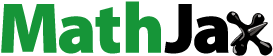
ABSTRACT
Pectin degradation in the cell walls and middle lamella greatly affects the texture and the quality of the fruit including the taste and storage time. This directly affects its acceptability, supply to the consumer market, and economic benefits. It is therefore essential to establish the relationship between the chemical changes of pectin and the texture of the fruit to improve its preservation. This study was designed to evaluate the degradation of pectin in the peels of Tarocco blood orange and the textural changes during postharvest storage and further investigate their relationship over the study period. The texture of the fruit was characterized using the texture profile analyzer and the degradation of pectin was investigated by testing the content of the pectin, degree of esterification, and galacturonic acid content. The physicochemical properties, such as weight loss, moisture content, and anthocyanin content were also measured. Furthermore, the structural changes of the pectin were analyzed by molecular weight determination and FTIR test. The results showed that the Mw of pectin decreased from 568.05 kDa to 362.55 kDa, with a 42.1% decline in pectin content for storing for 63 d. The gradual solubilization, depolymerization, and de-esterification of the pectin consequently led to the decline in the textual properties of the fruit during postharvest storage. This can be applied and lead to improvements in quality control and process design in the food industry and the marketplace for the fruit during storage and preservation.
Introduction
Citrus fruits are non-climacteric fruits that represent a group of very significant crops globally.[Citation1] In recent times, consuming blood oranges has gained increased interest due to their high nutritional value, bioactive compounds, and high antioxidant activity, especially anthocyanins, ascorbic acid, flavonoids, and hydroxycinnamic acid concentration.[Citation2,Citation3] Tarocco blood orange is one of the main cultivars of blood orange and it is appreciated for its ease of peeling, juiciness, and low Brix-acidity ratio which attenuates its sweet taste.[Citation4] Thus, conducting a study on this fruit is of great importance to meet the increasing consumer demands.
When referring to the quality of fruit, the texture is the principal attribute, being firm and juicy are the most important properties for consumers.[Citation5,Citation6] A reduction in these attributes reduces fruit quality, which leads to postharvest decay or consumer rejection and finally the economic losses. Therefore, it is essential to understand how these biochemical changes, happening during ripening and postharvest storage affect the quality.[Citation7] An understanding of the mechanical, physical, and chemical properties of the fruit is an important tool to design new and improved strategies and processes for harvesting, transportation, and other postharvest operations.[Citation8] Textural changes of several fruits have been analyzed during storage under several conditions to understand the softening process in those fruits using texture profile analysis. Fruits such as pomegranate, pears, orange, strawberries, and others have been analyzed.[Citation8–11]
Several studies found that among the plant cell components, the cell walls have the most important influence on the texture of plant tissue.[Citation12,Citation13] The cell wall adhesion, elasticity, mechanical force, turgor, among other factors, are used in determining the texture of the fruit, and as these factors vary the texture of the fruit changes as well.[Citation7,Citation14] Fruit softening has been mainly linked to the degeneration and modification of primary cell walls and middle lamella. Pectin, an imperative component of fruit cell walls and middle lamella, is extensively modified during ripening and postharvest storage and is a structural complex heteropolysaccharide that mostly consists of polymers of α-galacturonic acid (at least 65%) with a variable number of methyl ester groups.[Citation15] Changes in pectin, such as solubilization, de-esterification, and depolymerization, have been proposed as the primary causes of fruit softening during ripening as a result of cell wall loosening and disintegration.[Citation16] Several works have been conducted to understand the biochemical processes leading to pectin degradation and how it can be delayed during storage in several fruits such as pear, mango, banana, apple, avocado, plum, nectarine,[Citation17–19] and many more.
To elucidate the mechanism of fruit softening at the molecular level, the monitoring of these chemical changes as a function of the storage time is essential.[Citation12,Citation13] Understanding the mechanism of softening and deterioration of fruits during storage is not achievable without the evaluation of the mechanical properties of the cell walls, with pectin degradation being the main cause of softening of fruits during storage. The reference,[Citation20] established the link between the pectin content and texture changes during storage of different apple cultivars. Also, Defilippi et al.[Citation16] determined the changes that occurred in the cell wall pectin and its relationship to the postharvest mesocarp softening of avocados. That of apricots[Citation19] and highbush blueberries[Citation21] have also been studied. But cell wall modification mechanisms in different species and each fruit type differ and need to be investigated separately. The relationship between pectin degradation and texture has not been extensively studied for non-climacteric fruits. Estimating the chemical changes that occur on the pectin in the pericarp of Tarocco blood orange is essential; these estimations identify how the pectin is modified and how it affects the physicochemical properties and texture of the oranges.
Researches have already been done to either analyze the changes in the texture of oranges during storage/preservation or degradation of pectin during storage/preservation, but few studies have tried to connect these two phenomena. These considerations motivated the choice of this research topic to give a better understanding of the relationship between the textural changes and pectin degradation of Tarocco blood orange. This could then be applied and lead to improvements in quality control and process design in the food industry and the marketplace for the fruit during storage and preservation.
Materials and methods
Materials
Tarocco blood oranges used originated from an orchard in the Changshou District, Chongqing, China. Ripe and mature fruits were harvested in the early part of January and immediately transported to the laboratory. The chemical reagents used in this study were of analytical grade and were supplied by Aladdin Biochemical Technology Co., Ltd. (Shanghai, China) and Chengdu Kelong Co. Ltd.
Pre-treatment
Fruits were thoroughly washed and rinsed under running water to reduce surface contamination and then dried with blotting paper. They were then divided into four lots based on their size and stored in clean ventilated baskets and placed in a ventilated and thermostatic room at 16°C for 63 days. Fruits were randomly selected from all four lots for every assay.
