07.126.
- Moeller, H.; Martin, D.; Schrader, K.; Hoffmann, W.; Chr, P. Spray-or Freeze-drying of Casein Micelles Loaded with Vitamin D2: Studies on Storage Stability and in Vitro Digestibility. LWT - Food Sci. Technol. 2018, 97, 87–93. DOI: https://doi.org/10.1016/j.lwt.2018.04.003.
- Zhang, Y.; Ma, J.; Xu, Q. Polyelectrolyte Complex from Cationized Casein and Sodium Alginate for Fragrance Controlled Release. Colloids Surf. B Biointerfaces. 2019, 178, 439–444. DOI: https://doi.org/10.1016/j.colsurfb.2019.03.017.
- Cohen, Y.; Ish-shalom, S.; Segal, E.; Nudelman, O.; Shpigelman, A.; Livney, Y. D. The Bioavailability of Vitamin D3, a Model Hydrophobic Nutraceutical, in Casein Micelles, as Model Protein Nanoparticles: Human Clinical Trial Results. J. Funct. Foods. 2017, 30, 321–325. DOI: https://doi.org/10.1016/j.jff.2017.01.019.
- Roach, A.; Dunlap, J.; Harte, F. Association of Triclosan to Casein Proteins through Solvent-Mediated High-Pressure Homogenization. J. Food Sci. 2009, 74, 23–29. DOI: https://doi.org/10.1111/j.1750-3841.2009.01048.x..
- Pan, K.; Chen, H.; Davidson, P. M.; Zhong, Q. Thymol Nanoencapsulated by Sodium Caseinate: Physical and Antilisterial Properties. J. Agric. Food Chem. 2014, 62, 1649–1657. DOI: https://doi.org/10.1021/jf4055402.
- Danino, D.;. Celecoxib Encapsulation in β ‑ Casein Micelles: Structure, Interactions, and Conformation. Langmuir. 2015, 31, 7183–7192. DOI: https://doi.org/10.1021/acs.langmuir.5b01397..
- Yang, M.; Wei, Y.; Ashokkumar, M.; Qin, J.; Han, N.; Wang, Y.; Yang, M.; Wei, Y.; Ashokkumar, M.; Qin, J. Effect of Ultrasound on Binding Interaction between Emodin and Micellar Casein and Its Microencapsulation at Various Temperatures. Ultrason. Sonochem. 2019, 62, 104861. DOI: https://doi.org/10.1016/j.ultsonch.2019.104861..
- Basurto, M. A. S.; Mart, A. C.; Casta, E. Study of the Interactions Occurring during the Encapsulation of Sesamol within Casein Micelles Reformed from Sodium Caseinate Solutions. J. Food Sci. 2018, 1–10. DOI: https://doi.org/10.1111/1750-3841.14293..
- Cheng, H.; Dong, H.; Liang, L. A Comparison of β -casein Complexes and Micelles as Vehicles for Trans -/cis - Resveratrol. Food Chem. 2020, 330, 127209. DOI: https://doi.org/10.1016/j.foodchem.2020.127209..
- Khan, M. A.; Fang, Z.; Cheng, H.; Gao, Y.; Deng, Z. Encapsulation and Protection of Resveratrol in Kafirin and Milk Protein Nanoparticles. Int. J. Food Sci. Technol. 2019, 54, 2998–3007. DOI: https://doi.org/10.1111/ijfs.14212.Khan.
- Cheng, Z.; Li, R.; Qin, J.; Lu, W.; Wang, J. Menthol-modified Casein Nanoparticles Loading 10-hydroxycamptothecin for Glioma Targeting Therapy. Acta Pharm. Sin. B. 2019, 9, 843–857. DOI: https://doi.org/10.1016/j.apsb.2019.01.006..
- Rahman, A.; Vasudevan, T. Encapsulation of Isoflavone with Milk, Maltodextrin and Gum Acacia Improves Its Stability. Curr Res Food Sci. 2020, 2, 77–83. DOI: https://doi.org/10.1016/j.crfs.2019.12.003.
- Zhuang, F.; Li, X.; Hu, J.; Liu, X.; Zhang, S.; Tang, C. Effects of Casein Micellar Structure on the Stability of Milk Protein-based Conjugated Linoleic Acid Microcapsules. Food Chem. 2018, 269, 327–334. DOI: https://doi.org/10.1016/j.foodchem.2018.07.018..
