ABSTRACT
In this study, total phenols (TP) and proanthocyanidins (PAs) in the leaves and branches of Cinnamomum camphora were investigated, and isolated PAs were determined using reversed-phase high performance liquid chromatography electrospray ionization mass spectrometry (HPLC-ESI-MS). The anti-tyrosinase and antioxidant activities were also evaluated. Furthermore, scanning study and dihydroxyphenylalanine (L-DOPA) oxidation were performed to further analyze the inhibition mechanism of PAs on tyrosinase catalytic activity. PAs had strong inhibitory effects on tyrosinase monophenolase activity, with effectively prolonged the delay time and decreased the steady-state of monophenolase activity. For diphenolase activity, PAs both showed reversible and mixed inhibition. Moreover, the PAs showed strong antioxidant activities in scavenging 2,2-Diphenyl-1-picrylhydrazyl (DPPH), 1,2ʹ-azino-bis(3-ethylbenzthiazoline-6-sulphonicacid) (ABTS) and the ferric reducing antioxidant power (FRAP) assays. The PAs in leaves showed stronger anti-tyrosinase and antioxidant capacity, suggesting that C. camphora may be a good resource for tyrosinase inhibitors and antioxidants. This study could provide a scientific basis for the resource utilization of C. camphora and the development of new natural tyrosinase inhibitors and antioxidants in medical, cosmetic, food, and agricultural industries.
Introduction
Tyrosinase (EC 1.14.18.1), a copper-containing oxidoreductase with a complex structure, is the key enzyme and rate-limiting enzyme in melanin synthesis, and it is closely related to many pigmentation disorders, malignant melanoma, insect fusion, and browning of fruits and vegetables.[Citation1] Melanin production can be controlled by controlling the activity of tyrosinase; thus, tyrosinase inhibitors have been highly applied into beauty and whitening, health care, storage of fruits and vegetables, biopesticide, and so on.[Citation1,Citation2] In addition, due to the long-term use and side effects of synthetic antioxidants and anti-tyrosinase agents, such as kojic acid, hydroquinone and arbutin, in the medicine, food and beauty industries, there are many health problems, including residual toxicity, induced diseases, and carcinogenesis.[Citation3] Natural, low toxicity, high-efficiency antioxidants, and anti-tyrosinase from plants, the natural treasure house of bioactive substances, have the advantages of high selectivity and low residue, which meet the requirements of the human body and the future development direction of antioxidants and anti-tyrosinase agents.[Citation4,Citation5] Therefore, it is urgent to find natural antioxidants and anti-tyrosinase agents with strong activity and good safety from plants.
C. camphora is an evergreen tree of Lauraceae that is usually used in architecture, furniture, sculpture, shipbuilding, beautifying cities, and so on. C. camphora, as a traditional Chinese medicine in China, contains complex chemical components, including volatile oil, lignans, flavonoids, glycosides, and so on.[Citation6] Therefore, it has excellent biological activity, with antibacterial, antioxidant, anti-inflammatory, insecticidal, analgesic, anticancer, and other pharmacological effects.[Citation7–11] Recently, quite a few bioactive substances have been extracted from camphora .[Citation12,Citation13] Lee et al.[Citation9] found that C. camphora leaves had considerable antioxidant activity. Additionally, flavonoids and polyphenols in plant extracts were discovered to have a significant correlation with their antioxidation and scavenging free radical capabilities.[Citation14,Citation15] However, few reports concern the development of proanthocyanidins (PAs) from C. camphora. Thus, to make full use of C. camphora, separating PAs from the leaves and branches of C. camphora and examining their biological activities to offer a academic theoretical reference for the exploration of new efficient and safe antioxidants and anti-tyrosinase agents are warranted.
PAs are secondary metabolites synthesized during plant development. They are a class of polyphenols based on flavan-3-ol and linked by carbon bonds. Monoploids are the structural units of PAs and consist of two benzene rings, A and B, and a heterocycle, C.[Citation16] There are two kinds of connections, including A-type PAs linked by C2-O-C7 ether bonds and C4→C8 (C6) bonds, and B-type PAs linked together by a C4→C8 (C6) bond, existing in most plants in nature.[Citation17] In addition, the hydroxyl part of PAs is the donor of hydrogen atoms, and the hydroxyl part has many electrons, which can react with double bonds. Moreover, PAs are soluble in organic solvents, including water and ethanol, and form stable macromolecular complexes with proteins and polysaccharides through certain kinds of chemical bonds. Therefore, PAs have the biochemical properties of convergence, solubility, UV absorption and stability, which result in many special biological functions. For example, due to its strong-free radical scavenging ability and inhibit oxidative damage, PAs have become an internationally recognized natural antioxidant; it can also prevent hypertension, protect cardiovascular, and possess anti-aging, antitumor, skin care, and beauty effects. In addition, the anti-tyrosinase activity of PAs was also reported.[Citation16,Citation18]
In this study, PAs from the leaves and branches of C. camphora were isolated and purified, and the antioxidant activity and anti-tyrosinase activity were also investigated. To the best of our knowledge, there is currently no report on the structure and composition of PAs from C. camphora, or the antioxidant and anti-tyrosinase activities of the PAs. Therefore, this study could provide a theoretical foundation for the comprehensive development of high value-added products of C. camphora and provide a greater possibility for potent antioxidant and anti-tyrosinase agents.
