ABSTRACT
This study was aimed to analyze and compare the volatile compounds of flesh, peel and seed parts of the pumpkin (Cucurbita maxima). The volatile compounds were extracted from three different parts of pumpkin using a hydro-distillation method. Extracts from the three different parts of pumpkin were injected into the gas chromatography-mass spectrometry system. Mass spectral data were collected from 40–650 m/z. The volatile components were identified by matching their mass spectra with those of reference compounds recorded in the mass spectral library and retention indices. A homologous series of n-alkanes (C9–C30) were run under the same operating conditions as the volatile oils to determine the retention indices. The chromatographic analysis revealed the presence of 15, 23 and 28 components in the essential oils obtained from flesh, peel and seed parts of pumpkin representing a total composition of 81.5, 84.4, and 97.7%, respectively. Out of volatile compounds that were identified, three were common to all 3 parts, six were common to flesh and peel, eight were common to peel and seed, and five were common to flesh and seed. Hydrocarbons (43.6%) and esters (26.4%) were the most abundant volatile compounds identified in the seed part of pumpkin followed by sulfur containing compounds (30.3%) in the peel, (23.7%) in the flesh and (12.7%) in seed parts of pumpkin. Terpinene compounds were also found (23.5%) in the flesh, (22.9%) in the peel and (5.02%) in the seed parts of pumpkin. The compositions of the volatile compounds vary from one part to another of the pumpkin. Some of the volatile chemicals discovered in this investigation matched with the published data from other nations. According to the findings of this study, different volatile chemicals detected in various parts of the pumpkin indicated increased medicinal and nutritional potential.
Introduction
Pumpkin is one of the most widely grown crops in the Cucurbitaceae family, and it is used as a vegetable and medicine all over the world.[1Citation2] Cucurbita is a genus of plants belonging to the Cucurbitaceae family, which includes pumpkin, zucchini, squash, marrow, courgette, gourd melon, cushaw, and other names for cultivated Cucurbita species. Cucurbita pepo, Cucurbita maxima, and Cucurbita moschata are the three most important domesticated Cucurbita species.[Citation3,Citation4]
The pumpkin is made up of several diverse elements that when combined produce a wonderful fruit. It contains peel, flesh, and seed, among other things. The skin of the pumpkin is referred to as the peel. The skin, often known as the rind, is the outermost coat of the pumpkin. This is a protective coating protects the fruit from insects and illness. The meat of the fruit is called as flesh, and it is utilized to prepare veggie dishes. The seeds are connected to the fibers in the middle of the pumpkin. The seeds are separated, dried, and consumed, or saved for the next harvest.[Citation5]
Pumpkins are an annual plant that can be found in temperate and subtropical climates.[Citation3] Ethiopia is located in the tropics, thus the weather is ideal for growing pumpkins. Fruit size, weight, shape, color, vine length and branching, leaf size, overall fruit subjective and chemical attributes, and seed size vary significantly between types. Pumpkins are widely grown in Ethiopia for its fruit, which is successfully and efficiently used for the stew preparation and consumption with Ethiopian traditional spongy thin-layer bread (injira) made from teff cereal grain.[Citation6]
Because of the world’s rapidly rising population and scarcity of food supplies, there has been a growing interest in identifying and utilizing new natural and readily available food materials. Pumpkin is one of these foods, and it has been the subject of numerous research in recent years.[Citation3] The widespread use of pumpkin in traditional treatments for a variety of diseases (antidiabetic, antihypertensive, antitumor, immunomodulation, antibacterial, antihypercholesterolemia, intestinal antiparasitic, anti-inflammation, antalgic) has created scientific interest in the plant.[Citation7] Pumpkin is rich in polysaccharides, amino acids,[Citation8] fatty acids,[Citation9] phenolic, flavonoid, carotenoid, and mineral[Citation1] components, as well as volatile chemicals.[Citation4,Citation10,Citation11]
Low molecular weight organic molecules with a significant vapor pressure under ambient circumstances are classified as volatile organic compounds.[Citation12] Plant volatile compounds are incredibly significant to the business and are used to make a variety of items, including foods, beverages, cosmetics, and perfumes. Volatiles, as food fragrances, contribute to the palatability and appreciation of foods, and are responsible for food flavor, along with sugars, organic acids, salts, and other components that activate taste receptors. Volatiles encourage insects to pollinate flowers and signal that fruit is ripe and ready for seed distribution in plants.[Citation12,Citation13] Esters, hydrocarbons, aldehydes, alcohols, sulfur containing compounds, terpenes, and their derivatives are the volatiles that give fruits and vegetables their distinct flavor. Terpenes,[Citation14] sulfur containing compounds,[Citation15–18] hydrocarbons,[Citation19] and alcohols,[Citation20–25] have anti-inflammatory, antibacterial, antiviral, antifungal, antimicrobial and anticancer properties.