- Yan, B.; Davachi, S. M.; Ravanfar, R.; Dadmohammadi, Y.; Deisenroth, T. W.; Van Pho, T.; Odorisio, P. A.; Hiremath Darji, R.; Abbaspourrad, A. Lmprovement of Vitamin C Stability in Vitamin Gummies by Encapsulation in Casein Gel. Food Hydrocoll. 2020, 113, 106414. DOI: https://doi.org/10.1016/j.foodhyd.2020.106414.
- Nogueira, C. I. O. H.; Tavares, G. M.; Casanova, F.; Silva, C. R. J.; Rocha, J. C. G.; Stringheta, P. C.; Stephani, R.; Carvalho, A. F. D. E. Cross-linked Casein Micelle Used as Encapsulating Agent for Jaboticaba (Plinia Jaboticaba) Phenolic Compounds by Spray Drying. Int J Diary Technol 2020, 1–6. DOI: https://doi.org/10.1111/1471-0307.12704.
- Masoomeh, E.; Hamid, D.; Mahvash, H. Surface Modification of Super Paramagnetic Iron Oxide Nanoparticles via Milk Casein for Potential Use in Biomedical Areas. J. Biomol. Struct. Dyn. 2020, 39, 977–987. DOI: https://doi.org/10.1080/07391102.2020.1722751.
- Li, Y.; Li, X.; Cao, Z.; Xu, Y.; Gong, Y.; Shi, X. Fabrication of Uniform Casein/CaCO3 Vaterite Microspheres and Investigation on Its Formation Mechanism. Cryst. Growth Des. 2017, 17, 6178–6188. DOI: https://doi.org/10.1021/acs.cgd.7b00306.
- Liu, J.; Hui, A.; Ma, J.; Chen, Z.; Peng, Y. Fabrication and Application of Hollow ZnO Nanospheres in Antimicrobial Casein-based Coatings. Int. J. Appl. Ceram. Technol. 2016, 1–7. DOI: https://doi.org/10.1111/ijac.12635.
- Rakhimol, K. R.; Thomas, S.; Kalarikkal, N.; Jayachandran, K. Casein Mediated Synthesis of Stabilized Metal/metal-oxide Nanoparticles with Varied Surface Morphology through pH Alteration. Mater. Chem. Phys. 2020, 246, 122803. DOI: https://doi.org/10.1016/j.matchemphys.2020.122803.
- H. Chen, H. Wooten, L. Thompson, and K. Pan. Nanoparticles of casein micelles for encapsulation of food ingredients,” Biopolymer Nanostructures for Food Encapsulation Purposes.2019, 1, 39–68. https://doi.org/https://doi.org/10.1016/B978–0–12–815663–6.00002–1
- Abd El‐Salam, M. H.; El‐Shibiny, S. Formation and Potential Uses of Milk Proteins as Nano Delivery Vehicles for Nutraceuticals: A Review. Int. J. Dairy Technol. 2012, 65(1), 13–21. DOI: https://doi.org/10.1111/j.1471-0307.2011.00737.x.
- Rasmussen, L. K.; Højrup, P.; Petersen, T. E. Disulphide Arrangement in Bovine Caseins: Localization of Intrachain Disulphide Bridges in Monomers of Kappa- and Alpha S2-casein from Bovine Milk. J. Dairy Res. 1994 Nov, 61(4), 485–493. doi:https://doi.org/10.1017/s0022029900028417..
- Li, M.; Wang, K.; Wang, Y.; Han, Q.; Ni, Y.; Wen, X. Effects of Genipin Concentration on Cross-linked β -casein Micelles as Nanocarrier of Naringenin: Colloidal Properties, Structural Characterization and Controlled Release. Food Hydrocoll. 2020, 108. DOI: https://doi.org/10.1016/j.foodhyd.2020.105989..
- Cheng, H.; Fan, Q.; Liu, T.; Liang, L. Co-encapsulation of α -tocopherol and Resveratrol in Oil-in-water Emulsion Stabilized by Sodium Caseinate: Impact of Polysaccharide on the Stability and Bioaccessibility. J. Food Eng. 2020, 264, 109685. DOI: https://doi.org/10.1016/j.jfoodeng.2019.109685..
