ABSTRACT
Anthocyanins are abundant phytochemicals in nature that draw the public interest not only in their health effects, such as antioxidant, anti-inflammatory and anti-carcinogenic properties. It also showed their function in providing foodstuffs with appealing and distinctive color. In this study, anthocyanins from hibiscus (Hibiscus sabdariffa L.) calyces were microencapsulated by spray drying and freeze drying techniques using a wide variety of carriers including maltodextrin (MD), gum Arabic (GA) and their binary blends with inulin (INU) and konjac glucomannan (KON). The results showed that freeze-dried hibiscus powder using KON as carrier had the highest phenolic, anthocyanin and antioxidant activity, followed by spray-dried and freeze-dried MD/KON samples, which indicated the role of KON in the effective retention of antioxidants during the drying process. In addition, the ferric and cupric ion reduction activity (FRAP and CUPRAC) of the spray-dried samples was significantly higher than those of the freeze-dried powder. However, in terms of encapsulation efficiency (EE) of anthocyanins, KON was shown to be ineffective in entrapping these compounds in microcapsules with the lowest EE of freeze-dried KON and spray-dried MD/KON of 43.6% and 55.4%, respectively. By contrast, MD/GA was the most effective carrier, retaining anthocyanins inside the carrier matrix and limiting their loss to the surface of the microcapsules in both spray-drying and freeze-drying methods (EE of 91.8% and 95.7%, respectively). In addition, the moisture content of spray-dried powder samples was significantly higher than that of lyophilized powders, and the solubility of all samples was above 94.1%.
Introduction
In recent years, researchers have focused on the nutritional and health benefits of typical natural antioxidants such as anthocyanins.[Citation1] Anthocyanins are found in various fruits and vegetables with a wide variety of colors ranging from bright red to purple or dark green with high antioxidant capacity and present in glycoside form of anthocyanidin (aglycone).[Citation2] While anthocyanins have the advantage of being a possible substitute for synthetic colors, their unique molecular structure makes them unstable and prone to decomposition. The stability of anthocyanins is affected by pH, temperature, the presence of light, metal ions, enzymes, oxygen, ascorbic acid, sugars, proteins, and sulfur dioxide.[Citation3,Citation4]
Roselle (Hibiscus sabdariffa L.) is a kind of herbaceous, bushy plant that grows mainly in tropical and subtropical regions.[Citation5] Roselle has been widely used in the food production, particularly in products such as beverages, jams, jellies, wines as a food colorant, and flour for biscuit manufacture.[Citation6] Roselle calyces are a rich source of antioxidants with calyces containing anthocyanins as high as 2.5 g/100 g on the dry weight along with organic acids, minerals, amino acids, carotene, α-tocopherol and linoleic acid.[Citation7] Regarding the anthocyanin composition, the calyces contain delphinidin-3-glucoside, cyanidin-3-glucoside, delphinidin-3-sambubioside, and cyanidin-3-sambubioside being these compounds responsible for the red color of hibiscus.[Citation8,Citation9] Researchers have demonstrated the health benefits of roselle, such as anti-bacterial, anti-inflammatory, and anti-carcinogenic, anti-hypertensive effects. These effects are attributed to the antioxidant properties of bioactive compounds, such as phenolic acids, anthocyanins, and carotenoids in hibiscus.[Citation10–12]
To develop a roselle-based food colorant, it is necessary to maintain the stability of anthocyanins during processing and storage. Microencapsulation has become a viable method for protecting these bioactive compounds that present sensitivity to environmental factors such as temperature, light, and oxygen.[Citation13,Citation14] The microencapsulation has been defined as a process of trapping active ingredients in other material matrices to create particles ranging from a few micrometers to several nanometers. The particles or droplets are surrounded by a wall, or embedded in a homogeneous or heterogeneous matrix to form small capsules.[Citation15–17] Among the available microencapsulation methods, spray drying is one of the commonly used to encapsulate anthocyanins, which can help to protect their biological activity. Spray drying is especially useful for the encapsulation of heat-sensitive food ingredients, as the drying process is very rapid.[Citation18] According to Fang and Bhandari (2012), spray drying is the most suitable method to encapsulate polyphenols.[Citation19] Typically, spray drying is operated by atomization of the feed solution in a drying chamber where air is circulated inside, contacting atomized particles and evaporating the solvent to result in powdered products which are collected in the cyclone.[Citation20,Citation21] Meanwhile, freeze-drying is a process that involves the sublimation of water under low pressure and is thus suitable for heat-labile substances. Due to its extended operation duration (24–48 hours), complex equipment, and significant energy consumption, this process is considered an expensive drying method.[Citation22] In these two drying methods, diverse wall materials, including carbohydrates or proteins, can cover the solid, liquid, or gaseous substances in microscopic capsules which gradually diffuse through the capsule wall, and thus provide their controlled release in the desired conditions.[Citation23]
Maltodextrin is a hydrolyzed starch produced by partially hydrolyzing starch with acid or enzyme, commonly used as a raw material in the process of encapsulation of food ingredients[Citation17–24] with high solubility and low viscosity at high solids content.[Citation25–27] However, the biggest disadvantages of this wall material are its low emulsibility and retention of volatile compounds. Therefore, it is often used in combination with other wall materials.[Citation28,Citation29]
Gum Arabic obtained from the natural exudate of the stems and branches of acacia plant is a polysaccharide consisting of galactose, arabinose, rhamnose, glucuronic acid, and 4-O-methyl glucuronic acid.[Citation30] Gum Arabic is an effective and widely used wall material because of its stable emulsion formation and high retention of volatile compounds.[Citation31] Gum Arabic has low viscosity and high solubility (up to 50%) in both cold and hot water because it contains a heteropolysaccharide with a highly branched structure and a protein content of approximately 2%.[Citation32]
Konjac is a neutral and water-soluble polysaccharide found in the roots and tubers of the Amorphophallus konjac plant consisting of β-(1,4) linked D-glucosyl and D-mannosyl residues (in the molar ratio of 1.5: 1 to 1.6:1) as the main chain with the β-(1,3) branch, and the degree of branching is about 8%.[Citation33,Citation34] Konjac also possesses the high biodegradability and excellent film-forming ability, and konjac films have good water barrier properties compared to other polysaccharide films,[Citation35] facilitating wide application in food, chemical, biology, and health.[Citation36,Citation37]
Inulin is an important fructan found in plants and vegetables, such as garlic, leeks, bananas, and Jerusalem artichoke. Inulin belongs to the fructan polysaccharide group, which usually contains about 2–60 fructose units in the linear chain with β-(2,1) glycosidic bonds and is usually associated with one end glucose unit. There is a growing concern for inulin because of the role of fructans in both food and pharmaceuticals.[Citation38] Inulin is accredited by the US Food and Drug Administration (FDA) as a safe polysaccharide (GRAS) with high biodegradability, biocompatibility, water-solubility, renewable, and nontoxic.[Citation39,Citation40]
To the best of our knowledge, there are plenty of studies on the microencapsulation of plant-derived antioxidants such as roselle,[Citation41–43] coffee grounds,[Citation44] black glutinous rice bran anthocyanins,[Citation45] acerola,[Citation46] blackberry,[Citation47] red cabbage anthocyanins,[Citation48] and chokeberry[Citation49] using whey proteins, maltodextrin, heteropolysaccharides (gum Arabic, pectin, carboxymethyl cellulose), and their blends. However, studies on the support of homopolysaccharides such as inulin and konjac glucomannan in the microencapsulation of roselle anthocyanin are quite limited. Therefore, the objective of this study was to compare microencapsulation efficiency of anthocyanin and antioxidant activity retention of hibiscus extracts by spray-drying and freeze-drying techniques using a variety of carriers and their binary blends including maltodextrin (MD), gum Arabic (GA), inulin (INU), konjac glucomannan (KON). In addition, Pearson correlation was performed to evaluate the relationship between phenolics, anthocyanins and antioxidant activities of spray-dried and freeze-dried hibiscus powder samples.
Materials and Methods
Materials and Chemicals
Dried hibiscus calyces were purchased from Viet Hibiscus Company (Ho Chi Minh City, Vietnam). The plants were grown in Bien Hoa City (Dong Nai Province, Vietnam). After harvesting in 2019, fresh flowers were air-dehydrated by convection using hot air at 60°C for 12 h to reach the moisture content of 10.54%. Dried products were stored in polyethylene bags in a cool, dry place, away from direct sunlight.
Gallic acid, 2,2-diphenyl-1-picrylhydrazyl (DPPH), 2,4,6-tripyridyl-s-triazine (TPTZ), 2,2′-azino-bis(3-ethylbenzothiazoline-6-sulfonic acid) (ABTS), 2,9-dimethyl-1,10-phenanthroline (neocuproine), and 6-hydroxy-2,5,7,8 tetramethylchroman-2-carboxylic acid (Trolox) were purchased from Sigma-Aldrich (Singapore). Folin-Ciocalteu reagent (2 N) was prepared basically from solid sodium tungstate, sodium molybdate, and lithium sulfate.
