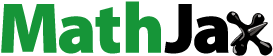
ABSTRACT
The aim of this study was to investigate the main volatiles and polyphenolic compounds in hydrosols extracted from three Romanian Lamiaceae plants, sage (Salvia officinalis), rosemary (Rosmarinus officinalis) and lavender (Lavandula angustifolia) by steam distillation. The antioxidant and acetylcholinesterase inhibition capacity of hydrosols were assessed for their further use as biopesticides. The results have shown that sage and rosemary hydrosols presented better antioxidant activity than lavender hydrosol in all free radical scavenging and metal chelating assays. A strong relationship between the antioxidant activity of hydrosols’ and their total phenolic and flavonoid content was found based on Pearson correlation coefficients. Sage and rosemary hydrosols exerted higher acetylcholinesterase inhibition than lavender hydrosol, but this activity was moderate correlated to phenolic content and low correlated to flavonoid content. These results suggested that volatiles and other compounds present in hydrosols were also involved in this process. In conclusion, Lamiaceae plant hydrosols could be further tested, as such or mixed, as natural products for the control of pests in eco-agrosystems.
Introduction
Hydrosols or hydrolates are aqueous solutions obtained as by-products at the end of herbs and spices distillation, after essential oil separation.[Citation1] Hydrosols contain the aqueous fraction of volatile compounds and polyphenols with bioactive properties.[Citation2] The volatiles composition and total phenolics content (TPC) was previously studied in various aromatic plant hydrosols, but information regarding their polyphenolic compounds composition was scarce and limited to few characteristic ones. Several studies have shown that hydrosols were used in products for aromatherapy, cosmetics, cakes, and drinks.[Citation3,Citation4] Moreover, hydrosols extracted from aromatic plant species, such as Mexican oregano (Poliomintha longiflora),[Citation5] Polish lavender (Lavandula augustifolia),[Citation6] Morrocan and Colombian thyme (Thymus vulgaris),[Citation4,Citation7] belonging to Lamiaceae family, Algerian marigold (Calendula arvensis) from Asteraceae family[Citation8] and bitter orange flowers (Citrus aurantium) from Rutaceae family[Citation9] have demonstrated antioxidant, antibacterial, and antifungal activity, indicating their potential for biomedical formulations.
In recent times, the pesticide and repellent activity of hydrosols against aphids, mites, and beetles gained more attention. Thus, sweet basil (Ocimum basilicum) and fringed rue (Ruta chalepensis) hydrosols have inhibited the reproduction and negatively affected the survival of Aphis gossypii aphid and Tetranychus urticae mite, as shown by leaf disk assay.[Citation10] Lavender (Lavandula intermedia) hydrosol had a repellent effect on Tribolium confusum beetle, but only when used at 1000 times higher concentration than that of MR-08, a synthetic compound.[Citation11] Hydrosols extracted from two mint species (Mentha pulegium, Mentha suaveolens) have shown a dose-dependent pesticide activity on Toxoptera aurantii aphid in Petri biotests.[Citation12] Hydrosols of marjoram (Origanum majorana) and pennyroyal (Mentha pulegium) have induced mortality of Myzus persicae aphids, as determined by leaf disk assay, after 24 h exposure.[Citation13]
Studies on individual bioactive compounds isolated from different plant extracts and essential oils, such as trans-cinnamic acid from Alpinia galanga, the isoflavone rotenone from Derris elliptica, the terpenoids azadirahtin from neem oil and pyrethrin from Chrysanthemum cinerariifolium, and the alkaloid veratrine revealed their activity toward the nervous system functioning, weight reduction, behavior change, and repellent effect against various insects.[Citation14–16] Other phytochemicals, also present in hydrosols, for example, terpenes, phenols, and alkaloids had the capacity to control redox and neurological processes, acting as natural pesticides.[Citation17] In addition, they were able to limit the development of a wide range of plant pathogens and acted as natural food sanitizers, allowing the preservation of fresh fruit and vegetables quality during storage.[Citation7–9,Citation18,Citation19]
One of the important targets used for the development of new pesticides was represented by acetylcholinesterase (AChE), a key enzyme of the pests’central nervous system. Plant essential oils and their derived compounds, like monoterpenes and sesquiterpenes, have exerted AChE inhibition.[Citation20] They could bind to specific amino acids from the enzyme’s active site, causing inhibition of its activity, or to other sites when allosterically altered the enzyme’s functionality.[Citation21] These biochemical processes result in hyperactivity of the nervous system, leading to alteration of the normal physiological processes in pests and affecting their larval growth, development, reproduction, feeding, or even their survival.[Citation15] To our knowledge, there are no studies focused on the in vitro inhibition of AChE in the presence of hydrosols.
In this context, the aim of this study was to investigate the chemical composition of hydrosols obtained by steam distillation of three Romanian Lamiaceae species, sage (S. officinalis), rosemary (R. officinalis) and lavender (L. angustifolia), in order to identify the main volatile and phenolic compounds using gas chromatography coupled with mass spectrometry (GC-MS) and high performance liquid chromatography (HPLC) techniques, respectively. Special emphasis was given to the determination of the in vitro antioxidant and AChE inhibition activity of hydrosols, and the relationship to their polyphenolic content based on the correlation coefficient analysis, targeting further use in novel biopesticide formulations.
Materials and methods
Materials
Sage (S. officinalis), rosemary (R. officinalis) and lavender (L. angustifolia) were harvested from ecologic cultures of Hofigal Export-Import and their aerial parts were air-dried at 25°C to constant weight and powdered. The reagents 2,2’-azino-bis(3-ethylbenzothiazoline-6-sulfonic acid) (ABTS), 2,2-diphenyl-1-picrylhydrazyl (DPPH), AChE, 5,5’-dithiobis(2-nitrobenzoic acid) (DTNB) were purchased from Sigma-Aldrich (Germany). All other reagents were of analytical purity and purchased from Sigma-Aldrich (Germany), unless otherwise specified.
Plant hydrosols preparation
Powdered plant material (25 g) was placed in a round-bottomed flask, moistened in ultrapure water and extracted by steam distillation in ultrapure water (750 mL), at 100°C, for 2 h using a Clevenger-type extraction apparatus (J.P. Selecta, Spain). The essential oil fraction was separated from the aqueous fraction representing the hydrosol, based on their density. The hydrosols were filtered and stored in the dark, at 4°C, until analysis.