Pectin content
The rapid and quantitative method[Citation22] was employed. This method is based on the titration of de-esterified pectin after acid precipitation. The gravitational method was also used to determine pectin content. Briefly, 10 g of the dried and pulverized peels was weighed into a 250 mL beaker and dissolved with 150 mL distilled water. The solution was boiled for 1 h with continuous stirring and then cooled and the volume increased to 250 mL. It was filtered and 25 mL of the filtrate was placed into a 500 mL beaker. Afterward, 100 mL of 0.1 M NaOH was added to the solution and left to stand for 30 min. Then 50 mL of 1 M Acetic acid was added followed by the addition of 50 mL of 1 M CaCl2, then left to stand for an hour and boiled for 5 min. The solution was then filtered and the residue was washed with hot water until there was no trace of Chloride ions present. The filter paper and residue were dried to a constant weight at 105°C and then weighed. Pectin content was calculated as shown in EquationEq. 3(3)
(3) :
where, 0.9235: Coefficient of colloid for the conversion of calcium pectinate, G: Filter slag weight (g), and W: Sample weight (g). Pectin content was also quantified using the modified carbazole assay by Yeoh et al.[Citation23] This assay is based on the colorimetric assay of the reaction of a colorless sulfuric acid-uronide product, 5-formyl-furan-carboxylic acid with carbazole to form a pink-colored product.
Pectin characterization
The degree of esterification (DE) of pectin was determined using the method described by Hosseini et al.[Citation24] DE was calculated as shown in EquationEq. 4(4)
(4) :
Galacturonic acid content (GalA) of pectin was determined according to the method reported by[Citation25] with some modifications. Briefly, 1 mL of pectin solution (100 mg/L) was mixed thoroughly with 6 mL concentrated H2SO4. and allowed to stand for 20 min. Afterward, 0.2 mL of 0.15 (w/v) % carbazole-ethanol solution was added and allowed to stand for 2 h to develop a pink color. The absorbance was recorded at 520 nm using a spectrophotometer. The GalA was calculated from a standard curve of D-(+)-Galacturonic acid (0–250 mg/L). The degree of methylation (DM) of pectin was measured according to the method described by Ogutu et. al.[Citation26] DM was calculated as shown in EquationEq. 5(5)
(5) :
where CNaOH is the concentration of NaOH, V2 is the final titer value, M is the mass of pectin, and 31 represents the molar mass of the methoxyl group. The molecular weight (Mw) of pectin was determined by gel permeation chromatography (GPC/SEC-MALS, HELEOSII, Wyatt DAWN, USA). The mobile phase used was 0.1 M NaNO3 containing 0.02% NaN3 as a microbial inhibitor. Samples were dissolved in the mobile phase and filtered through 0.22 µm filter membranes. Samples were run for 30 min. and the data acquisition and calculations were performed by the ASTRA software, version5.3.4 (Wyatt Technology).
FTIR
FT-IR spectra of the pectin were carried out using a Fourier transform infrared spectroscopy (FTIR; Nicolet-6700; Perkin Elmer Instruments Corporation) with the KBr method in the wavenumber range of 4000–500 cm−1 and resolution of 4 cm−1.
Texture profile analysis
Double compression test
TA.XT. plus Texture Analyzer (Stable Micro Systems) was used as described by[Citation9] with some modifications. A P100, 100 mm compression platen probe was used. Twelve parallel measurements were made under the same experimental conditions with 3 fruits selected from each lot. The tests were performed in the stem-calyx axis orientation in the horizontal direction. The operating conditions were: test speed 5 mm/s, trigger force 10 g, and compression distance 10 mm. The force deformation curve obtained for each test was interpreted using the software Exponent v.6 (Stable Micro Systems). For credible data, both the minimum and maximum values of the measured fruits were removed and the remaining 10 data were averaged and recorded for the fruits on every test day.
Fruit cutting test
The Texture Analyzer was used with HDP/BSK Blade set as described by[Citation10] with some modifications. The fruit was positioned with its stem/calyx axis parallel to the platform for each test. Twelve parallel measurements were made under the same experimental conditions with 3 fruits selected from each lot. The operating conditions were: test speed 1 mm/s, trigger force 15 g, and cutting distance 50 mm. The data generated were interpreted using Exponent v.6., which was used to evaluate the peak cutting force and albedo peak cutting force. For credible data, both the minimum and maximum values of the measured fruits were removed and the remaining 10 data were averaged and recorded for the fruits on every test day.
Physicochemical properties
Weight loss (WL) was measured by weighing the fruit on the first day (W1), and at different sampling dates during storage (W2).[Citation27] WL was calculated according to Equationequation 1(1)
(1) :
The moisture content (MC) of the peels of the fruits was evaluated based on losing weight in an oven at 105°C for 24 h.[Citation28] MC was calculated according to Equationequation 2(2)
(2) :
where W1 is the initial weight and W2 is the weight after drying. Drying Rate was also expressed on the fresh weight bases per the dry weight and time, gH2O kg−1h−.[Citation1] The anthocyanin concentrations (TAC) were evaluated spectrophotometrically by the pH differential method.[Citation29] Two buffer systems were used, Potassium chloride buffer of pH 1.0 (0.025 M), and sodium acetate buffer of pH 4.5 (0.4 M). The samples were diluted with the corresponding buffer (1:4) and the absorbance was measured at 510 and 700 nm using a spectrophotometer. TAC was expressed as cyanidin-3-glucoside equivalents (mg/L).