Maltodextrin with DE 8–10 and solubility >98% (Baolingbao Biology Co. Ltd., Shandong, China), gum Arabic (Sigma Aldrich, Singapore), inulin with molecular weight of 5000 Da (Merck‐Millipore, Darmstadt, Germany), konjac glucomannan (Hubei Konson Konjac Gum Co. Ltd., Hubei, China) were purchased from the local suppliers. Sodium acetate trihydrate, acetic acid, sodium carbonate, methanol, ethanol, potassium persulfate, phosphoric acid, hydrochloric acid, potassium chloride, ferric chloride hexahydrate, cuprous chloride, ammonium acetate and other chemicals of analytical grade were obtained from Xilong Scientific Co., Ltd. (Guangdong, China).
Preparation of roselle anthocyanin extracts
250 g of dried roselle calyces were extracted at the temperature of 50°C for 30 minutes in 400 mL of 70% (v/v) ethanol acidified to pH 2.0 by 2 N hydrochloric acid using the HI 2211–02 pH meter (Hanna Instruments, Mauritius, Romania). After extraction, the extract was obtained by filtering through Whatman No. 2 filter paper. The filtrate was then concentrated under vacuum conditions in the Hei-VAP Value rotary vacuum evaporator (Heidolph Instruments, Schwabach, Germany) at 55°C to remove the ethanol solvent. To determine the amount of carrier required in the microencapsulation process, the concentrate was preliminarily analyzed for total monomeric anthocyanin content. The results showed that the concentrated roselle extract contained anthocyanin with the content of 0.87 g of cyanidin-3-glucoside /L.
Spray drying of anthocyanin extracts
The concentrated anthocyanin extract was mixed with different carriers () according to the mass ratio of 1:100 (w/w) between anthocyanin and carriers. Then, spray drying was conducted in the SD-06AG spray drier (Lab Plant Ltd., Keison, Chelmsford, UK). The feed flow rate was set at 500 mL/h, and the inlet temperature was fixed at 150°C, with corresponding outlet temperatures of 91°C. After spray drying, the powdered samples were refrigerated at 4°C in polyethylene bags for further analysis.
Table 1. Composition of different carriers used in the spray drying and freeze drying of roselle anthocyanin extracts
Freeze drying of anthocyanin extracts
The concentrated anthocyanin extract was mixed with different carriers () according to the mass ratio of 1:100 (w/w) between anthocyanin and carriers. Then, freeze drying was conducted in the FDU-2100 freeze dryer (Eyela Tokyo Rikakikai Co., Tokyo, Japan). After freeze drying, the lyophilized samples were refrigerated at 4°C in polyethylene bags for further analysis.
Total anthocyanin content (TAC)
The total anthocyanin content (TAC) of spray-dried powder was measured according to the method described in the literature with slight modification.[Citation50] Briefly, 100 mg of the sample was weighed and mixed with 1 mL of distilled water. The sample was then crushed with a set of pestle and mortar to destroy the microstructure. 10 mL of 96% ethanol was used to extract for 5 minutes. The filtrate was collected and analyzed for TAC using total monomeric anthocyanin assay.
Total monomeric anthocyanin content was determined using differential pH method.[Citation51] Two buffer solutions were used to dilute the sample solution: 0.2 M KCl buffer (pH 1.0) and 0.1 M acetate buffer (pH 4.5). The absorbances of the two samples were determined using the UV-1800 spectrophotometer (Shimadzu Inc., Kyoto, Japan) at two different wavelengths of 520 and 700 nm. The total monomeric anthocyanin content was calculated using the formula: [(A520 – A700) pH1.0 – (A520 – A700) pH4.5] × df × 1000 × 26900/449.2; where, A is the absorbance of the sample, df is the dilution factor, 26900 is the extinction coefficient, 449.2 is molecular mass of cyanidin-3 glucoside, and 1000 is the g to mg conversion coefficient. The results were expressed as mg of cyanidin-3-glucoside/g of sample on the dry weight (mg C3G/g DW).
Surface anthocyanin content (SAC)
The surface anthocyanin content (SAC) of spray-dried powder was measured based on the method described in the literature with slight modification.[Citation50] Briefly, 100 mg of the sample was weighed and mixed with 10 mL of 96% ethanol. After vortexed for 20s in the VELP ZX4 advanced IR vortex mixer (VELP Scientifica, Milan, Italy) and centrifuged in the PLC-05 centrifuge (Gemmy Industrial Corp., Taipei, Taiwan) at 3000 rpm for 3 minutes, the supernatant was collected and filtered through a membrane filter with pore size of 0.45 μm. The filtrate was collected and analyzed for SAC using total monomeric anthocyanin assay. In addition, encapsulation efficiency (%) was calculated as (TAC – SAC) × 100/TAC.
Total phenolic content (TPC)
The phenolic content (TPC) was performed according to the Folin-Ciocalteu method described in ISO 14502–1:2005.[Citation52] Briefly, 100 mg of the sample was weighed and mixed with 10 mL of distilled water. To determine the phenolic content, 0.6 mL of the diluted sample was added to 1.5 mL of Folin-Ciocalteu reagent (10-fold dilution) and incubated in the dark for 5 minutes. After adding 1.2 mL of 7.5% (w/v) Na2CO3, the reaction mixture was incubated for 60 minutes in the dark. The absorbance was then measured at 765 nm using the UV-Vis spectrophotometer and the distilled water was used as blank. Total phenolic content was calculated using the gallic acid calibration curve and expressed as mg gallic acid equivalent/g of sample on the dry weight (mg GAE/g DW).
DPPH free radical scavenging activity
DPPH free radical reduction assay was performed according to the method described in the literature with some modifications.[Citation53] Briefly, 100 mg of the sample was weighed and mixed with 10 mL of distilled water. To prepare the DPPH reagent, 0.024 g of DPPH was dissolved in 100 mL of methanol and stored at 4°C for 24 hours. The working reagent was then prepared by mixing the stock DPPH solution with methanol so that the absorbance of the mixture reaches 1.10 ± 0.02 at 515 nm with the UV-Vis spectrophotometer. To determine the DPPH free radical reduction activity, 2.85 mL of the working DPPH reagent was added to 0.15 mL of the diluted sample. The reaction mixture was incubated for 30 minutes in the dark and the absorbance was measured at 515 nm over methanol blank. The percentage of DPPH free radical inhibition was calculated using the following formula: % Inhibition = (1 – Absorbance of sample/Absorbance of control) × 100. DPPH antioxidant activity was calculated against the Trolox concentration – % inhibition curve and expressed as mg Trolox equivalent/g of sample on the dry weight (mg TE/g DW).
ABTS cation radical reduction assay
ABTS free radical reduction assay was performed according to the method described in the literature with some modifications.[Citation54] Briefly, 100 mg of the sample was weighed and mixed with 10 mL of distilled water. To prepare the ABTS reagent, 7.4 mM ABTS solution was mixed with 2.6 mM potassium persulfate solution at a ratio of 1:1 (v/v) and then refrigerated at 4°C for 24 hours. The working reagent was prepared by mixing the stock ABTS solution with methanol so that the absorbance of the mixture reaches 1.10 ± 0.02 at a wavelength of 734 nm using the UV-Vis spectrophotometer. To determine the ABTS free radical scavenging activity, 2.85 mL of the stock ABTS reagent was added to 0.15 mL of the diluted sample. The reaction mixture was incubated for 30 minutes in the dark and the absorbance was measured at 734 nm using the UV-Vis spectrophotometer over methanol blank. The percentage of ABTS free radical inhibition was calculated using the formula: % Inhibition = (1 – Absorbance of sample/Absorbance of control) × 100. The ABTS cation radical reduction ability was calculated against the Trolox concentration – % inhibition curve and expressed as mg Trolox equivalent /g of sample on the dry weight (mg TE/g DW).
Ferric reducing antioxidant power (FRAP)
The ferric reducing antioxidant power (FRAP) assay was performed according to the method described in the literature with some modifications.[Citation55] Briefly, 100 mg of the sample was weighed and mixed with 10 mL of distilled water. To prepare the reagent, the acetate buffer (0.3 M, pH 3.6), TPTZ (10 mM in 40 mM HCl) and FeCl3.6H2O (20 mM) solution were mixed at a 10:1:1 (v/v) ratio. To determine the ferric reducing antioxidant power (FRAP), 2.85 mL of FRAP reagent was added to 0.15 mL of diluted sample. The reaction mixture was incubated for 30 minutes in the dark and the absorbance was measured at 593 nm with the UV-Vis spectrophotometer over distilled water as blank. The ferric reducing antioxidant power (FRAP) was calculated based on the Trolox calibration curve and expressed as mg Trolox equivalent/g of sample on the dry weight (mg TE/g DW).
