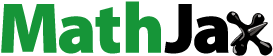
ABSTRACT
Niangpi (cold skin noodles) with northwestern characteristics is one of the traditional foods in China. To promote the development of traditional food, the current study was designed to improve the formulation and production process of Niangpi. A single factor, Box-Behnken design of response surface methodology (RSM) was employed to investigate the appropriate amount of polysaccharides (including potato starch, konjac flour, and sodium alginate) and their influence on instrumental texture profile analysis (TPA) and sensory evaluation of Niangpi. The effect of different wobbling times (0s, 70s, 140s, 220s) on sensory evaluation, microstructure, face rate, and TPA of Niangpi was explored. The optimum level of potato starch, konjac flour, and sodium alginate determined by the single factor test and RSM was 1.0, 0.15, and 0.03% and 1.24, 0.16, and 0.03%, respectively. The quality of Niangpi was the best at 220 s wobbling time. The wobbling time increased the pore structure and hierarchy of Niangpi, and presented a strongly positive correlation with the hardness, gumminess, and a strongly negative correlation with adhesiveness, chewiness, and cohesiveness. The obtained results revealed that both the optimized formulation and production process not only simplified the preparation but also improved the quality and sensory characteristics of Niangpi.
Introduction
“Niangpi” is a special kind of noodles. Compared with spaghetti and wheat noodles, it tastes waxy and is more suitable for the elderly and children.[Citation1] Niangpi has always been handmade without industrialized food processing, and the handicraft production steps are complex. It is necessary to wash most of the protein in wheat flour,[Citation2] and then precipitate and discharge a large amount of supernatant wastewater in 8–12 h to improve the starch concentration. It has a high microbial risk and short shelf life, which is not conducive to promotion.[Citation3] Therefore, it is necessary to replace the traditional manual workshop processing through modern processing technology.
There are few research data on the optimal raw material ratio and production technology of Niangpi. The mixing of starch and wheat flour failed to achieve a certain gel structure. Furthermore, several studies have reported that the addition of polysaccharides could be used to modify and control the texture properties and to enhance the overall quality of food products.[Citation4–6] Polysaccharides such as potato starch (PS), konjac flour (KF) and sodium alginate (SA) are frequently used in starch-based food products because they enable the modification and control of physical properties. Potato starch, as a highly branched amylopectin, forms a semi-transparent gel with a certain intensity and elasticity after gelatinization, which affects the texture of flour products.[Citation7,Citation8] It has the largest granules (25–100 μm), highest peak and breakdown viscosity, and lowest pasting temperature of all starches,[Citation9] and is thus the preferred starch in most industrial food applications. Konjac flour is a hydrophilic complex polysaccharide, widely used as a food additive and dietary supplement. It has been authorized in Europe and classified as generally recognized as safe (GRAS) by the Food and Drug Administration (FDA).[Citation10] Konjac flour exhibits the characteristics of strong water absorption and high sol viscosity.[Citation11] In particular, under suitable alkaline conditions, a stable gel can be formed with sol to produce certain effects on the texture of flour products.[Citation12] As a hydrophilic macromolecule, sodium alginate can not only promote the swelling of starch, but can also enhance the consistency of starch paste, and promote the formation of viscoelastic gel, thus improving the stability of starch paste and exerting a certain effect on texture.[Citation13,Citation14] It has also been found that polyelectrolytes such as sodium alginate can improve the water binding ability of dough by binding with glutenin protein,[Citation15] thereby increasing the matrix continuity of noodles and contributing to a more compact structure, which has a certain impact on texture.[Citation16] The gel structure of different polysaccharides has a very important effect on the taste.[Citation17–19] The wobble process may be an important factor in the gel forming process. The liquid in general evaporation process is static in the horizontal direction, and the gel can be formed in layers due to heat by wobbling process, but such studies are rarely reported.
In this study, wheat flour and wheat starch were used as raw materials, and the effect of mechanized production of Niangpi was evaluated by adding polysaccharide and wobbling process. It not only omitted the gluten washing process but also standardized the proportion of starch and other raw components. This work aimed to retain the traditional flavor of Niangpi, while simplifying the process and improving the quality of its production. The formulation of Niangpi was optimized via the addition of different proportions of potato starch, konjac flour and sodium alginate, and subsequent observation of their effects on texture profile analysis (TPA) and sensory attributes. Optimal levels were evaluated using a single-factor Box-Behnken design with response surface methodology (RSM). Furthermore, the effects of different wobbling processing time variabilities on TPA and microstructure were observed, with Pearson’s correlation analysis applied to assess the correlation between the parameters and wobbling time. The findings of this systematic investigation of the microstructure and texture of Niangpi provide better understanding of the influences of auxiliary materials and steaming processes on the quality of this traditional food product.
Materials and methods
Materials and reagents
Wheat flour (protein 10.5% and water 8.9%) and wheat starch (water 8.5%) were purchased from Xinxiang Liangrun Whole Grain Food Co., Ltd.; potato starch was obtained from Beijing Gusong Economy and Trade Co., Ltd.; sodium alginate and konjac flour were supplied by Shanghai Luan Biological Technology Co., Ltd.; edible soda ash was purchased from Nanjing Ganzhiyuan Sugar Co., Ltd.; and edible salt was provided by Zhongyan Shanghai Salt Industry Co., Ltd.