For the extraction of volatile chemicals, various procedures are used, including hydrodistillation (HD), solvent extraction,,[Citation26,Citation27] static headspace (HS) extraction,[Citation11,Citation28] and supercritical fluid extraction (SFE).[Citation29] Solvent extraction, on the other hand, recovers nonvolatile chemicals, but the SFE, while dependable and effective, is confined to commercial applications due to its high cost. Static headspace (HS) analysis is a potentially quick way to extract volatiles that requires very little plant material, but it comes with a danger of cross-contamination and low sensitivity because the total recovery is only possible for highly volatile compounds. Because of its convenience of use, low cost, and ability to perform comprehensive extraction from the raw materials, hydrodistillation was chosen as the extraction method in this investigation.[Citation10]
Several investigations have shown that the volatile compound composition of the pumpkin varies greatly depending on different factors such as, portion of a fruit, cultivar, ripening stage, and geographical origin.[Citation30,Citation31] The volatile chemical components from the meat, seeds, and fibers of raw pumpkin (Cucurbita pepo) farmed in the United States were determined by Leffingwell et al.[Citation4] Dynamic headspace GC-MS studies were used to evaluate the volatile contents of raw pumpkin (Cucurbita pepo) different volatile components were discovered. The fibrous stringy and/or seedy regions yielded mostly (Z)-3-hexenol and 1-hexanol, whereas 2-propanol and dimethyl disulfide dominated the flesh portion. All samples contained significant amounts of dimethyl disulfide and dimethyl trisulfide. The volatile chemicals in the fruit, leaf, and seed of the pumpkin (Cucurbita maxima) growing in Serbia were determined by Velikovi et al.[Citation10] The Clevenger type apparatus was used to accomplish the hydrodistillation procedure, which was used to isolate volatiles. Techniques such as GC-MS were used to identify volatiles. Heneicosane was the most abundant volatile chemical in the fruit, followed by benzaldehyde, tricosane, eicosane, and pentacosane. (2E)-hexenal, nonadecane, benzaldehyde, and terpenes (E)-damascone and (E)-ionone were the most abundant on the leaf. Heptadecane, tetradecane, octadecane, hexadecane, tridecane, and benzaldehyde were the most prevalent volatiles in the seed. The study of volatile chemicals in the puree pumpkin (Cucurbita moschata) grown in Spain was reported by Parra et al.[Citation11] 1-Hexanol, 1-octen-3-ol, (E)-6-nonen-1-ol, (E,Z)-3,6-nonadien-1-ol, hexanal, (E)-2-hexenal, (E)-6-nonenal, (E)-1,6-undecadiene, β-cyclocitral, trans-geranyl acetone and β-ionone (6.2%) were the most prevalent volatile chemicals recovered in the headspace of pumpkin purée. The volatile compound profile of several cultivars of different parts pumpkin derived from diverse pumpkin sources shows substantial variances.
Despite the fact that extensive research on volatile compounds from various sections of pumpkin have been published, there is no any published data on the comparative study of volatiles from diverse parts of pumpkin (Cucurbita maxima) cultivated in Africa. As a result, the goal of this research is to accumulate data in order to understand more about the volatile compounds found in the flesh, peel, and seed portions of Ethiopian pumpkins, as well as to compare the results with those published from other countries. The analysis of volatiles in food is a crucial part of the food manufacturing process. It provides information on food quality and its relation to the preferences of consumers.
Materials and methods
Apparatus and instrument
Electronic balance (ARA520, OHAUS CORP, China), Clevenger apparatus, Agilent Technology 7820A GC system coupled with an Agilent Technology 5977E MSD were used.
Chemicals
n-Hexane (99%, GC-MS grade, Labachemic Pvt. Ltd, India) was used for the extraction of volatile compounds after hydro-distillation of pumpkin flesh, peel and seed samples. Anhydrous sodium sulfate (Techno, Pharmachem, Bahadurgarh, India) was used for drying the n-hexane extract.