Cupric reducing antioxidant capacity (CUPRAC)
Cupric reducing antioxidant capacity (CUPRAC) assay was performed according to the method described in the literature with some modifications.[Citation56] Briefly, 100 mg of the sample was weighed and mixed with 10 mL of distilled water. Sample solution (0.5 mL) was added to premixed reaction mixture containing CuCl2 (1 mL, 10 mM), neocuproine (1 mL, 7.5 mM) and ammonium acetate buffer (1 mL, 1 M, pH 7.0) adjusted to pH 7.0. The reaction mixture was incubated for 30 minutes in the dark and the absorbance was measured at 450 nm with the UV-Vis spectrophotometer over distilled water as blank. The cupric reducing antioxidant capacity (CUPRAC) was calculated based on the Trolox calibration curve and expressed as mg Trolox equivalent/g of sample on the dry weight (mg TE/g DW).
Moisture content
The moisture content was determined based on the method described in literature.[Citation57] The beads (2 g) were weighed and spread evenly on the petri dishes. The sample was then dried at 105°C until reaching constant mass in the LO-FS100 forced convection oven (LK Lab, Namyangju, Korea) to constant mass.
Solubility
The solubility of powder was determined based on the method described in literature with slight changes.[Citation25] Briefly, 0.1 g sample was mixed with 10 mL of distilled water, and the mixture was stirred in the magnetic stirrer for 5 minutes. Then, the solution was centrifuged at 3000 rpm for 10 minutes. The supernatant was transferred to a petri dish and dried at 105°C until it reached constant mass. Solubility was calculated as the difference in solid weight between the reconstituted solution and the original solid sample.
Statistical analysis
Experimental data were analyzed using SPSS 15 software (SPSS Inc., Chicago, U.S.A) using basic statistical techniques. Pearson correlation and analysis of variance were used to determine the differences between samples, and Tukey’s Multiple Range test was applied to determine significant differences between mean values at the significance level of 5%. All experiments were conducted in triplicate.
Results and Discussion
Effect of different carriers on TPC of spray-dried and freeze-dried roselle powder
In this study, maltodextrin was combined with several different carriers (gum Arabic, inulin, and konjac) to improve the microencapsulation of anthocyanins from hibiscus with a mass ratio of 1:1 in the binary blend and a mass ratio of 1:100 between the anthocyanin and carriers. Apart from the established anthocyanins, hibiscus calyces contain significant amounts of a wide range of other phenolic compounds, such as hibiscus acid derivatives, hydroxybenzoic acids, caffeoylquinic acids, and flavonols.[Citation58] As a consequence, it is critical to assess the effect of processing on the antioxidant content and activity of hibiscus powder to ensure that the health advantages associated with spray-dried hibiscus powder are maintained. The effects of different carriers on the TPC of spray-dried and freeze-dried roselle powder are shown in . The results exhibited that the TPC of the freeze-dried hibiscus powder sample using 100% konjac (64.95 mg GAE/g DW) was superior to those of other carriers. In addition, it should be emphasized that when konjac was solely used, there was no spray-dried hibiscus powder formed. However, combining maltodextrin and konjac at a ratio of 1:1 improved TPC content in both spray-dried and freeze-dried products with values of 40.15 and 40.86 (mg GAE/g DW), respectively. The TPC of the other samples were shown to be insignificantly different.
Figure 1. Effects of different carriers on total phenolic content (TPC, mg GAE/g DW) of spray-dried (SD) and freeze-dried (FD) roselle powder. (Notes: MD: 100% maltodextrin, GA: 100% gum Arabic, MD/GA: 50% maltodextrin + 50% gum Arabic, MD/INU: 50% maltodextrin + 50% inulin, MD/KON: 50% maltodextrin + 50% konjac, KON: 100% konjac; Different letters within each method indicate that the mean values were significantly different at 95% confidence level).
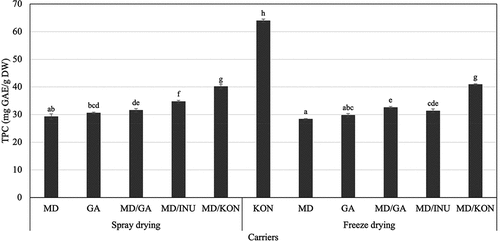
In spray drying and freeze drying, biopolymers such as proteins and polysaccharides are frequently employed as carriers. Polysaccharides such as gums, modified starch, and maltodextrin (DE < 21) are regarded to be effective wall materials for protecting the core material.[Citation22,Citation59] The majority of gums and hydrocolloids, such as gum Arabic, alginates, and pectins, are thickening and gelling agents used to enhance the viscosity of the continuous phase in emulsions or gels.[Citation22,Citation59,Citation60] Spray drying is a continuous drying process that has the advantages of a shorter drying time and a higher effectiveness of microencapsulation than other methods.[Citation22,Citation61] However, decomposition may occur as a result of the use of high temperatures.[Citation62,Citation63] Meanwhile, freeze-drying is a process that involves the sublimation of water under low pressure and is thus suitable for heat-labile substances.[Citation22] The loss of phenolic compounds during spray drying is due to oxygen exposure and high temperatures, which result in breakdown and polymerization of phenolics, while the grinding stage following freeze drying also results in oxidation.[Citation64] Ballesteros et al.[Citation44] concluded that the freeze-drying process was shown to be more effective than spray drying in encapsulating phenolic components of spent coffee grounds. This is partially explained by the distinct morphologies of the microcapsules formed by the two drying methods. The sawdust-like shape of lyophilized powder samples had a lower surface area/volume ratio than the spray-dried sample’s microsphere form, which led to the release of active compounds to the surface and subsequent decomposition.[Citation44] Nevertheless, our study revealed negligible difference in the TPC of samples obtained from two drying methods using the same carriers.
The type of microencapsulation material plays an important role in the retention of antioxidants in microcapsules. The research of Díaz-Bandera et al.[Citation41] showed that within multiple carriers (maltodextrin, gum Arabic, gelatin, pectin, carboxymethyl cellulose, whey protein) in the spray drying of anthocyanin extracts from roselle calyces, gum Arabic exhibited a higher recovery of TPC (82.01 mg GAE/100 g) than maltodextrin (77.6 mg GAE/100 g). In addition, Ballesteros et al.[Citation44] used spray-drying and freeze-drying to encapsulate phenolic compounds from spent coffee grounds. The combination of a maltodextrin carrier (20 g/100 mL extract) and freeze-drying generated samples with a significantly high phenolic and flavonoid content. However, spray-dried maltodextrin samples at 100°C had the highest FRAP activity. When comparing the effectiveness of different carriers of the same drying technique, maltodextrin outperformed gum Arabic and a 1:1 blend of maltodextrin and gum Arabic in terms of total phenolic content during freeze-drying, whereas the carrier blend maintained this group of compounds better during spray drying.[Citation44] Additionally, Idham et al.[Citation42] reported that among spray-dried powder samples of roselle anthocyanins using four different carriers (maltodextrin, gum Arabic, a combination of maltodextrin and gum Arabic, and soluble starch), the sample containing a mixture of carriers had the lowest decomposition rate. Previously, inulin was found to be less effective than maltodextrin in encapsulating polyphenols from cactus fruit.[Citation65] These results were consistent with the findings of Gharsallaoui et al.[Citation17] who concluded that using a single wall material in microencapsulation process does not have all the necessary properties; therefore, a mixture of carbohydrates with proteins and other polysaccharides leads to the highest efficiency and retention.
Effect of different carriers on TAC, SAC, and EE of spray-dried and freeze-dried roselle powder
Microencapsulation effectiveness is determined by the retention of the core material and the diminution of active chemicals on the surface of the powder particles, which is quantified as the encapsulation efficiency. Microencapsulation efficiency is a critical metric for microcapsules because it indicates the wall material’s ability to encapsulate or retain the core material inside the microcapsules.[Citation50] For anthocyanin was chosen as the core material in this research, the EE of anthocyanin was determined. Effects of different carriers on TAC and SAC of spray-dried and freeze-dried roselle powder are exhibited in . When the TAC of spray-dried and freeze-dried powder samples with various carriers was compared, it was observed that this value followed a similar trend to that of TPC. Spray-dried MD/INU, MD/KON, and freeze-dried KON samples, on the other hand, exhibited substantially higher SAC values than the other samples. This led to a substantial reduction in the microencapsulation effectiveness of all three samples, especially the lyophilized KON, which had a lowest EE of 43.6%. The EE of the remaining samples varied between 83.0 and 95.7%. Additionally, freeze-drying was shown to be more effective than spray drying in encapsulating anthocyanins within microcapsules, and a binary mix of MD/GA was found to be the best carrier in terms of antioxidant contents and encapsulation efficiency. Due to the high solubility and emulsion property of maltodextrin/gum Arabic blend, when the food mixture was sprayed dried, the resultant powder consisted of hollow particles with a matrix of the carrier enclosing the extracts.[Citation66] As mentioned above, the combination of carbohydrates and proteins enhances core material encapsulation within the polymer matrix.[Citation17,Citation50] Gum Arabic is a branched heteropolysaccharide that contains a minor amount of protein attached to carbohydrate chains. It has a high capacity for film formation, which assists maltodextrin in forming a dense network and preventing active compounds from being leaked to the surface.[Citation50,Citation67] The protein fraction plays a crucial role in determining the functional properties of gum Arabic.[Citation68] In contrast, starchy materials such as konjac glucomannan may be unable to create thick wall systems to protect anthocyanins from loss, and the pigments will only adhere to the surface of starch granules.