Statistical analysis
The results were analyzed using Excel 2016 (Microsoft) and SPSS v20.0 (SPSS Inc., Chicago, IL, USA). All data are expressed as the mean ± standard error of 3–10 replicates. P < .05 was considered significant. Pearson correlation coefficient was calculated for all measured parameters to assess the nature and extent of the relationship between them.
Results and discussion
Pectin content
As shown in , during storage, the pectin content measured decreased continuously while the soluble pectin content increased. The gravitational method operates on the principle of using a precipitator to precipitate soluble pectin into a calcium pectinate. With this method, there was an increase in the pectin content from 3.5% on 1 d to 7.2% by 63 d as shown in . This increase showed that the pectins in the cell walls were being solubilized with an increase in storage time. This activity of the pectin weakened the cell walls and made them more permeable and porous, which greatly contributed to the loss of moisture (p < .01) in the fruit which in turn led to a decline in the hardness of the fruit (p < .01). Pectin can be found in the cell wall covalently and tightly held in the cell wall matrix or loosely bound to the cell wall by non-covalent and nonionic bonds, i.e., soluble pectin.[Citation30] There is evidence that indicates that there is an increase in the soluble pectin content caused by solubilization of other forms of pectin during ripening and postharvest storage, with one hypothesis suggesting that the depolymerization of chelated and/or covalently bound pectin is the cause.[Citation6,Citation18,Citation19]
Figure 1. Changes in the A (Pectin content (Rapid assay, Gravitational Method & Carbazole method)) and B (Mw and Polydispersity) in the peels of Tarocco blood Orange during storage. Data represent the mean ± SE (n = 3).
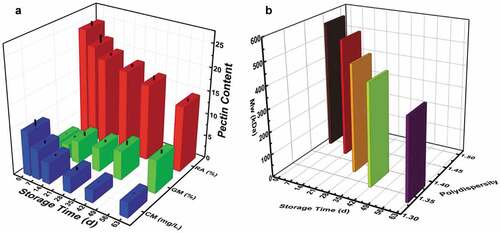
Solubilization of the pectin increased the susceptibility of the fruit to senescence and loss of textural integrity during storage. This suggests that one of the mechanisms involved in the degradation of pectin in the pericarp of Tarocco blood orange was through the process of solubilization.
From , the pectin content significantly decreased from 24.7% to 14.3% by 63 d in the rapid method, and there was also a significant loss of pectin content from 9.8 mg/L on 1 d to 3.2 mg/L on 63 d in the carbazole method. This shows that the pectin present in the peels of the fruit degraded with an increase in storage time. The rapid method does a quantitative measurement of both the soluble and insoluble amount of pectin present.[Citation22] Therefore, this data represents the total amount of pectin that was present in the peels during the study. The decline in content signified that pectin degraded with an increase in storage time. Due to the role of pectin in both the cell wall and middle lamella, the degradation of pectin led to a decline in the texture and other physicochemical properties of the fruit. The decline in pectin content positively correlated to the storage time, hardness, springiness, resilience, cohesiveness, fruit cutting force, and moisture loss, but inversely proportional to the WL and TAC.
Molecular weight
From , Mw of the pectin significantly decreased with an advance in the storage time, from 568.05 kDa on 1 d to 362.55 kDa on 63 d. This supported the fact that pectin was being depolymerized, with the pectin at various stages during storage being polydisperse. The polydispersity index ranged from 1.31 to 1.46, and this suggested that the pectins at the various stages were macromolecules with a relatively medium width distribution. This polydispersity nature can be attributed to the complex mixture of the various segments that come together to form the pectin macromolecule.[Citation31] The results for the polydispersity index decreased from 1 d to 63 d and this could be associated with the hydrolysis of the pectin molecule during storage. L. Zhang, et al.[Citation32] reported that apple pectin when degraded led to the macromolecules having a uniform and narrower distribution of Mw compared to the less degraded ones. Therefore, the decline in polydispersity observed corroborated the other results that proved that the pectin was degrading. It confirmed the increasing amount of degraded pectin at the various stages of storage. These results presented showed a decline in both Mw and polydispersity (p < .01), which confirmed and suggested that the pectin molecules were depolymerized with an increase in storage time. This also explains the reason the soluble pectin content increased during the period and the decline in pectin content.
Degree esterification
DE of pectin during postharvest storage was measured under similar conditions and the results were shown in , it decreased from 37.1% on 1 d to 27.7% by 63 d. The trend showed that pectin was de-esterified during the storage period. There was a significant correlation (p < .01) between the DE and the increase in soluble pectin during storage. De-esterification of the carboxyl groups could be the reason for the solubilization of pectin during storage. The decline in DE during plant development is not entirely due to the loss of methyl groups but also the breaking of inter-pectate ester links.[Citation33] The breaking of inter-pectate ester links could be one of the factors that led to the solubilization of the pectin molecules during storage. Monitoring these changes as a function of the storage time is essential to help understand the mechanism of fruit softening at the molecular level. This is because, during the storage of fruit, the softening that occurs is not only related to the changes in the cell wall pectin composition but also the modification of the pectin molecule such as de-esterification.[Citation12] The de-esterification process led to the loosening of the cell walls and other hydrolytic processes, which further degraded the pectin and could be a reason why the Mw (p < .05) of the pectin kept decreasing as storage time increased. From , there is a strong correlation between DE, pectin content, and molecular weight as storage time increased.
