ABSTRACT
The influence of alcohols with different alkyl chain length (methanol, ethanol and propanol) on the physicochemical properties (turbidity, surface hydrophobicity, exposed and total free sulfhydryl groups, denaturation temperature; Tm) of pasteurized liquid egg white (PLEW), including gel properties (gel hardness and expressible liquid) and microstructure of heat-induced PLEW gel prepared at 75°C were studied. The results showed that alkyl chain length of alcohol and alcohol concentration had no impact on the total free sulfhydryl groups, but significantly increased the turbidity, surface hydrophobicity, exposed free sulfhydryl groups, gel hardness and expressible water. Increase the alkyl chain length of alcohol also significantly decreased the Tm and the total enthalpy change during heat-induced gelation of PLEW. Addition of 5% alcohol altered the microstructure of the PLEW gels in an alkyl chain length dependent manner.
INTRODUCTION
The many functions of proteins relate to their physicochemical and conformational properties,[Citation1] which can be affected in food processing by protein concentration, heat processing, pH, solvent nature and salt concentration.[Citation2–4] The molecular structure, the composition of solution surrounding proteins, and the thermal-mechanical history also impact their ability to provide the desirable functional characteristics in particular food applications.[Citation5–8] Several of the physical and functional properties of protein such as solubility, emulsifying, foaming and gelling properties are governed by their surface charge.[Citation9,Citation10]
Gel formation is an important function of proteins that is related to their capability to form a three-dimensional network that traps water and other soluble components, which has a profound effect on the texture and sensory properties of the final food product.[Citation11–13] Characteristics of the protein molecules, the ionic strength and the pH of the solution are factors that govern the microstructure of the gel network and the bulk physicochemical properties of the protein gel.[Citation11,Citation13–15] Native protein solutions may not gel at neutral pH because their intermolecular repulsive interactions, e.g. electrostatic forces, hydration and configurational entropies, are higher than their attractive interactions, e.g. van der Waals forces and hydrophobic interactions.[Citation14,Citation16] However, gels can be formed when the electrostatic repulsions between the proteins are lower than the attractive interactions, such as by heating above the thermal denaturation temperature (Tm) of the proteins.[Citation17] The heat induces molecular protein aggregation and the self-assembly to form gel.[Citation15] Protein gels can be produced in various ways such as heat-induced gelation, hydrostatic pressure-induced gelation, salt-induced gelation, acid-induced gelation and enzyme-induced gelation.[Citation4,Citation11,Citation18–21] Heat-induced gelation is the most common technique to produce protein gels in the food industry.[Citation19]
Alcohol can denature protein.[Citation14,Citation15,Citation22] The degree of denaturation of protein caused by alcohol depends on the alcohol concentration. Alcohol increases the protein aggregation and the viscosity of the protein to varying degrees depending on the concentration and type of alcohol.[Citation14,Citation22,Citation23] It has been reported that about 20 wt% of ethanol causes partial protein denaturation and around 50 wt% of ethanol has the maximum effect on protein denaturation.[Citation24–27] Ishino and Kudo[Citation28] found that less hydrophobic alcohols increased the viscosity of 7S and 11S components in soybean globulin. Alcohol also impacted the thermal stability of protein by reducing the enthalpy and melting temperature of protein.[Citation29] Ethanol also induced cold-denaturation and reduced the denaturation temperature of whey protein isolate.[Citation30] In the presence of 50 wt% ethanol, whey protein can form gel at temperature around 15°C below its normal gelation temperature.[Citation30]
It has been reported that ethanol can impact protein conformation, e.g. a change from β-sheet to an α-helix-rich conformation observed in β-lactoglobulin.[Citation26] From the study of protein conformation of α-chymotrypsin enzyme using a circular dichroism (CD) spectra analysis, around 50 wt% of ethanol alters the conformation of the enzyme resulting in loss of its activity. However, the enzyme returned to its native-like state and enzyme activity was restored in the presence of ethanol at a concentration higher than 70 wt%.[Citation31] Alcohol also had an impact on the protein conformation of chicken egg white lysozyme.[Citation32–34] In the presence of 60% ethanol, lysozyme conformation was changed from native to α-helical structure resulting in less protein solubility. In addition, lysozyme gel formation occurred in the presence of ethanol concentration between 60 and 75%.[Citation35] Research showed that gelation of β-lactoglobulin was not only dependent on the alcohol concentration, but also the pH of the protein solution.[Citation36,Citation37]
Egg white is a widely used food ingredient because it provides a unique taste, high nutritional value, and good functionality. It contains 9.7–12% protein, of which ovalbumin is the major protein (54%), followed by ovotransferrin (12–13%), ovomucoid (11%), ovoglobulins (8%), ovomucin (3.5%) and lysozyme (3.4%).[Citation38,Citation39] Egg white can improve the gelling and foaming properties of other proteins. Pasteurized liquid egg white (PLEW) is used in the food industry to reduce the labor cost needed to separate and collect egg white from fresh eggs and to avoid potential Salmonella contamination from fresh egg white, however, the pasteurization temperatures required could impair its functional properties.[Citation39] The functional properties of PLEW depend on pH, salt content, and thermal history. PLEW can enhance food texture due to its ability to form heat set gels. However, this technique may alter the quality of food since it requires heat to raise the temperature of the food to above the Tm of protein in food matrix.[Citation40] At temperature equal to or above the Tm, proteins denature and then aggregate. Heating time is also crucial to complete gelation process and influence gel properties. These changes may be beneficial or undesirable to product quality.
Research on the effect of alcohol on the denaturation of different proteins is widely reported, however, little work has been performed that investigates the impact of alkyl chain length of alcohol on the physicochemical properties of PLEW and gel properties of heat-induced PLEW gel. We hypothesize that alcohols of different alkyl chain length will affect the physicochemical and functional properties of PLEW. Thus, the objective of this study was to elucidate the influence of low concentration of alcohols with different alkyl chain lengths on the physicochemical properties of PLEW and gel properties of heat-induced PLEW gel.
MATERIALS AND METHODS
Materials
Pasteurized liquid egg white (PLEW) was purchased from a local supermarket (Pathum Thani, Thailand). Alcohols of different alkyl chain lengths (methanol, ethanol, and 1-propanol) were purchased from RCI Labscan (RCI Labscan Limited, Bangkok, Thailand), Merck (Merck KGaA, Damstadt, Germany), and Fisher Chemical (Fisher Scientific, Leics, United Kingdom), respectively. Sodium dihydrogenphosphate and tri-sodium phosphate were purchased from Wako Pure Chemical Industries, Ltd. (Osaka, Japan). TNBS (2,4,6-trinitrobenzene sulfonic acid), ANS (1,8-anilinonaphthalenesulfonate), and DTNB (5,5ʹ-dithiobis (2-nitrobenzoic acid)) were purchased from Sigma Aldrich (St. Louis, MO, USA). Analytical grade hydrochloric acid and sodium hydroxide were purchased from Carlo Erba Reagents (Carlo Erba, Milan, Italy). All chemicals were used directly from the container without further purification. Distilled and de-ionized water were used for preparation of all buffer solutions. The pH of all buffer solutions used in this study was measured using a pH metre (SevenEasyTM pH Meter S20, Mettler-Toledo, Schwerzenbach, Switzerland).