Preparation of Niangpi
Niangpi was produced for this study using basic ingredients, wheat flour (20 g) and wheat starch (50 g) (YP20002B electronic balance). The process by which Niangpi is made is shown in . First, the above mentioned basic ingredients were mixed homogeneously with the addition of different proportions of potato starch (0.5, 1.0, 1.5 and 2.0% w/w), konjac flour (0.05, 0.10, 0.15, 0.20, 0.25 and 0.30% w/w) and sodium alginate (0.01, 0.02, 0.03, 0.04 and 0.06% w/w), after which it was blended in the multifunctional cooking machine (TM5-1 electric food processor) to ensure uniformity (at 37°C for 15 min). The resultant paste was then poured into a flat pan (diameter 21 cm), brushed with oil, and placed into a pot with a cage drawer, where it was wobbled at an oscillation frequency of 0.25 Hz until it was evenly solidified. Finally, the pot was covered, and the product was steamed for a certain length of time (C21-WH2106 multi-function induction cooker), then cooled and removed from the mold. Additional samples were wobbled for different time intervals including 0 s (control group), 70s, 140s and 220s, and the effects of wobbling on quality parameters (microstructure and TPA) were investigated. Traditional Niangpi processing does not involve wobbling, and the batter emulsion is heated directly in a stable container for gel maturation. The wobbling process causes the batter emulsion to roll back and forth inside the container and to gelatinize in layers from the bottom up, depending on the speed of the vibration frequency.
Response surface design
The response surface design was carried out using Minitab 17 statistical software. Based on the single factor test, a Box-Behnken experimental design with three variables and three levels was implemented. Taking sensory evaluation as the response value, potato starch (0.5, 1.0 and 1.5%), konjac flour (0.05, 0.15 and 0.25%) and sodium alginate (0.01, 0.03 and 0.05%) were added as the main independent variables, expressed as A, B and C, respectively, with – 1, 0 and 1 representing the low, medium, and high levels, respectively, of the independent variables. The design and levels are shown in .[Citation20,Citation21] The zero-point experiment was repeated five times to estimate the experimental error. The experiment sequence was carried out in a random order generated by the computer, to avoid the influence of external factors on the experimental results.
Table 1. Design of Box-Behnken experiments.
Instrumental texture profile analysis
Texture profile analysis (TPA) is an important, simple and suitable test for the evaluation of food texture, providing a variety of textural characteristics via a single test. TPA can be used to examine the textural properties of flour products.[Citation22] In this study, texture analysis experiments were performed in terms of hardness, gumminess, springiness, adhesiveness, chewiness, and cohesiveness of the uniform demolded Niangpi, using the Brookfield CT310K texture analyzer. Tests were carried out using two different probes, namely a cylindrical probe (diameter 12.7 mm, TA10) and a light knife probe (TA7, 60 mm length, 30 mm height, 3 mm thickness).[Citation23,Citation24] Testing parameters were set as follows: pretest, test, and posttest speeds of 1.00 mm/s; shape variable was 50%; induction force was 50 g; interval time was 5 s. The tests were repeated 12 times for each sample. According to the data selection requirements, data with large differences were removed, and the average value was taken for further evaluation.
Sensory evaluation
Sensory evaluation can reflect the overall characteristics of samples and is an important parameter to ensure product quality for consumer acceptance. In order to test the acceptability of Niangpi, a sensory evaluation was carried out according to .[Citation25,Citation26] The sensory evaluation panel comprised eight trained laboratory members (half male and half female). The panel members had an experience of sensory evaluation for approximately three years, and they were habitual of eating noodles. The new samples were cooled for 20 min and then stored in an airtight bag at 4°C. Sensory evaluation was performed after 1 h at room temperature. The team members were asked to evaluate the color, texture, springiness, adhesiveness, stretchability, and taste of the samples. The final scores for different events are the average of the 8 scores and graphically represented. Evaluators were asked to gargle each sample before testing it.
Table 2. Criteria for sensory evaluation of Niangpi.
Microstructure analysis
The Niangpi samples (wobbled for different time periods of 0s, 70s, 140s and 220s) were cut into pieces and frozen for 48 h at −40°C. The frozen samples were then placed into a vacuum refrigerator for freeze-drying. Treated samples were fixed on the sample short column with the cross-section upward and sprayed gold. Finally, the microstructure of the cross-section of the sample was observed via a Zeiss Gemini 300 scanning electron microscope (SEM, Carl Zeiss, Jena, Germany) at 500x magnification (accelerating voltage 1.5kV and signal source secondary electron).
Data processing and statistical analysis
Origin 2021, Minitab (version 17) and Image Pro Plus 6.0 were used for data and face rate analysis (face rate = [pore area/(pore area+solid area)]%). The level of significance and Pearson’s correlation of the data were analyzed using SPSS Pro 2021 (where p< .01 and p < .05 indicated extremely significant and significant, respectively).
Results and discussion
Single factor test results
Effects of potato starch addition on sensory evaluation and texture analysis
According to the significance of change noted in the texture and sensory evaluation, additions of 0.5–1.5% potato starch were selected for subsequent optimization experiments. As shown in ) and Table S1, when the added amount of potato starch was 1%, the Niangpi achieved the relatively highest scores (95.32) in the sensory evaluation, however, increases in the quantity of potato starch (1.5 and 2.0%) added in the production of the Niangpi had negative effects on its sensory scores, which decreased to 90.76 and 78.58, respectively. The texture of the Niangpi changed significantly after the addition of different quantities of potato starch, as shown in . The texture parameters presented in ) were measured with a cylindrical probe, and it is evident that compared with the control, the hardness and gumminess of Niangpi decreased significantly after adding potato starch. Overall, the addition of different proportions of potato starch influenced hardness, gumminess, springiness and adhesiveness. The texture parameters in ) were measured by a light knife cutting probe. Compared with the control, it was found that the addition of potato starch significantly increased hardness and chewiness while the cohesiveness was not significantly influenced. Overall, the addition of different proportions of potato starch had a great influence on hardness and chewiness, but only slightly influenced cohesiveness.