GalA content
Since pectin mostly contains GalA units, changes in these units play a vital role in its nature and function. GalA content decreased from 82.65 mg/L on 1 d to 52.15 mg/L by 63 d as shown in . This decline confirmed that the pectin molecules in the pericarp were depolymerized since some of its compositional monomers were lost. This was confirmed by the reduction in the Mw as shown in . The decline in Mw showed that the pectin molecules became smaller as storage time increased, which meant the pectin was losing some of its units during storage and confirmed the decline in GalA units during storage. In addition, the decline could be a reason why the DE and DM (p < .05) declined during storage since it is the GalA units that are esterified during the development stages of the fruit. The decline in GalA units in the peels of the fruit during storage can be associated with the decline in textural properties of the fruit during storage. This was in correlation with the report of,[Citation34] where the appearance and disappearance of 1,4-β-galactan, which is the major form of GalA in the plant cell wall, correlated with an increase and decrease in wall firmness.
Degree of methylation
The results for DM shown in , corroborate the results obtained for the decrease in pectin content and DE during storage. The DM decreased from 14.5% on 1 d to 10.0% 63 d, with the lowest DM observed on 42 d (9.7%). The decline in the DM was not extremely high, but it supplemented the degradation of pectin during storage. Previous studies show that the removal of methoxy groups from pectin makes it more susceptible to depolymerization by other enzymes present in plant cells which contributes to the softening of the cell which in turn softens the fruit.[Citation35] Therefore, although the decline was gradual, the pectin became more susceptible to further degradation. Depolymerization via eliminative decarboxylation is facilitated by an increase in the number of free carboxyl present, which occurs as a result of demethylation.[Citation36] This could have led to the increase in total depolymerization of the pectin with an increase in storage time, this was confirmed by the decline in pectin content, GalA content, and Mw as shown in & 1B. There was a significant correlation between these parameters (p < .05). Thus, although the decline was minimal during the storage period, the resultant effect was still great, leading to the increase in the degradation of the pectin.
FTIR
Further information about the changes that occurred in the pectin can also be analyzed using the FTIR spectrum. The changes that occurred in the height or area absorbance peaks during storage give insight into the modification that occurred in the pectin as shown in . All pectins showed bands centered around 1749 and 1630 cm−1 which can be used to monitor the structural changes that occurred in the pectin during storage. The bands at 1749 cm−1 correspond to the absorption of esterified carboxylic groups while those observed at 1630 cm−1 correspond to the non-esterified carboxylic groups.[Citation12] The peak area of the esterified carboxylic group and the sum of the peaks of esterified and non-esterified carboxylic groups can be considered as DE. From , it can be seen that the intensity of the transmittance of the bands at 1749 cm−1 diminished while the intensity of the band at 1630 cm−1 was enhanced from 1 d to 63 d. These reflected the decrease of DE of pectin in the cell walls of the peels during storage. It confirmed the de-esterification and degradation of pectin. The spectra for the pectin molecules had similarities in their transmission patterns to those previously studied and reported for citrus fruits.[Citation24,Citation28]
Figure 3. FTIR Spectra of pectin in the peels of Tarocco blood Orange at various stages during storage.
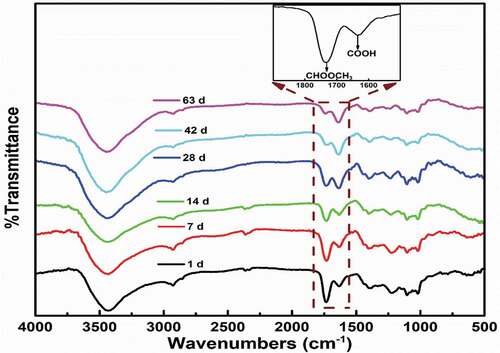
Texture profile analysis
Double compression test
The parameters such as the hardness, cohesiveness, springiness, and resilience of the fruit during post-harvest storage, were provided by the Exponent v.6 software for the test, as shown in . From , the hardness drastically decreased with an advance in the post-harvest storage under ambient conditions. The average hardness recorded for 1 d was 83.22 N and significantly decreased to 48.59 N by 63 d when the fruit had become senescent. The decrease in the hardness has a strong relationship with the storage time and the trend is in agreement with those reported by[Citation8–10,Citation19,Citation37] for Apricots, Lanelate and Valencia Oranges, Nagpur Mandarin, pomegranate, and pear. The degradation of pectin during storage strongly correlated with the decline in the hardness of the fruit. The pectin degeneration weakened the cell wall, decreased the integrity, and increased the porosity of the cell walls, which led to an increase in loss of moisture. From , it can be seen that moisture loss as a result of pectin degradation increased concomitantly as hardness decreased in the fruit during storage. This correlation showed that the decline in the hardness could be due to the elevated loss of moisture, which resulted from the degradation of pectin during storage. Additionally, from springiness, cohesiveness, and resilience decreased within the storage period and correlated with the increase in storage time and pectin degradation. As the storage time proceeded, springiness, cohesiveness, and resilience gradually decreased from 0.87 to 0.73, 0.82 to 0.70, and 0.47 to 0.30, respectively. The observed decline in cohesiveness during storage can be attributed to the solubilization of pectin in the middle lamellae of adjacent cells during storage.[Citation38] From , there is evidence of increased solubilization of pectin during the storage period, which significantly correlates with cohesiveness (p < .01). The cohesiveness of the fruit declined as a result of pectin solubilization. The cell walls are responsible for turgor pressure maintenance, indicating that a close relationship between turgor and the mechanical properties of the cell wall exists, and it plays an integral part in the texture of the fruit.[Citation7] Therefore, the ability of the fruit to resist and fight back to regain its original position and the rate of returning to the undeformed state is directly linked to the turgor pressure within the cells of the fruit. For plant cells, to maintain their rigidity, pressure from the fluids within the cell is required, therefore as the moisture is lost, it leads to plasmolysis which results in the decline of the cell turgor.[Citation39] From , there was a significant loss of moisture in the entire fruit during the storage period, which was due to the degradation of pectin which increased the porosity of the cell walls. The degradation also weakened the cell walls and middle lamella. These changes led to the decline in resilience and springiness of the fruit. These changes ascribed to the decline in the texture of the fruit have a strong relation to pectin degradation. The texture of Tarocco blood orange was strongly dependent on the storage duration and degradation of pectin, since the factors observed under this test decreased with an increase in storage time and degradation of pectin.