Preparation of Protein Solution with Different Alcohol Contents
PLEW was used without pH and solid content adjustments. Nitrogen content of PLEW was determined using Kjeldahl method[Citation41] and protein content was calculated from the Kjeldahl nitrogen with the conversion factor of 6.25. Moisture of PLEW was evaluated according to AOAC.[Citation41] The density of PLEW was determined using a10-mL glass pycnometer (BRAND, Wertheim, Germany).
PLEW solutions with different alcohol contents were prepared by first equilibrating PLEW to room temperature (25–28°C) for 1 h. Then, the PLEW solutions were mixed with methanol, ethanol or propanol to get the final 0–20 wt% alcohol with moderately stirring for 10 min. Then, the pH was adjusted to 9.2 ± 0.1 using 0.1 N HCl or 0.1 N NaOH. All PLEW solutions were continually stirred at room temperature for 1 h to ensure that the solutions were well mixed. All solution containers were wrapped with aluminum foil then sealed the edge with paraffin film while stirring to minimize the solvent evaporation.
Turbidity Measurements
The turbidity of the PLEW solutions treated with alcohol was measured using a multimode microplate reader (Model SPARK 10 M, Tecan Austria, GesmbH, Grödig, Austria). Briefly, the PLEW solutions (200 µL) treated with alcohol were transferred to a UV-Star 96-well microplate (Costar, Corning, USA). The turbidity of PLEW solution caused by protein aggregation was recorded as the optical density (OD) at 600 nm. Deionized water was used as blank. Solution with initial OD higher than 1.0 was diluted with alcohol solution corresponding to its alcohol concentration in PLEW and adjusted its pH to 9.2 ± 0.1 using 0.1 N HCl or 0.1 N NaOH until the OD of the diluted solution was less than 1.0. The turbidity was calculated as follow:
Turbidity = OD600 х Dilution factor
where,OD600 is absorbance of sample at 600 nm, dilution factor is [Volume of diluted sample/ Volume of original sample].
Exposed and total free Sulfhydryl groups
The influence of alcohol alkyl chain length and concentration on the exposed and total free sulfhydryl (SH) groups of the alcohol-treated PLEW solutions was studied using Ellman’s reagent [5,5ʹ-dithiobis (2-nitrobenzoic acid)] or DTNB as described by Ai and coworkers[Citation42] with modification. Briefly, 500 µL of alcohol-treated PLEW solutions were mixed with 500 µL of a standard buffer pH 8.0 ± 0.1 (86 mM TRIS, 90 mM glycine and 4 mM EDTA). PLEW solution (1 mL) was mixed with 10 μL of Ellman’s reagent (4 mg of DTNB/mL of standard buffer) then allowed to incubate at room temperature for 1 h. The mixture was centrifuged at 12,000хg, 4°C for 10 min. The supernatant was collected and absorbance was recorded at 412 nm using a UV-Vis spectrophotometer (Helios Alpha, Thermo Electron Corporation, England) to determine exposed free sulfhydryl groups. The total free sulfhydryl group content was determined using the same technique with a denaturing agent (8 M urea and 0.5% (w/v) SDS) in the buffer. The standard buffer was used as blank. Free sulfhydryl groups (μmole SH/g protein) of each sample were calculated using a molar extinction coefficient of 1.36х104 M−1cm−1.
Surface Hydrophobicity
The surface hydrophobicity (S0) of the alcohol-treated PLEW solutions was determined using 1,8-anilinonapthalenesulfonate (ANS−) as described by Zhou and coworkers.[Citation43] ANS− stock solution (8 mM) was prepared by dissolving the ANS− in 5 mM of phosphate buffer (pH 7.0). The protein suspensions of 0.004–0.020 wt% of PLEW were prepared in 10 mM of phosphate buffer (pH 7.0). Diluted protein suspension (4 mL) was mixed with 20 µL of ANS− stock solution and vortexed for 5 s. The fluorescence intensity (FI) of the mixtures was measured at an excitation wavelength of 370 nm and emission wavelength of 470 nm using a microplate reader (Model SPARK 10 M, Tecan Austria, GesmbH, Grödig, Austria). The FI of each sample was calculated by subtracting the FI of the buffer. The S0 was the initial slope of the subtracted FI versus the protein concentration calculated by linear regression analysis.
Gel hardness
The influence of the alkyl chain length of alcohols on the gel hardness of the 10 wt% proteins was determined by transferring the alcohol-treated PLEW solutions (25 mL) to sealed 50-mL polypropylene screw-capped centrifuge tubes (Corning Incorporated, NY) and heating at 75°C for 0, 5, 10, 20, 30 and 40 min using shaking water bath (Memmert, Germany). Heated samples were immediately cooled using an ice bath for 10 min, then stored at 4°C for overnight. Prior to analysis, sample tubes were equilibrated to room temperature (about 25–28°C) for 2 h. The samples were uncapped and subjected to a compression using a TA-XT2i texture analyzer (Stable Micro Systems, Godalming, UK) equipped with the 1/2” Cylinder Delrin probe at a crosshead speed of 2 mm/s until reach to a maximum Cauchy strain of 0.5 with trigger force of 3.0 g. The maximum compression force (Fmax) of PLEW gel was reported.
Expressible liquid
The influence of alkyl chain length of alcohols on the expressible liquid of the 10 wt% protein gels was determined by transferring alcohol-treated PLEW solutions (20 mL) to 2.5 cm diameter plastic casing sealed at both ends, which were then boiled for 15 min in a water bath (Boiling-Sterilizer, Applied Medic Ltd., Thailand). Boiled samples were immediately cooled in an ice bath for 10 min and stored at 4°C overnight. Prior to measurement, samples were equilibrated to room temperature (about 25–28°C) for 2 h. The percentage of the expressible liquid from the gel was determined by a modified method from Gao and coworkers[Citation44] in which the PLEW gels were cut into a cylinder shape (2.0 cm high х 2.5 cm diameter), weighed, then placed between double layers of filter papers (Whatman No. 4) and compressed using a TA-XT2i texture analyzer (Stable Micro Systems, Godalming, UK) equipped with a 50 mm diameter aluminum probe at a crosshead speed 3 mm/s to 70% strain and held for 60 s. Moisture of the samples before and after compression was determined using a moisture analyzer MA 30 (Sartorius, Germany). The expressible liquid content (%) was calculated from the following equation:
Expressible liquid (%) = 100 х {(moisture content of PLEW gel before compression – moisture content of PLEW gel after compression)/(moisture content of PLEW gel before compression)}.