Sample collection and preparation
The ripe pumpkins (Cucurbita maxima) were purchased from Addis Ababa (Ethiopia) local markets. All the pumpkins were first washed with water to remove any foreign material attached, and parts required for the study were carefully separated using a knife. The three parts (peel, flesh and seed) were cleaned properly from the fibrous material attached. All the three parts of pumpkin (Cucurbita maxima) were comminuted and passed through the screen of 6 mm holes.
Extraction of volatile compounds
Extraction of volatile compounds was done following a modified procedure described by Veličković et al.[Citation10] Hydro-distillation technique was used to extract pumpkin’s flesh, peel and seed volatile compounds. 500 g of flesh, 500 g of peel and 250 g of seed samples were separately placed in different 6 L round-bottom flask each filled with 2 L of distilled water. The flasks were connected to the Clevenger apparatus and heated using electric heating mantle. The extraction time was 5 h, after which no more volatile compounds were obtained. The obtained volatile compounds were extracted with n-hexane and dried over anhydrous Na2SO4. The solutions were filtered and stored at 4°C until GC-MS analysis. Each extract was analyzed three times under the same conditions.
Analysis of volatile compounds
GC-MS analysis was carried out on an Agilent 7820A GC system (USA) coupled with an Agilent Technology 5977E MSD equipped with an auto-sampler (USA). The chromatographic separation was carried out on a DB-1701 micro column (30 m length × 0.25 mm internal diameter, 0.25 μm film thickness) at pressure of 8 psi and a flow rate 1 mL/min. Ultra-high pure helium was used as the carrier gas at constant flow mode. An Agilent G4567A auto sampler was used to inject 1.0 μL of the sample with a split less injection mode into the inlet heated to 270°C in a total run time of 29.33 min. The oven temperature was programmed with the initial column temperature of 60°C for 2 min. The column temperature increased to 200°C at a rate of 10°C/min and, then heated again at rate of 3°C/min until the temperature reached 240°C. The transfer line and the ion source temperatures were 280°C and 230°C, respectively. The electron energy was 70 eV. Mass spectral data were collected in the range 40–650 m/z. The volatile compounds were identified by matching their mass spectra with those of reference compounds recorded in National Institute of Standards and Technology (NIST-14) mass spectral library. A homologous series of n-alkanes (C9–C30) were run under the same operating conditions as the volatile oils to determine relative indices (RIs). The relative content of each constituent was determined by area normalization.
Statistical analysis of data
All measurements were done in triplicates and results were presented as mean ± standard deviation (SD) (n = 3). The relative peak areas of the different compounds in each category were calculated as a ratio of the total peak area. Statistical analyses were conducted using Origin 6.0 (Microcal Software, Inc., Northampton, USA).
Results
Profiles of volatile compounds in flesh, peel and seed parts of pumpkin
The gas chromatographic analysis of the volatile compounds from flesh, peel and seed parts of a pumpkin (Cucurbita maxima) showed several peaks in the generated chromatograms (). These peaks represent the metabolites identified in the essential oils. The chromatographic analysis revealed 15, 23 and 28 compounds in flesh, peel and seed parts representing 81.5, 84.4, and 97.7% of the total volatile compounds, respectively. The area percentage covered by the individual peaks, their retention times, retention indices, chemical classes, relative matches and compositions of flesh, peel and seed parts of the pumpkin are presented in .
Figure 1. The chromatogram of volatile compounds detected in sample of A) flesh, B) peel and C) seed part of pumpkin (Cucurbita maxima).
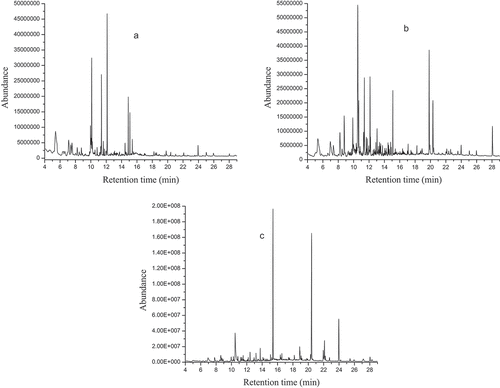
Table 1. List of volatile compounds identified in the flesh, peel and seed parts of pumpkin
The GC-MS analysis of the volatile compounds obtained from flesh, peel and seed parts of the pumpkin were composed of eight chemical classes: aldehydes, alcohols, hydrocarbons, esters, terpenes, sulfur containing compounds, ethers and pyridine ( and ). Three different portions of the pumpkin (Cucurbita maxima) were compared for volatile components ( and ). The study’s findings are compared to those found in the literature about pumpkin from different countries ().