Fruit cutting force
The peak cutting force and the Albedo peak cutting force decreased from 98.60 N to 66.63 N and from 94.71 N to 63.83 N respectively, by 63 d as shown in . These trends were in conformation with others reported for Orange.[Citation10] Just like the double compression test, this test established the relationship between pectin degradation and the decline in textural properties of the fruit during storage. It had a strong correlation with pectin content, DE, Mw, polydispersity, and MC. The decline in the peak cutting force and albedo peak cutting force could be attributed to the degradation of pectin, which led to the increase in the loss of moisture and structural integrity of the cell walls and resulted in the peel thinning. This increased the dryness and brittleness of the peels as the loss of moisture increased and therefore reduced the resistance to cutting. By 63 d, most of the fruit tested snapped open with just a minimal force applied. From the loss in weight and height due to the decline in moisture content confirms the peel thinning, which resulted in the decline in the peak cutting force and albedo peak cutting force overtime.
Physicochemical properties
Changes such as Height, WL, MC, drying rate, and TAC of Tarocco blood orange during storage were shown in . Fruits used in this study presented an average weight ranging from 170 to 250 g and an average transverse height ranging from 68 to 90 mm. From , the average height of the fruit decreased from 73.3 mm on 1 d to 61.7 mm by 63 d. The height decreased with an increase in storage time. Moisture loss is a factor that causes stress in the cellular structure in foods and leads to a decrease in dimension.[Citation27] Therefore, the shrinkage in size could be associated with the structural collapse that occurred during storage as a result of pectin degradation, which led to an increase in moisture loss. The heights were averages of fruit heights from all lots at various stages.
By 63 d, the cumulative loss of weight significantly increased to 32.3% as shown in , which was significantly influenced by storage time and pectin degradation. Important factors that affect the WL of fruit are respiration, transpiration, and it is also accelerated by cellular breakdown.[Citation3] From , the pectin content decreased with an increase in storage time and represented the degeneration of the cell wall. The continuous decline in pectin content overtime led to the cellular breakdown which increased the rate of transpiration and respiration and led to the increase in WL of the fruit during the storage period. There is also a significant negative correlation between WL and the changes in the textural properties. Previous studies indicated that the softening of fruit was caused by the degradation and solubilization of cell wall polysaccharides which are used in the respiration process during storage, and this consequently decreased the cell wall strength, integrity, and intercellular adhesion.[Citation10,Citation19] The trend in WL is in agreement with previous studies of oranges and other fruit.[Citation8,Citation10,Citation21,Citation40]
Moisture is the major component of the fresh citrus peels and on 1 d, it was 74.5% (), which is similar to previous reports.[Citation41] The MC decreased from 74.5% to 24.2% by 63 d. From , there is a strong correlation observed between pectin degradation and loss of moisture. Pectin degradation played a major role in the increase in loss of moisture. The degradation of pectin led to an increase in the porosity of the cell wall. This weakened the cell walls and led to the reduction in the resistance to moisture movement across the cells. This resulted in the significant loss of moisture since moisture migrates preferentially through the cell walls. The increase in the loss of moisture during the storage period is an influential factor in the decline of textural properties of the Tarocco blood orange during the storage period. During the postharvest storage of citrus fruit, water loss is a big challenge. This is because it causes the loss of weight and freshness, reduces storability and disease resistance, and accelerates deterioration.[Citation42] The increase in the loss of moisture observed confirmed the degradation of pectin in the cell-matrix during storage. The loss in moisture observed was also related to the juice sacs as well.
The drying rate was also significantly reduced from 3.37 to 0.37 gH2O kg−1hr−[Citation1] by 63 d (). Drying rate slowing down during long storage periods could be attributed to the progressive water activity reduction of the external cells. When a great number of cell layers in the tissue have lost a considerable amount of moisture, the dried cell layers offer much greater resistance to water diffusion through the interface.[Citation41] The increase in loss of moisture as storage progressed as a result of pectin degradation, which accounts for the reduction of moisture in the cells, this could have led to an increase in the number of dried outer cell layers which offered resistance to further moisture loss. This also confirms the thinning of the peels of the fruit that contributed to the decline in the cutting force of the fruit discussed below.
By 63 d, TAC increased in the fruit by 8-fold, from 0.63 to 5.10 mg/L (). The highest anthocyanin content was observed on 42 d (5.70 mg/L), and similar trends were also reported by Habibi et al.[Citation40] The presence of the anthocyanin observed during this study and the trend confirms that the fruit used for this study was Tarocco blood orange. Previous reports elucidate that TAC is enhanced by different postharvest abiotic stresses induced by both physical or chemical elicitors.[Citation3] Therefore, the increase in TAC showed that the fruits were under stress which could be due to the previously mentioned physicochemical changes that occurred during storage. The decline observed on 63 d can be associated with the degradation of anthocyanins.