Analysis of thermal properties
Thermal properties of the alcohol-treated PLEW were evaluated using a differential scanning calorimeter (DSC) model 822E (Mettler-Toledo GmbH, Switzerland). Initially, PLEW solution was mixed with distilled water and alcohol to a final concentration of 10 wt% protein and 5 wt% alcohol. The solutions were adjusted the pH to 9.2 ± 0.1 with 0.1 N NaOH and 0.1 N HCl then stirred for at least 2 h. All solution containers were wrapped with aluminum foil then sealed the edge with paraffin film while stirring to minimize the solvent evaporation. Protein solution without alcohol was used as control. Each alcohol-treated PLEW solution (about 10 mg) was transferred to the sample pan (40-µL aluminum DSC hermetic pan) then sealed. The sample pan was placed in the sample cell of the DSC instrument and the empty pan was placed in the reference cell. The heat flow required to keep the two cells thermally balanced was then recorded as their temperatures were increased from 30 to 100°C at the rate of 10°C per min. The cells were then held at 100°C for 5 min, cooled back to 30°C and rescanned. The first scan was of native protein and the second scan was of heat-treated protein. The data were normalized with respect to protein concentration, determined using Lowry’s method[Citation45,Citation46] with BSA as a standard protein. Peak temperature (Tm) and enthalpy (ΔH) were analyzed using the STARe Thermal Analysis software 14.00.
Gel microstructure observation
Microstructure of heat-induced PLEW gel with alcohols of different alkyl chain lengths (methanol, ethanol, and 1-propanol) was examined using field-emission scanning electron microscope (FE-SEM) model SU5000 (Hitachi High Technologies Corporation, Japan). According to the method described by Su et al. (2015)[Citation47] with a slight modifications. The gel containing 10 wt% protein was cut into 0.5 cm3 cube, then immerged in 2.5% (V/V) glutaraldehyde overnight. The fixed gel was washed with phosphate buffer (pH 7.2–7.4) for 30 min. The gel was dehydrated using gradient concentration of ethanol (60%, 70%, 80%, 90% and 95% V/V) for 30 min each step[Citation14] then the ethanol was volatilized in the fume hood for 30 min. The gel was pre-frozen at −80°C and freeze dried for 2 days. Microstructure was performed using the FE-SEM.
Statistical analysis
Experiments were performed in triplicate using freshly prepared samples at different times. Completely randomized design (CRD) was used in this study. Measurements were repeated three times on the same sample. The averages and standard deviations were calculated from these measurements using a spreadsheet (Microsoft Excel, Microsoft Corporation, Redmond, WA). Data were analyzed by analysis of variance (ANOVA) using SPSS version 11.5 for windows. Duncan’s multiple range tests was used to compare the mean with significance determined at p < 0.05.
RESULTS AND DISCUSSION
The PLEW used in this study had an experimentally determined solids content of 12.43 ± 0.50 g/L, protein content of 10.73 ± 0.11 wt%, pH of 9.22 ± 0.02 and density of 1.03 ± 0.00 g/mL.
Influence of alcohol concentration and alkyl chain length of alcohol on turbidity of PLEW
The influence of alcohol concentration and alkyl chain length on the turbidity of the PLEW is shown in . PLEW solutions without alcohol served as the control and had a low turbidity (0.18 ± 0.02), which indicates that PLEW contains small particles that can be attributed to the aggregation of the egg white protein molecules.
Figure 1. Influence of alcohol alkyl chain length and concentration on the turbidity (measured by optical density (OD600)) of pasteurized liquid egg white (PLEW) solutions
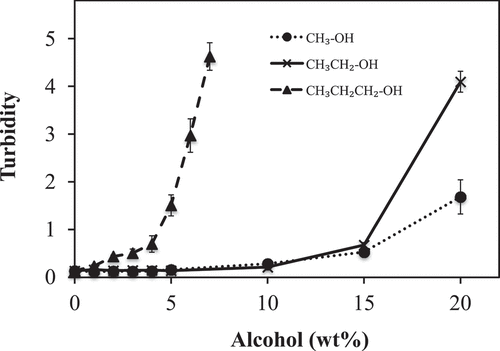
Increasing the alcohol concentration caused a significant increase (p < 0.05) on the turbidity of the PLEW solutions and the longer the alkyl chain length of the alcohol, the greater the impact on turbidity. The longest alkyl chain length alcohol used in this study, propanol, had the most profound impact on turbidity. PLEW became a gel in the presence of propanol at concentrations higher than 7.0 wt%, so here we can report only on the effect of 0–7 wt% of propanol on the turbidity of PLEW. The dramatically increased of the turbidity of PLEW in the presence of propanol suggested that propanol enhanced the aggregation between protein molecules.
The impact on turbidity of the PLEW with the medium alkyl chain length (ethanol) and shortest alkyl chain length (methanol) used in this study can be divided into two regions based on concentration: a no impact region from 0 to 10 wt% and strong impact region from 10 to 20 wt% (). Unlike the PLEW solutions with the longest alkyl chain length alcohol (propanol), these results indicate that addition of up to 10 wt% ethanol and methanol had little effect on the balance of the attractive forces (hydrophobic interactions and van der Waals forces) and electrostatic repulsive forces that would have caused the turbidity of the PLEW to increase. However, ethanol and methanol concentrations between 10 and 20 wt% resulted in greater than 300-fold and 130-fold increases in the turbidity of the PLEW, respectively. This indicates that these concentrations enhance molecular aggregation of smaller egg white protein molecules into large particles. It has been reported that increase in the ethanol concentration resulted in the increasing of turbidity of egg white and gliadin-polyphenol haze.[Citation15,Citation48] Our data suggest that high ethanol and methanol concentrations in the PLEW tilted the balance in favour of attractive forces (hydrophobic interactions and van der Waals forces) compared to repulsive forces (electrostatic repulsive forces) resulting in the increase in protein aggregation.
The highest turbidity observed in this study, found in the PLEW in the presence of propanol, could be attributed to increased aggregation of protein. Conversely, the lowest turbidity observed in this study, found in the PLEW in the presence of methanol, could be attributed to less aggregation of protein. These phenomena could be explained as; (1) PLEW in the presence of propanol (long alkyl chain length) could have highest attractive forces (hydrophobic interaction) and (2) the PLEW in the presence of methanol could have low attractive forces.