Materials and methods
Preparation of C. camphora PAs
Mature leaves (fully expanded leaves without aging symptoms) and newly-grown branches of C. camphora were randomly selected from Xiangyun Park, Pingdingshan City. After being rinsed with deionized water several times, samples were grinded into powder after freeze-drying using a desktop freeze-dryer at – 56°C for 72 h and stored at −80°C. 20 g of freeze-dried ground powder was added to 250 mL 70% (V/V) acetone aqueous solution and ultrasonically extracted for 30 min, followed by vacuum filtration to obtain the filtrate. The residue was extracted three times, and the filtrate was combined. The acetone was eliminated using vacuum-rotary evaporation and then extracted by petroleum ether and ethyl acetate successively. The aqueous phase kept was performed concentration and freeze-drying to obtain the crude extract of PAs from C. camphora. The crude extracts were dissolved in 50% (V/V) methanol solution, followed by supernatant added into a Sephadex LH-20 column (Pharmacia Biotech, Uppsala, Sweden). Used 50% methanol to remove the impurities and the purified PAs were collected after elution with 70% acetone. Acetone was removed by rotary decompression at 30°C, and purified PAs were stored at −20°C after freeze-drying.
Total polyphenols by the Folin phenol method
The method for the determination of total phenols with FC reagent in reference[Citation19] was slightly modified. 1 mL of sample solution was transferred, 5 mL 10% Folin phenol reagent, and 4 mL 7.5% Na2CO3 solution were added within 5 min after mixing, and then water was added to fix the volume. The standard solution and blank sample were placed at 20°C for 1 h, and then the absorption value was measured by Beckman DU-800 spectrophotometer at 765 nm.
Determination of PAs
The procedure for the determination of PAs referred to reference.[Citation19] 1 mL of the sample solution was added to 6 mL N-butanol-HCl solution, and then boiled for 75 min. After cooling to room temperature, colorimetry was performed at 550 nm and took distilled water and N-butanol-HCl as a control.
RP-HPLC-ESI-MS analysis
The procedure carried out in RP-HPLC-ESI-MS analysis followed references.[Citation20,Citation21] 5% trifluoroacetic acid and acetonitrile were used as mobile phase for linear gradient elution. Elute with 12–80% acetonitrile at 0–45 min and 80–12% acetonitrile at 45–50 min. The flow rate was 1 ml/min and the detection wavelength was 280 nm. The sample was taken for analysis by RP-HPLC-ESI-MS on an Agilent 1100 system (Agilent, Santa Clara, CA, USA) with a diode array detector; further analysis was performed by LC/MS (QTRAP 3200, USA) equipped with a Hypersil ODS column (4.6 × 250 mm) (Elite, Dalian, China). Catechin and epicatechin were used as standards.
Effect of C. camphora PAs on the activity of monophenolase
The 3 mL reaction system including 0.1 mL PAs solution, 2.8 mL phosphate buffer containing 0.05 mol/L L-Tyrosine (L-Tyr) (St. Louis, MO, USA) and 0.1 mL 0.2 mg/mL mushroom tyrosinase (St. Louis, MO, USA) solution was controlled at 30°C using a constant temperature water bath and determined at 475 nm. The optical density value was read once every 5 s, with a total determination time of 300 s by a Beckman DU-800 spectrophotometer.
Effect of C. camphora PAs on the activity of diphenolase
The 3 mL reaction system containing 0.1 mL PAs, 2.8 mL of phosphate buffer containing substrate 0.05 mol/L L-DOPA (dihydroxyphenylalanine) (St. Louis, MO, USA), and 0.1 mL 0.2 mg/mL mushroom tyrosinase solution was detected at 475 nm. The detection was carried out every 5 s, with a total time of 300 s by a Beckman DU-800 spectrophotometer.
Inhibition mechanism of C. camphora PAs on diphenolase
According to the method in reference, [Citation18,Citation22] 0.5 mmol/l L-DOPA was used as the substrate, and the concentration of the enzyme was changed in a system containing PAs at different concentrations (1.33, 3.33, 5.33, 7.33, and 9.33 μg/mL). The relationship between enzymatic reaction rate and tyrosinase concentration was determined, and the inhibition mechanism was judged according to the correlation.
Inhibition type and inhibition constant of C. camphora PAs on diphenolase
In a 3 mL reaction system, the effect of different concentrations of PAs on the catalytic activity of L-DOPA catalyzed by tyrosinase was determined, with fixed enzyme concentration and varied L-DOPA amount. The inhibition type of the inhibitor was determined by a Lineweaver–Burk double reciprocal plot with the reciprocal of substrate concentration as the X axisand the reciprocal of reaction speed as the Y axis. The Lineweaver–Burk equation for mixed-type inhibition mechanism was:
were the concentration of substrate and inhibitor, respectively. The inhibition constant (KI) of the PAs to tyrosinase and the inhibition constant (KIS) of the PAs to the enzyme-substrate complex can be obtained by plotting the concentration of the PAs with the slope of the straight line and the intercept of the Y axis, respectively.
Scanning study
Took 0.5 mmol/L L-Tyr as substrate, 125 μL 2 mg/mL PAs (leaves) and 2.3 mg/mL PAs (branches) were added in 50 mmol/L Na2HPO4-NaH2PO4 buffer (pH 6.8) at 25°C. The final concentration of tyrosinase in 3 mL reaction system was 16.67 μg/mL and the spectrophotometer (Beckman DU-8000) was used to record continuously at scanning wavelength of 240–800 nm for 10 minutes.