- Malekhosseini, P.; Alami, M.; Khomeiri, M.; Esteghlal, S.; Nekoei, A.-R.; Hosseini, S. M. H. Development of Casein- Based Nanoencapsulation Systems for Delivery of Epigallocatechin Gallate and Folic Acid. Food Sci. Nutr. 2019, 00, 1–9. DOI: https://doi.org/10.1002/fsn3.827.
- Dezhampanah, H.; Esmaili, M.; Hasani, L. Milk Caseins as Useful Vehicle for Delivery of Dipyridamole Drug. J. Biomol. Struct. Dyn. 2017, 1102, 0–1. DOI: https://doi.org/10.1080/07391102.2017.1329100..
- Chen, H.; Zhang, Y.; Zhong, Q. Physical and Antimicrobial Properties of Spray-dried Zein–casein Nanocapsules with Co-encapsulated Eugenol and Thymol. J. Food Eng. 2015, 144(93–102), 93–102. DOI: https://doi.org/10.1016/j.jfoodeng.2014.07.021.
- Guan, Y.; Zhong, Q. Encapsulation of Ferulic Acid Ethyl Ester in Caseinate to Suppress Off-flavor Formation in UHT Milk. Food Chem. 2017, 237, 532–537. DOI: https://doi.org/10.1016/j.foodchem.2017.05.140.
- Ouyang, Y.; Chen, L.; Qian, L.; Lin, X.; Fan, X.; Teng, H. Fabrication of Caseins Nanoparticles to Improve the Stability of Cyanidin 3- O - Glucoside. Food Chem. 2020, 317, 126418. DOI: https://doi.org/10.1016/j.foodchem.2020.126418.
- Augustin, M. A.; Oliver, C. M.; Hemar., Y. Casein, Caseinates, and Milk Protein Concentrates. In Dairy Ingredients Food Process, 2011; pp 161–178. Wiley-Blackwell. https://doi.org/http://dx.doi.org/10.1002/9780470959169.ch7
- Ghasemi, S.; Abbasi, S. Formation of Natural Casein Micelle Nanocapsule by Means of pH Changes and Ultrasound. Food Hydrocoll. 2014, 42, 6–11. DOI: https://doi.org/10.1016/j.foodhyd.2013.10.028..
- Sarabandi, K.; Sadeghi, A.; Hamishekar, H. Microencapsulation of Casein Hydrolysates: Physicochemical, Antioxidant and Microstructure Properties. J. Food Eng. 2018, 237, 86–95. DOI: https://doi.org/10.1016/j.jfoodeng.2018.05.036..
- Sarabandi, K.; Sadeghi, A.; Hamishehkar, H. Protection of Casein Hydrolysates within Nanoliposomes: Antioxidant and Stability Characterization. J. Food Eng. 2019, 251, 19–28. DOI: https://doi.org/10.1016/j.jfoodeng.2019.02.004..
- Tan, S.; Ebrahimi, A.; Langrish, T. Controlled Release of Caffeine from Tablets of Spray-dried Casein Gels. Food Hydrocoll. 2019, 88, 13–20. DOI: https://doi.org/10.1016/j.foodhyd.2018.09.038.
- Moeller, H.; Martin, D.; Schrader, K.; Hoffmann, W.; Pargmann, S.; Kurz, J. Comparative Studies of Loading Lipophilic Substances into Casein Micelles and Investigating the Influence of Whey Proteins and Heat Treatment on Loading Stability. Int. J. Dairy Technol. 2018, 70, 1–12. DOI: https://doi.org/10.1111/1471-0307.12535..
- Panão Costa, J.; Carvalho, S.; Jesus, S.; Soares, E.; Marques, A. P.; Borges, O. Optimization of Chitosan-α-casein Nanoparticles for Improved Gene Delivery: Characterization, Stability, and Transfection Efficiency. AAPS Pharm. Sci. Tech. 2019Feb28, 20(3), 132. DOI: https://doi.org/10.1208/s12249-019-1342-y.
- Diels, A. M. J.; Michiels, C. W. High-Pressure Homogenization as a Non-Thermal Technique for the Inactivation of Microorganisms. Crit. Rev. Microbiol. 2006, 32, 201–216. DOI: https://doi.org/10.1080/10408410601023516..