Figure 2. Sensory profile analysis of Niangpi various proportions of potato starch (PS), konjac flour (KF), sodium alginate (SA) and wobbling times (WT).
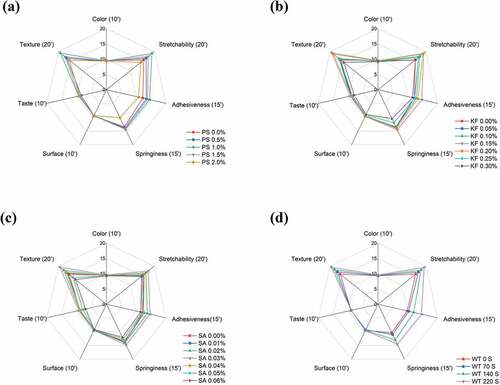
Figure 3. Effects of potato starch on textural characteristics of Niangpi.
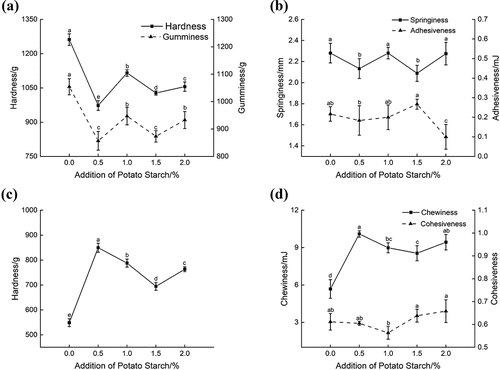
This may be because when starch molecules are heated in excess water, their microcrystalline structure is destroyed, and the exposed hydroxyl groups of direct starch and amylopectin are linked with water molecules in the form of hydrogen bond, which leads to the increase of water absorption, expansion and solubility of starch.[Citation27] It was found that with the addition of high expansion starch, the smoothness of noodles increased and the hardness decreased.[Citation28] This may be due to the high content of phosphate group in potato starch molecules. The hydrophilicity of the phosphate group is greater than that of the hydroxyl group in the molecule, which makes the starch easy to absorb water and expand, resulting in the destruction of crystal structure and turning into viscous paste.[Citation17] When water is added to flour, the gluten will be hydrated to form protein network structure.[Citation29] The presence of salt enhances the aggregation of gluten protein and induces the changes in gluten molecular conformation and network structure. In addition, it can also reduce the content of free sulfhydryl,[Citation30] which affects the physicochemical properties of Niangpi. The findings in this study show that the addition of potato starch can improve the texture of Niangpi, with the results of the sensory and texture analysis indicating that the optimum addition of potato starch was 1%.
Effects of konjac flour on sensory evaluation and texture analysis
According to the significant changes in texture and sensory evaluation, quantities of 0.05–0.25% konjac flour were selected for subsequent optimization experiments. As shown in ) and Table S1, sensory evaluation scores increased at first and then decreased with increasing amounts of konjac flour. The sample containing 0.15% konjac flour obtained the highest score (96.20), and scores decreased gradually in samples with increasing levels (0.20–0.30%) of konjac flour. This may be attributed to the qualities of good water absorption, water retention and fishy smell with which the konjac flour affected the taste of the Niangpi.
The effects of the addition of konjac powder on Niangpi texture parameters are shown in . ) show the texture parameters after measurement with a cylindrical probe. Compared with the control, the addition of konjac flour was found to decrease the hardness and adhesiveness of Niangpi. The addition of various amounts of konjac flour was seen to greatly affect the hardness and gumminess of the Niangpi, while only slightly influencing the springiness and adhesiveness. ) show the texture parameters measured by light knife probe. Compared with the control, hardness and chewiness significantly increased while cohesiveness was significantly decreased with the addition of konjac flour. This may be related to the gelatinization of starch and the expansion of the gluten network.[Citation31] Polysaccharides can compete with starch for water absorption, change the gelatinization viscosity of starch, delay the regeneration of starch, and significantly change the physicochemical properties of starch.[Citation19,Citation32] Konjac flour can enhance the hydration of the protein starch matrix and enhance the continuity of the gluten network.[Citation18] In addition, konjac flour can also form a network structure under alkaline conditions, which affects the texture of Niangpi.[Citation33] Experiments show that adding konjac flour to wheat noodles has a positive effect on the texture of noodles.[Citation34] Therefore, the addition of konjac powder can improve the texture and rheology of products, maintain moisture, and play a good role in controlling the stability and quality of food.[Citation35] In this study, it was concluded from the results of the sensory and texture analysis of the Niangpi that the optimal addition of konjac flour was 0.15%.
Figure 4. Effect of konjac flour on texture profile analysis of Niangpi.