Effect of PAs on the oxidation spectra of L-DOPA by sodium periodate
The 3 mL reaction system containing 0.3 mL 1 mg/mL L-DOPA and 0.5 mL phosphate buffer saline (50 mmol/L, pH 6.8) was tested in the absence and present of 1 mg/mL NaIO4.[Citation23] The effects of PAs on the oxidation of L-DOPA were detected by adding different concentrations of PAs into L-DOPA and adding different concentrations of PAs in the presence of L-DOPA and NaIO4. 0, 50, 100 μg/mL and 0, 80, 160 μg/mL was used as the concentration of PAs in leaves and branches of C. camphora, respectively. The spectral changes of oxidation products of L-DOPA were detected using Du-650 spectrophotometer at 230–800 nm.
Evaluation of DPPH radical scavenging ability
3 mL DPPH solution (25 μg/mL methanol dissolution) was added to 0.1 mL sample solutions of different concentrations, and methanol solution was used as a blank instead of sample solution. Followed by reacted for 30 min and the tested absorbance was 517 nm. The determination was carried out twice, and three parallel tests were carried out according to the above operation steps. The clearance formula of DPPH was SADPPH (%) = [(A1-A2)/A1]×100%. A1 is the blank absorbance; A2 is the absorbance after adding the sample solution to be tested. The IC50 value is the sample concentration when the absorbance value is reduced by 50%.
ABTS free radical scavenging ability
Referring to ReRobert et al., [Citation24] 0.1 mL sample solution and 3.9 mL ABTS free radical working solution were fully mixed. After reacting for 6 min, the absorbance A2 was measured at 734 nm. The mixture of 80% ethanol solution and ABTS was used as a control, and the absorbance was A1. The formula for the ABTS radical scavenging rate (%) = [(A1-A2)/A1] × 100%.
Total oxidation capacity by the FRAP method
A 0.1 mL sample solution and 3 mL FRAP working solution were added to the centrifuge tube, and after incubation for 5 min in a water bath at 25°C, the absorbance value (A value) was tested at 593 nm. Deionized water was added into the FRAP working solution as a blank. According to the A value after the reaction, the corresponding concentration was obtained on the FeSO4 standard curve, which was defined as the FRAP value.
Statistical analysis
All experiments were repeated three times and plotted with the average value. SPSS version 13.0 software was used for statistical analysis.
Results
Total phenol (TP) and PAs
The relative contents of total phenols and PAs in the leaves and branches of C. camphora were obtained by comparison with a standard curve. The contents of TP and PAs in the leaves of C. camphora were 221 ± 18 mg/g and 152 ± 9 mg/g, respectively, and in the branches of C. camphora, they were 118 ± 12 mg/g and 90 ± 8 mg/g, respectively. The results showed that the content of TP and PAs in the leaves of C. camphora was 22% and 15%, respectively, and that in the branches of C. camphora was 12% and 9%, respectively.
Reversed-phase HPLC-ESI-MS
The HPLC-ESI-MS results of sulfurized PAs from C. camphora leaves and branches are shown in . It can be seen that the PAs from leaves and branches have obvious difference in the peak position and intensity, indicating that there are difference in the composition of two parts in C. camphora, in which C-thio (catechin benzylthioether) and AF/EAF (afzelechin/epiafzelechin) were detected in leaves, while no significant AF/EAF was detected in branches. But for both, the terminal units were C (catechin) and the extension units were mainly C/EC-thio (catechin/epicatechin benzylthioether).
Monophenolase activity
Tyrosinase has monophenolase activity of hydroxylation of monophenol to diphenol and diphenolase activity of oxidation of bisphenol to quinone. Therefore, we first measured the monophenolase activity using L-tyrosine (L-Tyr) as the substrate. As the results shown in a, the X-axis intercept of the linear extension was the delay time of the enzymatic reaction, and the slope of the straight line was the steady-state activity of tyrosinase. shows the catalytic reaction of the inhibition of monophenolase activity by the PAs and plots the concentration of PAs with steady state activity and lag time ( and ). The results showed that the Pas from the leaves and branches of C. camphora could both effectively prolong the delay time of monophenolase activity and decrease its steady-state activity, and the inhibitory effect showed an obvious dose-dependent manner. It was found that when the concentration of the PAs from leaves and branches reached 200 μg/mL and 306.67 μg/mL, the lag time increased from 13.14 s to 120 s and 3.6 s to 33 s, respectively, while the steady-state activity decreased from 100% to 43.23% and 42.86%, respectively. The IC50 of PAs was 167 ± 18 μg/mL and 268 ± 24 μg/mL, respectively (), indicating that PAs extracted from leaves possess a better inhibitory effect on tyrosinase monophenolase activity than those from branches.
Table 1. Inhibition activity and constants of proanthocyanidins (PAs) extracted from leaves and branches of Cinnamomum camphora.