Figure 2. Relative composition (%) of the chemical classes of volatiles in three parts of pumpkin (Cucurbita maxima). HD: hydrocarbon; ER: ester; ALD: aldehydes; ALC: alcohol; TRP: terpinene; SCC: sulfur containing compounds; ETR: ether; PYR: pyridine.
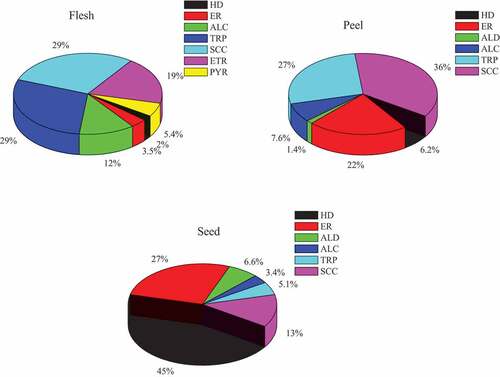
Figure 3. Correlation of volatile compounds in different parts of pumpkin (Cucurbita maxima) A) volatile compounds in flesh verses in peel, B) volatile compounds in flesh verses in seed and C) volatile compounds in peel versus in seed.
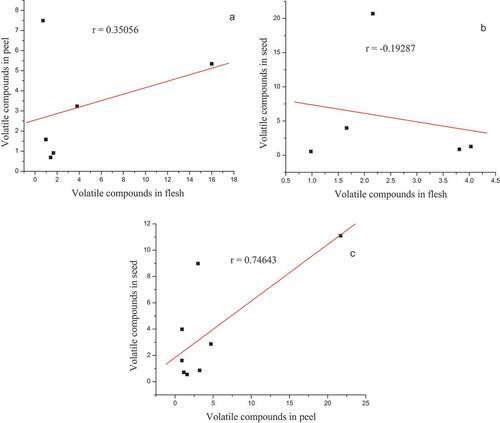
Table 2. Chemical classes occurring in the volatile compounds from different parts of pumpkin (Cucurbita maxima)
Table 3. Common volatile compounds shared among the flesh, peel and seed parts of pumpkin (Cucurbita maxima)
Table 4. Comparison of volatile components detected in different parts of pumpkin growing in Ethiopia with different countries
The volatile compounds from the pumpkin flesh were composed of 15 components, 81.5% of the total oil ( and ) and were quantitatively characterized, being formed by sulfur containing compounds (23.9%), terpenes (23.5%), ether (15.4%), alcohol (9.84%) and pyridine (4.4%) (). Main representatives of these groups were 2,6-dimethyl-5,6-dihydro-2H-thiopyran-3-carbaldehyde (16.0%), 6Z-nonen-1-ol (11.8%), 1-methylbutyl-oxirane (15.4%), 1-nonanol (4.03%) and 5-ethyl-2-methyl-pyridine (4.40%), respectively (). Ester (2.88%) and hydrocarbon (1.66%) were less abundant components in pumpkin flesh. The aldehyde was not detectable in this sample ().
There were 23 volatile compounds found in the pumpkin peel, accounting for 84.4% of the total ( and ). Sulfur containing compounds constituted the major content (30.3%) of the oil, followed by terpenes (22.9%), ester (18.3%), alcohol (6.42%) and hydrocarbon (5.26%) (). Main representatives of these groups were, 5,6-dihydro-2,4,6-trimethyl-4 H-1,3,5-dithiazine (21.7%), 4-isopropenyltoluene (4.81%), 1,2-benzenedicarboxylic acid, bis(2-methylpropyl) ester (7.49%), 2,4-di-tert-butylphenol (3.23%) and hexacosane (3.01%), respectively (). Aldehyde (1.15%) was less abundant components in the pumpkin peel. Ether and pyridine derivative were not detected in the pumpkin peel ().