- San Martin-González, M. F.; Roach, A.; Harte, F. Rheological Properties of Corn Oil Emulsions Stabilized by Commercial Micellar Casein and High-pressure Homogenization. LWT-Food Sci. Technol. 2009, 42(1), 307–311. DOI: https://doi.org/10.1016/j.lwt.2008.04.005.
- Ye, R.; Harte, F. High Pressure Homogenization to Improve the Stability of Casein–hydroxypropyl Cellulose Aqueous Systems. Food Hydrocolloid. 2014, 35, 670–677. DOI: https://doi.org/10.1016/j.foodhyd.2013.08.022.
- Timilsena, Y. P.; Akanbi, T. O.; Khalid, N.; Adhikari, B.; Barrow, C. J. Complex Coacervation: Principles, Mechanisms and Applications in Microencapsulation. Int. J. Biol. Macromol. 2019, 121, 1276–1286. DOI: https://doi.org/10.1016/j.ijbiomac.2018.10.144.
- Elzoghby, A. O.; El-Fotoh, W. S. A.; Elgindy, N. A. Casein-based Formulations as Promising Controlled Release Drug Delivery Systems. J. Control. Release. 2011, 153(3), 206–216. DOI: https://doi.org/10.1016/j.jconrel.2011.02.010.
- Santinho, A. J. P.; Ueta, J. M.; Freitas, O.; Pereira, N. L. Physicochemical Characterization and Enzymatic Degradation of Casein Microcapsules Prepared by Aqueous Coacervation. J. Microencapsul. 2002, 19(5), 549–558. DOI: https://doi.org/10.1080/02652040110105391.
- Bayomi, M. A.; Al-Suwayeh, S. A.; El-Helw, A. M.; Mesnad, A. F. Preparation of Casein–chitosan Microspheres Containing Diltiazem Hydrochloride by an Aqueous Coacervation Technique. Pharm. Acta Helv. 1998, 73(4), 187–192. DOI: https://doi.org/10.1016/S0031-6865(98)00020-X.
- Semo, E.; Kesselman, E.; Danino, D.; Y.d.l.. Casein Micelle as a Natural Nano-capsular Vehicle for Nutraceuticals. Food Hydrocoll. 2007, 21, 936–942. DOI: https://doi.org/10.1016/j.foodhyd.2006.09.006..
- Penalva, R.; Esparza, I.; Agüeros, M.; Gonzalez-navarro, C. J.; Gonzalez-ferrero, C.; Irache, P. J. M. Casein Nanoparticles as Carriers for the Oral Delivery of Folic Acid. Food Hydrocoll. 2014, 44, 399–406. DOI: https://doi.org/10.1016/j.foodhyd.2014.10.004.
- Nogueira, M. H.; Ben-Harb, S.; Schmutz, M.; Doumert, B.; Nasser, S.; Derensy, A.; A.,; Peixoto, P. P. Multiscale Quantitative Characterization of Demineralized Casein Micelles: How the Partial Excision of Nano-clusters Leads to the Aggregation during Rehydration. Food Hydrocoll. 2020, 105, 105778. DOI: https://doi.org/10.1016/j.foodhyd.2020.105778.
- Nuyttens, D.; Baetens, K.; De Schampheleire, M.; Sonck, B. Effect of Nozzle Type, Size and Pressure on Spray Droplet Characteristics. Biosyst Eng. 2007, 97(3), 333–345. DOI: https://doi.org/10.1016/j.biosystemseng.2007.03.001.
- Bastan, F. E.; Erdogan, G.; Moskalewicz, T.; Ustel, F. Spray Drying of Hydroxyapatite Powders: The Effect of Spray Drying Parameters and Heat Treatment on the Particle Size and Morphology. J. Alloy. Compd. 2017, 724(586–596), 586–596. DOI: https://doi.org/10.1016/j.jallcom.2017.07.116.
- Yang, M.; Wei, Y.; Ashokkumar, M.; Qin, J.; Han, N.; Wang, Y. Effect of Ultrasound on Binding Interaction between Emodin and Micellar Casein and Its Microencapsulation at Various Temperatures. Ultrason. Sonochem. 2020, 62, 104861. DOI: https://doi.org/10.1016/j.ultsonch.2019.104861..