GC-MS analysis
The analysis was performed on a Trace 1310 gas chromatograph, fitted with a capillary column (TG-WAXMS, 30 m × 0.25 i.d. and 0.25 µm film thickness) and equipped with a ISQ 7000 MS detector (Thermo Fisher Scientific, USA) combined with Chromeleon 7 cromatography data system. The oven temperature was set to 40 °C for 10 min and then, increased up to 200 °C, at a rate of 4 °C/min. After the temperature reached 200 °C, it was increased again up to 240 °C, at 15 °C/min. The inlet was kept at 250°C and the temperature of the MS detector for transfer line and ion source was 250°C. The recording mass spectra range was set between 45 and 250 m/z. The samples were injected in split mode, using a split flow of 500 ml/min and a split ratio of 333. Helium was used as carrier gas at a flow rate of 1.5 ml/min. Peak identification of main volatile compounds was based on retention time (RT) compared to that of the used standards and accomplished using NIST mass spectral library with software version 2.4.
HPLC analysis
HPLC analysis of polyphenolic compounds was carried out on a reverse phase column C18 Zorbax Eclipse XDB (150 × 4.6 i.d. mm) mounted on an Agilent 1200 HPLC system consisting of a quaternary pump, thermostated autosampler, and diode array detector (Agilent Technologies, Germany). A sample (10 μL) was injected and then eluted using mobile phase A consisting of 2 mM sodium acetate, pH 3, and mobile phase B, acetonitrile, using the following gradient: 2–20% B, 0–30 min; 20–30% B, 30–40 min; 30% B, 40–50 min; 30–2% B, 50–60 min.[Citation22] The identification of compounds was performed by comparison to the RT of phenolic acids and flavonoids standards at 280 and 370 nm. Quantification of the identified compounds was performed by peak area integration. Standard curves were built for each analyzed phenolic acid and flavonoid in the range of concentrations 10–500 µg/mL. The results were expressed as µg/g dry weight (d.w.).
Determination of total phenolic and flavonoid content
TPC of each hydrosol was determined using the Folin-Ciocalteu assay.[Citation23] Briefly, a volume of 150 µL sample was mixed with 750 μL Folin-Ciocalteu reagent and incubated in the dark, for 5 min. Then, 2 mL of sodium carbonate solution (12%, w/w) and distilled water to reach 15 mL were added, the mixture was vortexed, and incubation continued at room temperature, for 30 min. The optical density (OD) was read at 765 nm using a V-650 UV–VIS spectrophotometer (Jasco Corporation, Japan). The standard curve was built using different concentrations of caffeic acid in the range of 0–500 µg/mL. The results were expressed as µg caffeic acid equivalents (CAE) per g d.w.
Total flavonoids content (TFC) was evaluated by aluminum chloride assay, as previously described.[Citation24] Briefly, hydrosol sample (0.5 mL) was mixed with 1.5 mL methanol, 0.1 mL aluminum chloride solution (10%, w/w), 0.1 mL sodium acetate solution (1 M), and 2.8 mL distilled water. The mixture was incubated at room temperature, for 30 min and then the OD was read at 415 nm using a V-650 UV–VIS spectrophotometer (Jasco Corporation, Japan). The standard curve was built using quercetin solution in the range of concentrations 0–500 µg/mL. The results were expressed as µg quercetin equivalents (QE) per g d.w.
Determination of antioxidant activity
Trolox equivalent antioxidant capacity (TEAC) assay: TEAC assay based on sample’s ability to scavenge cationic-free radicals of ABTS was conducted as previously described.[Citation25] Briefly, ABTS stock solution was prepared by mixing 7 mM ABTS solution with 2.45 mM potassium persulfate in a ratio of 1:1 (v/v) and incubation in the dark, for 16 h. The solution was diluted in distilled water to reach an OD of 0.7 at 734 nm. Hydrosol samples (100 µL) were mixed with 1 mL ABTS reagent and, after 10 min of incubation at room temperature, the OD was measured at 734 nm using a V-650 UV–VIS spectrophotometer (Jasco Corporation, Japan). The standard curve was built using Trolox, an analogue of vitamin E with known antioxidant activity, in the range of concentrations 0–250 µM. The results were expressed as µM Trolox equivalents (TE)/g d.w.
Cupric reducing antioxidant capacity (CUPRAC) assay: The antioxidant activity against cupric ion was analyzed as previously described[Citation19] with minor modifications. Briefly, 1 mL CuCl2 solution (10 mM) was mixed with 1 mL neocuproine (7.5 mM) and 1 mL ammonium acetate buffer (1 M), pH 7. After vortexing, the mixture was incubated at the room temperature, for 10 min, to develop the complex. Then, 100 µL of sample and 1 mL of distilled water were added and incubation continued at the room temperature, for 1 h. The OD was read at 450 nm using a V-650 UV–VIS spectrophotometer (Jasco Corporation, Japan). The blank was prepared by replacing the sample with distilled water. The standard curve was built using Trolox in the range of concentrations 0.1–1.0 mM. The results were expressed as µM TE/g d.w.
Free DPPH radical inhibition assay: DPPH assay based on sample’s capacity to inhibit the formation of free DPPH radicals was performed according to Sugahara et al. protocol,[Citation26] with minor modifications. Briefly, a volume of 1.35 mL DPPH methanolic solution (0.25 mM) was mixed with 150 µL sample at different concentrations (5–500 µg/mL) and 0.9 mL Tris-HCl buffer (0.1 M), pH 7.4. The solutions were placed in the dark, for 30 min and the OD was read at 517 nm using a V-650 UV–VIS spectrophotometer (Jasco Corporation, Japan). The blank was prepared by replacing the sample with buffer. The results were expressed as µM TE/g d.w. The sample concentration that inhibited 50%-free DPPH radicals (IC50) was determined from the DPPH inhibition curve by linear regression analysis.
Superoxide anion scavenging assay: The hydrosols’ capacity to scavenge superoxide radicals was determined according to Yang et al. protocol,[Citation27] with minor modifications. Briefly, different concentrations of sample (5–1500 µg/mL) were mixed with 3 ml of Tris-HCl buffer (50 mM, pH 8.2) and incubated at room temperature, for 10 min. Then, 50 µL of pyrogallol solution (30 mM) were added and, after 4 min of incubation at room temperature, the reaction was stopped by adding 300 µL HCl (8 M). The OD was read at 325 nm using a V650 UV/VIS spectrophotometer (Jasco Corporation, Japan). The blank was prepared by replacing the pyrogallol solution with buffer. The results were expressed as µM TE/g d.w. The IC50 values were calculated by linear regression analysis.