A total of 28 volatile components were identified from the pumpkin seeds corresponding to 97.7% of the total composition ( and ). Hydrocarbons (43.6%) were the most prevalent chemical compounds in the pumpkin seed, followed by esters (26.4%), sulfur containing compounds (12.7%), aldehydes (6.48%), and terpenes (5.02%) (). Main representatives of these groups were heneicosane (26.1%), [2,2,4-trimethyl-3-(2-methylpropanoyloxy)pentyl]2-methylpropanoate (20.7%), 5,6-dihydro-2,4,6-trimethyl-4H-1,3,5-dithiazine (11.1%), 2-phenylacetaldehyde (1.56%), and eucalyptol (2.86%), respectively (). Alcohols (3.32%) were less abundant components in the pumpkin seeds. Ether and pyridine derivative were not detected in the pumpkin seeds ().
Volatile compounds common to flesh, peel and seed parts of pumpkin (Cucurbita maxima)
Different volatile compounds were identified and compared in the three parts of pumpkin. Among the total compounds found in the volatile oils, three volatile compounds, such as 2,4-ditert-butylphenol, 3-carene and eicosane, were found in all parts of the pumpkin. The flesh and peel of the pumpkin included six volatile compounds, the peel and seed were found to contain eight volatile compounds, and the flesh and seed sections of pumpkin included five volatile compounds ().
Correlation of the common volatile compounds between the flesh, peel and seed parts of pumpkin (Cucurbita maxima)
The relationship between common volatile chemicals in the three parts of the pumpkin was investigated. The correlation between the flesh and peel (r = 0.350), flesh and seed (r = −0.193), and peel and seed (r = 0.746) was determined using the Pearson correlation ().
Discussion
Several reports[Citation4,Citation10,Citation11] have been published on the identification and quantification of volatile chemicals in various parts of pumpkin from different countries. These studies have revealed that the volatile chemical composition of pumpkin varies substantially based on a variety of criteria including the parts of the fruit, cultivar, ripening stage, and geographical origin.[Citation30,Citation31] To the best of our knowledge there is no published data on the comparative examination of volatiles from various parts of pumpkin (Cucurbita maxima) cultivated in Africa. In the present study the composition of volatile compounds in the flesh, peel, and seed of pumpkin cultivated in Ethiopia were determined, and the results are compared to those previously reported from other countries.
compared the data of present study with those reported in the literature concerning pumpkin growing in United States, Serbia and Spain. It was noticed that there are similarities and differences in the chemical profiles. Volatile constituents were identified from the flesh, seeds, and fibers of raw pumpkin (Cucurbita pepo) farmed in the United States were determined by Leffingwell et al.[Citation4] All samples contained nonanal, β-cyclocitral and β-ionone which are consistent with those found in the present study ().
The volatile chemicals in the fruit, leaf, and seed of the pumpkin (Cucurbita maxima) growing in Serbia were determined by Veličković et al.[Citation10] Seven of which are consistent with those found in the present study such as heneicosane, eicosane, heptadecane, hexadecane, (E) β-damascone, benzaldehyde, β-cyclocitral and (E) β-ionone ().
Pumpkin (Cucurbita moschata) grown in Spain was analyzed by Parra et al.[Citation11] and different volatile compounds were identified in the pumpkin purée. (E)-6-Nonen-1-ol, (E,Z)-3,6-nonadien-1-ol, β-cyclocitral, trans-geranyl acetone and β-ionone are consistent with those found in the different parts of pumpkin of the present study (). As a result, some of the volatile chemicals found in this investigation matched with previous data from other countries.
In the present study eight chemical classes of volatile compounds were identified and compared in three parts of pumpkin. illustrates the variation in the level of chemical classes in the three parts of pumpkin. The volatile oils extracted from the seed were characterized by the predominance of hydrocarbons followed by esters and sulfur containing compounds. The volatile oils obtained from the peel and flesh of the pumpkin showed the predominance of sulfur containing compounds, followed by terpinenes. Alcohols were found higher in flesh followed by peel and seed parts of pumpkin. Ether and pyridine were only detected in flesh parts of the pumpkin. On the other hand, some amount of aldehydes were detected only in the peel and seed parts of pumpkin.
In the present study, identified volatiles accounted for 81.5, 84.4, and 97.7% of the total volatiles constituents in volatile oils of the flesh, peel, and seed of the pumpkin, respectively (). Compared with literature values, there are considerable differences in the number of identified volatiles compounds. Veličković et al.[Citation10] accounted 100, 98.6 and 100% of fruit, leaf and seed volatile oils, respectively of the pumpkin grown in Serbia. Thirty three compounds of the pumpkin puree were detected corresponding to 100.0% of the oil in the pumpkin cultivated in Spain.[Citation11] Marked quantitative and qualitative variations were observed by comparing the composition between the three volatile oils. indicates that hydrocarbon (45%), sulfur containing compounds (36%), and terpinene (29%) were the major chemical compounds for the seed, peel and flesh volatile oils, respectively.