Figure 2. Inhibition effects of proanthocyanidins (PAs) extracted from leaves and branches in Cinnamomum camphora on monophenolase (a), diphenolase (b) activity and inhibitory mechanism (c) of mushroom tyrosinase. Progress curve for the oxidation of L-Tyr by the enzyme. In leaves, the concentrations of proanthocyanidins (PAs) for curve 0–4 was 0, 33.33, 66.67, 133.33 and 200 μg/mL, respectively. In branches, curves 0–4 represent the reaction curve of the system when the concentration of proanthocyanidins (PAs) was 0, 76.67, 153.33, 230 and 306.67 μg/mL, respectively. Effects of proanthocyanidins (PAs) on the steady-state rate of monophenolase. Effects of proanthocyanidins (PAs) on the lag time of mushroom tyrosinase. In leaves, lines 0–4 represent the reaction curve of the system when the concentration of proanthocyanidins (PAs) was 0, 13.33, 40, 66.67, 93.33 μg/mL. In branches, lines 0–4 represent the reaction curve of the system when the concentration of proanthocyanidins (PAs) was 0, 30.67, 46, 76.67, 92 μg/mL
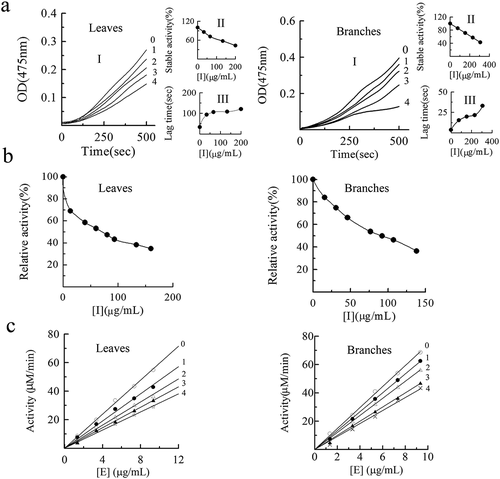
Diphenolase activity
L-DOPA was used as a substrate to determine the activity of tyrosinase diphenolase, and the reaction curve obtained is shown in b. The enzyme activity decreased with increasing PAs concentration, and the IC50 values were 70 ± 7 μg/mL and 91 ± 8 μg/mL, respectively (). Both of them had good inhibitory activity on tyrosinase diphenolase, especially the extract of PAs from leaves of C. camphora.
Inhibition mechanism
The inhibition mechanism of PAs on tyrosinase is shown in c. The slope of straight lines decreased with increasing PAs concentration, and two sets of straight lines passing through the origin were obtained by mapping the enzyme activity to the PAs concentration. These suggested that instead of reducing the amount of effective enzyme, PAs reduced the catalytic efficiency of enzyme to achieve the inhibitory activity, which can be concluded that the inhibition of tyrosinase by PAs is a reversible process.
Inhibition type and inhibition constant of PAs from C. camphora on tyrosinase
In the diphenolase detection system, by measuring the effect of L-DOPA on tyrosinase activity, a group of straight lines with different slope intersected in the second quadrant were obtained, as shown in a and b, in which the Km increased and Vm decreased with the increase of substrate concentration, showed mixed competitive inhibition. The inhibition constant KI and KIS of leaves PAs can be calculated as 33.36 μg/mL and 344.44 μg/mL, respectively (). For PAs from branches of C. camphora, the inhibition constants KI and KIS were 17.97 μg/mL and 349.89 μg/mL, respectively. For PAs from the leaves and branches of C. camphora, KIS was 10 and 19 times that of KI, respectively.
Figure 3. Determination of the inhibitory type and constants of proanthocyanidins (PAs) extracted from leaves (a) and branches (b) of Cinnamomum camphora. In leaves, lines 0–4 represent the reaction curve of the system when the concentration of proanthocyanidins (PAs) was 0, 13.33, 40, 66.67, 93.33 μg/mL with L-DOPA as substrate. In branches, lines 0–4 represent the reaction curve of the system when the concentration of proanthocyanidins (PAs) was 0, 30.67, 46, 76.67, 92 μg/mL. The plot of intercept versus proanthocyanidins (PAs) concentration for determining the inhibition constants KIS. The plot of slope versus PAs concentration for determining the inhibition constants KI.
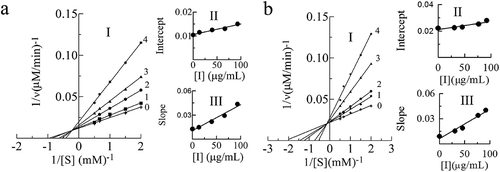
Scanning study
The inhibition mechanism of PAs on tyrosinase was further studied by UV-Vis spectroscopy. shows the time-dependent UV spectra of PAs acting on tyrosinase catalyzed oxidation of tyrosine. The absorption value in 315 nm and 475 nm increased with the increase of catalytic time, suggesting that 315 nm and 475 nm are the two characteristic peaks of the oxidation of L-Tyr by tyrosinase in the ultraviolet and visible region, respectively. Compared with , the absorption values of absorption peaks at 315 nm and 475 nm caused by PAs decreased ( b-c), indicating that PAs could effectively inhibit the oxidation of L-Tyr by tyrosinase.
L-DOPA oxidation
The product of L-DOPA catalyzed by tyrosinase can also be obtained from the non-enzymatic catalysis, such as oxidized by NaIO4. Using spectral analysis to assess the effect of PAs on the oxidation of L-DOPA, and shows that the characteristic peaks of L-DOPA oxidized by NaIO4 are located at 315 nm (ultraviolet region) and 475 nm (visible region) after adding PAs, and the absorbance value obviously decreases. It is suggested that PAs can decline the L-DOPA oxidation products.