Relationship between pectin degradation and texture
The results gathered showed that there was a significant correlation between pectin degradation and decline in the texture of the fruits, which ultimately led to the decline in fruit quality and senescing. The hardness of the orange depends on its turgidity and water content, therefore as the water content and turgidity decrease, the hardness of the fruit decreases.[Citation37] This relationship is observed in . Pectin degraded as storage time increased as shown in , the degradation led to a loss in mechanical properties, an increase in permeability, and a decline of adhesion in the cell walls and middle lamella. This led to an increase in loss of moisture which was confirmed by the decrease in weight of the fruit and resulted in the decline of texture. This accounts for the loss of textural properties during storage. There was a significant correlation between pectin and texture as shown in , the pectin content decreased concomitantly with the decrease in the textural properties overtime.
Table 1. Correlation coefficients between measured factors of Tarocco blood Orange stored for up to 63 days. HD(Hardness), SP(Springiness), CO(Cohesiveness), RE(Resilience), PCF (Peak Cutting Force), APCF (Albedo Peak Cutting Force), RA (Rapid Analysis), GM (Gravitational Method), CM (Carbazole Method), Poly (Polydispersity)
Conclusion
In this study, we reported for the first time a combined analysis of both the degradation of pectin in the pericarp and fruit texture during postharvest storage in Tarocco blood orange at the same time. The amount of solubilized pectin increased by 51.4% during the study period, which indicated pectin degradation. The pectin content decreased by at least 42% as examined by both the rapid and carbazole methods. The degradation of pectin also occurred as a result of the increase in de-esterification and depolymerization, which was confirmed by the decline in DE, DM, GalA content, Mw, and FTIR spectra. The decline in the texture of the fruits during prolonged storage was promoted, due to the degradation of pectin, which weakened the cell walls and middle lamella. These changes also led to changes in the physicochemical properties such as the elevated loss of moisture from 74.5% to 24.2%, which in turn led to 32.3% weight loss. Based on the physicochemical properties, pectin changes, and texture attributes, it can be concluded that there was a strong correlation between these changes, which helps to prove and suggest that pectin degradation is the pivotal cause of loss of texture and mechanical strength of Tarocco blood orange during prolonged storage. Thus, the findings could also be used to further study the influence of postharvest storage regimes and how to optimize them.
Highlights
Pectin degraded by de-esterification, depolymerization, and solubilization
Elevated porosity, weakening and reduction in cell wall adhesion and middle lamella
An increased decline in the physicochemical properties due to pectin degradation
Texture declined concomitantly with pectin degradation
Acknowledgments
The authors would like to thank the technology and financial support from Engineering Research Center of Biomass Materials, Ministry of Education, Southwest University of Science and Technology, and the project of Agriculture and Rural Committee of Changshou District (Chongqing) “Demonstration of application technology of Tarocco blood orange green preservation” (19zh0333). This work was also sponsored by the Innovation and Entrepreneurship Training Program for College Students of Southwest University of Science and Technology (S201910619089).
Disclosure statement
No potential conflict of interest was reported by the author(s).
Additional information
Funding
References
- Prakash Maran, J.; Sivakumar, V.; Thirugnanasambandham, K.; Sridhar, R. Optimization of Microwave Assisted Extraction of Pectin from Orange Peel [J]. Carbohydr. Polym. 2013, 97(2), 703–709. DOI: 10.1016/j.carbpol.2013.05.052.
- Fallico, B.; Ballistreri, G.; Arena, E.; Brighina, S.; Rapisarda, P. Bioactive Compounds in Blood Oranges (Citrus Sinensis (L.) Osbeck): Level and Intake [J]. Food Chem. 2017, 215, 67–75. DOI: 10.1016/j.foodchem.2016.07.142.
- Habibi, F.; Ramezanian, A. Vacuum Infiltration of Putrescine Enhances Bioactive Compounds and Maintains Quality of Blood Orange during Cold Storage [J]. Food Chem. 2017, 227(1–8), 1–8. DOI: 10.1016/j.foodchem.2017.01.057.
- Pannitteri, C.; Continella, A.; Lo Cicero, L.; Legua, P.; D’Aquino, S.; Palma, A.; La Malfa, S. Effects of Postharvest Storage Conditions on ‘Tarocco’ Orange Fruit Quality [J]. Acta Hortic. 2018, 1194, 873–878. DOI: 10.17660/ActaHortic.2018.1194.123.
- Menesatti, P.; Pallottino, F.; Lanza, G.; Paglia, G. Prediction of Blood Orange MT Firmness by Multivariate Modelling of Low Alterative Penetrometric Data Set: A Preliminary Study [J]. Postharvest. Biol. Technol. 2009, 51(3), 434–436. DOI: 10.1016/j.postharvbio.2008.08.007.
- Posé, S.; Paniagua, C.; Matas A, J.; Gunning, A. P.; Morris, V. J.; Quesada, M. A.; Mercado, J.A Nanostructural View of the Cell Wall Disassembly Process during Fruit Ripening and Postharvest Storage by Atomic Force Microscopy [J]. Trends Food Sci. Technol. 2019, 87(47–58), 47–58. DOI: 10.1016/j.tifs.2018.02.011.
- Sañudo-Barajas J, A.; Lipan, L.; Cano-Lamadrid, M.; de la Rocha, R. V.; Noguera-Artiaga, L; Sanchez-Rodriguez, L.; Carbonell-Barrachina, A. A.; Hernandez, F. Texture [M]//elhadi M Y, Armando C-L.Postharvest Physiology and Biochemistry of Fruits and Vegetables. Duxford, United Kingdom. Elsevier Sci. 2019, 293–314.
- Arendse, E.; Fawole, O. A.; Opara, U. L. Influence of Storage Temperature and Duration on Postharvest Physico-chemical and Mechanical Properties of Pomegranate Fruit and Arils [J]. CyTA - J. Food. 2014, 12(4), 389–398. DOI: 10.1080/19476337.2014.900114.