Figure 2. Effects of different carriers on total anthocyanin (TAC, mg C3G/g DW), surface anthocyanin (SAC, mg C3G/g DW) contents and encapsulation efficiency (EE, %) of spray-dried and freeze-dried roselle powder. (Notes: MD: 100% maltodextrin, GA: 100% gum Arabic, MD/GA: 50% maltodextrin + 50% gum Arabic, MD/INU: 50% maltodextrin + 50% inulin, MD/KON: 50% maltodextrin + 50% konjac, KON: 100% konjac; Different letters within each method indicate that the mean values were significantly different at 95% confidence level).
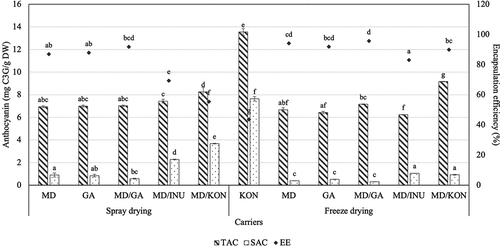
Laokuldilok and Kanha[Citation45] used maltodextrin to encapsulate anthocyanins from black glutinous rice bran. The results indicated that the freeze-dried sample retains more anthocyanin than the spray-dried sample. According to the authors, freeze drying more successfully mitigates the detrimental effects of high temperature on thermosensitive components such as anthocyanins.[Citation45] However, anthocyanin degradation occurred due to the prolonged freeze drying procedure, which increased the organic acid content in the raw material, while the flavylium ions were protected in the microcapsules due to their low pH.[Citation45,Citation69] Rezende et al.[Citation46] also obtained acerola powder using spray drying and freeze drying using a mixture of maltodextrin and gum Arabic. The results showed that freeze-dried samples had a lower level of anthocyanin loss than the spray-dried one. This is due to the use of low temperature drying and vacuum conditions during operation.[Citation46]
According to Jafari et al.,[Citation15] the properties of wall and core materials, as well as emulsion properties and drying parameters (especially spray drying conditions such as inlet and outlet temperature, speed feed flow, airflow and humidity, particle size), are factors that may affect microencapsulation performance. Idham et al.[Citation70] employed maltodextrin, gum Arabic, a maltodextrin-gum Arabic combination, and soluble starch in their work on spray-drying of roselle anthocyanin extracts to compare the degradation kinetics of the resulting powder with the control sample. The findings indicated that the microencapsulation effectiveness of the four types of carriers was extremely high (>96.7%), with blend, maltodextrin, gum Arabic, and soluble starch ranked in the descending order. Ferrari et al.[Citation47] similarly observed that a mixture of maltodextrin and gum Arabic, or maltodextrin alone, retained the most anthocyanin and antioxidant activity in spray-dried blackberry powder samples. Additionally, Farias-Cervantes et al.[Citation43] used three different types of carriers, namely a gum Arabic-maltodextrin combination, whey protein isolate, and agave fructans to encapsulate anthocyanins from blackberry, raspberry, strawberry, and roselle. The results indicated that the best carrier for microencapsulating blackberry anthocyanins was a gum Arabic-maltodextrin combination, whereas the best carrier for strawberry and roselle was whey protein isolate. The gum Arabic-maltodextrin blend’s excellent efficiency can be ascribed to its emulsifying characteristics.[Citation43] Sakulnarmrata et al.[Citation48] used a drum drying process to encapsulate purple cabbage extracts with a combination of maltodextrin (DE 20) and gum Arabic in various ratios. The results indicated that a 20:80 combination of maltodextrin and gum Arabic had the highest anthocyanin microencapsulation efficiency (98.85%) and a moisture level of 3.40–4.66%.[Citation48] Combinations of two or three polymers are also effective for spray drying a variety of products, including cinnamon oleoresin (a 4:1:1 combination of gum Arabic, maltodextrin, and modified starch),[Citation71] anthocyanins from barberry (Berberis vulgaris) (a 3:1 combination of maltodextrin and gum Arabic),[Citation50] and kaffir lime oil (a 4:1 blend of konjac glucomannan and gum Arabic).[Citation72] However, Pieczykolan and Kurek[Citation49] reported that when MD/GA and MD/INU carrier mixes (mass ratio of 74:6) were employed to encapsulate chokeberry fruit extracts, the MD/INU carrier combination demonstrated a greater microencapsulation efficiency and anthocyanin content than the MD/GA carrier mixture. It can be seen that the ratio between the carriers also significantly affected their microencapsulation efficiency. In addition, the reduction in the molecular weight of the polymer also leads to a decrease in the strength of the microcapsules, and materials with good film-forming properties (such as those containing high amylose content) aid in protecting anthocyanins from the detrimental effects of environmental conditions during heat treatment.[Citation45]
Effect of different carriers on antioxidant activities of spray-dried and freeze-dried roselle powder
The presence of various antioxidants, each with a distinct mechanism of action, determines antioxidant capacity of food ingredients and products. As a result, the antioxidant capacity of food items should be evaluated using a variety of assays.[Citation73] In this study, in vitro antioxidant activities of spray-dried and freeze-dried roselle powder were evaluated using four assays, namely ABTS and DPPH radical scavenging method, ferric reducing antioxidant power (FRAP), and cupric reducing antioxidant capacity (CUPRAC). Regarding the action mechanisms, while the two former assays are associated with the radical quenching capacity, the two latter are related to the reduction of multi-valent transition metals such as ferric and cupric ions because these ions play a catalytic role in chain oxidation reactions.[Citation56] Effects of different carriers on antioxidant activities of spray-dried and freeze-dried roselle powder are exhibited in . The results showed that when the antioxidant activity of spray-dried and freeze-dried hibiscus powder samples was examined, distinct patterns of activity variation across samples were observed. The difference in the free radical scavenging activities of DPPH and ABTS was comparable to the change in the function of the metal ions. Due to the high TPC and TAC, the freeze-dried KON sample retained the highest antioxidant capacity, followed by the spray-dried MD/KON sample. It should be observed that, although the other samples had comparable free radical scavenging activity, the spray-dried samples had substantially greater activity to reduce metal ion (ferric and cupric ions) than the freeze-dried ones, especially CUPRAC values.
Figure 3. Effects of different carriers on antioxidant activities (mg TE/g DW) of spray-dried and freeze-dried roselle powder including DPPH radical scavenging activity, ABTS cation radical reduction ability, ferric reducing antioxidant power – FRAP, cupric reducing antioxidant capacity – CUPRAC. (Notes: MD: 100% maltodextrin, GA: 100% gum Arabic, MD/GA: 50% maltodextrin + 50% gum Arabic, MD/INU: 50% maltodextrin + 50% inulin, MD/KON: 50% maltodextrin + 50% konjac, KON: 100% konjac; Different letters within each method indicate that the mean values were significantly different at 95% confidence level).