Metal chelating assay: The ferrous ion (Fe2+) chelating activity of hydrosols was assessed using the method described by Santos et al.,[Citation28] with minor modifications. Briefly, various concentrations of hydrosol (75–3000 µg/mL) in a volume of 50 µL were added to 20 µL FeSO4 (0.3 mM) and 160 µL distilled water. The mixture was incubated at room temperature, for 5 min and then, the reaction was initiated by adding 30 µL ferrozine solution (0.8 mM). After 15 min of incubation, the OD of the solution containing the colored ferrozine complex was read at 562 nm, using a SpectroStar nano microplate reader (BMG Labtech, Germany). The blank was prepared by replacing the ferrozine solution with distilled water. The results were expressed as µM TE/g d.w. The IC50 values were calculated by linear regression analysis.
In vitro inhibition of acetylcholinesterase
An in vitro experimental model mimicking the effect of hydrosols on aphids’ nervous system through inhibition of AChE activity was developed to assess their biopesticide potential. The colorimetric assay based on thiocholine reaction with Ellman’s reagent was performed using a 96-well microplate adaptive protocol.[Citation29] Briefly, 100 µL DTNB reagent (3 mM) was mixed in the wells of a microplate with 20 µL of AChE (0.125 U/mL) in Tris buffer (50 mM, pH 8) containing 0.1% bovine serum albumin, and 20 µL sample of different concentrations. The mixture representing the control was incubated at 25°C with occasionally shaking, for 15 min and then, its OD (ODcontrol) was read at 412 nm using a SpectroStar nano microplate reader (BMG Labtech, Germany). Then, the enzymatic reaction was initiated by adding 20 µL of acetylthiocholine iodide (7.5 mM) as substrate and the hydrolysis reaction was followed by OD recording at 412 nm, every 3 min, for 30 min (ODsample). A blank was similarly processed after sample replacement with an equal volume of buffer (ODblank). Quercetin solution was used as positive control. The results of AChE inhibition were calculated using the following formula and then, IC50 values were determined for each sample.
Statistical analysis
The experiments were performed in triplicate and the results were expressed as mean ± standard deviation (SD) (n = 3). Statistical differences were calculated on each pair of interest by two-tailed, equal variance Student t-test (Excel v.10). They were considered significant at p < .05. The relationship between TPC and TFC values and the antioxidant and AChE inhibition activity was analyzed by Pearson correlation coefficients.
Results
In this study, hydrosols were obtained as brown colored solutions with pleasant odor by steam distillation of aerial parts of sage, rosemary, and lavender, after separation of essential oils. The extraction yield had values of 11.97 ± 1.09% (w/w) for sage, 12.07 ± 0.77% for rosemary and 12.17 ± 0.85% for lavender hydrosols.
Chemical composition of hydrosols
GC-MS analysis of hydrosols and the relative abundance of main volatile compounds are presented in . In sage hydrosol, camphor was the prevalent compound, but thujone and isothujone were also identified. In rosemary hydrosol, six volatile compounds were detected and among them, camphor, verbenone, and eucalyptol (1,8-cineole) were the most abundant compounds. Lavender hydrosol presented a composition of volatile compounds rich in linalool and its derivatives, coumarin, α-terpineol, and caryophyllene oxide. These results indicated that steam distilled hydrosols contained cyclic monoterpenes and their oxygenated derivatives, which have previously shown more bioactivity than acyclic ones.[Citation30] The composition in volatile compounds of the analyzed hydrosols was similar to that of corresponding essential oils, but components distribution and abundance was lower (data not showed).
Table 1. The composition in volatile compounds of sage, rosemary, and lavender hydrosols, determined by GC-MS analysis
The polyphenolic compounds identified and quantified by HPLC analysis of sage, rosemary, and lavender hydrosols are presented in . Rosmarinic acid, a characteristic compound of Lamiaceae plant species was found in all hydrosols, but it was the prevailing compound in sage and rosemary hydrosols. Caffeic and ferulic acids were also present in all hydrosols, but higher quantities were found in sage hydrosol. The main flavonoids quercetin, rutin, luteolin, and the glycosylated derivatives of quercetin (isoquercetin) and kaempferol (astragalin) were identified in different proportions. The glycosylated derivative of luteolin (cynaroside) was detected only in sage hydrosol.
Table 2. The composition in phenolic compounds determined by HPLC analysis and the TPC and TFC values of sage, rosemary, and lavender hydrosols
The values are expressed as mean ± SD (n = 3). Further, TPC and TFC values of sage, rosemary, and lavender hydrosols were determined. The results have shown that sage hydrosol had the highest TPC, followed by rosemary and lavender hydrosols (). The same variation was observed for flavonoids content and 2 times higher TFC value was found in sage hydrosol, compared to those of rosemary and lavender hydrosols.
Antioxidant activity of hydrosols
In this study, the screening of the antioxidant activity of each hydrosol was performed using four assays of reactive oxygen species (ROS) with scavenging mechanisms based on hydrogen atom transfer (HAT) (TEAC assay), single-electron transfer (SET) (CUPRAC assay) and mixed mode of action (DPPH and superoxide assays), and one metal chelating assay. The results expressed as TE are presented in .
Table 3. The antioxidant activity of sage, rosemary, and lavender hydrosols determined by TEAC, CUPRAC, DPPH, superoxide inhibition, and metal chelating assays
The results were expressed as Trolox equivalents (TE) and inhibitory concentration of 50% radicals (IC50). The values are expressed as mean ± SD (n = 3). ap < .05, compared to sage; bp < .05, compared to rosemary; cp < .05, compared to lavender hydrosol.
In TEAC and CUPRAC assays, sage hydrosol exhibited the highest antioxidant activity, followed by rosemary hydrosol and both values were significantly (p < .05) higher than that of lavender hydrosols. In DPPH assay, sage and rosemary hydrosols had similar antioxidant activity and higher than that of lavender hydrosol. In superoxide anion scavenging assay, sage hydrosol presented the best inhibition capacity, while lavender and rosemary hydrosols had similar values, but significantly (p < .05) lower. In like manner, sage hydrosol was the best Fe2+ ion chelator, followed by rosemary and lavender hydrosols, presenting close values. Still, the results of metal chelating activity indicated lower antioxidant capacity of steam distilled hydrosols, compared to other assays.