- Guiné R P, F. Variation of Textural Attributes of S. Bartolomeu Pears at Maturation, Storage, and Drying [J]. Int. J. Food Prop. 2012, 16(1), 180–192. DOI: 10.1080/10942912.2010.535191.
- Singh, K. K.; Reddy, B. S. Post-harvest Physico-mechanical Properties of Orange Peel and Fruit [J]. J. Food Eng. 2006, 73(2), 112–120. DOI: 10.1016/j.jfoodeng.2005.01.010.
- Jouki, M.; Khazaei, N. J. J. O. F. P. Technology.The Effect of Modified Atmosphere Packaging and Calcium Chloride Dripping on the Quality and Shelf Life of Kurdistan Strawberries [J]. J Food Processing Technol. 2012, 3 (10).
- Chatjigakis A, K.; Pappas, C.; Proxenia, N.; Kalantzi, O.; Rodis, P.; Polissiou, M. FT-IR Spectroscopic Determination of the Degree of Esterification of Cell Wall Pectins from Stored Peaches and Correlation to Textural Changes [J]. Carbohydr. Polym. 1998, 37(4), 395–408. DOI: 10.1016/S0144-8617(98)00057-5.
- Zhang, C.; Xiong, Z.; Yang, H.; Wu, W. Changes in Pericarp Morphology, Physiology and Cell Wall Composition Account for Flesh Firmness during the Ripening of Blackberry (Rubus Spp.) Fruit [J]. Sci. Hortic. 2019, 250, 59–68. DOI: 10.1016/j.scienta.2019.02.015.
- Kullaj, E. New Insights on Postharvest Ecophysiology of Fresh Horticultural Crops [M]. Eco-Friendly Technol for Postharvest Produce Qual. 2016, 1–38.
- Grassino A, N.; Barba F, J.; Brncic, M.; Lorenzo, J. M.; Lucini, L.; Brnčić, S. R. Analytical Tools Used for the Identification and Quantification of Pectin Extracted from Plant Food Matrices, Wastes and By-products: A Review [J]. Food Chem. 2018, 266, 47–55. DOI: 10.1016/j.foodchem.2018.05.105.
- Defilippi B, G.; Ejsmentewicz, T.; Covarrubias M, P.; Gudenschwager, O.; Campos-Vargas, R. Changes in Cell Wall Pectins and Their Relation to Postharvest Mesocarp Softening of “Hass” Avocados (Persea Americana Mill.) [J]. Plant Physiol. Biochem. 2018, 128, 142–151. DOI: 10.1016/j.plaphy.2018.05.018.
- Airianah, O. B.; Vreeburg, R. A.; Fry, S. C. Pectic Polysaccharides are Attacked by Hydroxyl Radicals in Ripening Fruit: Evidence from a Fluorescent Fingerprinting Method [J]. Ann. Bot. 2016, 117(3), 441–455. DOI: 10.1093/aob/mcv192.
- Cardenas-Perez, S.; Chanona-Perez J, J.; Guemes-Vera, N.; Cybulska, J.; Szymanska-Chargot, M.; Chylinska, M.; Kozioł, A.; Gawkowska, D.; Pieczywek, P. M.; Zdunek, A.; et al. Structural, Mechanical and Enzymatic Study of Pectin and Cellulose during Mango Ripening [J]. Carbohydr. Polym. 2018, 196(313–321), 313–321. DOI: 10.1016/j.carbpol.2018.05.044.
- Deng L, Z.; Pan, Z.; Zhang, Q.; Liu, Z.-L.; Zhang, Y.; Meng, J.-S.; Gao, Z.-J.; Xiao, H.-W.Effects of Ripening Stage on Physicochemical Properties, Drying Kinetics, Pectin Polysaccharides Contents and Nanostructure of Apricots [J]. Carbohydr. Polym. 2019, 222(114980), 114980. DOI: 10.1016/j.carbpol.2019.114980.
- Billy, L.; Mehinagic, E.; Royer, G.; Renard, C. M. G. C.; Arvisenet, G.; Prost, C.; Jourjon, F. Relationship between Texture and Pectin Composition of Two Apple Cultivars during Storage [J]. Postharvest. Biol. Technol. 2008, 47(3), 315–324. DOI: 10.1016/j.postharvbio.2007.07.011.
- Liu, B.; Wang, K.; Shu, X.; Liang, J.; Fan, X.; Sun, L. Changes in Fruit Firmness, Quality Traits and Cell Wall Constituents of Two Highbush Blueberries (Vaccinium Corymbosum L.) During Postharvest Cold Storage [J]. Sci. Hortic. 2019, 246(557–562), 557–562. DOI: 10.1016/j.scienta.2018.11.042.
- Shelukhina, N.; Fedichkina, L. A Rapid Method for Quantitative Determination of Pectic Substances [J]. Acta Bot. Neerl. 1994, 43 (2), 205–207. DOI: 10.1111/j.1438-8677.1994.tb00745.x.
- Yeoh, S.; Shi, J.; Langrish, T. G. Comparisons between Different Techniques for Water-based Extraction of Pectin from Orange Peels [J]. Desalination. 2008, 218(1–3), 229–237. DOI: 10.1016/j.desal.2007.02.018.
- Hosseini, S. S.; Khodaiyan, F.; Yarmand, M. S. Aqueous Extraction of Pectin from Sour Orange Peel and Its Preliminary Physicochemical Properties [J]. Int. J. Biol. Macromol. 2016, 82 (920–926), 920–926. DOI:10.1016/j.ijbiomac.2015.11.007.