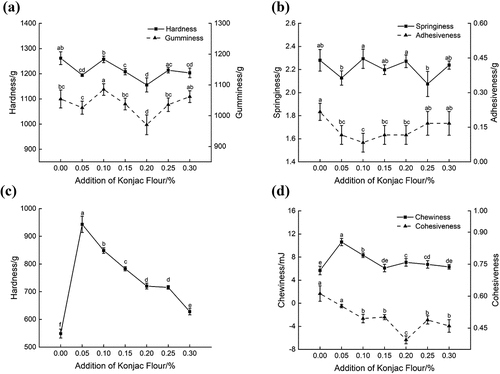
Effect of sodium alginate on sensory evaluation and texture analysis
According to the significant changes in the texture and sensory evaluation, the addition of sodium alginate quantities ranging from 0.01–0.05% were selected for subsequent optimization experiments. The sample containing 0.03% sodium alginate was preferred by the sensory panelists and obtained the highest score of 94.73 () and Table S1). However, increasing amounts of sodium alginate (0.04–0.06%) had a negative effect on sensory scores. Furthermore, it can be seen from , that the addition of various amounts of sodium alginate had different impacts on the texture parameters of the Niangpi. ) show texture parameters when measured with a cylindrical probe. Compared with the control, the hardness, gumminess, springiness, and adhesiveness of the Niangpi were significantly decreased after sodium alginate was added. The addition of various amounts of sodium alginate was seen to greatly affect the hardness, gumminess, and springiness of the Niangpi, but no significant difference was found in the adhesiveness. Hardness was increased by adding 0.01 to 0.03% sodium alginate and then observed to be decreased. A slight increase in springiness was found by addition of 0.02–0.03% sodium alginate, however, springiness was declined by rising amount of sodium alginate. The texture parameters displayed in ) were measured via the light knife probe. Compared with control, hardness was found to be significantly increased with the addition of sodium alginate. The chewiness was significantly increased in the early while no significant difference when the dosage was increasing. With the increase of sodium alginate, the cohesiveness gradually decreased, with only one exception.
Figure 5. Effects of sodium alginate on texture of Niangpi.
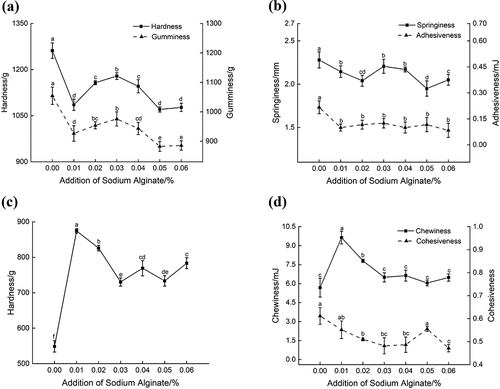
The reasons for the above texture changes are as follows: on the one hand, the -OH and -COO groups in the molecular chain of sodium alginate interact with the -OH group of starch,[Citation36] which affects the interaction between amylose molecules and the recrystallization of amylopectin.[Citation19] On the other hand, these changes may be related to sodium alginate improving the strength, water holding capacity and viscosity of gluten protein network structure.[Citation37] In addition, there are a large number of carboxyl and hydroxyl groups on the molecular chains of alginate, which can be cross-linked with two valent calcium ions to form a three-dimensional network gel, while other substances can be adsorbed by physical action, thereby improving the texture properties of products.[Citation38,Citation39] Yu, Z et al.[Citation40,Citation41] also measured by the cylindrical probe and found that the apparent hardness of starch samples decreased after adding sodium alginate. However, alginate changed the internal network structure of Niangpi, which led to an increase in its hardness when it was measured with a light knife cutting probe. Overall, the results of the sensory evaluation and texture analysis of the Niangpi in this study showed that the optimal additional amount of sodium alginate was 0.03%.
Response surface test design results
Establishment of regression model and analysis of variance (ANOVA)
Regarding experimental design, a Box-Behnken experimental design applied for 3 variables was used to optimize the formulation of the Niangpi and to determine the combined effect of the potato starch (A), konjac flour (B) and sodium alginate (C) on the sensory evaluations (Y). A response surface design with three factors and three levels was adopted, and a total of 17 test results were obtained (). A second-order quadratic polynomial equation of the following form was fitted to the data of all responses for prediction. The proposed model was as follows:
Table 3. Box-Behnken test design and results of sensory evaluation.
The ANOVA results are shown in Table S2. The regression model was found to be highly significant (p < .01), while the lack of fit was not significant (p > .05), indicating that the regression model had a good fit with the actual test and that the relationships between independent variables and response values were significant. The order in which the three single factors influenced the sensory evaluation scores of Niangpi was A > B > C, demonstrating that potato starch had the most significant effect on the sensory evaluation. The significance level of an independent variable is related to the F value, and the greater the F value, the more significant the influence. The potato starch (A), konjac flour (B) and sodium alginate (C) had significant (p < .05) quadratic effects, while only the interactions between konjac flour (B) and sodium alginate (C) had highly significant (p < .01) effects. For the sensory score, second-order models were fitted and high coefficients of determination (R2) were observed, indicating that the reliability of the regression model was high. Hence, this model can be used to effectively analyze and predict the processing technology of Niangpi.
Data analysis of response surfaces
According to the three-dimensional response surfaces shown in , the sensory evaluation scores of the Niangpi increased initially after the additions of various quantities of potato starch, konjac flour and sodium alginate, but subsequently decreased gradually as these additions were increased. The optimal values for the potato starch, konjac flour and sodium alginate additions were obtained via the formation of interactions. Analysis of the contour map showed that the contour of the additional amounts of the three independent variables was oval, indicating that there was a significant interaction between them. The scores of sensory attributes increased with the addition of all polysaccharides because the wheat starch characteristic was changed with the addition of polysaccharides during gelatinization, thus improving the properties of the Niangpi.[Citation42] However, higher concentrations of polysaccharides may reduce the characteristics of flour products because their high hydrophilicity competes with wheat protein and starch for water, thus interfering with gluten network formation.[Citation43]
Validation of response surface test model
The following optimal process parameters for Niangpi were obtained from the Box-Behnken design analysis and subsequent data processing: The optimal additions of potato starch, konjac flour and sodium alginate were 1.24, 0.16, and 0.03%, respectively. Under these conditions, the sensory score of the Niangpi was 95.86, the theoretical value was 96.2305, and the relative deviation between the theoretical value and the experimental value was 0.3705%. The accuracy and effectiveness of this model were verified.