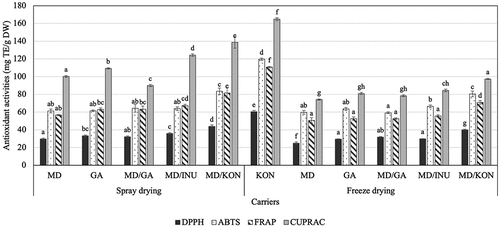
Farias-Cervantes et al.[Citation43] observed that the inclusion of a carrier greatly increased the powder’s antioxidant activity. Additionally, the authors discovered that samples with a high degree of microencapsulation had a significant level of antioxidant activity, which was particularly noticeable in samples with a high anthocyanin and phenolic content.[Citation43] Kuck et al.[Citation64] microencapsulated phenolic extracts from Bordo grape skins by spray drying and freeze drying and concluded that both drying techniques resulted in the loss of phenolic components. Spray drying induces thermal denaturation of proteins and alters their hydrophobic, electrostatic, hydrogen bonding, and disulfide–sulfhydryl interactions, all of which have an influence on their antioxidant capacity.[Citation43] During the spray drying process, the high temperature dramatically accelerates the rate of moisture removal, resulting in the rugged surface of the microsphere, releasing a portion of the active chemicals and causing their deterioration.[Citation46,Citation74] Freeze drying results in the formation of a porous product as a result of the sublimation of water.[Citation64] However, the anthocyanin content and DPPH free radical scavenging activity were not substantially different between freeze-dried and spray-dried samples employing 10% gum Arabic, but the TPC and CUPRAC value of spray-dried samples were significantly higher.[Citation64] Oki et al.[Citation75] also reported that the dominant antioxidant activity in purple sweet potato powder was attributed to anthocyanin. At least one caffeoyl group acylated to anthocyanin contributes to high free radical activity, suggesting that total antioxidant activity is highly correlated with total phenolic and anthocyanin content.[Citation76] The increase in antioxidant activity in these samples is probably due to the hydrolysis of phenolic compounds in the drying process produced with a blend of carriers,[Citation65,Citation77] even to the formation or release of antioxidants that can inactivate free radicals.[Citation78] In addition, the antioxidant activity of the sample also depends on the oxidative stability of the carrier.[Citation79]
Effect of different carriers on moisture content and solubility of spray-dried and freeze-dried roselle powder
Moisture and solubility are two important parameters that represent the quality of instant powder products. Products with low moisture content and high solubility are considered good quality products.[Citation80] Moisture is an important powder property, related to drying efficiency, the flowability of powder, stickiness, and storage stability due to its effect on glass transition and crystallization behavior. Furthermore, lower moisture content limits the ability of water activity as a plasticizer and reduces the glass transition temperature.[Citation81] Solubility is an important quality property of food powders because it affects the functional properties of powder in the food system.[Citation82] The term solubility of the user used here refers to the ability of the powder to form a solution or suspension in water. During spray drying, the high feed flow rate negatively affects the moisture content of the powder, stemming from the short contact time between the atomized feed and the drying agent, leading to inefficient heat transfer and low water evaporation rate.[Citation83] In this study, the feed flow rate was fixed at 500 mL/h based on preliminary investigations. Effects of different carriers on moisture content and solubility of spray-dried and freeze-dried roselle powder are exhibited in . Regarding the physical properties of roselle powder, spray-dried powder showed significantly higher moisture content than freeze-dried samples with values ranging from 6.5–12.1% and 2.7–5.0%, respectively. Solubility between samples showed negligible difference and was in the range of 94.1–98.5%. Additionally, we discovered that the freeze-dried powder sample had a considerably greater hygroscopicity than the spray-dried sample, resulting in fast humidification from the surrounding environment.
Figure 4. Effects of different carriers on moisture content (%) and solubility (%) of spray-dried and freeze-dried roselle powder. (Notes: MD: 100% maltodextrin, GA: 100% gum Arabic, MD/GA: 50% maltodextrin + 50% gum Arabic, MD/INU: 50% maltodextrin + 50% inulin, MD/KON: 50% maltodextrin + 50% konjac, KON: 100% konjac; Different letters within each method indicate that the mean values were significantly different at 95% confidence level).
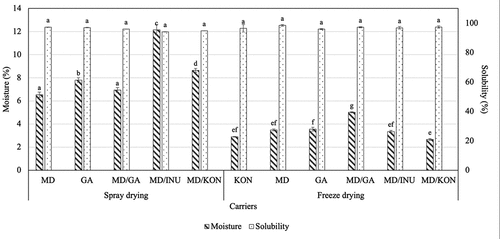
Typically, spray-dried products have a moisture content of less than 5%. The moisture content of the product is greatly affected by the parameters of spray drying conditions, particularly types of carriers. Furthermore, the moisture content of the spray-dried samples was substantially lower (2.41–2.57%) than that of the freeze-dried samples (7.65–8.06%).[Citation64] Inulin does not have any emulsifying properties, and inulin is a strong hygroscopic material is the reason why the EE of spray-dried MD/INU showed highest moisture content (12.10%). The change in moisture content can be due to the chemical structure of INU and MD, with the high degree of branching with hydrophilic groups and therefore, can easily bind the water molecules from the surrounding air in the powder.
The solubility of spray-dried and freeze-dried powders was comparable, similar to the results for acerola powder (99.0–99.1%).[Citation46] Other findings showing the differences in solubility of spray-dried and freeze-dried powder were obtained in terms of solubility, with values falling within the range of 94.3–97.99% and 85.96–88% in grape skin powder[Citation64] and 76.23–91.79% and 82.14–84.15% in rice bran anthocyanin powder[Citation45] for spray-dried and freeze-dried samples, respectively.
Pearson correlation between contents of phenolics, anthocyanins and antioxidant activities of spray-dried and freeze-dried roselle powder
Pearson correlation coefficients between contents of phenolics, anthocyanins with antioxidant activities and color parameters of spray-dried and freeze-dried roselle powder are exhibited in . It can be observed that TPC and TAC had a strong positive correlation (P < .01) with all four antioxidant activity values with correlation coefficients for DPPH, ABTS, FRAP all above 0.942. However, cupric ion reducing activity of phenolic and anthocyanin of hibiscus powder had a lower correlation with correlation coefficients of 0.825 and 0.799, respectively.
Table 2. Pearson correlation between the contents of phenolics, anthocyanins and antioxidant activities (DPPH free radical scavenging activity, ABTS cation radical scavenging capacity, ferric reducing antioxidant power – FRAP, and cupric reducing antioxidant capacity – CUPRAC) of spray-dried and freeze-dried roselle powder
In our other study on spray drying conditions of hibiscus extract using maltodextrin as carrier, the correlation between TPC, TAC and antioxidant activities was also calculated.[Citation84] The results showed that the correlation coefficients ranged between 0.703 and 0.884, with the correlation coefficients of ABTS with the TPC and TAC content being particularly low (0.718 and 0.713, respectively). In comparison to the present study, this further confirmed the role of the carriers, the drying methods and conditions in the retention of activity and content of antioxidant compounds in the resulting powder.
Conclusion
Spray-drying and freeze-drying are two popular and effective methods for microencapsulating phenolic compounds, especially anthocyanins, with the aim of protecting the activity of this group of compounds. When comparing the efficiency between a wide range of carriers, including single carriers and binary blends, the use of a mixture of maltodextrin and gum Arabic as carriers led to the development of spray-dried and freeze-dried powder with relatively high antioxidant content and exceptional encapsulation efficiency (above 90%). Meanwhile, although the use of single konjac glucomannan, a homopolysaccharide, created the best powder with highest antioxidant contents and activities, anthocyanins seemed to be mostly located on the surface of microcapsules, as demonstrated by low encapsulation efficiency. However, this drawback of konjac glucomannan can be overcome by using different coatings to limit oxidation of anthocyanins on the surface of particles. Overall, there was no significant difference in phenolic, anthocyanin content and free radical scavenging activity between the two drying methods, except that the multivalent metal ion reduction capacities of the spray-dried samples were significantly higher than those of the freeze-dried samples. Additionally, spray-dried powder had a substantially greater moisture content than freeze-dried powder.
Acknowledgments
The authors would like to thank Nguyen Tat Thanh University for permission and for providing facilities during the research period.
Disclosure statement
No potential conflict of interest was reported by the author(s).
References
- Konczak, I.; Zhang, W. Anthocyanins—more than Nature’s Colours. Biomed Res. Int. 2004, 2004(5), 239–240.
- Wang, W.; Jung, J.; Zhao, Y. Chitosan-cellulose Nanocrystal Microencapsulation to Improve Encapsulation Efficiency and Stability of Entrapped Fruit Anthocyanins. Carbohydr. Polym. 2017, 157, 1246–1253. DOI: 10.1016/j.carbpol.2016.11.005.
- Fernández-López, J. A.; Angosto, J. M.; Giménez, P. J.; León, G. Thermal Stability of Selected Natural Red Extracts Used as Food Colorants. Plant Foods Human Nutr. 2013, 68(1), 11–17. DOI: 10.1007/s11130-013-0337-1.
- Yonekura-Sakakibara, K.; Nakayama, T.; Yamazaki, M., and Saito, K. Modification and Stabilization of Anthocyanins. In Anthocyanins: Biosynthesis, Functions, and Applications eds. Gould, K., Davies, K. M., and Winefield, C.; New York: Springer Science & Business Media, 2008; pp 169–190.
- Cid-Ortega, S.; Guerrero-Beltrán, J. A. Roselle Calyces (Hibiscus Sabdariffa), an Alternative to the Food and Beverages Industries: A Review. J. Food Sci. Technol. 2015, 52(11), 6859–6869. DOI: 10.1007/s13197-015-1800-9.