In vitro inhibition of AChE activity
In vitro inhibition of AChE activity in the presence of the analyzed hydrosols is given in . The results showed that all hydrosols exerted inhibition of this enzyme activity in a dose-dependent manner. Rosemary hydrosol presented a steep increase of AChE inhibition from 10.65% at 2.5 mg/mL to 42.48% at 5 mg/mL. In the same range of concentrations, sage, and lavender hydrosols presented 160 and 130% increase of AChE inhibition, respectively. At 5 mg/mL, rosemary hydrosol presented significantly (p < .05) higher AChE inhibition than sage and lavender hydrosols. Its effect on AChE inhibition was similar to that of quercetin, used as control, indicating potential as neurological agent on pests. The IC50 values varied in the same order, that is, rosemary was more efficient (6.23 mg/mL) than sage (7.48 mg/mL) and lavender (15.08 mg/mL) hydrosols.
Figure 1. In vitro capacity of acetylcholinesterase (AChE) inhibition in the presence of sage, rosemary and lavender hydrosols. The values are expressed as mean ± SD (n = 3). ap<.05, compared to sage; bp<.05, compared to rosemary; cp<.05, compared to lavender hydrosol; dp<.05, compared to quercetin.
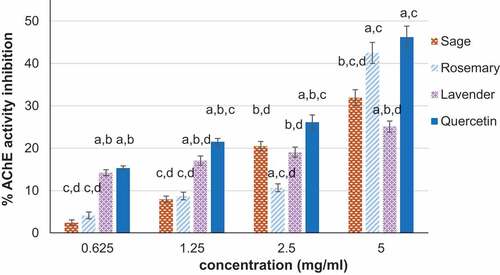
Chemical composition – biological activity relationship
The Pearson correlation coefficients, showing the relationship between the biological activity of the analyzed hydrosols and their chemical composition, are presented in . The TEAC and CUPRAC were strongly correlated (R2 > 0.8) in a linear, positive manner to TPC of aromatic plants hydrosols. Moderate (R2 > 0.6) and low (R2 < 0.6) correlation of the antioxidant activity and the flavonoid content of hydrosols was observed in CUPRAC and TEAC assays, respectively. Metal chelating and superoxide anion scavenging activity presented strong, linear, and positive correlation to the content of flavonoid compounds, while moderate correlation to TPC was observed. Free DPPH radical scavenging activity had moderate correlation to TPC and low correlation to TFC of the analyzed hydrosols. This analysis confirmed that the increasing content of polyphenolic compounds, either TPC or TFC, the more significant antioxidant activity of the hydrosol, excepting DPPH assay. These compounds provided higher number of hydroxyl groups, efficient in redox processes. Moderate correlation was observed between AChE inhibition and TPC of hydrosols and low correlation coefficient value in relation to TFC. This analysis indicated that other compounds, such as volatile compounds present in hydrosols, might also influence AChE inhibition and further, pests’ neurological system.
Table 4. Pearson correlation coefficients between the antioxidant and AChE inhibition activity and the TPC, TFC values of sage, rosemary, and lavender hydrosols
Discussion
This study aimed to investigate the antioxidant and biopesticide potential of three hydrosols obtained by steam distillation of Lamiaceae plants (sage, rosemary, and lavender), as natural alternative products to synthetic pesticides, and intended for an ecological protection of crops, low risk of developing pest resistance and sustainable management of soil and environment. In the same time, valorization of by-products in the form of hydrosols represents a biotechnological advantage.
Unlike essential oils, hydrosols contain low amount of volatile compounds. However, this could be an advantage in terms of reduced toxicity when used as natural pesticides. Several investigators have studied the volatile compounds of Lamiaceae hydrosols, revealing different composition. The variability of the volatiles profile of plant extracts depends on various factors, like agronomic practices, climatic changes, species, regions, and extraction method.[Citation18,Citation31] Thus, camphor was the major compound found in hydrosols obtained by steam distillation of Moroccan (51%) and Turkish (43.38%) sage, followed by 1,8-cineole (24%), thujone (12.9%), and cis-thujone (15.46%), respectively.[Citation4,Citation31] A recent study on Italian sage hydrosol revealed similar constituents, but in different proportions, that is, 1,8-cineole (61.4%), camphor (22.5%), and α-thujone (8.4%).[Citation32] Besides these volatile compounds, sage hydrosol also contained linalool (13.41%), δ-cadinene (10.18%) and carvacrol (8.96%),[Citation18] borneol (7.66%), humulene oxide (1.12%) and myrtenol (0.55%).[Citation31] These results were consistent with those obtained in the present study, reporting camphor and thujone as main volatile components of Romanian sage hydrosol prepared by steam distillation. This was a similar composition to that of the essential oil extracted from S. officinalis, but presented a different distribution of volatile compounds.[Citation33]
Rosemary hydrosols obtained by various steam distillation procedures contained camphor (41–51.9%) and 1,8-cineole (29.30–38.20%), as main volatile compounds, and lower percentage of verbenone (1.8–4.2%), borneol (7.4%), terpinen-4-ol (3.4%), and 3-octanone (2.1–3.2%).[Citation7,Citation34] Other studies reported the prevalence of terpinen-4-ol (51.9%), 1,8-cineole (50.25%), or verbenone (45.31%) in the chemical profile of rosemary hydrosols, according to the geographical region of rosemary cultivation in Italy,[Citation35] Morocco,[Citation4] Colombia,[Citation36] or Korea.[Citation37] Moreover, the chemical profile of rosemary hydrosols varied in relation to the harvesting season; for example, the hydrosol harvested in August presented equal proportions of aroma components, that is, camphor (24.9%), α-terpineol (21.19%), and borneol (20.4%).[Citation34] The findings of this study on Romanian rosemary hydrosol were in accordance to previous results, showing that camphor, 1,8-cineole, and verbenone were the main volatile compounds in steam distilled preparations.