Effects of different wobbling times on quality parameters of Niangpi
Microstructure: Cross-sections of freeze-dried Niangpi were examined using scanning electron microscopy (SEM), as shown in . The Niangpi samples prepared at different wobbling times exhibited considerable differences in their three-dimensional mesh structures. Comparative analysis of the microstructures of the samples with wobbling ()) and without wobbling (control) ()), revealed that the pore structures of the control sample were small and disordered, while the overall structure was compact. In contrast, the wobbling samples had large porous structures and a continuously flaking wall layer, presenting a certain sense of hierarchy. Furthermore, comparisons of the samples with different wobbling times ()) showed that longer wobbling resulted in the largest porous structures and a more obvious sense of hierarchy. At the same time, by combining the face rate of different samples (), it was evident that the pore structures of samples were intensified with prolonged wobbling and, likewise, longer wobbling time resulted in a greater face rate. This may be because the wobbling process increased the time and area of which the thin paste was in contact with steam. Consequently, the crystalline area in the starch particles absorbed a large amount of water and formed an interconnected and winding network of aqueous colloids,[Citation44] thus enabling full gelatinization and the absorption of a large amount of water before it was freeze-dried. At the same time, the wobbling process reduced the weight borne by the bottom layer of the sample, making the structure of the Niangpi looser and causing the taste to become softer and waxier. In addition, the wobbling process causes the thin paste to solidify in even layers under the action of steam, providing the structure of the Niangpi with a certain sense of hierarchy and improving the taste of Niangpi on the basis of its soft, waxy mouthfeel.
Figure 7. Microstructure of Niangpi fabricated at different wobbling times.
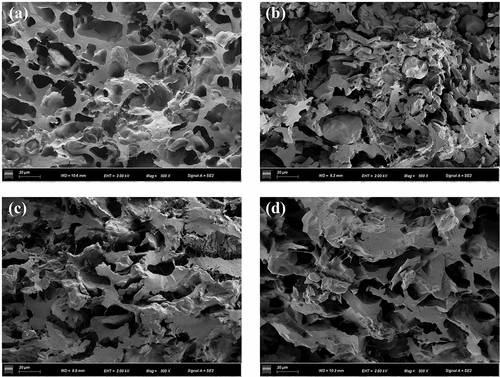
Figure 8. Surface area ratios of microstructure at different wobbling times.
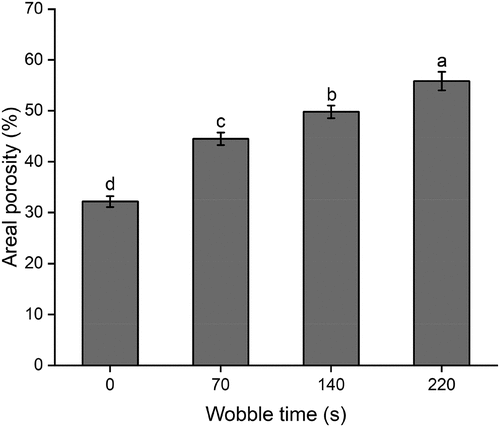
Instrumental Texture analysis: As shown in ) and Table S1, when the wobbling time is 220s, the Niangpi achieved the relatively highest scores (96.1) in the sensory evaluation. shows the effects of different wobbling times on Niangpi texture parameters. In the texture parameters in ), measured using a cylindrical probe, with the change of wobbling times, differences were observed among the hardness, gumminess, springiness and adhesiveness. After wobbling for 70s, gumminess and adhesiveness changed significantly, while wobbling for 140s, hardness and springiness became to change significantly. Pearson’s correlation analysis (Table S3) showed a strong positive correlation between the wobbling time, hardness (0.877, p < .01), and gumminess (0.795, p < .01), however, a strongly negative correlation was noticed between the wobbling time and adhesiveness (−0.670, p < .01). The texture parameters in ) were measured using a light knife cutting probe, with the change of wobbling times, differences were observed among the hardness, chewiness and cohesiveness. After wobbling for 70s hardness changed significantly while wobbling for 220s, cohesiveness and chewiness started to change significantly. Furthermore, correlation analysis indicated a strongly negative correlation between the wobbling time and chewiness (−0.670, p < .01), and cohesiveness (−0.725, p < .01). This might be related to the migration of water molecules. In addition, the moisture on the surface of Niangpi evaporates faster with prolongation of wobbling time, as the external migration of water is greater than its internal migration, which is why the surface hardness of the Niangpi increased with the longer wobbling times.[Citation44] Moreover, with the increase in wobbling time, the contact between starch and water vapor was extended, resulting in a large number of water molecules that entered the starch microcrystalline gaps, causing the expansion of starch particles. The speed of outward migration of water was less than the speed of inward migration and, thus, the gumminess, adhesiveness, chewiness and cohesiveness of Niangpi are significantly related to wobbling time.[Citation45] In addition, wobbling may have increased the pore structure and sense of hierarchy of the samples, which greatly influenced the texture of the Niangpi. The optimal wobbling time for Niangpi was found to be 220 s on the basis of microstructure, sensory, and texture analysis results of Niangpi.
Figure 9. Effects of wobbling times on texture of Niangpi.