The presence of alcohol in PLEW could decrease the dielectric constant of the aqueous phase, resulting in a decrease of the repulsive forces between protein molecules and could disrupt the hydrogen bonds between the protein and water. This would promote protein-protein interactions and could alter the unfolding of the protein molecules. The result would be conformational changes in the protein structure that favor attractive forces. This could explain why it has been reported that both pH and alcohol concentration were key factors to enhance the turbidity of protein in beer.[Citation48]
Influence of alcohol concentration and alkyl chain length of alcohol on the exposed and total free sulfhydryl (SH) groups of PLEW
The influence of the concentration and alkyl chain length of alcohols (methanol, ethanol and propanol) on exposed and total free SH groups of proteins were studied (). PLEW without alcohol served as the control and the exposed and total free SH groups of the control proteins were 0.46 ± 0.03 and 50.19 ± 0.12 μmol/g protein, respectively. Neither the concentration nor the alkyl chain length of alcohol had any impact (p ≥ 0.05) on the total free SH groups of PLEW.
Figure 2. Influence of alcohol alkyl chain length and concentration on the exposed and total free sulfhydryl groups of pasteurized liquid egg white (PLEW) solutions
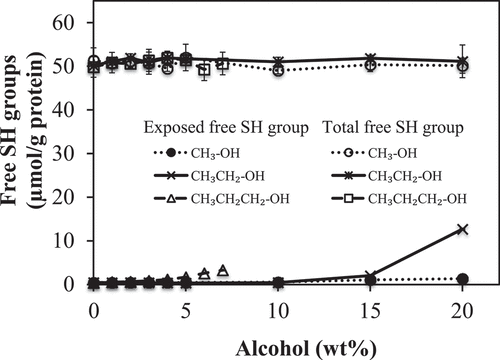
With respect to exposed free SH groups, the short alkyl chain length alcohol (methanol) had no impact (p ≥ 0.05) at any concentration on exposed free SH groups. The medium and long alkyl chain length alcohols (ethanol, propanol) did have a significant impact (p < 0.05) on the exposed free SH groups in a concentration-dependent manner. For ethanol, the effect of concentration of exposed free SH groups was similar for that of turbidity in that it can be divided into similar regions: a low impact region from 0 to 10 wt% and a high impact region from 10 to 20 wt%. The low impact region caused no increase (p ≥ 0.05) in exposed free SH groups, however, higher ethanol concentrations significantly increased (p < 0.05) the exposed free SH groups of the PLEW, especially between 15 and 20 wt% ethanol. These results suggested that SH groups previously buried inside of the egg white proteins that were not exposed to the aqueous surface at low ethanol concentration became exposed to the aqueous surface at higher ethanol concentrations.[Citation14] This effect was especially pronounced when the ethanol concentration was higher than 15 wt%.
The addition of the long alkyl chain length alcohol (propanol) to PLEW caused gel formation at concentrations higher than 7 wt%, so here we can report only on the effect of low concentrations of propanol (0–7 wt%) on exposed free SH groups. The results show that propanol significantly (p < 0.05) increased the exposed free SH groups in a concentration dependent manner. This suggests that propanol facilitates the exposure of the SH groups previous buried inside of the egg white protein molecules to the aqueous surface. Compared to ethanol, propanol had more impact on the increase of exposed free SH groups of PLEW from 0 to 7 wt%. Thus, propanol (long alkyl chain length) was a more effective facilitator of the exposure free SH groups in the PLEW than that of ethanol (medium alkyl chain length).
Influence of alcohol concentration and alkyl chain length of alcohol on the surface hydrophobicity of the PLEW
The effect of concentration and alkyl chain length of alcohols (methanol, ethanol and propanol) on the hydrophobicity (S0) of PLEW was investigated (). PLEW without alcohol served as the control with a S0 of 106.16 ± 4.78х103. Although the results show that concentration and alkyl chain length of alcohol had a significant impact (p <0 .05) on the S0 of PLEW, methanol had lowest and propanol had highest impact on the S0. Low concentrations (0–10 wt%) of methanol (short alkyl chain length) had no impact (p ≥ 0.05) on the S0 of the PLEW. However, at concentrations higher than 10 wt%, methanol had a significant impact (p < 0.05) on the S0 that increased with increasing of the methanol concentration. These data are similar to the results of previous work[Citation49] where high methanol concentrations caused more hydrophobic groups of protease molecules were exposed to the solvents. Very low ethanol concentrations (0–2 wt%) had no impact (p ≥ 0.05) on the S0 of the PLEW. However, at ethanol concentration higher than 2 wt%, ethanol caused a significant increase in S0 (p < 0.05) of the PLEW that increased with increasing of the ethanol concentration. The addition of ethanol from 0 to 20 wt% resulted in a 72% increase of S0. The addition of the long alkyl chain length alcohol (propanol) to PLEW caused gel formation at concentrations higher than 7 wt%, so here we can report only on the effect of low concentrations of propanol (0–7 wt%) on S0. Propanol (long alkyl chain length) had strong impact on the S0 of PLEW. Increasing the propanol concentration from 0 to 7 wt% resulted in a 314% increase of S0.
Figure 3. Influence of alcohol alkyl chain length and concentration on the surface hydrophobicity (S0) of pasteurized liquid egg white (PLEW) solutions
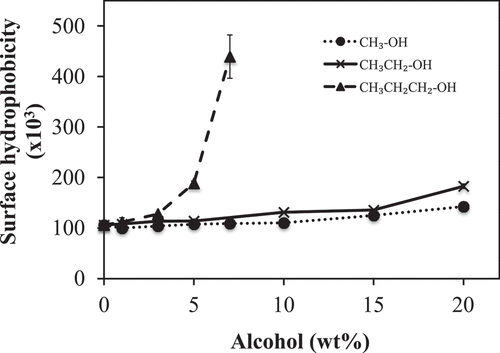
The S0 of protein depends on the amount of hydrophobic amino acid residues located at the protein surface. The increased S0 of the PLEW with increasing the alcohol concentration could be due to the unfolding of the egg white protein in the presence of alcohol, which could affect the ability of the ANS− probe to bind to the nonpolar site of the protein molecule. Alkyl chain length and polarity are inversely correlated such that propanol has the longest alkyl chain length and lowest polarity, while methanol has the lowest alkyl chain length and highest polarity. The solvent surrounding the PLEW in the presence of propanol has a low polarity and likely decreased the dielectric constant of the solvent surrounding PLEW protein molecules more than ethanol or methanol, facilitating greater unfolding of the protein and more surface hydrophobicity. Chang and coworkers also found that lentil protein isolate treated with ethanol and propanol enhanced surface hydrophobicity to produce emulsions as compared with the untreated lentil protein isolate.[Citation50]
Impact of alkyl chain length of alcohol on the denaturation temperature of the PLEW
The influence of alkyl chain length of alcohols (methanol, ethanol and propanol) on the denaturation temperature (Tm) of the PLEW was studied. The thermographs of PLEW in its native state and heat-treated, with and without 5 wt% alcohol were compared. The first scans (native PLEW with and without alcohol) indicated that an endothermic process occurred, whereas the second scans (heat-treated PLEW with and without alcohol) exhibited no thermal transition, indicating that the thermal denaturation of PLEW was irreversible in both the presence and absence of alcohol. The same phenomenon was also found for whey protein in the presence of sucrose or sorbitol,[Citation51] BSA in the presence of glycerol,[Citation52] BSA in the presence of sorbitol,[Citation17] and β-lactoglobulin in the presence of sorbitol and glycerol.[Citation53]
Proteins change their state from native to denature when they are heated above their denaturation temperature. The endothermic transition observed for the PLEW has been attributed to the thermal denaturation of proteins,[Citation51] and it has been suggested that the thermal denaturation of proteins leads to substantial changes in protein conformation and aggregation, as well as a redistribution of disulfide bonds and free SH groups within and between protein molecules.[Citation54,Citation55] These changes likely account for the irreversible nature of the thermal transition.