- Archaina, D.; Vasile, F.; Jiménez‐Guzmán, J.; Alamilla‐Beltrán, L., and Schebor, C. Physical and Functional Properties of Roselle (Hibiscus Sabdariffa L.) Extract Spray Dried with Maltodextrin‐gum Arabic Mixtures. J. Food Process. Preserv. 43(9) 2019, e14065 doi:10.1111/jfpp.14065.
- Aurelio, D.; Edgardo, R. G.; Navarro‐Galindo, S. Thermal Kinetic Degradation of Anthocyanins in a Roselle (Hibiscus Sabdariffa L. cv.‘Criollo’) Infusion. Int. J. Food Sci. Technol. 2008, 43(2), 322–325. DOI: 10.1111/j.1365-2621.2006.01439.x.
- Borrás-Linares, I.; Fernández-Arroyo, S.; Arráez-Roman, D.; Palmeros-Suárez, P. A.; Del Val-Díaz, R.; Andrade-Gonzáles, I.; Fernández-Gutiérrez, A.; Gómez-Leyva, J. F.; Segura-Carretero, A., et al. Characterization of Phenolic Compounds, Anthocyanidin, Antioxidant and Antimicrobial Activity of 25 Varieties of Mexican Roselle (Hibiscus Sabdariffa). Ind. Crops Prod. 2015, 69, 385–394. DOI: 10.1016/j.indcrop.2015.02.053.
- Ekici, L.; Simsek, Z.; Ozturk, I.; Sagdic, O.; Yetim, H. Effects of Temperature, Time, and pH on the Stability of Anthocyanin Extracts: Prediction of Total Anthocyanin Content Using Nonlinear Models. Food Anal. Methods. 2014, 7(6), 1328–1336. DOI: 10.1007/s12161-013-9753-y.
- Zhao, J. Nutraceuticals, Nutritional Therapy, Phytonutrients, and Phytotherapy for Improvement of Human Health: A Perspective on Plant Biotechnology Application. Recent patents on biotechnology. 2007, 1(1), 75–97. DOI: 10.2174/187220807779813893.
- Abou-Arab, A. A.; Abu-Salem, F. M.; Abou-Arab, E. A. Physico-chemical Properties of Natural Pigments (Anthocyanin) Extracted from Roselle Calyces (Hibiscus Subdariffa). J. Am. Sci. 2011, 7(7), 445–456.
- Hounkpè, L. H., et al. Nutritional Valorization and Chemical Composition of Seeds of Hibiscus Sabdariffa Sabdariffa from Benin. American Journal of Food Science and Technology. 2019, 7(5), 146–151.
- de Vos, P.; Faas, M. M.; Spasojevic, M.; Sikkema, J. Encapsulation for Preservation of Functionality and Targeted Delivery of Bioactive Food Components. Int. Dairy J. 2010, 20(4), 292–302. DOI: 10.1016/j.idairyj.2009.11.008.
- Champagne, C. P.; Fustier, P. Microencapsulation for the Improved Delivery of Bioactive Compounds into Foods. Curr. Opin. Biotechnol. 2007, 18(2), 184–190. DOI: 10.1016/j.copbio.2007.03.001.
- Jafari, S. M.; Assadpoor, E.; He, Y.; Bhandari, B. Encapsulation Efficiency of Food Flavours and Oils during Spray Drying. Drying Technol. 2008, 26(7), 816–835. DOI: 10.1080/07373930802135972.
- Calvo, P.; Castaño, Á. L.; Hernández, M. T.; González‐Gómez, D. Effects of Microcapsule Constitution on the Quality of Microencapsulated Walnut Oil. Eur. J. Lipid Sci. Technol. 2011, 113(10), 1273–1280. DOI: 10.1002/ejlt.201100039.
- Gharsallaoui, A.; Roudaut, G.; Chambin, O.; Voilley, A.; Saurel, R. Applications of Spray-drying in Microencapsulation of Food Ingredients: An Overview. Food Res. Int. 2007, 40(9), 1107–1121. DOI: 10.1016/j.foodres.2007.07.004.
- Angel, R. C. M.; Espinosa-Muñoz, L. C.; Aviles-Aviles, C.; González-García, R.; Moscosa-Santillán, M.; Grajales-Lagunes, A.; Abud-Archila, M., et al. Spray-drying of Passion Fruit Juice Using Lactose-maltodextrin Blends as the Support Material. Braz. Arch. Biol. Technol. 2009, 52(4), 1011–1018.
- Fang, Z.; Bhandari, B. Comparing the Efficiency of Protein and Maltodextrin on Spray Drying of Bayberry Juice. Food Res. Int. 2012, 48(2), 478–483. DOI: 10.1016/j.foodres.2012.05.025.
- Augustin, M. A.; Hemar, Y. Nano-and Micro-structured Assemblies for Encapsulation of Food Ingredients. Chem. Soc. Rev. 2009, 38(4), 902–912. DOI: 10.1039/B801739P.
- Deshmukh, R.; Wagh, P.; Naik, J. Solvent Evaporation and Spray Drying Technique for Micro-and Nanospheres/particles Preparation: A Review. Drying Technol. 2016, 34(15), 1758–1772. DOI: 10.1080/07373937.2016.1232271.
- Rezvankhah, A.; Emam-Djomeh, Z.; Askari, G. Encapsulation and Delivery of Bioactive Compounds Using Spray and Freeze-drying Techniques: A Review. Drying Technol. 2020, 38(1–2), 235–258. DOI: 10.1080/07373937.2019.1653906.
- Fang, Z.; Bhandari, B. Encapsulation of Polyphenols–a Review. Trends Food Sci. Technol. 2010, 21(10), 510–523. DOI: 10.1016/j.tifs.2010.08.003.
- Goula, A. M.; Adamopoulos, K. G. A Method for Pomegranate Seed Application in Food Industries: Seed Oil Encapsulation. Food Bioprod. Process. 2012, 90(4), 639–652. DOI: 10.1016/j.fbp.2012.06.001.
- Cano-Chauca, M.; Stringheta, P. C.; Ramos, A. M.; Cal-Vidal, J. Effect of the Carriers on the Microstructure of Mango Powder Obtained by Spray Drying and Its Functional Characterization. Innovative Food Sci. Emerg. Technol. 2005, 6(4), 420–428. DOI: 10.1016/j.ifset.2005.05.003.
- Gabas, A. L.; Telis, V. R. N.; Sobral, P. J. A.; Telis-Romero, J. Effect of Maltodextrin and Arabic Gum in Water Vapor Sorption Thermodynamic Properties of Vacuum Dried Pineapple Pulp Powder. J. Food Eng. 2007, 82(2), 246–252. DOI: 10.1016/j.jfoodeng.2007.02.029.
- Quek, S. Y.; Chok, N. K.; Swedlund, P. The Physicochemical Properties of Spray-dried Watermelon Powders. Chem. Eng. Process. 2007, 46(5), 386–392. DOI: 10.1016/j.cep.2006.06.020.
- Finney, J.; Buffo, R.; Reineccius, G. A. Effects of Type of Atomization and Processing Temperatures on the Physical Properties and Stability of Spray‐dried Flavors. J. Food Sci. 2002, 67(3), 1108–1114.
- Krishnan, S.; Bhosale, R.; Singhal, R. S. Microencapsulation of Cardamom Oleoresin: Evaluation of Blends of Gum Arabic, Maltodextrin and a Modified Starch as Wall Materials. Carbohydr. Polym. 2005, 61(1), 95–102. DOI: 10.1016/j.carbpol.2005.02.020.
- Williams, P. A.; Idris, O. H. M., and Phillips, G. O. Structural Analysis of Gum from Acacia Senegal (Gum Arabic). In Cell and Developmental Biology of Arabinogalactan-proteins eds. Northnagel, E. A., Bacic, A., and Clarke, A. E.; New York: Springer Science & Business Media, 2012; pp 241–251.
- Hosseini, A.; Jafari, S. M.; Mirzaei, H.; Asghari, A.; Akhavan, S. Application of Image Processing to Assess Emulsion Stability and Emulsification Properties of Arabic Gum. Carbohydr. Polym. 2015, 126, 1–8. DOI: 10.1016/j.carbpol.2015.03.020.
- McNamee, B. F.; O’Riorda, E. D.; O’Sullivan, M. Emulsification and Microencapsulation Properties of Gum Arabic. J. Agric. Food Chem. 1998, 46(11), 4551–4555. DOI: 10.1021/jf9803740.
- Yuan, Y.; Wang, L.; Mu, R.-J.; Gong, J.; Wang, Y.; Li, Y.; Ma, J.; Pang, J.; Wu, C., et al. Effects of Konjac Glucomannan on the Structure, Properties, and Drug Release Characteristics of Agarose Hydrogels. Carbohydr. Polym. 2018, 190, 196–203. DOI: 10.1016/j.carbpol.2018.02.049.