DPPH radical scavenging assay
The relationship between the scavenging capacity of PAs from two plant parts on DPPH free radicals is shown in a. In the range of experimental concentrations, with increasing concentration, the scavenging effect of each part on DPPH free radicals also showed an increasing trend. The antioxidant activities of different extracts were significantly different, and the DPPH scavenging ability of PAs extracted from these two parts was relatively weak compared to that of VC. The IC50 of DPPH radical scavenging activity was 78 ± 13 μg/mL and 274 ± 28 μg/mL (). The DPPH scavenging ability of leaves was higher than that of branches.
Table 2. Comparison of antioxidant capacity between leaves and branches proanthocyanidins (PAs) from Cinnamomum camphora. and VC
Figure 6. The antioxidant activity of the leaves and branch proanthocyanidins (PAs) from Cinnamomum camphora. is determined by 2,2-Diphenyl-1-picrylhydrazyl (DPPH) assay (a), 1,2ʹ-azino-bis(3-ethylbenzthiazoline-6-sulphonicacid) (ABTS) assay (b) and ferric reducing antioxidant power (FRAP) assay (c)
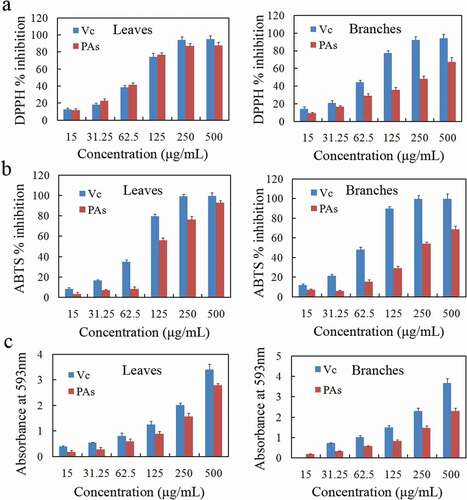
ABTS free-radical scavenging assay
b shows that the ABTS radical scavenging rate of PAs and VC was positively correlated with the concentration, and the changing trend was similar to the scavenging rate of DPPH radicals. The IC50 values of leaves and branches in are greater than those of VC.
FRAP assay
The results of the FRAP assay were expressed in the form of VC equivalent, that is, the ability of a 1 g sample to scavenge free radicals is equivalent to the capacity in mmol of VC. It can be seen from the results in that leaves PAs (4.7 ± 0.5 mmol AAE/g) > branches PAs (3.6 ± 0.4 mmol AAE/g).
DISCUSSION
Due to the strong correlation between the biological activity and the content of polyphenols, the determination of polyphenol content by Folin phenol colorimetry can explain the mechanism of biological activity of the samples to be tested. Thus, first, we used the FC method to determine TP content and the HCl-N-butanol method to determine PAs content. The content of PAs in leaves was more abundant than that in branches, suggesting that leaves may be a good raw material for PAs supply. Then, the basic structural units and polymer-chain lengths of PAs were predicted by measuring the conjugates of acid-catalyzed PAs with benzyl mercaptan. In this study, the basic structural units of C/EC and AF/EAF, which have very active phenolic hydroxyl groups and dihydroxy phthalic groups, were found in the structural identification of the PAs from leaves of C. camphora, while no AF/EAF was found in branches, which may be the main reason for the better anti-tyrosinase and antioxidant activity of leaves PAs.
Tyrosinase has monophenolase activity of hydroxylation of monophenol to diphenol and diphenolase activity of oxidation of bisphenol to quinine.[Citation22,Citation25] PAs from the leaves and branches of C. camphora could both effectively prolong the delay time of monophenolase activity and decrease its steady-state activity, and the inhibitory effect showed an obvious dose-dependent manner. Moreover, PAs extracted from leaves have a better inhibitory effect on tyrosinase monophenolase activity than those from branches. Qu et al.[Citation26]reported the effects of puerarin on the tyrosinase monophenolase activity with an IC50 value of 0.537 mg/mL. Cui et al.[Citation27] reported that T. grandis “Xiangyafei” seed oil (XYSO) exhibited the highest activities in the tested seed oils against tyrosinase monophenolase (IC50 = 817.5 μg/mL). For tyrosinase diphenolase, both of them had good inhibitory activity on tyrosinase diphenolase, especially the extract of PAs from leaves of C. camphora. XYSO in T. grandis was tested against tyrosinase diphenolase with IC50 values of 227 ± 3 μg/mL.[Citation27] A. aucheri oil exhibited anti-tyrosinase activity at a 50% concentration (IC50) of 6.43 mg/mL.[Citation28] Obviously, PAs extracted from the leaves and branches of C. camphora both showed better inhibitory effect on tyrosinase monophenolase and diphenolase, suggesting that C. camphora may be a good resource for tyrosinase inhibitors. Thus, it is feasible to seek potent tyrosinase inhibitor PAs from C. camphora.
In the diphenolase determination system, the inhibitory effect of PAs on tyrosinase was a reversible process and a mixed competitive inhibition. Reduced catalytic efficiency reversible has been a common inhibition mode of PAs from plants on tyrosinase diphenolase.[Citation22,Citation29,Citation30] As shown in HPLC-ESI-MS, the basic structural units of C/EC and AF/EAF in PAs, which have very active phenolic hydroxy groups and dihydroxy phthalic groups, may be the structural reason for its reversible inhibition of tyrosinase activity. The inhibition constant KIS was much larger than KI further indicated that the binding ability of PAs extracted from the two parts of C. camphora to free enzyme is much stronger than that to the enzyme substrate complex. In conclusion, the results demonstrated for the first time that the leaves and branches of C. camphora might be good sources for further development of tyrosinase inhibitors, which indicated the possible application of these compounds, especially PAs from leaves, in food, agricultural, cosmetic, and medical industries.