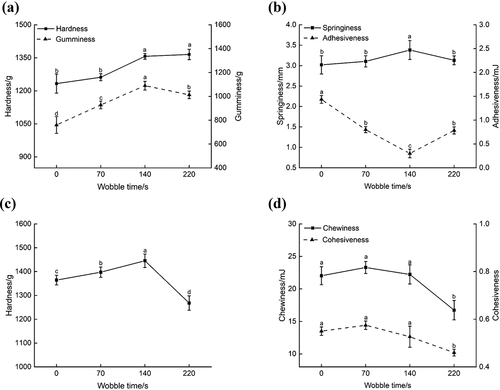
Conclusion
“Niangpi” is a special kind of noodles, and there is little research on its formula and production technology. In order to establish its industrial processing method, this paper carried out research on the formula and key technology. On the one hand, the optimal addition amount was determined by a single factor test and RSM. Through experimental verification, it is found that the predicted response value is consistent with the model. On the other hand, the quality of Niangpi was further improved by adding wobbling technology. The sensory score, pore structure, layering and specific volume of Niangpi increase with the increase of wobbling time, which plays a particularly important role in simulating handicraft production. Finally, it was found that when the contents of potato starch, konjac flour and sodium alginate were 1.24%, 0.16%, and 0.03% respectively and the wobbling time was 220 s, the quality of Niangpi reached the best and the taste of hand-made Niangpi was high fidelity. However, this study needs to further determine the interaction between polysaccharides (potato starch, konjac flour, sodium alginate) and the wobbling process, which can be explored by confocal light scanning microscopy (CLSM), differential scanning calorimetry (DSC) and rheometer.
Disclosure statement
No potential conflict of interest was reported by the author(s).
Correction Statement
This article has been corrected with minor changes. These changes do not impact the academic content of the article.
Additional information
Funding
References
- Zeng, J.; Gao, H. Y.; Li, G. L. Functional Properties of Wheat Starch with Different Particle Size Distribution. J. Sci. Food Agric. 2014, 94(1), 57–62. DOI: 10.1002/jsfa.6186.
- Zheng, S. S.; Yang, Y.; Li, Z.; Pan, Z. L.; Huang, Z. M.; Ai, Z. L. A Comparative Study of Different Freezing Methods on Water Distribution, Retrogradation, and Digestion Properties of Liangpi (Starch Gel Food). Starch-Starke. 2022, 74(3–4), 2100205. DOI: 10.1002/star.202100205.
- Liu, S. C.; Xiao, Y. H.; Shen, M. Y.; Zhang, X. W.; Wang, W. J.; Xie, J. H. Effect of Sodium Carbonate on the Gelation, Rheology, Texture and Structural Properties of Maize Starch-Mesona Chinensis Polysaccharide Gel. Food Hydrocolloids. 2019, 87, 943–951. DOI: 10.1016/j.foodhyd.2018.09.025.
- Chen, Z.; Schols, H. A.; Voragen, A. G. J. The Use of Potato and Sweet Potato Starches Affects White Salted Noodle Quality. J. Food Sci. 2003, 68(9), 2630–2637. DOI: 10.1111/j.1365-2621.2003.tb05781.x.
- Whitney, K.; Simsek, S. Potato Flour as a Functional Ingredient in Bread: Evaluation of Bread Quality and Starch Characteristics. Int. J. Food Sci. Technol. 2020, 55(12), 3639–3649. DOI: 10.1111/ijfs.14698.
- Zaidul, I. S. M.; Yamauchi, H.; Matsuura-Endo, C.; Takigawa, S.; Noda, T. Thermal Analysis of Mixtures of Wheat Flour and Potato Starches. Food Hydrocolloids. 2008, 22(4), 499–504. DOI: 10.1016/j.foodhyd.2007.01.003.
- Ma, M. T.; Liu, Y.; Chen, X. J.; Brennan, C.; Xu, X. M.; Sui, Z. Q.; Corke, H. Thermal and Pasting Properties and Digestibility of Blends of Potato and Rice Starches Differing in Amylose Content. Int. J. Biol. Macromol. 2020, 165, 321–332. DOI: 10.1016/j.ijbiomac.2020.09.189.
- Ortega-Ojeda, F. E.; Larsson, H.; Eliasson, A. C. Gel Formation in Mixtures of High Amylopectin Potato Starch and Potato Starch. Carbohydr. Polym. 2004, 56(4), 505–514. DOI: 10.1016/j.carbpol.2004.03.021.
- Wang, J.; Guo, K.; Fan, X. X.; Feng, G. N.; Wei, C. X. Physicochemical Properties of C-Type Starch from Root Tuber of Apios Fortunei in Comparison with Maize, Potato, and Pea Starches. Molecules. 2018, 23(9), 2132. DOI: 10.3390/molecules23092132.
- Jimenez-Colmenero, F.; Cofrades, S.; Herrero, A. M.; Solas, M. T.; Ruiz-Capillas, C. Konjac Gel for Use as Potential Fat Analogue for Healthier Meat Product Development: Effect of Chilled and Frozen Storage. Food Hydrocolloids. 2013, 30(1), 351–357. DOI: 10.1016/j.foodhyd.2012.06.015.
- Zhou, Y.; Zhao, D.; Winkworth-Smith, C. G.; Foster, T. J.; Nirasawa, S.; Tatsumi, E.; Cheng, Y. Q. Effect of A Small Amount of Sodium Carbonate on Konjac Glucomannan-induced Changes in Wheat Starch Gel. Carbohydr. Polym. 2015, 116, 182–188. DOI: 10.1016/j.carbpol.2014.02.087.