In general, the results of the present study is in accordance with previous study conducted by Leffingwell et al.,[Citation4] who have identified hydrocarbons, aldehydes, alcohols, terpenes and sulfur containing compounds from the flesh, seeds and fibrous of raw pumpkin (Cucurbita pepo). Veličković et al.[Citation10] reported that the dominant component of the volatile compounds in the pumpkin (Cucurbita maxima) were hydrocarbons, aldehydes, alcohols and terpenes. Parra et al.[Citation11] have reported the changes in the volatile profile of a pumpkin (Cucurbita moschata). Alcohols, aldehydes, hydrocarbons, and terpenes were the volatile compounds most abundantly isolated in the headspace of pumpkin purée. Hasbullah et al.[Citation32] reported the chemical class of volatile compounds from melon (Cucumis melo L.), a member of the Cucurbitaceae plant family. The results showed that some identified aromatic volatile compounds consisted of alcohols, esters, ketones and hydrocarbons. According to literature, variation in volatile composition can be attributed to different factors as well as to different parts, stages of maturity, and growth.
The volatile components of three distinct sections of the pumpkin (Cucurbita maxima) were compared (). Some components were detected in all parts of the pumpkin, while others were only found in the remaining two parts, according to this research. Correlations of the common volatile compounds were assessed (). The common volatile chemicals found in pumpkin peel and seed portions show a strong and favorable relationship. The relationship between common volatile chemicals in pumpkin flesh and skin is both positive and weak. However, there is a negative and weak link between the common volatile chemicals found in pumpkin flesh and seed portions.
Volatile sulfur compounds were the most abundant chemical group of compounds in the pumpkin (Cucurbita maxima). Sulfur containing compounds were found (30.3%) in the peel, (23.7%) in flesh, and (12.7%) in the seed parts of pumpkin. In general, volatile sulfur compounds are natural compounds contributing the overall flavor and aroma of plant. Various volatile sulfur compounds may be present in foods naturally or as a result of processing and storage conditions. Common foods have antibacterial properties due to volatile sulfur compounds.[Citation15] Garlic (Allium sativum) and onion (Allium cepa) are two of the world’s oldest cultivated plants, rich in flavonols and natural organosulfur compounds. Their use was investigated because it was thought to be good for heart disease, malignancies (including esophageal, gastric, colorectal, breast, lung, skin, and prostate cancers), and immunological processes, among other things.[Citation16–18]
Volatile terpenes are another group of compounds found in pumpkin flesh, skin, and seeds that are likely formed as a result of carotenoids breakdown. Terpene volatile compounds were identified in pumpkin flesh (23.5%), peel (22.9%), and seed portions (5.02%). Terpenes, which are responsible for a wide range of pleasant notes, are significant components of the perfume of many fruits and vegetables.[Citation33]
α-Terpineol were detected in the peel parts of pumpkin in this study. α-Terpineol is a monocyclic monoterpene tertiary alcohol having a lilac-like fragrance. As a result, it is widely employed in the production of perfumes, cosmetics, soaps, and antibacterial agents, and is regarded as one of the most important chemicals.[Citation34] α-Terpineol also draws a lot of attention because it has a lot of biological applications as an antioxidant, anticancer, anticonvulsant, antiulcer, antihypertensive, and anti-nociceptive molecule. It also has insecticidal qualities and is used to improve skin penetration.[Citation35]
Several investigations have looked into the cardiovascular and antihypertensive effects of α-terpineol. These findings suggested that taking α-terpineol orally could lower mean arterial pressure and cause endothelium-independent vasodilation. Furthermore, in L-NAME-induced hypertension, α-terpineol was able to restore enzymatic antioxidant.[Citation36,Citation37] Furthermore, α-terpineol exhibited anti-proliferative (antioxidant) activity, which could be used in the prevention or even treatment of cancer, as it was discovered that α-terpineol exhibited a potential antioxidant capacity effect against a variety of human cancer cell lines (breast, lung, prostate, ovarian, and leukemia). α-Terpineol suppresses tumor cell development and induces cell death in tumor cells by inhibiting NF-B activity.[Citation38,Citation39]
α-Terpineol has also been shown to have antiulcer properties in several investigations.[Citation40,Citation41] In the pentobarbital-induced sleep test, α-terpineol also displayed anticonvulsant and sedative properties via a depressive action.[Citation42] Another significant biological activity of α-terpineol was its potential as a chemical skin penetration enhancer, which is thought to boost solubility within the stratum corneum or increase the lipid fluidity of intracellular bilayers.[Citation43,Citation44]
In addition, several scientists were intrigued by α-terpineol insecticidal properties. As a result, it has been claimed that α-terpineol may be used to generate natural insecticides or fumigants, as well as repellents for insect control.[Citation45–48] As a result of its use in the pharmaceutical and agricultural industries, α-terpineol has shown potential satisfaction in specific activities. α-Terpineol is a very promising candidate in pharmaceutical and agricultural applications, based on encouraging results from a wide range of biological activities.