To further analyzed the inhibition mechanism of PAs on tyrosinase catalytic activity, UV-Vis spectroscopy was used to assess the L-Tyr oxidation and L-DOPA oxidation of PAs. PAs in C. camphora could inhibit the oxidation of L-Tyr by tyrosinase, and the effect of PAs on the spectra of oxidation products of L-DOPA indicated that PAs could depress the oxidation products of L-DOPA and prevent the formation of L-DOPA pigment, which leads to the reduction of characteristic absorption peak of the product. Due to the rich hydroxyl structure, PAs can prevent the chain reaction of free radicals by releasing H+ in the structure to compete with free radicals, eliminate free radicals, and protect lipids from oxidation.[Citation31–35] Thus, DPPH, ABTS, and FRAP methods were used to investigate the antioxidant capacity of PAs in leaves and branches of C. camphora. Wang et al. reported that the Fuwan 8, Dongliang, and FD97 varieties of Dimocarpus longan Lour. had the strongest DPPH scavenging activity, with an IC50 of 1.03 g/mL.[Citation32,Citation33] The anthocyanins isolated from Lycium ruthenicum Murr posessed ABTS radical scavenging capacity, with IC50 of 0.50 ± 0.01 mg/mL[Citation36] . Because the total phenol content (TPC) was the greatest antioxidant contributor in the DPPH and FRAP assays, the radical scavenging ability of PAs in leaves was better than that of PAs in branches, which may be due to the relatively high TPC. In short, the results demonstrated for the first time that PAs from the leaves and branches of C. camphora might be good sources for the further development of antioxidants.
Conclusion
In conclusion, in this study, the separation, purification, and determination of the content of total phenols and PAs in leaves and branches of C. camphora were carried out first. Then, through the analysis of their structure and evaluation of their activity, including anti-tyrosinase and antioxidant activity, the resource availability of PAs from the leaves and branches of C. camphora was clarified and the relationship between the two was identified based on structure and activity. Therefore, this study could lay a theoretical foundation for better research, development, and utilization of the biological activity of PAs and provide a possibility for the development of new efficient, natural tyrosinase inhibitors and antioxidants.
Acknowledgments
This work was supported by the Key Laboratory of Forest Germplasm Conservation, Selection, and Breeding of Improved Variety of Henan Province [No. 2020JB02009].
Additional information
Funding
References
- Seo, S. Y.; Sharma, V. K.; Sharma, N. Mushroom Tyrosinase: Recent Prospects. J. Agric. Food Chem. 2003, 51(10), 2837–2853. DOI: https://doi.org/10.1021/jf020826f.
- Ullah, S.; Son, S.; Yun, H. Y.; Kim, D. H.; Chun, P.; Moon, H. R. Tyrosinase Inhibitors: A Patent Review (2011-2015). Expert Opin. Ther. Pat. 2016, 26(3), 347. DOI: https://doi.org/10.1517/13543776.2016.1146253.
- You, A.; Zhou, J.; Song, S.; Zhu, G.; Song, H.; Yi, W. Rational Design, Synthesis and Structure–activity Relationships of 4-alkoxy- and 4-acyloxy-phenylethylenethiosemicarbazone Analogues as Novel Tyrosinase Inhibitors. Bioorg. Med. Chem. 2015, 23(5), 924–931. DOI: https://doi.org/10.1016/j.bmc.2015.01.024.
- Ghosh, A.; Das, B. K.; Chatterjee, S. K.; Chandra, G. Antibacterial Potentiality and Phytochemical Analysis of Mature Leaves of Polyalthia Longifolia (Magnoliales: Annonaceae). South Pacific Journal of Natural & Applied Sciences. 2008, 26(26), 68–72. DOI: https://doi.org/10.1071/SP08011.
- Braga, T. V.; Dores, R. G. R. D.; Ramos, C. S.; Evangelista, F. C. G.; Sabino, A. Antioxidant, Antibacterial and Antitumor Activity of Ethanolic Extract of the Psidium Guajava Leaves. American Journal of Plant Sciences 2014, 5(23), 3492–3500.
- Chen, J.; Tang, C.; Zhang, R.; Ye, S.; Zhao, Z.; Huang, Y.; Xu, X.; Lan, W.; Yang, D. Metabolomics Analysis to Evaluate the Antibacterial Activity of the Essential Oil from the Leaves of Cinnamomum Camphora (Linn.) Presl. Journal of Ethnopharmacology 2020, 253, 112652. DOI: https://doi.org/10.1016/j.jep.2020.112652.
- Zhou, H.; Ren, J.; Li, Z. Antibacterial Activity and Mechanism of Pinoresinol from Cinnamomum Camphora Leaves against Food-related Bacteria. Food Control. 2017, 79, 192–199. DOI: https://doi.org/10.1016/j.foodcont.2017.03.041.
- Herman, A.; Tambor, K.; Herman, A. Linalool Affects the Antimicrobial Efficacy of Essential Oils. Curr. Microbiol. 2016, 72(2), 165–172. DOI: https://doi.org/10.1007/s00284-015-0933-4.