The effect of alkyl chain length of alcohol on the thermal transition temperature (Tm) of PLEW is shown in . Thermographic scans of the PLEW without alcohol displayed endothermic transitions between ~62 and ~94°C, with four endothermic peaks at 66.59, 78.20, 82.90 and 87.59°C. It has been reported that ovotransferin, lysozyme, ovomucoid and ovoalbumin in egg white have denaturation temperature around 61.0, 75.0, 79.0 and 84°C, respectively.[Citation39] Thus the four endothermic peaks could correspond to ovotransferin, lysozyme, ovomucoid and ovalbumin, respectively. The presence of alcohols deceased the Tm of PLEW () and increasing the alkyl chain length of alcohol significantly lowered (p < 0.05) the Tm.
Figure 4. Influence of alcohol alkyl chain length on the thermal properties of pasteurized liquid egg white (PLEW) gels containing 5 wt% alcohols. The first scan (solid line) represents native protein, while the second scan (dashed line) represents heat-treated protein
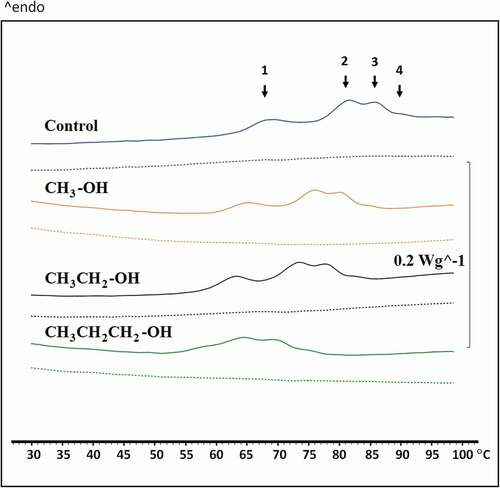
Shown in , the Tm value of ovotransferin without alcohol was 66.59°C and progressively shifted to lower temperatures in the presence of alcohols of increasing alkyl chain length. Propanol had highest and methanol had the lowest capability to reduce the Tm of the ovotransferin of the PLEW, as evidenced by the shift to 60.59, 58.34 and 52.84°C in the presence of 5 wt% methanol, 5 wt% ethanol and 5 wt% propanol, respectively. A similar effect of alcohol on the Tm of the other protein components in the PLEW was observed (). This suggests that the effect of alcohols on unfolding of PLEW proteins preferentially occurs in the order of propanol > ethanol > methanol > water.
Table 1. Influence of alcohol alkyl chain length on the denaturation temperature (Tm) and total enthalpy change during protein denaturation of pasteurized liquid egg white (PLEW). Means within a column with different superscripts are significantly different at p < 0.05
also shows the effect of alkyl chain length of alcohol on the total enthalpy change during protein denaturation and revealed that alkyl chain length had a significant (p < 0.05) impact on the total enthalpy change during denaturation. The PLEW in the presence of alcohol had lower total enthalpy change during denaturation than that of the PLEW without alcohol. The total enthalpy change during denaturation of the PLEW was progressively lowered with increasing alkyl chain length, confirming that propanol had the highest and methanol had the lowest ability to facilitate the unfolding of PLEW during heat treatment.
The ability of alcohols to alter the thermal stability of proteins can be attributed to the preferential accumulation or exclusion of alcohol (cosolvent) molecules around the protein surface compared to water (solvent) molecules.[Citation56,Citation57] Two different physicochemical phenomena contribute to the preferential accumulation or exclusion of cosolvent and solvent molecules around proteins, namely steric exclusion and differential interactions.[Citation5] The steric exclusion contribution depends on the relative size of the cosolvent and solvent molecules, whereas the differential interaction contribution depends on differences in the strength of the molecular interactions between the cosolvent and solvent molecules and the protein surface. Results of this study suggest that the addition of alcohols to PLEW deceased the free energy difference between the unfolded state and folded states of the protein, thereby decreasing the thermal stability of the protein.[Citation55,Citation58] Propanol is almost twice the molecular weight of methanol (60 g/mol, 32 g/mol), so it seems likely that differential interaction effects may play an important role in stabilizing the protein. In other words, PLEW protein molecules preferred to be surrounded by alcohol molecules.
Impact of alkyl chain length of alcohol on the hardness of the PLEW gels
The effect of 5 wt% of alcohols with increasing alkyl chain length (methanol, ethanol and propanol) on the gel hardness of PLEW is shown in . Gelation can occur as proteins become denatured when heated, causing the structure to unfold and expose and aggregate the hydrophobic residues that were previously buried inside the core of the molecule.[Citation14] The results showed that both heating time and alkyl chain length caused a significant (p < 0.05) increase in gel hardness (Fmax) of the PLEW. PLEW solutions with and without 5 wt% alcohol did not gel during the first 5 min of heating. However, all PLEW solutions gelled when they were heated longer than 5 min. These results are likely due to the fact that heating PLEW for less than 5 min was not enough to unfold the protein,[Citation14] but the protein molecules subsequently unfolded and aggregated when they were heated longer than 5 min. Yu and coworkers[Citation14] also indicated that heating time and ethanol can significantly enhance the gel hardness of duck egg white.
Influence of alcohol concentration and alkyl chain length of alcohol on the expressible liquid of PLEW gels
shows the impact of alcohol concentration and alkyl chain length of alcohol on the expressible liquid of PLEW gels. The expressible liquid of the gel was about 6.88 ± 0.32 g liquid/100 g gel for the 0% alcohol (control) PLEW gels. Addition of alcohol significantly (p < 0.05) increased the expressible liquid of the PLEW gels in a concentration dependent manner. Longer alkyl chain length also caused an increase in expressible liquid. For example, increasing the alcohol concentration from 0 to 2 wt% resulted in a 14, 34 and 82% increase of expressed liquid of the PLEW gels for methanol, ethanol and propanol, respectively.