- Ransmark, E.; Svensson, B.; Svedberg, I.; Göransson, A.; Skoglund, T. Measurement of Homogenization Efficiency of Milk by Laser Diffraction and Centrifugation. Int. Dairy J. 2019, 96, 93–97. DOI: https://doi.org/10.1016/j.idairyj.2019.04.011.
- Wang, N.; Cheng, X.; Li, N.; Wang, H.; Chen, H. Nanocarriers and Their Loading Strategies. Adv. Healthc. Mater. 2019, 8(6), 1801002. DOI: https://doi.org/10.1002/adhm.201801002.
- Mondragon, R.; Enrique, J. J.; Barba, A.; Jarque, J. C. Characterization of Silica–water Nanofluids Dispersed with an Ultrasound Probe: A Study of Their Physical Properties and Stability. Powder Technol. 2012, 224, 138–146. DOI: https://doi.org/10.1016/j.powtec.2012.02.043.
- Chu, B.; Liu, T. Characterization of Nanoparticles by Scattering Techniques. J. Nanopart. Res. 2000, 2, 29–41. DOI: https://doi.org/10.1023/A:1010001822699.
- Dobrovolskaia, M. A.; Patri, A. K.; Zheng, J.; Clogston, J. D.; Ayub, N.; Aggarwal, P.; Neun, B. W.; Hall, J. B.; McNeil, S. E. Interaction of Colloidal Gold Nanoparticles with Human Blood: Effects on Particle Size and Analysis of Plasma Protein Binding Profiles. Nanomedicine. 2009 Jun, 5(2), 106–117. doi:https://doi.org/10.1016/j.nano.2008.08.001..
- Fissan, H.; Ristig, S.; Kaminski, H.; Asbach, C.; Epple, M. Comparison of Different Characterization Methods for Nanoparticle Dispersions before and after Aerosolization. Anal. Methods. 2014, 6, 7324–7334. DOI: https://doi.org/10.1039/C4AY01203H.
- Bryant, G.; Thomas, J. C. Improved Particle Size Distribution Measurements Using Multiangle Dynamic Light Scattering. Langmuir. 1995, 11, 7. DOI: https://doi.org/10.1021/la00007a028.
- Fery, A.; Weinkamer, R. Mechanical Properties of Micro- and Nanocapsules: Single-capsule Measurements. Polymer. 2007, 48, 7221–7235. DOI: https://doi.org/10.1016/j.polymer.2007.07.050..
- Magno, M. C.; Venti, F.; Gaglianone, G.; Perfrancheschi, G.; Romano, E. Grain Size Analysis: A Comparison between Laser Granulometer and Sedigraph. IMEKO International Conference ON Metrology for the sea, 23012, 11–13. Italy, NAPLES.
- Li, M.; Fokkink, R.; Ni, Y.; Mieke Kleijn, J. Bovine Beta-casein Micelles as Delivery Systems for Hydrophobic Flavonoids. Food Hydrocolloid. 2019, 96, 653–662. DOI: https://doi.org/10.1016/j.foodhyd.2019.06.005..
- Raj, J.; Uppuluri, K. B. Metformin Loaded Casein Micelles for Sustained Delivery: Formulation, Characterization, and In-vitro Evaluation. Biomed. Pharmacol. J. 2015, 8(1), 83–89. DOI: https://doi.org/10.13005/bpj/585.
- Purushothaman, B. K.; U.m. P,; M.s.b.k. M. International Journal of Biological Macromolecules Magnetic casein-CaFe 2 O 4 Nanohybrid Carrier Conjugated with Progesterone for Enhanced Cytotoxicity of Citrus Peel Derived Hesperidin Drug Towards Breast and Ovarian Cancer. Int. J. Biol. Macromol. 2020, 151, 293–304. DOI: https://doi.org/10.1016/j.ijbiomac.2020.02.172..
- Jafari, S. M.; Esfanjani, A. F. Instrumental Analysis and Characterization of Nanocapsules. In Nanoencapsulation Technol Food Nutraceutical; 2017; pp 524–544. Elsevier. doi:https://doi.org/10.1016/B978-0-12-809436-5/00014-8.
- Singh, A.; Bajpai, J.; Bajpai, A. K. Investigation of Magnetically Controlled Water Intake Behavior of Iron Oxide Impregnated Superparamagnetic Casein Nanoparticles (Ioicnps). J. Nanobiotechnol. 2014, 12(1), 1–13. DOI: https://doi.org/10.1186/s12951-014-0038-4.