Linalool was found as major compound (26.5–45%) of the hydrosols prepared from lavender harvested from Moroccan, Italian, and Polish regions.[Citation4,Citation35,Citation38] Variable linalool content between 39.2% and 53.0% was reported according to the lavender plant part used as raw material, for example, fresh or dried flowers, or herbs,[Citation39] and also to the used distillation method, being 23.2% after hydrodistillation and 7.9% after steam distillation.[Citation40] The main volatile compounds identified according to the ISO standard in lavender essential oils were linalyl acetate (36.55%), linalool (31.17%), cis-β-ocimene (3.33%), lavandulyl acetate (1.86%), 1-octen-3-yl acetate (1.64%), 1,8-cineole (1.46%), and camphor (0.53%).[Citation41] Comparison of different distillation extracts of Italian L. intermedia showed that the major compound of the essential oil, linalyl acetate, was replaced by linalool and linalool oxide in the hydrosol of the same species, being completed by lower percentage of 1,8-cineole, camphor, geraniol, and borneol.[Citation11] The results of this study were consistent with previous studies, indicating a broad distribution of several volatiles in the lavender hydrosol prepared by steam distillation, with linalool and derivatives, α-terpineol and terpinen-4-ol, and coumarin as main compounds.
In regard of phenolics, sage hydrosol had higher TPC and TFC values than rosemary and lavender hydrosols, which presented close values, in accordance to a recent report of Jakubczyk et al..[Citation42] Furthermore, the HPLC analysis of sage, rosemary, and lavender hydrosols has shown the presence of ferulic and caffeic acids, while flavonoids quercetin and luteolin were present as aglycones and glycosylated derivatives, isoquercetin and cynaroside, together with kaempferol 3-glucoside (astragalin). No reports were found in the literature reporting similar HPLC analysis of hydrosols.
ROS (hydroxyl, superoxide, singlet oxygen, hydrogen peroxide) are the result of the metabolic activity of normal or inflamed cells, or a response to external factors, such as pesticides, pollutants, organic solvents, radiation, smoking.[Citation43] It is known that polyphenolic compounds and volatile oils present in plants act as antioxidant compounds, which catalyze the transformation of ROS into stable products, favoring the ability to defend against oxidative stress, at the cellular and molecular level. In addition, the metal chelating ability plays an important role in disrupting the chain of ROS propagation. Polyphenolic compounds, in particular, phenolic acids and flavonoids presenting aromatic rings substituted in ortho- and para-position, demonstrated antioxidant activity and plant protection against aggression by environmental factors,[Citation44] but no studies correlated their presence in hydrosols obtained as by-products to the consistent ROS scavenging capacity. The latter was mostly linked to the presence of water-soluble compounds of essential oils, such as 1,8-cineole and linalool, with established antioxidant activity[Citation4,Citation6] and also found in rosemary and lavender hydrosols from this study. In turn, the oxygenated monoterpenes camphor and thujone were previously granted as least active compounds in TEAC and DPPH assays.[Citation45] However, sage hydrosol prepared in this study by steam distillation had the best free radical scavenging capacity, closely followed by rosemary hydrosol, similar to previous results on Italian sage and rosemary hydrosols.[Citation32,Citation35]
To the best of our knowledge, this is the first report on the potential of aromatic plant hydrosols to inhibit AChE activity and its correlation to their TPC and TFC values. AChE enzyme is involved in the termination of synaptic transmission in vivo by rapid hydrolysis of the neurotransmitter acetylcholine to choline and acetic acid, which cause pest development disturbances.[Citation46] The mechanism of AChE activity inhibition attributed to high concentrations of monoterpenoids was based on chloride channels activity alteration through octopaminergic pathways, changing the neurohormonal levels, the gustatory and olfactory response.[Citation20,Citation47] Another mechanism of AChE inhibition was based on alkaloids and saponins binding to the amino acids from the enzyme’s active site and modulation of sodium channels, slowing down its functioning and affecting the cytoplasmic calcium level, which led to neurological effects .[Citation17]
AChE inhibitory potential was previously investigated only for essential oils and plant extracts of the studied species, showing variation in relation to the extraction method. Thus, essential oil of rosemary prevailed over different organic solvent (methanol, petroleum ether, chloroform, ethyl acetate) extracts, being effective AChE activity inhibition[Citation48] and the same observation was reported for sage and lavender essential oils, compared to ethanolic and aqueous extracts.[Citation49] In addition, individual monoterpenes were tested for AChE inhibition potential and only the cyclic monoterpene 1,8-cineole showed a dose-dependent manner activity.[Citation50,Citation51] Its presence in sage essential oil was correlated to the AChE inhibition.[Citation52] In this study, 1,8-cineole was present in high proportion in rosemary hydrosol, which also exerted AChE inhibition activity, indicating its potential effect on insects’ nervous system. No activity toward AChE was determined for camphor and linalool,[Citation50] the major compounds detected in this study in sage/rosemary and lavender hydrosols, respectively. Other monoterpenes, like β-pinene and borneol found in sage essential oil contributed to AChE activity inhibition,[Citation53] but they were not detected in Romanian sage hydrosol. In addition, 1,8-cineole and camphor, detected in high proportions in sage and rosemary hydrosols, had unpleasant odor and were classified as insect repellents and antifeedants.[Citation11,Citation54]
The inhibitory effect on AChE activity was also reported for individual phenolic acids, in the following order rosmarinic acid > caffeic acid > gallic acid = chlorogenic acid > homovanillic acid > sinapic acid,[Citation17,Citation55] but also for ferulic and chlorogenic acid.[Citation56] Flavonoids, like quercetin, kaempferol and, in a lesser extent, luteolin were also reported as efficient AChE inhibitors[Citation17,Citation57] and detected as such or in their glycosylated forms in all hydrosols analyzed in this study. A previous study showed that the ethanolic extract of sage, rich in phenolic compounds, had higher AChE inhibition rate than rosemary and lavender extracts.[Citation56] According to this study, rosemary and sage hydrosols were better AChE inhibitors, compared to lavender hydrosol. Correlation coefficients of hydrosols’ AChE inhibition and their TPC and TFC values were lower than those for their antioxidant activity, thus suggesting the involvement of both volatiles and polyphenolic compounds found in hydrosols in the process of AChE inhibition.
Conclusion
The results showed that sage and rosemary hydrosols were more effective antioxidants in comparison to lavender hydrosol. The antioxidant activity of hydrosols obtained by steam distillation from Lamiaceae plants was strongly correlated to the total phenolic and flavonoid content in all free radical scavenging and metal chelating assays, excepting the DPPH assay. In addition, sage and rosemary hydrosols had the capacity to inhibit AChE, thus presenting the potential to affect pests at neurological level. The moderate to low correlation between AChE inhibition and TPC, TFC values suggested that other bioactive compounds present in hydrosols could also intervene in this important process. In conclusion, Lamiaceae plant hydrosols could be further tested as natural products or mixtures in biopesticide formulations for the treatment of eco-agrosystems.