- Lee, H. J.; Hyun, E. A.; Yoon, W. J.; Kim, B. H.; Rhee, M. H.; Kang, H. K.; Cho, J. Y.; Yoo, E. S. In Vitro Anti-inflammatory and Anti-oxidative Effects of Cinnamomum Camphora Extracts. J. Ethnopharmacol. 2006, 103(2), 208–216. DOI: https://doi.org/10.1016/j.jep.2005.08.009.
- Hao, J.; Jin, W.; Li, S.; Xianshuang, C.; Xi, Y.; Feng, T.; Yongde, Y. GC×GC-TOFMS Analysis of Essential Oils Composition from Leaves, Twigs and Seeds of Cinnamomum Camphora L. Presl and Their Insecticidal and Repellent Activities. Molecules. 2016, 21(4), 423.
- Hamidpour, R.; Hamidpour, S.; Hamidpour, M.; Shahlari, M. Camphor (Cinnamomum Camphora), a Traditional Remedy with the History of Treating Several Diseases. International Journal of Case Reports & Images. 2013, 4(2), 86–89. DOI: https://doi.org/10.5348/ijcri-2013-02-267-RA-1.
- Li, Y. R.; Fu, C. S.; Yang, W. J.; Wang, X. L.; Feng, D.; Wang, X. N.; Ren, D. M.; Lou, H. X.; Shen, T. Investigation of Constituents from Cinnamomum Camphora (L.) J. Presl and Evaluation of Their Anti-inflammatory Properties in Lipopolysaccharide-stimulated RAW 264.7 Macrophages. J. Ethnopharmacol. 2018, 221, 37–47. DOI: https://doi.org/10.1016/j.jep.2018.04.017.
- Lu, Z. M.; Geng, Y.; Li, H. X.; Sun, Q.; Shi, J. S.; Xu, Z. H. Alpha-terpineol Promotes Triterpenoid Production of Antrodia Cinnamomea in Submerged Culture. FEMS Microbiol. Lett. 2014, 358(1), 36–43. DOI: https://doi.org/10.1111/1574-6968.12545.
- Jemia, M. B.; Chaabane, S.; Senatore, F.; Bruno, M.; Kchouk, M. E. Studies on the Antioxidant Activity of the Essential Oil and Extract of Tunisian Tetraclinis Articulata (Vahl) Mast (Cupressaceae). Natural Product Research. 2013, 27(16), 1419–1430. DOI: https://doi.org/10.1080/14786419.2012.717289.
- Liu, Z.; Kong, L.; Lu, S.; Zou, Z. Application of a Combined Homogenate and Ultrasonic Cavitation System for the Efficient Extraction of Flavonoids from Cinnamomum Camphora Leaves and Evaluation of Their Antioxidant Activity in Vitro. J. Anal. Methods Chem. 2019, 2019, 4892635. DOI: https://doi.org/10.1155/2019/4892635.
- Chai, W. M.; Chen, C. M.; Gao, Y. S.; Feng, H. L.; Ding, Y. M.; Shi, Y.; Zhou, H. T.; Chen, Q. X. Structural Analysis of Proanthocyanidins Isolated from Fruit Stone of Chinese Hawthorn with Potent Antityrosinase and Antioxidant Activity. J. Agric. Food Chem. 2014, 62(1), 123–129. DOI: https://doi.org/10.1021/jf405385j.
- Cos, P.; Bruyne, T. D.; Hermans, N.; Apers, S.; Vlietinck, A. J.; Vlietinck, A. Proanthocyanidins in Health Care: Current and New Trends. Curr. Med. Chem. 2004, 11(10), 1345–1359. DOI: https://doi.org/10.2174/0929867043365288.
- Song, W.; Zhu, X. F.; Ding, X. D.; Yang, H. B.; Qin, S. T.; Chen, H.; Wei, S. D.; Features, S. Antioxidant and Tyrosinase Inhibitory Activities of Proanthocyanidins in Leaves of Two Tea Cultivars. Int. J. Food Prop. 2017, 20(6), 1348–1358. DOI: https://doi.org/10.1080/10942912.2016.1209682.
- Lin, Y. M.; Liu, J. W.; Xiang, P.; Lin, P.; Ye, G. F.; Sternberg, L. S. L. D. Tannin Dynamics of Propagules and Leaves of Kandelia Candel and Bruguiera Gymnorrhiza in the Jiulong River Estuary, Fujian, China. Biogeochemistry. 2006, 78(3), 343–359. DOI: https://doi.org/10.1007/s10533-005-4427-5.
- Chen, X. X.; Liang, G.; Chai, W. M.; Feng, H. L.; Zhou, H. T.; Shi, Y.; Chen, Q. X. Antioxidant and Antityrosinase Proanthocyanidins from Polyalthia Longifolia Leaves. Journal of Bioscience & Bioengineering. 2014, 118(5), 583–587. DOI: https://doi.org/10.1016/j.jbiosc.2014.04.015.
- Wei, S. D.; Lin, Y. M.; Liao, M. M.; Zhou, H. C.; Li, Y. Y. Characterization and Antioxidative Properties of Condensed Tannins from the Mangrove Plant Aegiceras Corniculatum. Journal of Applied Polymer ence. 2012, 124(3), 2463–2472. DOI: https://doi.org/10.1002/app.35258.
- Song, W.; Qin, S. T.; Fang, F. X.; Gao, Z. J.; Liang, D. D.; Liu, L. L.; Tian, H. T.; Yang, H. B. Isolation and Purification of Condensed Tannin from the Leaves and Branches of Prunus Cerasifera and Its Structure and Bioactivities. Appl. Biochem. Biotechnol. 2018, 185(2), 464–475. DOI: https://doi.org/10.1007/s12010-017-2635-9.