- Molavi, H.; Behfar, S.; Shariati, M. A.; Kaviani, M.; Atarod, S. A Review on Biodegradable Starch Based Film. J. Microbiol. Biotechnol. Food Sci. 2021, 2021, 456–461.
- Nair, S. B.; Jyothi, A. N.; Sajeev, M. S., and Misra, R. Starch‐Stärke 63(11) . 2011 Rheological, mechanical and moisture sorption characteristics of cassava starch‐konjac glucomannan blend films , 728–739. doi:10.1002/star.201100051
- Wang, K.; Gao, S.; Shen, C.; Liu, J.; Li, S.; Chen, J.; Ren, X.; Yuan, Y., et al. Preparation of Cationic Konjac Glucomannan in NaOH/urea Aqueous Solution. Carbohydr. Polym. 2018, 181, 736–743. DOI: 10.1016/j.carbpol.2017.11.084.
- Chen, H.; Lan, G.; Ran, L.; Xiao, Y.; Yu, K.; Lu, B.; Dai, F.; Wu, D.; Lu, F., et al. A Novel Wound Dressing Based on A Konjac Glucomannan/silver Nanoparticle Composite Sponge Effectively Kills Bacteria and Accelerates Wound Healing. Carbohydr. Polym. 2018, 183, 70–80. DOI: 10.1016/j.carbpol.2017.11.029.
- Afinjuomo, F.; Fouladian, P.; Parikh, A.; Barclay, T. G.; Song, Y.; Garg, S. Preparation and Characterization of Oxidized Inulin Hydrogel for Controlled Drug Delivery. Pharmaceutics. 2019, 11(7), 356. DOI: 10.3390/pharmaceutics11070356.
- López-Molina, D.; Chazarra, S.; How, C. W.; Pruidze, N.; Navarro-Perán, E.; García-Cánovas, F.; García-Ruiz, P. A.; Rojas-Melgarejo, F.; Rodríguez-López, J. N., et al. Cinnamate of Inulin as a Vehicle for Delivery of Colonic Drugs. Int. J. Pharmaceutics.2015, 479(1), 96–102. DOI: 10.1016/j.ijpharm.2014.12.064.
- Jain, A. K.; Sood, V.; Bora, M.; Vasita, R.; Katti, D. S. Electrosprayed Inulin Microparticles for Microbiota Triggered Targeting of Colon. Carbohydr. Polym. 2014, 112, 225–234. DOI: 10.1016/j.carbpol.2014.05.087.
- Díaz-Bandera, D.; Villanueva-Carvajal, A.; Dublán-García, O.; Quintero-Salazar, B.; Dominguez-Lopez, A. Assessing Release Kinetics and Dissolution of Spray-dried Roselle (Hibiscus Sabdariffa L.) Extract Encapsulated with Different Carrier Agents. LWT Food Sci. Technol. 2015, 64(2), 693–698. DOI: 10.1016/j.lwt.2015.06.047.
- Idham, Z.; Muhamad, I. I.; Mohd Setapar, S. H., and Sarmidi, M. R. Effect of Thermal Processes on Roselle Anthocyanins Encapsulated in Different Polymer Matrices. J. Food Process. Preserv. 2012, 36(2), 176–184.
- Farias‐Cervantes, V. S.; Chávez‐Rodríguez, A.; García‐Salcedo, P. A.; García‐López, P. M.; Casas‐Solís, J.; Andrade‐González, I. Antimicrobial Effect and in Vitro Release of Anthocyanins from Berries and Roselle Obtained via Microencapsulation by Spray Drying. J. Food Process. Preserv. 2018, 42(10), e13713. DOI: 10.1111/jfpp.13713.
- Ballesteros, L. F.; Ramirez, M. J.; Orrego, C. E.; Teixeira, J. A.; Mussatto, S. I. Encapsulation of Antioxidant Phenolic Compounds Extracted from Spent Coffee Grounds by Freeze-drying and Spray-drying Using Different Coating Materials. Food Chem. 2017, 237, 623–631. DOI: 10.1016/j.foodchem.2017.05.142.
- Laokuldilok, T.; Kanha, N. Effects of Processing Conditions on Powder Properties of Black Glutinous Rice (Oryza Sativa L.) Bran Anthocyanins Produced by Spray Drying and Freeze Drying. LWT Food Sci. Technol. 2015, 64(1), 405–411. DOI: 10.1016/j.lwt.2015.05.015.
- Rezende, Y. R. R. S.; Nogueira, J. P.; Narain, N. Microencapsulation of Extracts of Bioactive Compounds Obtained from Acerola (Malpighia Emarginata DC) Pulp and Residue by Spray and Freeze Drying: Chemical, Morphological and Chemometric Characterization. Food Chem. 2018, 254, 281–291. DOI: 10.1016/j.foodchem.2018.02.026.
- Ferrari, C. C.; Pimentel, S.; Germer, M.; Alvim, I. D., and Vissotto, F. Z. Influence of Carrier Agents on the Physicochemical Properties of Blackberry Powder Produced by Spray Drying Int. J. Food Sci . , 47(6) , 1237–1245 2012. doi:10.1111/j.1365-2621.2012.02964.x
- Sakulnarmrat, K.; Wongsrikaew, D.; Konczak, I. Microencapsulation of Red Cabbage Anthocyanin-rich Extract by Drum Drying Technique. LWT. 2021, 137, 110473. DOI: 10.1016/j.lwt.2020.110473.
- Pieczykolan, E.; Kurek, M. A. Use of Guar Gum, Gum Arabic, Pectin, Beta-glucan and Inulin for Microencapsulation of Anthocyanins from Chokeberry. Int. J. Biol. Macromol. 2019, 129, 665–671. DOI: 10.1016/j.ijbiomac.2019.02.073.
- Mahdavi, S. A.; Jafari, S. M.; Assadpoor, E.; Dehnad, D. Microencapsulation Optimization of Natural Anthocyanins with Maltodextrin, Gum Arabic and Gelatin. Int. J. Biol. Macromol. 2016, 85, 379–385. DOI: 10.1016/j.ijbiomac.2016.01.011.
- Pantelidis, G. E.; Vasilakakis, M.; Manganaris, G. A.; Diamantidis, G. Antioxidant Capacity, Phenol, Anthocyanin and Ascorbic Acid Contents in Raspberries, Blackberries, Red Currants, Gooseberries and Cornelian Cherries. Food Chem. 2007, 102(3), 777–783. DOI: 10.1016/j.foodchem.2006.06.021.
- ISO, “ISO14502-1: 2005 Determination of Substances Characteristic of Green and Black Tea. Part 1. Content of Total Polyphenols in Tea—colorimetric Method Using Folin–Ciocalteu Reagent.,” 2005.
- Brand-Williams, W.; Cuvelier, M.-E.; Berset, C. Use of a Free Radical Method to Evaluate Antioxidant Activity. LWT Food Sci. Technol. 1995, 28(1), 25–30. DOI: 10.1016/S0023-6438(95)80008-5.
- Arnao, M. B.; Cano, A.; Acosta, M. The Hydrophilic and Lipophilic Contribution to Total Antioxidant Activity. Food Chem. 2001, 73(2), 239–244. DOI: 10.1016/S0308-8146(00)00324-1.
- Benzie, I. F. F.; Strain, J. J. The Ferric Reducing Ability of Plasma (FRAP) as a Measure of ‘Antioxidant Power’: The FRAP Assay. Anal. Biochem. 1996, 239(1), 70–76. DOI: 10.1006/abio.1996.0292.
- Apak, R.; Güçlü, K.; Özyürek, M.; Esin Karademir, S.; Erçağ, E. The Cupric Ion Reducing Antioxidant Capacity and Polyphenolic Content of Some Herbal Teas. Int. J. Food Sci. Nutr. 2006, 57(5–6), 292–304. DOI: 10.1080/09637480600798132.
- de Almeida Paula, D.; Martins, E. M. F.; de Almeida Costa, N.; de Oliveirade Oliveira, P. M.; de Oliveira, E. B.; Ramos, A. M. Use of Gelatin and Gum Arabic for Microencapsulation of Probiotic Cells from Lactobacillus Plantarum by a Dual Process Combining Double Emulsification Followed by Complex Coacervation. Int. J. Biol. Macromol. 2019, 133, 722–731. DOI: 10.1016/j.ijbiomac.2019.04.110.
- Ramírez-Rodrigues, M. M.; Plaza, M. L.; Azeredo, A.; Balaban, M. O.; Marshall, M. R. Phytochemical, Sensory Attributes and Aroma Stability of Dense Phase Carbon Dioxide Processed Hibiscus Sabdariffa Beverage during Storage. Food Chem. 2012, 134(3), 1425–1431. DOI: 10.1016/j.foodchem.2012.03.042.