Figure 6. Influence of alcohol alkyl chain length and concentration on the expressible liquid of pasteurized liquid egg white (PLEW) gels
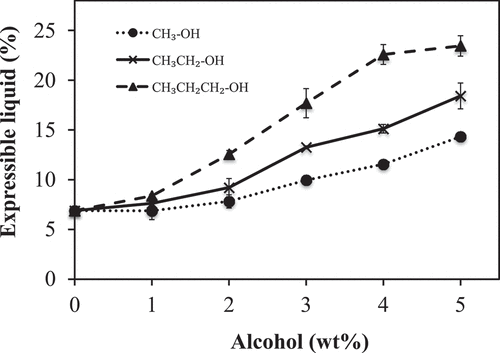
The results suggest that PLEW gels with and without alcohol consist of a protein matrix network that traps water and alcohol inside the network. The expressible liquid of PLEW gels is related to the ability of the gel to hold liquid and other soluble molecules inside the protein network.[Citation14] These results indicate that the expressible liquid did not bind tightly to the protein molecules and was loosely trapped in the gel matrix. We hypothesize that the presence of alcohol altered the strength of the water–protein interaction and the higher concentration of alcohol disrupted hydrogen bonding among water molecules trapped inside the gels, which resulted in the increase of the expressible liquid of the PLEW gels. This phenomenon likely is exacerbated by alcohols with longer alkyl chain length because they have lower polarity and would alter the protein conformation resulting in the change of the protein network and weakened protein-water interactions.
Impact of alkyl chain length of alcohol on the microstructure of the PLEW gels
shows the impact of alkyl chain length of alcohol on the scanning electron microscope (SEM) images of the cross-section of PLEW gels. Addition of alcohol at the level of 5 wt% was able to alter the microstructure of the PLEW gels in an alkyl chain length dependent manner. This microstructure could relate to the results of expressible liquid when the force was applied and the addition of longer alkyl chain length alcohol caused the lower capability of trapping liquid in the gel matrix. The differences in microstructure confirmed that polarity of solvent as affected by addition of alcohol with different alkyl chain length had influence on the molecular interactions of PLEW gels resulting in the gel properties. Yu and coworkers[Citation14] also found that ethanol can change microstructure of duck egg white gel as observed by SEM.
CONCLUSION
Alcohol concentration and type of alcohol can alter the physicochemical and gel properties of PLEW. However, this research clearly showed that the type of alcohol, in this case differentiated by alkyl chain length, plays a much more important role in altering physicochemical and gel properties of PLEW. Propanol had the most and methanol the least impact on the alteration of all the dependent variables measured in this study. Compared to methanol and ethanol, propanol caused an increase in turbidity, surface hydrophobicity, and exposed free SH groups, and a decrease in denaturation temperature and gel hardness. It is widely acknowledged that the factors that impact the functional characteristics of food proteins are governed by their molecular structure the characteristics of the solvent surrounding the proteins. The nexus of research in this area has focused on ionic strength and pH. The practical implications of this research lie in the connection between what already is known about solvent interactions with proteins and alcoholic denaturation. The profound impact of propanol on the physicochemical and gelation properties of PLEW suggest a research approach that focuses on food grade solvents of different alkyl chain lengths and their potential for use as cold-denaturants that positively impact food quality.
Acknowledgments
The authors gratefully acknowledge the financial support provided by NSTDA, Thailand.
References
- Damodaran, S. Amino Acids, Peptides and Proteins. In Fennema’s Food Chemistry, 5th ed.; Damodaran, S., Parkin, K. L., Eds.; CRC Press: Boca Raton, 2017; pp 235–356.
- Bryant, C. M.; McClements, D. J. Influence of NaCl and CaCl2 on Cold-set Gelation of Heat-denatured Whey Protein. J. Food Sci. 2000, 65(5), 801–804. DOI: https://doi.org/10.1111/j.1365-2621.2000.tb13590.x.
- Damodaran, S. Food Proteins: An Overview. In Food Proteins and Their Applications, S. Damodaran, S., Paraf, A., Eds. 1997; Marcel Dekker: New York, 1997, pp 1–24.
- Gharbi, N.; Labbafi, M. Effect of Processing on Aggregation Mechanism of Egg White Proteins. Food Chem. 2018, 252, 126–133. DOI: https://doi.org/10.1016/j.foodchem.2018.01.088.
- McClements, D. J. Modulation of Globular Protein Functionality by Weak Interaction Cosolvents. CRC Critical Reviews in Food Sci. Nutr. 2002, 42(5), 417–471. DOI: https://doi.org/10.1080/20024091054210.
- Schmidt, R. H. Gelation and Coagulation. Protein Functionality in Foods. Cherry, J. P. Ed.. ACS Symposium Series, 147, American Chemical Society: Washington, D.C., 1981, Chap. 7. pp 131–147
- Ziegler, G. R.; Foegeding, E. A. The Gelation of Proteins. Adv. Food Nut. Res. 1990, 34, 203–298.
- McClements, D. J.; Zou, L.; Zhang, R.; Salvia-Trujillo, L.; Kumosani, T.; Xiao, H. Enhancing Nutraceutical Performance Using Excipient Foods: Designing Food Structures and Compositions to Increase Bioavailability. Compr. Rev. Food Sci. Food Saf. 2015, 14(6), 824–847. DOI: https://doi.org/10.1111/1541-4337.12170.
- Zayas, J. F. Functionality of Proteins in Food; Springer-Verlag: New York, 1997.
- Foegeding, E. A.; Luck, P. J.; Davis, J. P. Factors Determining the Physical Properties of Protein Foams. Food Hydrocolloids. 2006, 20(2–3), 284–292. DOI: https://doi.org/10.1016/j.foodhyd.2005.03.014.
- Doi, E. Gels and Gelling of Globular-proteins. Trends Food Sci. Technol. 1993, 4(1), 1–5. DOI: https://doi.org/10.1016/S0924-2244(05)80003-2.
- Liu, T. X.; Relkin, P.; Launay, B. Thermal Denaturation and Heat-induced Gelation Properties of β-lactoglobulin. Effect of Some Chemical Parameters. Thermochim. Acta. 1994, 246(2), 387–403. DOI: https://doi.org/10.1016/0040-6031(94)80104-5.
- Chen, Z.; Li, J.; Tu, Y.; Zhao, Y.; Luo, X.; Wang, J.; Wang, M. Changes in Gel Characteristics of Egg White under Strong Alkali Treatment. Food Hydrocolloids. 2015, 45, 1–8. DOI: https://doi.org/10.1016/j.foodhyd.2014.10.026.
- Yu, L.; Xiong, C.; Li, J.; Luo, W.; Xue, H.; Li, R.; Tu, Y.; Zhao, Y. Ethanol Induced the Gelation Behavior of Duck Egg Whites. Food Hydrocolloids. 2020, 105, 105765. DOI: https://doi.org/10.1016/j.foodhyd.2020.105765.