Disclosure statement
No potential conflict of interest was reported by the author(s).
Additional information
Funding
References
- Acimovic, M. G.; Tesevic, VV; Smiljanic, K. T.; Cvetkovic, M. T.; Stankovic, J. M.; Kiprovski, B. M.; Sikora, V. S.; Tešević, V. Hydrolates: by-products of essential oil distillation: chemical composition, biological activity and potential uses. Adv Technol. 2020, 9(2), 54–70. DOI: 10.5937/savteh2002054A.
- D’Amato, S.; Serio, A.; Lopez, C. C.; Paparella, A. Hydrosols: biological activity and potential as antimicrobials for food applications. Food Control. 2018, 86, 126–137. DOI: 10.1016/j.foodcont.2017.10.030.
- Paolini, J.; Leandri, C.; Desjobert, J. M.; Barboni, T., and Costa, J. Comparison of liquid-liquid extraction with headspace methods for the characterization of volatile fractions of commercial hydrolates from typically Mediterranean species. J. Chromatogr. A. 2008, 1193(1–2), 37–49. DOI: 10.1016/j.chroma.2008.04.021.
- Aazza, S.; Miguel, M. G. Antioxidant activity of some Moroccan hydrosols. J. Med. Plant Res 2011, 5, 6688–6696.
- Cid-Perez, T. S.; Avila-Sosa, R.; Ochoa-Velasco, C. E.; Rivera-Chavira, B. E.; Nevarez-Moorillon, G. V. Antioxidant and antimicrobial activity of Mexican oregano (Poliomintha longiflora) essential oil, hydrosol and extracts from waste solid residues. Plants. 2019, 8(1), 22. DOI: 10.3390/plants8010022.
- Prusinowska, R.; Smigielski, K.; Stobiecka, A.; Kunicka-Styczynska, A. Hydrolates from lavender (Lavandula angustifolia) – their chemical composition as well as aromatic, antimicrobial and antioxidant properties. Nat. Prod. Res 2016, 30(4), 386–393. DOI: 10.1080/14786419.2015.1016939.
- Hay, Y. O. M.; Abril Sierra, M. A.; Tellez, M.; Sequeda, C. L. G.; Tellez, A. A. N.; Bonnafous, C.; Raynaud, C. Phytochemical, antioxidant and antimicrobial parameters of essential oils and hydrosols of Colombian thyme and rosemary obtained using two different steam distillation methods. Int J Phytocos Nat Ingred. 2015, 2(1), 7. DOI: 10.15171/ijpni.2015.07.
- Belabbes, R.; Dib, M. E. A.; Djabou, N.; Ilias, F.; Tabti, B.; Costa, J., and Muselli, A. Chemical variability, antioxidant and antifungal activities of essential oils and hydrosol extract of Calendula arvensis L. from Western Algeria. Chem. Biodiv 2017, 14(5), e1600482. DOI: 10.1002/cbdv.201600482.
- Degirmenci, H., and Erkurt, H. Relationship between volatile components, antimicrobial and antioxidant properties of the essential oil, hydrosol and extracts of Citrus aurantium L. flowers. J. Infect Public Health. 2020, 13(1), 58–67. DOI: 10.1016/j.jiph.2019.06.017.
- Traka, C. K.; Petrakis, E. A.; Kimbaris, A. C.; Polissiou, M. G., and Perdikis, D. C. Effects of Ocimum basilicum and Ruta chalepensis hydrosols on Aphis gossypii and Tetranychus urticae. J Appl Entomol. 2018, 142(4), 413–420. DOI: 10.1111/jen.12486.
- Politi, M.; Menghini, L.; Conti, B.; Bedini, S.; Farina, P.; Cioni, P. L.; Braca, A.; de, L. M. Reconsidering hydrosols as main products of aromatic plants manufactory: the lavandin (Lavandula intermedia) case study in Tuscany. Molecules. 2020, 25(9), 2225. DOI: 10.3390/molecules25092225.
- Zekri, N.; Handaq, N.; Caidi, A. E.; Zair, T., and Belghiti, M. A. E. Insecticidal effect of Mentha pulegium L. and Mentha suaveolens Ehrh. hydrosols against a pest of citrusToxoptera aurantii (Aphididae). Res Chem Intermed. 2016, 42(3), 1639–1649. DOI: 10.1007/s11164-015-2108-0.
- Petrakis, E. A.; Kimbaris, A. C.; Lykouressis, D. P.; Polissiou, M. G., and Perdikis, D. C. Hydrosols evaluation in pest control: insecticidal and settling inhibition potential against Myzus persicae (Sulzer). J Appl Entomol. 2015, 139(4), 260–267. DOI: 10.1111/jen.12176.
- Poonsri, W.; Pengsook, A.; Pluempanupat, W.; Yooboon, T., and Bullangpoti, V. Evaluation of Alpinia galanga (Zingiberaceae) extracts and isolated trans-cinnamic acid on some mosquitoes larvae. Chem. Biol. Technol. Agric 2019, 6(1), 17. DOI: 10.1186/s40538-019-0157-0.
- Spochacz, M.; Chowanski, S.; Walkowiak-Nowicka, K.; Szymczak, M.; Adamski, Z. Plant-derived substances used against beetles-pests of stored crops and food and their mode of action: a review. Compr. Rev. Food Sci. Food Saf 2018, 17(5), 1339–1366. DOI: 10.1111/1541-4337.12377.
- Hernandez-Carlos, B.; Gamboa-Angulo, M. Insecticidal and nematicidal contributions of Mexican flora in the search for safer biopesticides. Molecules. 2019, 24(5), 897–931. DOI: 10.3390/molecules24050897.
- Szwajgier, D. Anticholinesterase activity of selected phenolic acids and flavonoids-interaction testing in model solutions. Ann. Agric. Environ. Med 2015, 22(4), 690–694. DOI: 10.5604/12321966.1185777.
- Tornuk, F.; Cankurt, H.; Ozturk, I.; Sagdic, O.; Bayram, O., and Yetim, H. Efficacy of various plant hydrosols as natural food sanitizers in reducing Escherichia coli O157:H7 and Salmonella typhimurium on fresh cut carrots and apples. Int. J. Food Microbiol 2011, 148(1), 30–35. DOI: 10.1016/j.ijfoodmicro.2011.04.022.