- Tonon, R. V.; Pedro, R. B.; Grosso, C. R. F.; Hubinger, M. D. Microencapsulation of Flaxseed Oil by Spray Drying: Effect of Oil Load and Type of Wall Material. Drying Technol. 2012, 30(13), 1491–1501. DOI: 10.1080/07373937.2012.696227.
- Bouyer, E.; Mekhloufi, G.; Rosilio, V.; Grossiord, J.-L.; Agnely, F. Proteins, Polysaccharides, and Their Complexes Used as Stabilizers for Emulsions: Alternatives to Synthetic Surfactants in the Pharmaceutical Field? Int. J. Pharmaceutics. 2012, 436(1–2), 359–378. DOI: 10.1016/j.ijpharm.2012.06.052.
- Huang, X.; Sormoli, M. E.; Langrish, T. A. G. Review of Some Common Commercial and Noncommercial Lab-scale Spray Dryers and Preliminary Tests for a Prototype New Spray Dryer. Drying Technol. 2018, 36(15), 1900–1912. DOI: 10.1080/07373937.2018.1459679.
- Sosnik, A.; Seremeta, K. P. Advantages and Challenges of the Spray-drying Technology for the Production of Pure Drug Particles and Drug-loaded Polymeric Carriers. Adv. Colloid Interface Sci. 2015, 223, 40–54. DOI: 10.1016/j.cis.2015.05.003.
- Rajabi, H.; Ghorbani, M.; Jafari, S. M.; Mahoonak, A. S.; Rajabzadeh, G. Retention of Saffron Bioactive Components by Spray Drying Encapsulation Using Maltodextrin, Gum Arabic and Gelatin as Wall Materials. Food Hydrocolloids. 2015, 51, 327–337. DOI: 10.1016/j.foodhyd.2015.05.033.
- Kuck, L. S.; Noreña, C. P. Z. Microencapsulation of Grape (Vitis Labrusca Var. Bordo) Skin Phenolic Extract Using Gum Arabic, Polydextrose, and Partially Hydrolyzed Guar Gum as Encapsulating Agents. Food Chem. 2016, 194, 569–576. DOI: 10.1016/j.foodchem.2015.08.066.
- Saénz, C.; Tapia, S.; Chávez, J.; Robert, P. Microencapsulation by Spray Drying of Bioactive Compounds from Cactus Pear (Opuntia Ficus-indica). Food Chem. 2009, 114(2), 616–622. DOI: 10.1016/j.foodchem.2008.09.095.
- Tonon, R. V.; Brabet, C.; Pallet, D.; Brat, P.; Hubinger, M. D. Physicochemical and Morphological Characterisation of Açai (Euterpe Oleraceae Mart.) Powder Produced with Different Carrier Agents. Int. J. Food Sci. Technol. 2009, 44(10), 1950–1958. DOI: 10.1111/j.1365-2621.2009.02012.x.
- Burin, V. M.; Rossa, P. N.; Ferreira‐Lima, N. E.; Hillmann, M. C. R.; Boirdignon‐Luiz, M. T. Anthocyanins: Optimisation of Extraction from Cabernet Sauvignon Grapes, Microcapsulation and Stability in Soft Drink. Int. J. Food Sci. Technol. 2011, 46(1), 186–193. DOI: 10.1111/j.1365-2621.2010.02486.x.
- Randall, R. C.; Phillips, G. O.; Williams, P. A. The Role of the Proteinaceous Component on the Emulsifying Properties of Gum Arabic. Food Hydrocolloids. 1988, 2(2), 131–140. DOI: 10.1016/S0268-005X(88)80011-0.
- Tang, B., et al. Kinetic Investigation into pH-dependent Color of Anthocyanin and Its Sensing Performance. Dyes Pigm. 2019, 170, 107643. DOI: 10.1016/j.dyepig.2019.107643.
- Idham, Z.; Muhamad, I. I.; Sarmidi, M. R. Degradation Kinetics and Color Stability of Spray‐dried Encapsulated Anthocyanins from Hibiscus Sabdariffa L. J. Food Process Eng. 2012, 35(4), 522–542. DOI: 10.1111/j.1745-4530.2010.00605.x.
- Vaidya, S.; Bhosale, R.; Singhal, R. S. Microencapsulation of Cinnamon Oleoresin by Spray Drying Using Different Wall Materials. Drying Technol. 2006, 24(8), 983–992. DOI: 10.1080/07373930600776159.
- Adamiec, J.; Borompichaichartkul, C.; Srzednicki, G.; Panket, W.; Piriyapunsakul, S.; Zhao, J. Microencapsulation of Kaffir Lime Oil and Its Functional Properties. Drying Technol. 2012, 30(9), 914–920. DOI: 10.1080/07373937.2012.666777.
- Moo-Huchin, V. M.; Estrada-Mota, I.; Estrada-León, R.; Cuevas-Glory, L.; Ortiz-Vázquez, E.; Vargas, M. D. L. V. Y.; Betancur-Ancona, D.; Sauri-Duch, E., et al. Determination of Some Physicochemical Characteristics, Bioactive Compounds and Antioxidant Activity of Tropical Fruits from Yucatan, Mexico. Food Chem. 2014, 152, 508–515. DOI: 10.1016/j.foodchem.2013.12.013.
- Ramírez, M. J.; Giraldo, G. I.; Orrego, C. E. Modeling and Stability of Polyphenol in Spray-dried and Freeze-dried Fruit Encapsulates. Powder Technol. 2015, 277, 89–96. DOI: 10.1016/j.powtec.2015.02.060.
- Oki, T.; Masuda, M.; Furuta, S.; Nishiba, Y.; Terahara, N.; Suda, I. Involvement of Anthocyanins and Other Phenolic Compounds in Radical‐scavenging Activity of Purple‐fleshed Sweet Potato Cultivars. J. Food Sci. 2002, 67(5), 1752–1756. DOI: 10.1111/j.1365-2621.2002.tb08718.x.
- Huang, Y.-C.; Chang, Y.-H.; Shao, -Y.-Y. Effects of Genotype and Treatment on the Antioxidant Activity of Sweet Potato in Taiwan. Food Chem. 2006, 98(3), 529–538. DOI: 10.1016/j.foodchem.2005.05.083.
- Robert, P.; Gorena, T.; Romero, N.; Sepulveda, E.; Chavez, J.; Saenz, C. Encapsulation of Polyphenols and Anthocyanins from Pomegranate (Punica Granatum) by Spray Drying. Int. J. Food Sci. Technol. 2010, 45(7), 1386–1394. DOI: 10.1111/j.1365-2621.2010.02270.x.
- Pitalua, E.; Jimenez, M.; Vernon-Carter, E. J.; Beristain, C. I. Antioxidative Activity of Microcapsules with Beetroot Juice Using Gum Arabic as Wall Material. Food Bioprod. Process. 2010, 88(2–3), 253–258. DOI: 10.1016/j.fbp.2010.01.002.
- Samantha, S. C; Bruna, A. S. M.; Adriana, R. M.; Fabio, B.; Sandro, A. R.; Aline, R. C. A. Drying by Spray Drying in the Food Industry: Micro-encapsulation, Process Parameters and Main Carriers Used. Afr. J. Food Sci. 2015, 9(9), 462–470. DOI: 10.5897/AJFS2015.1279.
- Vardin, H.; Yasar, M. Optimisation of Pomegranate (Punica Granatum L.) Juice Spray‐drying as Affected by Temperature and Maltodextrin Content. Int. J. Food Sci. Technol. 2012, 47(1), 167–176. DOI: 10.1111/j.1365-2621.2011.02823.x.
- Nayak, C. A.; Rastogi, N. K. Effect of Selected Additives on Microencapsulation of Anthocyanin by Spray Drying. Drying Technol. 2010, 28(12), 1396–1404. DOI: 10.1080/07373937.2010.482705.
- Barbosa-Cánovas, G. V., and Juliano, P. Physical and Chemical Properties of Food Powders. In Encapsulated and Powdered Foods ed. Onwulata, C.; New York: CRC Press, 2005; pp 51–86.
- V Tonon, R.; Brabet, C.; Hubinger, M. D. Influence of Process Conditions on the Physicochemical Properties of Açai (Euterpe Oleraceae Mart.) Powder Produced by Spray Drying. J. Food Eng. 2008, 88(3), 411–418. DOI: 10.1016/j.jfoodeng.2008.02.029.
- Nguyen Q. D., Dang T. T., Nguyen T. V. L., Nguyen T. T. D., and Nguyen N. N. (2022). Microencapsulation of roselle (Hibiscus sabdariffa L.) anthocyanins: Effects of drying conditions on some physicochemical properties and antioxidant activities of spray‐dried powder. Food Science & Nutrition, 10(1), 191–203. 10.1002/fsn3.2659