- Yao, L.; Jiang, A.; Chen, L. Characterization of Ethanol-induced Egg White Gel and Transportation of Active Nutraceuticals. LWT Food Sci. Technol. 2020, 130, 109530. DOI: https://doi.org/10.1016/j.lwt.2020.109530.
- Bryant, C. M.; McClements, D. J. Molecular Basis of Protein Functionality with Special Consideration of Cold-set Gels Derived from Heat-denatured Whey. Trends Food Sci. Technol. 1998, 9(4), 143–151. DOI: https://doi.org/10.1016/S0924-2244(98)00031-4.
- Baier, S. K.; McClements, D. J. Impact of Sorbitol on the Thermostability and Heat-induced Gelation of Bovine Serum Albumin. Food Res. Int. 2003, 36(9–10), 1081–1087. DOI: https://doi.org/10.1016/j.foodres.2003.09.003.
- Dickinson, E. Proteins in Solution and at Interfaces. In Interactions of Surfactant with Polymers and Proteins; Goddard, E. D., Ananthapadmanabhan, K. P., Eds.; CRC Press: Boca Raton, FL, 1993; pp 295–318.
- Totosaus, A.; Montejano, J. G.; Salazar, J. A.; Guerrero, I. A Review of Physical and Chemical Protein-gel Induction. Int. J. Food Sci. Technol. 2002, 37(6), 589–601. DOI: https://doi.org/10.1046/j.1365-2621.2002.00623.x.
- Li, J.; Li, X.; Wang, C.; Zhang, M.; Xu, Y.; Zhou, B.; Su, Y.; Yang, Y. Characteristics of Gelling and Water Holding Properties of Hen Egg White/yolk Gel with NaCl Addition. Food Hydrocolloids. 2018, 77, 887–893. DOI: https://doi.org/10.1016/j.foodhyd.2017.11.034.
- Weijers, M.; van de Velde, F.; Stijnman, A.; van de Pijpekamp, A.; Visschers, R. W. Structure and Rheological Properties of Acid-induced Egg White Protein Gels. Food Hydrocolloids. 2006, 20(2–3), 146–159. DOI: https://doi.org/10.1016/j.foodhyd.2005.02.013.
- Gopinath, A.; Shanmugam, G.; Madhan, B.; Rao, J. R. Differential Behavior of Native and Denatured Collagen in the Presence of Alcoholic Solvents: A Gateway to Instant Structure Analysis. Int. J. Biol. Macromol. 2017, 102, 1156–1165. DOI: https://doi.org/10.1016/j.ijbiomac.2017.04.121.
- Yoshida, K.; Fukushima, Y.; Yamaguchi, T. A Study of Alcohol and Temperature Effects on Aggregation of β-lactoglubulin by Viscosity and Small-angle X-ray Scattering Measurements. J. Mol. Liq. 2014, 189, 1–8. DOI: https://doi.org/10.1016/j.molliq.2013.06.022.
- Buck, M. Trifluoroethanol and Colleagues: Cosolvents Come of Age. Recent Studies with Peptides and Protein. Quarterly Review of Biophysics. 1998, 31(3), 297–355. DOI: https://doi.org/10.1017/S003358359800345X.
- Nikolaidis, A.; Andreadis, M.; Moschakis, T. Effect of Heat, pH, Ultrasonication and Ethanol on the Denaturation of Whey Protein Isolate Using a Newly Developed Approach in the Analysis of Difference-UV Analysis. Food Chem. 2017, 215, 235–244. DOI: https://doi.org/10.1016/j.foodchem.2016.07.133.
- Shiraki, K.; Nishikawa, K.; Goto, Y. Trifluoroethanol-induced Stabilization of the α-helical Structure of β-lactoglobulin: Implication for Non-hierarchical Protein Folding. J. Mol. Biol. 1995, 245(2), 180–194. DOI: https://doi.org/10.1006/jmbi.1994.0015.
- Uversky, V. N.; Narizhneva, N. V.; Kirschstein, S. O.; Winter, S.; Lӧber, G. Conformational Transitions Provoked by Organic Solvents in β-lactoglobulin: Can a Molten Globule Like Intermediate Be Induced by the Decrease in Dielectric Constant? Folding and Design. 1997, 2(3), 163–172. DOI: https://doi.org/10.1016/S1359-0278(97)00023-0.
- Ishino, K.; Kudo, S. Gelation Phenomena Induced by Alkali-alcohol Treatment of 7s and 11s Components in Soybean Globulin. Agric Biol Chem. 1977, 41(8), 1347–1352.
- Miyawak, O.; Tatsuno, M. Thermodynamic Analysis of Alcohol Effect on Thermal Stability of Protein. J. Biosci. Bioeng. 2011, 111(2), 198–201. DOI: https://doi.org/10.1016/j.jbiosc.2010.09.007.
- Nikolaidis, A.; Moschakis, T. On the Reversibility of Ethanol-induced Whey Protein Denaturation. Food Hydrocolloids. 2018, 84, 389–395. DOI: https://doi.org/10.1016/j.foodhyd.2018.05.051.
- Sasaki, M.; Sasaki, T.; Kobayashi, M.; Kise, H. Time-dependent Structure and Activity Changes of α-chymotrypsin and Water/alcohol Mixed Solutions. Biosci. Biotechnol. Biochem. 2000, 64(12), 2552–2558. DOI: https://doi.org/10.1271/bbb.64.2552.
- Kamatari, Y. O.; Konno, T.; Kataoka, M.; Akasaka, K. The Method-induced Transition and the Expanded Helical Conformation in Hen Lysozyme. Protein Sci. 1998, 7, 681–688. DOI: https://doi.org/10.1002/pro.5560070317.
- Walker, A. R.; Baddam, N.; Cisneros, G. A. Unfolding Pathways of Hen Egg-white Lysozyme in Ethanol. Int. J. Biol. Macromol. 2019, 123(15), 3267–3271.
- Magsumov, T.; Li, Z. Y.; Sedov, I. Comparative Study of the Protein Denaturing Ability of Different Organic Cosolvents. Int. J. Biol. Macromol. 2020, 160(3), 880–888. DOI: https://doi.org/10.1016/j.ijbiomac.2020.05.260.
- Yoshikawa, H.; Hirano, A.; Arakawa, T.; Shiraki, K. Mechanistic Insights into Protein Precipitation by Alcohol. Int. J. Biol. Macromol. 2012, 50(3), 865–871. DOI: https://doi.org/10.1016/j.ijbiomac.2011.11.005.
- Dufour, E.; Robert, P.; Renard, D.; Llamas, G. Investigation of β-lactoglobulin Gelation in Water/ethanol Solutions. Int. Dairy J. 1998, 8(2), 87–93. DOI: https://doi.org/10.1016/S0958-6946(98)00024-7.