- Song, W.; Liu, L. L.; Ren, Y. J.; Wei, S. D.; Yang, H. B. Inhibitory Effects and Molecular Mechanism on Mushroom Tyrosinase by Condensed Tannins Isolation from the Fruit of Ziziphus Jujuba Mill. Var. Spinosa (Bunge) Hu Ex H. F. Chow. Int. J. Biol. Macromol. 2020, 165(Pt B), 1813–1821. DOI: https://doi.org/10.1016/j.ijbiomac.2020.09.259.
- Re, R.; Pellegrini, N.; Proteggente, A.; Pannala, A.; Yang, M.; Rice-Evans, C. Antioxidant Activity Applying an Improved ABTS Radical Cation Decolorization Assay. Free Radical Biol. Med. 1999, 26(9–10), 1231–1237. DOI: https://doi.org/10.1016/S0891-5849(98)00315-3.
- Chai, W.; Wei, Q.-M.; Deng, W.; Zheng, Y.-L.; Chen, X.-Y.; Huang, Q.; Ou-Yang, C.; Peng, -Y.-Y. Anti-melanogenesis Properties of Condensed Tannins from Vigna Angularis Seeds with Potent Antioxidant and DNA Damage Protection Activities. Food Funct. 2019, 10(1), 99–111. DOI: https://doi.org/10.1039/C8FO01979G.
- Qu, L.; Song, K.; Zhang, Q.; Guo, J.; Huang, J. Simultaneous Determination of Six Isoflavones from Puerariae Lobatae Radix by CPE-HPLC and Effect of Puerarin on Tyrosinase Activity. Molecules. 2020, 25(2), 344. DOI: https://doi.org/10.3390/molecules25020344.
- Cui, H. X.; Duan, F. F.; Jia, S. S.; Cheng, F. R.; Yuan, K. Antioxidant and Tyrosinase Inhibitory Activities of Seed Oils from Torreya Grandis Fortex Lindl. Biomed Res. Int. 2018, 2018(5), 1–10.
- Taherkhani, M.;. Chemical Constituents, Total Phenolic Content, Antimicrobial, Antioxidant and Radical Scavenging Properties, Chelating Ability, Tyrosinase Inhibition and in Vitro Cytotoxic Effects of Artemisia Aucheri Herbs. Pharm. Chem. J. 2017, 50(11), 736–745. DOI: https://doi.org/10.1007/s11094-017-1523-5.
- Cabanes, J.; Chazarra, S.; Garcia-Carmona, F. Kojic Acid, a Cosmetic Skin Whitening Agent, Is a Slow-binding Inhibitor of Catecholase Activity of Tyrosinase. J. Pharm. Pharmacol. 1994, 46(12), 982–985. DOI: https://doi.org/10.1111/j.2042-7158.1994.tb03253.x.
- Chai, W. M.; Wang, R.; Wei, M. K.; Zou, Z. R.; Deng, R. G.; Liu, W. S.; Peng, Y. Y. Proanthocyanidins Extracted from Rhododendron Pulchrum Leaves as Source of Tyrosinase Inhibitors: Structure, Activity, and Mechanism. PLoS One. 2015, 10(12), e0145483. DOI: https://doi.org/10.1371/journal.pone.0145483.
- Blade, G, C.; Arola-Arnal, A., . A.; Muguerza, B.; Bravo, F. I.; Salvado, M. J.; Arola, L.; Suarez, M. Proanthocyanidins in Health and Disease. Biofactors. 2016, 42(1), 5–12.
- Zeng, Y. X.; Wang, S.; Wei, L.; Cui, Y. Y.; Chen, Y. H. Proanthocyanidins: Components, Pharmacokinetics and Biomedical Properties. Am. J. Chin. Med. 2020, 48(4), 813–869. DOI: https://doi.org/10.1142/S0192415X2050041X.
- Wang, Z.; Ga, O.; Li, X.; Tan, W.; Zheng, Q., S. Phenolic Content, Antioxidant Capacity, and α-amylase and α-glucosidase Inhibitory Activities of Dimocarpus Longan Lour. Food Sci. Biotechnol. 2020, 29(5), 683–692. DOI: https://doi.org/10.1007/s10068-019-00708-3.
- Wei, S.; Chen, D. ; Lin, Y. M, H. Comparison of Chemical Compositions and Antioxidant Activities of Condensed Tannins from Different Parts ofCalliandra Haematocephala. J. Wood Chem. Technol. 2015, 35(3), 193–206. DOI: https://doi.org/10.1080/02773813.2014.919596.
- Chen, H.; Sun, K.; Yang, Z.; Guo, X.; Wei, S. Identification of Antioxidant and Anti-α-amylase Components in Lotus (Nelumbo Nucifera, Gaertn.) Seed Epicarp. Appl. biochem. biotech. 2018, 187, 1–14.
- Shen, M.; Liu, L., .; Liang, Y. F, K.; Liu, G.; Sang, X.; Li, J.; C. Q. Extraction Optimization and Purification of Anthocyanins from Lycium Ruthenicum Murr. And Evaluation of Tyrosinase Inhibitory Activity of the Anthocyanins. J. Food Sci. 2020, 85(3), 696–706. DOI: https://doi.org/10.1111/1750-3841.15037.