- Georgiev, V.; Ananga, A.; Dincheva, I.; Badjakov, I.; Gochev, V.; Tsolova, V. Chemical composition, in vitro antioxidant potential, and antimicrobial activities of essential oils and hydrosols from native American muscadine grapes. Molecules. 2019, 24(18), 3355–3367. DOI: 10.3390/molecules24183355.
- Kostyukovsky, M.; Rafaeli, A.; Gileadi, C.; Demchenko, N.; Shaaya, E. Activation of octopaminergic receptors by essential oil constituents isolated from aromatic plants: possible mode of action against insect pests. Pest Manag. Sci 2002, 58(11), 1101–1106. DOI: 10.1002/ps.548.
- Jankowska, M.; Rogalska, J.; Wyszkowska, J.; Stankiewicz, M. Molecular targets for components of essential oils in the insect nervous system-a review. Molecules. 2017, 23(1), 34. DOI: 10.3390/molecules23010034.
- Craciunescu, O.; Constantin, D.; Gaspar, A.; Toma, L.; Utoiu, E., and Moldovan, L. Evaluation of antioxidant and cytoprotective activities of Arnica montana L. and Artemisia absinthium L. ethanolic extracts. Chem. Cent. J 2012, 6(1), 97. DOI: 10.1186/1752-153X-6-97.
- Moldovan, L.; Gaspar, A.; Toma, L.; Craciunescu, O.; Saviuc, C. Comparison of polyphenolic content and antioxidant capacity of five Romanian traditional medicinal plants. Rev. Chim 2011, 62, 299–305.
- Gaspar, A.; Craciunescu, O.; Trif, M.; Moisei, M., and Moldovan, L. Antioxidant and anti-inflammatory properties of active compounds from Arnica montana L. Rom. Biotechnol. Lett 2014, 19, 9353–9365.
- Savin, S.; Craciunescu, O.; Oancea, A.; Ilie, D.; Ciucan, T.; Antohi, L. S.; Toma, A.; Nicolescu, A.; Deleanu, C., and Oancea, F. Antioxidant, cytotoxic and antimicrobial activity of chitosan preparations extracted from Ganoderma lucidum mushroom. Chem. Biodiv 2020, 17(7), e2000175. DOI: 10.1002/cbdv.202000175.
- Sugahara, S.; Ueda, Y.; Fukuhara, K.; Kamamuta, Y.; Matsuda, Y.; Murata, T.; Kuroda, Y.; Kabata, K.; Ono, M., and Igoshi, K., et al. Antioxidant effects of herbal tea leaves from yacon (Smallanthus sonchifolius) on multiple free radical and reducing power assays, especially on different superoxide anion radical generation systems. J. Food Sci 2015, 80(11), C2420–9. DOI: 10.1111/1750-3841.13092.
- Yang, Y.; Liu, D.; Wu, J.; Chen, Y., and Wang, S. In vitro antioxidant activities of sulfated polysaccharide fractions extracted from Corallina officinalis. Int. J. Biol. Macromol 2011, 49(5), 1031–1037. DOI: 10.1016/j.ijbiomac.2011.08.026.
- Santos, J. S.; Brizola, V. R. A.; Granato, D. High-throughput assay and standardization for metal chelating capacity screening: a proposal and application. Food Chem 2017, 214, 515–522. DOI: 10.1016/j.foodchem.2016.07.091.
- Mathew, M.; Subramanian, S.; Forloni, G. In vitro screening for anti-cholinesterase and antioxidant activity of methanolic extracts of ayurvedic medicinal plants used for cognitive disorders. Plos One. 2014, 9(1), e86804. DOI: 10.1371/journal.pone.0086804.
- Zielinska-Blajet, M.; Feder-Kubis, J. Monoterpenes and their derivatives-recent development in biological and medical applications. Int. J. Mol. Sci 2020, 21(19), 7078. DOI: 10.3390/ijms21197078.
- Baydar, H.; Sangun, M. K.; Erbas, S.; Kara, N. Comparison of aroma compounds in distilled and extracted products of sage (Salvia officinalis L.). J. Essent. Oil Bear. Plants. 2013, 16(1), 39–44. DOI: 10.1080/0972060X.2013.764175.
- Ovidi, E.; Laghezza Masci, V.; Zambelli, M.; Tiezzi, A.; Vitalini, S., and Garzoli, S. Laurus nobilis, Salvia sclarea and Salvia officinalis essential oils and hydrolates: evaluation of liquid and vapor phase chemical composition and biological activities. Plants. 2021, 10(4), 707. DOI: 10.3390/plants10040707.
- Sharma, Y.; Velamuri, R.; Fagan, J.; Schaefer, J.; Streicher, C.; Stimson, J. Identification and characterization of polyphenols and volatile terpenoid compounds in different extracts of garden sage (Salvia officinalis L.). Pharmacogn. Res 2020, 12(2), 149. DOI: 10.4103/pr.pr_92_19.
- Tomi, K.; Kitao, M.; Konishi, N.; Murakami, H.; Matsumura, Y.; Hayashi, T. Enantioselective GC–MS analysis of volatile components from rosemary (Rosmarinus officinalis L.) essential oils and hydrosols. Biosci. Biotechnol. Biochem 2016, 80(5), 840–847. DOI: 10.1080/09168451.2016.1146066.
- Garzoli, S.; Laghezza Masci, V.; Franceschi, S.; Tiezzi, A.; Giacomello, P., and Ovidi, E. Headspace/GC–MS analysis and investigation of antibacterial, antioxidant and cytotoxic activity of essential oils and hydrolates from Rosmarinus officinalis l. and Lavandula angustifolia miller. Foods. 2021, 10(8), 1768. DOI: 10.3390/foods10081768.
- Hay, Y. O.; Abril-Sierra, M. A.; Sequeda-Castaneda, L. G.; Bonnafous, C.; Raynaud, C. Evaluation of combinations of essential oils and essential oils with hydrosols on antimicrobial and antioxidant activities. J Pharm Pharmacogn Res. 2018, 6, 216–230.
- Jeon, D. H.; Moon, J. Y.; Hyun, H. B., and Cho, K. S. Composition analysis and antioxidant activities of the essential oil and the hydrosol extracted from Rosmarinus officinalis L. and Lavandula angustifolia mill. produced in jeju. J. Appl. Biol. Chem 2013, 56(3), 141–146. DOI: 10.3839/jabc.2013.023.
- Smigielski, K. B.; Prusinowska, R.; Krosowiak, K., and Sikora, M. Comparison of qualitative and quantitative chemical composition of hydrolate and essential oils of lavender (Lavandula angustifolia). J. Essent. Oil Res 2013, 25(4), 291–299. DOI: 10.1080/10412905.2013.775080.