- Zhuang, Y.; Sterr, J.; Kulozik, U.; Gebhardt, R. Application of Confocal Raman Microscopy to Investigate Casein Micro-particles in Blend Casein/ Pectin Films. Int. J. Biol. Macromol. Mar 2015, 74, 44–48. DOI: https://doi.org/10.1016/j.ijbiomac.2014.11.017.
- Zhang, F.; Ma, J.; Xu, Q.; Zhou, J.; Simion, D.; Carmen, G.; Wang, J.; Li, Y. Hollow Casein-based Polymeric Nanospheres for Opaque Coatings. Appl. Mater. Interfaces. 2016, 8, 11739–11748. DOI: https://doi.org/10.1021/acsami.6b00611.F.
- Muhammad, R. S.; Muhammad, I.; Shafi, U. Lipid-Based Nanocarriers for Drug Delivery and Diagnosis. 2017, pp 111–137. Elsevier.
- Toofan, M.; Toofan., J. Chapter 5. A Brief Review of the Cleaning Process for Electronic Device Fabrication. 2015. Elsevier.
- Stevie, F.; Donley, C. Introduction to X-ray Photoelectron Spectroscopy. J. Vac. Sci. Technol. A. 2020, 38, 063204. DOI: https://doi.org/10.1116/6.0000412.
- Shard, A.;. Practical Guides for X-ray Photoelectron Spectroscopy: Quantitative XPS. J. Vac. Sci. Technol. A. 2020, 38, 041201. DOI: https://doi.org/10.1116/1.5141395.
- Greczynski, G.; Hultman, L. X-ray Photoelectron Spectroscopy: Towards Reliable Binding Energy Referencing. Prog. Mater. Sci. 2020, 107, 100591. DOI: https://doi.org/10.1016/j.pmatsci.2019.100591..
- Khan, M.; Oh, S. W.; Kim, Y.-J. Power of Scanning Electron Microscopy and Energy Dispersive X-Ray Analysis in Rapid Microbial Detection and Identification at the Single Cell Level. Sci. Rep-UK. 2020, 10, 2368.
- Scimeca, M.; Bischetti, S.; Lamsira, H.; Bonfiglio, R.; Bonanno., E. Energy Dispersive X-ray (EDX) Microanalysis: A Powerful Tool in Biomedical Research and Diagnosis. Eur J Histochem. 62, 2018. DOI:https://doi.org/10.4081/ejh.2018.2841.
- Abd Mutalib, M.; Rahman, M.; Othman, M. H.; Ismail, A.; Jaafar, J. Scanning Electron Microscopy (SEM) and Energy-Dispersive X-Ray (EDX) Spectroscopy. 2017. Elsevier, https://doi.org/10.1016/B978-0-444-63776-5.00009-7.
- Shojaei T.R.; Azhari S. Fabrication, functionalization, and dispersion of carbon nanotubes. Emerging Applications of Nanoparticles and Architecture Nanostructures. Elsevier, 2018, 501–531. https://doi.org/http://dx.doi.org/10.1016/b978-0-323-51254-1.00016-6.
- Dutta A. Chapter 4-Fourier Transform Infrared Spectroscopy. Spectroscopic Methods for Nanomaterials Characterization. Elsevier. 2017, 73–93. https://doi.org/http://dx.doi.org/10.1016/b978-0-323-46140-5.00004-2.
- Seifi, H.; Gholami, T.; Seifi, S.; Ghoreishi, S. M.; Salavati-Niasari., M. A Review on Current Trends in Thermal Analysis and Hyphenated Techniques in the Investigation of Physical, Mechanical and Chemical Properties of Nanomaterials. J. Anal. Appl. Pyrol. 2020, 149, 104840. DOI: https://doi.org/10.1016/j.jaap.2020.104840.
- Pandita, S.; Wang, L.; Mahendran, R.; Machavaram, V.; Irfan, M. S.; Harris, D.; Fernando., G. Simultaneous DSC-FTIR Spectroscopy: Comparison of Cross-linking Kinetics of an Epoxy/amine Resin System. Thermochim. Acta. 2012, 543, 9–17. DOI: https://doi.org/10.1016/j.tca.2012.04.024..