- Impaprasert, R.; Piyarat, S.; Sophontanakij, N.; Sakulnate, N.; Paengkanya, S.; Borompichaichartkul, C.; Srzednicki, G. Rehydration and Textural Properties of Dried Konjac Noodles: Effect of Alkaline and Some Gelling Agents. Horticulturae. 2017, 3(1), 20. DOI: 10.3390/horticulturae3010020.
- Pawar, S. N.; Edgar, K. J. Alginate Derivatization: A Review of Chemistry, Properties and Applications. Biomaterials. 2012, 33(11), 3279–3305. DOI: 10.1016/j.biomaterials.2012.01.007.
- Lee, S.; Bae, I. Y.; Jung, J. H.; Jang, K. I.; Kim, Y. W.; Lee, H. G. Physicochemical, Textural and Noodle-making Properties of Wheat Dough Containing Alginate. J. Texture Stud. 2008, 39(4), 393–404. DOI: 10.1111/j.1745-4603.2008.00149.x.
- Feng, Y. Y.; Mu, T. H.; Zhang, M.; Ma, M. M. Effects of Different Polysaccharides and Proteins on Dough Rheological Properties, Texture, Structure and in Vitro Starch Digestibility of Wet Sweet Potato Vermicelli. Int. J. Biol. Macromol. 2020, 148, 1–10. DOI: 10.1016/j.ijbiomac.2019.12.225.
- Lubowa, M.; Yeoh, S. Y.; Varastegan, B.; Easa, A. M. Effect of Pre-gelatinised High-amylose Maize Starch Combined with Ca2+-induced Setting of Alginate on the Physicochemical and Sensory Properties of Rice Flour Noodles. Int. J. Food Sci. Technol. 2021, 56(2), 1021–1029. DOI: 10.1111/ijfs.14754.
- Dupuis, J. H.; Liu, Q. Potato Starch: A Review of Physicochemical, Functional and Nutritional Properties. Am. J. Potato Res. 2019, 96(2), 127–138. DOI:10.1007/s12230-018-09696-2.
- Zhou, Y.; Cao, H.; Hou, M.; Nirasawa, S.; Tatsumi, E.; Foster, T. J.; Cheng, Y. Q. Effect of Konjac Glucomannan on Physical and Sensory Properties of Noodles Made from Low-protein Wheat Flour. Food Res. Int. 2013, 51(2), 879–885. DOI: 10.1016/j.foodres.2013.02.002.
- Tang, M. M.; Hong, Y.; Gu, Z. B.; Zhang, Y. Y.; Cai, X. R. The Effect of Xanthan on Short and Long-term Retrogradation of Rice Starch. Starch/Staerke. 2013, 65(7–8), 702–708. DOI: 10.1002/star.201200170.
- Strati, I. F.; Kostomitsopoulos, G.; Lytras, F.; Zoumpoulakis, P.; Proestos, C.; Sinanoglou, V. J. Optimization of Polyphenol Extraction from Allium Ampeloprasum Var. Porrum through Response Surface Methodology. Foods. 2018, 7(10), 162. DOI: 10.3390/foods7100162.
- Liu, X. L.; Mu, T. H.; Sun, H. N.; Zhang, M.; Chen, J. W.; Fauconnier, M. L. Effect of Ingredients on the Quality of Gluten-free Steamed Bread Based on Potato Flour. J. Food Sci. Technol. 2019, 56, 2863–2873. DOI: 10.1007/s13197-019-03730-9.
- Peng, B.; Li, Y. Q.; Ding, S. Y.; Yang, J. Characterization of Textural, Rheological, Thermal, Microstructural, and Water Mobility in Wheat Flour Dough and Bread Affected by Trehalose. Food Chem. 2017, 233, 369–377. DOI: 10.1016/j.foodchem.2017.04.108.
- Wang, L.; Hou, G. G.; Hsu, Y. H.; Zhou, L. R. Effect of Phosphate Salts on the Korean Non-fried Instant Noodle Quality. J. Cereal Sci. 2011, 54(3), 506–512. DOI: 10.1016/j.jcs.2011.09.008.
- Cham, S.; Suwannaporn, P. Effect of Hydrothermal Treatment of Rice Flour on Various Rice Noodles Quality. J. Cereal Sci. 2010, 51(3), 284–291. DOI: 10.1016/j.jcs.2010.01.002.
- Pal, G. K.; Kumar, S. B.; Prabhasankar, P.; Suresh, P. V. Inclusion of Poultry Based Food Ingredients in the Formulation of Noodles and Their Effects on Noodle Quality Characteristics. J. Food Meas. Charact. 2017, 11(3), 939–947. DOI: 10.1007/s11694-017-9467-8.
- Jafari, M.; Koocheki, A.; Milani, E. Physicochemical and Sensory Properties of Extruded Sorghum-wheat Composite Bread. J. Food Meas. Charact. 2018, 12(1), 370–377. DOI: 10.1007/s11694-017-9649-4.
- Singh, N.; Singh, J.; Kaur, L.; Singh Sodhi, N.; Singh Gill, B. Morphological, Thermal and Rheological Properties of Starches from Different Botanical Sources. Food Chem. 2003, 81(2), 219–231. DOI: 10.1016/S0308-8146(02)00416-8.
- Ross, A. S.; Quail, K. J.; Crosbie, G. B. Physicochemical Properties of Australian Flours Influencing the Texture of Yellow Alkaline Noodles. Cereal Chem. 1997, 74(6), 814–820. DOI: 10.1094/CCHEM.1997.74.6.814.