- Kunicka-Styczynska, A.; Smigielski, K.; Prusinowska, R.; Rajkowska, K.; Kusmider, B.; Sikora, M. Preservative activity of lavender hydrosols in moisturizing body gels. Lett Appl. Microbiol 2015, 60(1), 27–32. DOI: 10.1111/lam.12346.
- Silha, D.; Svarcova, K.; Bajer, T.; Kralovec, K.; Tesarova, E.; Mouckova, K.; Bajerova, P., and Bajerová, P. Chemical composition of natural hydrolates and their antimicrobial activity on Arcobacter-like cells in comparison with other microorganisms. Molecules. 2020, 25(23), 5654. DOI: 10.3390/molecules25235654.
- Beale, D. J.; Morrison, P. D.; Karpe, A. V.; Dunn, M. S. Chemometric analysis of lavender essential oils using targeted and untargeted GC-MS acquired data for the rapid identification and characterization of oil quality. Molecules. 2017, 22(8), 1339. DOI: 10.3390/molecules22081339.
- Jakubczyk, K.; Tuchowska, A.; Janda-Milczarek, K. Plant hydrolates–antioxidant properties, chemical composition and potential applications. Biomed. Pharmacother 2021, 142, 112033. DOI: 10.1016/j.biopha.2021.112033.
- Bhattacharyya, A.; Chattopadhyay, R.; Mitra, S.; Crowe, S. E. Oxidative stress: an essential factor in the pathogenesis of gastrointestinal mucosal disease. Physiol. Rev 2014, 94(2), 329–354. DOI: 10.1152/physrev.00040.2012.
- Francik, S.; Francik, R.; Sadowska, U.; Bystrowska, B.; Zawislak, A.; Knapczyk, A.; Nzeyimana, A. Identification of phenolic compounds and determination of antioxidant activity in extracts and infusions of salvia leaves. Materials. 2020, 13(24), 5811. DOI: 10.3390/ma13245811.
- Sharopov, F. S.; Wink, M.; Setzer, W. N. Radical scavenging and antioxidant activities of essential oil components-an experimental and computational investigation. Nat. Prod. Commun 2015, 10(1), 153–156.
- Picciotto, M. R.; Higley, M. J.; Mineur, Y. S. Acetylcholine as a neuromodulator: cholinergic signaling shapes nervous system function and behavior. Neuron. 2012, 76(1), 116–129. DOI: 10.1016/j.neuron.2012.08.036.
- Isman, M. B. Botanical insecticides, deterrents, and repellents in modern agriculture and an increasingly regulated world. Ann. Rev. Entomol. 2006, 51(1), 45–66. DOI: 10.1146/annurev.ento.51.110104.151146.
- Orhan, I.; Aslan, S.; Kartal, M.; Sener, B., and Baser, K. H. C. Inhibitory effect of Turkish Rosmarinus officinalis L. on acetylcholinesterase and butyrylcholinesterase enzymes. Food Chem 2008, 108(2), 663–668. DOI: 10.1016/j.foodchem.2007.11.023.
- Ferreira, A.; Proenca, C.; Serralheiro, M. L. M.; Araujo, M. E. M. The in vitro screening for acetylcholinesterase inhibition and antioxidant activity of medicinal plants from Portugal. J. Ethnopharmacol 2006, 108(1), 31–37. DOI: 10.1016/j.jep.2006.04.010.
- Kharraf, S. E.; Faleiro, M. L.; Abdellah, F.; El-Guendouz, S.; El Hadrami, E. M., and Miguel, M. G. Simultaneous hydrodistillation-steam distillation of Rosmarinus officinalis, Lavandula angustifolia and Citrus aurantium from Morocco, major terpenes: impact on biological activities. Molecules. 2021, 26(18), 5452. DOI: 10.3390/molecules26185452.
- Abdelgaleil, S. A. M.; Mohamed, M. I. E.; Badawy, M. E. I., and El-Arami, S. A. A. Fumigant and contact toxicities of monoterpenes to Sitophilus oryzae (L.) and Tribolium castaneum (Herbst) and their inhibitory effects on acetylcholinesterase activity. J. Chem. Ecol 2009, 35(5), 518–525. DOI: 10.1007/s10886-009-9635-3.
- Cutillas, A. B.; Carrasco, A.; Martinez-Gutierrez, R.; Tomas, V.; Tudela, J. Salvia officinalis L. essential oils from Spain: determination of composition, antioxidant capacity, antienzymatic, and antimicrobial bioactivities. Chem. Biodiv 2017, 14(8), e1700102. DOI: 10.1002/cbdv.201700102.
- Tundis, R.; Leporini, M.; Bonesi, M.; Rovito, S.; Passalacqua, N. G. Salvia officinalis L. from Italy: a comparative chemical and biological study of its essential oil in the mediterranean context. Molecules. 2020, 25(24), 5826. DOI: 10.3390/molecules25245826.
- da, S. M. R. M.; Ricci-Junior, E. An approach to natural insect repellent formulations: from basic research to technological development. Acta Trop 2020, 212, 105419. DOI: 10.1016/j.actatropica.2020.105419.
- Gulcin, I.; Scozzafava, A.; Supuran, C. T.; Koksal, Z.; Turkan, F.; Cetinkaya, S.; Bingol, Z.; Huyut, Z.; Alwasel, S. H. Rosmarinic acid inhibits some metabolic enzymes including glutathione S-transferase, lactoperoxidase, acetylcholinesterase, butyrylcholinesterase and carbonic anhydrase isoenzymes. J. Enzyme Inhib. Med. Chem 2016, 31(6), 1698–1702. DOI: 10.3109/14756366.2015.1135914.
- Vladimir-Knezevic, S.; Blazekovic, B.; Kindl, M.; Vladic, J.; Lower-Nedza, A. D., and Brantner, A. H. Acetylcholinesterase inhibitory, antioxidant and phytochemical properties of selected medicinal plants of the Lamiaceae family. Molecules. 2014, 19(1), 767–782. DOI: 10.3390/molecules19010767.
- Khan, H.; Amin, S.; Kamal, M. A.; Patel, S. Flavonoids as acetylcholinesterase inhibitors: current therapeutic standing and future prospects. Biomed. Pharmacother 2018, 101, 860–870. DOI: 10.1016/j.biopha.2018.03.007.