- Renard, D.; Lefebvre, J.; Robert, P.; Llamas, G.; Dufour, E. Structural Investigation of β-lactolobulin Gelation in Ethanol/water Solutions. Int. J. Biol. Marcromol. 1999, 26(1), 35–44. DOI: https://doi.org/10.1016/S0141-8130(99)00060-4.
- Mine, Y. Recent Advances in the Understanding of Egg-white Protein Functionality. Trends Food Sci. Technol. 1995, 6(7), 225–232. DOI: https://doi.org/10.1016/S0924-2244(00)89083-4.
- Stadelman, W. J.; Cotterill, O. J. Egg Science and Technology, 4th ed.; Haworth Food Products Press: New York, 1995.
- Wu, H.; Xie, J.; Morbidelli, M. Kinetics of Cold-set Diffusion-limited Aggregations of Denatured Whey Protein Isolate Colloids. Biomacromolecules. 2005, 6(6), 3189–3197. DOI: https://doi.org/10.1021/bm050532d.
- AOAC. AOAC Official Method 925.31. InOfficial Methods of Analysisof AOAC International,21st ed.; Latimer, G. W., Ed.; AOAC International: Rockville:MD, 2019.
- Ai, M.; Zhou, Q.; Xiao, N.; Guo, S.; Cao, Y.; Fan, H.; Ling, Z.; Zhou, L.; Li, S.; Long, J.; et al. Enhancement of Gel Characteristics of NaOH-induced Duck Egg White Gel by Adding Ca(OH)2 With/without Heating Comparative Study of High Intensity Ultrasound Effects on Food Proteins Functionality. Food Hydrocolloids. 2020, 103, 105654. DOI: https://doi.org/10.1016/j.foodhyd.2020.105654.
- Zhou, L.; Yang, Y.; Wang, J.; Wei, S.; Li, S. Effect of Low Fat Addition on Chicken Myofibrillar Protein Gelation Properties. Food Hydrocolloids. 2019, 90, 126–131. DOI: https://doi.org/10.1016/j.foodhyd.2018.11.044.
- Gao, Y.; Fukushima, H.; Deng, S.; Jia, R.; Osako, K.; Okazaki, E. Effect of Emulsifying Stability of Myofibrillar Protein on the Gel Properties of Emulsified Surimi Gel. Food Sci. Nutr. 2018, 6(5), 1229–1237. DOI: https://doi.org/10.1002/fsn3.663.
- Lowry, O. H.; Rosebrough, N. J.; Farr, A. L.; Randall, R. J. Protein Measurement with the Folin Phenol Reagent. J. Biol. Chem. 1951, 193(1), 265–275. DOI: https://doi.org/10.1016/S0021-9258(19)52451-6.
- Deepachandi, B.; Weerasinghe, S.; Andrahennadi, T. P.; Karunaweera, N. D.; Wickramarachchi, N.; Soysa, P.; Siriwardana, Y. Quantification of Soluble or Insoluble Fractions of Leishmania Parasite Proteins in Microvolume Applications: A Simplification to Standard Lowry Assay. Int. J. Anal. Chem. 2020, 6129132. DOI: https://doi.org/10.1155/2020/6129132.
- Su, Y.; Dong, Y.; Niu, F.; Wang, C.; Liu, Y.; Yang, Y. Study on the Gel Properties and Secondary Structure of Soybean Protein Isolate/egg White Composite Gels. Eur. Food Res. Technol. 2015, 240(2), 367–378. DOI: https://doi.org/10.1007/s00217-014-2336-3.
- Sibert, K. J.; Lynn, P. Y. Effect of Alcohol and pH on Protein-polyphenol Haze Intensity and Particle Size. J. Am. Soc. Brew. Chem. 2003, 61(2), 88–98. DOI: https://doi.org/10.1094/ASBCJ-61-0088.
- Gu, Z.; Lai, J.; Hang, J.; Zhang, C.; Wang, S.; Jiao, Y.; Liu, S.; Fang, Y. Theoretical and Experimental Studies on the Conformational Changes of Organic Solvent-stable Protease from Bacillus Sphaericus DS11 in Methanol/water Mixtures. Int. J. Biol. Macromol. 2019, 128, 603–609. DOI: https://doi.org/10.1016/j.ijbiomac.2019.01.196.
- Chang, C.; Stone, A. K.; Green, R.; Nickerson, M. T. Reduction of Off-flavours and the Impact on the Functionalities of Lentil Protein Isolate by Acetone, Ethanol, and Isopropanol Treatments. Food Chem. 2019, 277, 84–95. DOI: https://doi.org/10.1016/j.foodchem.2018.10.022.
- Dierckx, S.; Huyghebaert, A. Effects of Sucrose and Sorbitol on the Gel Formation of a Whey Protein Isolate. Food Hydrocolloids. 2002, 16(5), 489–497. DOI: https://doi.org/10.1016/S0268-005X(01)00129-1.
- Baier, S. K.; Decker, E. A.; McClements, D. J. Impact of Glycerol on Thermostability and Heat-induced Gelation of Bovine Serum Albumin. Food Hydrocolloids. 2004, 18(1), 91–100. DOI: https://doi.org/10.1016/S0268-005X(03)00046-8.
- Chanasattru, W.; Decker, E. A.; McClements, D. J. Modulation of Thermal Stability and Heat-induced Gelation of β-lactoglobulin by High Glycerol and Sorbitol Levels. Food Chem. 2007, 103(2), 512–520. DOI: https://doi.org/10.1016/j.foodchem.2006.08.020.
- Baldini, G.; Beretta, S.; Chirico, G.; Franz, H.; Maccioni, E.; Mariani, P.; Spinozzi, F. Salt-induced Association of β-lactoglobulin by Light and X-ray Scattering. Macromolecules. 1999, 32(19), 6128–6138. DOI: https://doi.org/10.1021/ma990709u.
- Freire, E. Thermodynamics of Partly Folded Intermediates in Proteins. Annu. Rev. Biophys. Biomo. 1995, 24(1), 141–165. DOI: https://doi.org/10.1146/annurev.bb.24.060195.001041.
- Arakawa, T.; Bhat, R.; Timasheff, S. N. Why Preferential Hydration Does Not Always Stabilize the Native Structure of Globular Proteins. Biochemistry. 1990, 29(7), 1924–1931. DOI: https://doi.org/10.1021/bi00459a037.
- Xie, G. F.; Timasheff, S. N. Mechanism of the Stabilization of Ribonuclease A by Sorbitol: Preferential Hydration Is Greater for the Denatured than for the Native Protein. Protein Sci. 1997, 6(1), 211–221. DOI: https://doi.org/10.1002/pro.5560060123.
- Leharne, S. A.; Chowdhry, B. Z. Introduction to Differential Scanning Calorimetry. In Biocalorimetry: Aplications of Calorimetry in the Biological Sciences; Ladbury, J. E., Chowdhry, B. Z., Eds.; J. Wiley: New York, 1998; pp 157–182.