- Autio, K.; Kruus, K.; Knaapila, A.; Gerber, N.; Flander, L.; Buchert, J. Kinetics of Transglutaminase-Induced Cross-Linking of Wheat Proteins in Dough. J. Agric. Food Chem. 2005, 53(4), 1039–1045. DOI: 10.1021/jf0485032.
- Chen, G. J.; Ehmke, L.; Sharma, C.; Miller, R.; Faa, P.; Smith, G.; Li, Y. H. Physicochemical Properties and Gluten Structures of Hard Wheat Flour Doughs as Affected by Salt. Food Chem. 2019, 275, 569–576. DOI: 10.1016/j.foodchem.2018.07.157.
- Majzoobi, M.; Ostovan, R.; Farahnaky, A. Effects of Hydroxypropyl Cellulose on the Quality of Wheat Flour Spaghetti. J. Texture Stud. 2011, 42(1), 20–30. DOI: 10.1111/j.1745-4603.2010.00264.x.
- Chen, L.; Ren, F.; Zhang, Z. P.; Tong, Q. Y.; Rashed, M. M. A. Effect of Pullulan on the Short-term and Long-term Retrogradation of Rice Starch. Carbohydr. Polym. 2015, 115, 415–421. DOI: 10.1016/j.carbpol.2014.09.006.
- Tang, C. H.; Chen, L.; Foegeding, E. A. Mechanical and Water-holding Properties and Microstructures of Soy Protein Isolate Emulsion Gels Induced by CaCl2, Glucono-δ-lactone (GDL), and Transglutaminase: Influence of Thermal Treatments before And/or after Emulsification. J. Agric. Food Chem. 2011, 59(8), 4071–4077. DOI: 10.1021/jf104834m.
- Zhao, D.; Zhou, Y.; Liu, H. D.; Liang, J. F.; Cheng, Y. Q.; Nirasawa, S. Effects of Dough Mixing Time before Adding Konjac Glucomannan on the Quality of Noodles. J. Food Sci. Technol. 2017, 54(12), 3837–3846. DOI: 10.1007/s13197-017-2831-1.
- Varela, M. S.; Navarro, A. S.; Yamul, D. K. Effect of Hydrocolloids on the Properties of Wheat/Potato Starch Mixtures. Starch/Staerke. 2016, 68(7–8), 753–761. DOI: 10.1002/star.201400257.
- Siddaramaiah; Swamy, T. M. M.; Lee, J. H.; Lee, J. H.; Lee, J. H. Sodium Alginate and Its Blends with Starch: Thermal and Morphological Properties. J. Appl. Polym. Sci. 2008, 109(6), 4075–4081. DOI:10.1002/app.28625.
- Tabara, A.; Miyajima, C.; Moki, N.; Kasahara, F.; Seguchi, M. Improvement of Bread Making Properties by the Addition of Alginates. Food Sci. Technol. Res. 2016, 22(1), 145–151. DOI: 10.3136/fstr.22.145.
- Liu, H. Y.; Yang, Z. Y.; Zhang, Q.; Dai, Z. Y.; Fan, S. G.; Liu, R. R. Effect of Sodium Alginate on the Quality of Frozen Noodles. Cereal Feed Ind. 2017, 8, 20–22. DOI: 10.7633/j.1003-6202.2017.08.005.
- Zhang, Y. W.; Chen, W. G.; Zhang, J. Research and Develop of Nutritious Noodles with Meat Flour of Giant Salamander. Cereal Feed Ind. 2019, 8, 23–25. DOI: 10.7633/j.1003-6202.2019.08.007.
- Yu, Z.; Wang, Y. S.; Chen, H. H.; Li, Q. Q.; Wang, Q. The Gelatinization and Retrogradation Properties of Wheat Starch with the Addition of Stearic Acid and Sodium Alginate. Food Hydrocolloids. 2018, 81, 77–86. DOI: 10.1016/j.foodhyd.2018.02.041.
- Li, Q. Q.; Wang, Y. S.; Chen, H. H.; Liu, S.; Li, M. Retardant Effect of Sodium Alginate on the Retrogradation Properties of Normal Cornstarch and Anti-retrogradation Mechanism. Food Hydrocolloids. 2017, 69, 1–9. DOI: 10.1016/j.foodhyd.2017.01.016.
- Amritpal, K.; Khetan, S.; Narpinder, S.; Parul, S.; Seeratpreet, K. Effect of Guar Gum and Xanthan Gum on Pasting and Noodle-making Properties of Potato, Corn and Mung Bean Starches. J. Food Sci. Technol. 2015, 52(12), 8113–8121. DOI: 10.1007/s13197-015-1954-5.
- Li, J. X.; Zhu, Y. P.; Yadav, M. P.; Li, J. L. Effect of Various Hydrocolloids on the Physical and Fermentation Properties of Dough. Food Chem. 2019, 271, 165–173. DOI: 10.1016/j.foodchem.2018.07.192.
- Chen, X.; Zhang, X. X.; Wang, B. Y.; Chen, P. R.; Xu, Y.; Du, X. F. Investigation of Water Migration and Its Impacts on Eating Qualities of Black Rice during Cooking Process. J. Cereal Sci. 2019, 89, 102810. DOI: 10.1016/j.jcs.2019.102810.
- De Bondt, Y.; Liberloo, I.; Roye, C.; Goos, P.; Courtin, C. M. The Impact of Wheat (Triticum Aestivum L.) Bran on Wheat Starch Gelatinization: A Differential Scanning Calorimetry Study. Carbohydr. Polym. 2020, 241, 10. DOI: 10.1016/j.carbpol.2020.116262.