- Rodsamran, P.; Sothornvit, R. Preparation and Characterization of Pectin Fraction from Pineapple Peel as a Natural Plasticizer and Material for Biopolymer Film [J]. Food Bioprod. Process. 2019, 118(198–206), 198–206. DOI: 10.1016/j.fbp.2019.09.010.
- Ogutu, F. O.; Mu, T. H. Ultrasonic Degradation of Sweet Potato Pectin and Its Antioxidant Activity [J]. Ultrason. Sonochem. 2017, 38(726–734), 726–734. DOI: 10.1016/j.ultsonch.2016.08.014.
- Omolola, A. O.; Jideani, A. I.; Kapila, P. F. Quality Properties of Fruits as Affected by Drying Operation [J]. Crit. Rev. Food Sci. Nutr. 2017, 57(1), 95–108. DOI: 10.1080/10408398.2013.859563.
- Hosseini S, S.; Khodaiyan, F.; Kazemi, M.; Najari, Z. Optimization and Characterization of Pectin Extracted from Sour Orange Peel by Ultrasound Assisted Method [J]. Int. J. Biol. Macromol. 2019, 125(621–629), 621–629. DOI: 10.1016/j.ijbiomac.2018.12.096.
- Rapisarda, P.; Fanella, F.; Maccarone, E. Reliability of Analytical Methods for Determining Anthocyanins in Blood Orange Juices [J]. J. Agric. Food Chem. 2000, 48(6), 2249–2252. DOI: 10.1021/jf991157h.
- Deng L, Z.; Mujumdar A, S.; Yang X, H.; Wang, J.; Zhang, Q.; Zheng, Z.-A.; Gao, Z.-J.; Xiao, H.-W. High Humidity Hot Air Impingement Blanching (HHAIB) Enhances Drying Rate and Softens Texture of Apricot via Cell Wall Pectin Polysaccharides Degradation and Ultrastructure Modification [J]. Food Chem. 2018, 261(292–300), 292–300. DOI: 10.1016/j.foodchem.2018.04.062.
- Alba, K.; Kontogiorgos, V. Pectin at the Oil-water Interface: Relationship of Molecular Composition and Structure to Functionality [J]. Food Hydrocolloids. 2017, 68(211–218), 211–218. DOI: 10.1016/j.foodhyd.2016.07.026.
- Zhang, L.; Ye, X.; Ding, T.; Sun, X.; Xu, Y.; Liu, D. Ultrasound Effects on the Degradation Kinetics, Structure and Rheological Properties of Apple Pectin [J]. Ultrason. Sonochem. 2013, 20(1), 222–231. DOI: 10.1016/j.ultsonch.2012.07.021.
- Brummell, D. A.; Harptster, M. H. Cell Wall Metabolism in Fruit Softening and Quality and Its Manipulation Intransgenic Plants. Plant Mol Biol. 2001, 47 (1–2), 311–339. DOI:10.1023/A:1010656104304.
- Brummell, D. A. Cell Wall Disassembly in Ripening Fruit [J]. Funct. Plant Biol. 2006, 33(2), 103–119. DOI: 10.1071/fp05234.
- Jolie R, P.; Duvetter, T.; Van Loey, A. M.; Hendrickx, M. E. Pectin Methylesterase and Its Proteinaceous Inhibitor: A Review [J]. Carbohydr. Res. 2010, 345(18), 2583–2595. DOI: 10.1016/j.carres.2010.10.002.
- Einhorn-Stoll, U.; Kastner, H.; Fatouros, A.; Krähmer, A.; Kroh, L. W.; Drusch, S. Thermal Degradation of Citrus Pectin in Low-moisture Environment – Investigation of Backbone Depolymerisation [J]. Food Hydrocolloids. 2020, 107, 105937. DOI: 10.1016/j.foodhyd.2020.105937.
- Olmo, M.; Nadas, A.; García J J J O F, S. Nondestructive Methods to Evaluate Maturity Level of Oranges [J]. J. Food Sci. 2000, 65(2), 365–369. DOI: 10.1111/j.1365-2621.2000.tb16008.x.
- Chauhan O, P.; Raju P, S.; Dasgupta D, K.; Bawa, A. S. Instrumental Textural Changes in Banana (Var. Pachbale) during Ripening under Active and Passive Modified Atmosphere [J]. Int. J. Food Prop. 2006, 9(2), 237–253. DOI: 10.1080/10942910600596282.
- Fricke, W. Turgor Pressure [M]. In: eLS; Chichester, England; John Wiley & Sons, Ltd, 2017; pp 1–6.
- Habibi, F.; Ramezanian, A.; Guillen, F.; Serrano, M.; Valero, D. Blood Oranges Maintain Bioactive Compounds and Nutritional Quality by Postharvest Treatments with Gamma-aminobutyric Acid, Methyl Jasmonate or Methyl Salicylate during Cold Storage [J]. Food Chem. 2020, 306(125634), 125634. DOI: 10.1016/j.foodchem.2019.125634.
- Ghanem, N.; Mihoubi, D.; Kechaou, N.; Mihoubi, N. B. Microwave Dehydration of Three Citrus Peel Cultivars: Effect on Water and Oil Retention Capacities, Color, Shrinkage and Total Phenols Content [J]. Ind. Crops Prod. 2012, 40(167–177), 167–177. DOI: 10.1016/j.indcrop.2012.03.009.
- Sun, C.; Van Beers, R.; Aernouts, B.; Saeys, W. Bulk Optical Properties of Citrus Tissues and the Relationship with Quality Properties [J]. Postharvest. Biol. Technol. 2020, 163(1–11), 111127. DOI: 10.1016/j.postharvbio.2020.111127.