β-Ionone is another terpene volatile organic compound, which is identified in the flesh and peel parts of pumpkin in this study. β-Ionone appears to be primarily involved in defense mechanisms in plants.[Citation49] Ansari et al.[Citation50] have examined the potential of β-ionone and similar molecules. Furthermore, various research have shown the particular features and prospective benefits of β-ionone in human health in terms of essential pharmacological activities, including antiinflammatory, cancer-prevention, antibacterial, antifungal, and antileishmanial.[Citation50–53]
According to the findings of the Asokkumar et al.[Citation54] study, dietary β-ionone has a potent potential to suppress benzo(a)pyrene-induced lung carcinogenesis, which is at least partly related to improved antioxidant enzymes and cell proliferation inhibition. β-Ionone has been shown to exhibit antitumor properties in melanoma, breast cancer, and chemical-induced rat carcinogenesis.[Citation55] In both in vitro and in vivo models, Xie et al.[Citation56] revealed that activating the prostate-specific G protein-coupled receptor with its ligand β-ionone can reduce prostate cancer cell proliferation.
Hydrocarbons were the most abundant chemical category of compounds, accounting for 43.6% in the seed and 5.26% in the peel and 1.66% in the flesh parts of pumpkin. In this investigation, heneicosane was identified in pumpkin seed portions (26.1%). According to Vanitha et al.[Citation19] heneicosane was discovered from P. zeylanica leaf extract and its microbicidal effectiveness was verified.
In this investigation, pumpkin alcohol was detected 9.84% in the flesh, 6.42% in the peel, and 3.32% in the seed sections. In addition, 2,4-ditertbutylphenol was found in all parts of the pumpkin. According to Varsha et al.[Citation22] the antifungal and antioxidant properties of 2,4 DTBP, which was isolated from a Lactococcus sp., show that this compound has the potential to be developed as a food additive that could improve food safety. 2,4-Ditertbutylphenol, a naturally occurring molecule, has been shown to have antibacterial,[Citation21] antifungal,[Citation22–24] and anticancer[Citation20,Citation25] properties. According to the findings of this study, the various portions of the pumpkin have more medicinal and nutritional values. In Ethiopia, most people discard the seeds and peels of pumpkins; nevertheless, this study discovered that the seeds and peels of pumpkins are rich in essential volatile compounds.
Conclusion
The volatile profile of pumpkin volatile oil and the specific features of separated volatile compounds from flesh, peel and seed parts were identified. The volatiles compounds in the pumpkin (Cucurbita maxima) were composed of 15 components in the flesh, 23 components in the peel, and 28 components in the seed parts. Hydrocarbons, sulfur containing compounds, terpienes and esters were amounted as the major chemical classes. Heneicosane, 5,6-dihydro-2,4,6-trimethyl-4H-1,3,5-dithiazine and 2,6-dimethyl-5,6-dihydro-2H-thiopyran-3-carbaldehyde were the main compounds in the seed, peel and flesh parts of the pumpkin, respectively. The results show that all parts of pumpkin (Cucurbita maxima) contains the different chemical classes of compounds. Some of the volatile compounds identified in this study were in agreement with published reports from different countries. Different volatile compounds discovered in various sections of the pumpkin signify its enhanced medicinal and nutritional values, according to the finding of this study.
Acknowledgments
The authors are grateful to the Department of Chemistry, College of Natural and Computational Sciences, Addis Ababa University, Addis Ababa, Ethiopia for proving laboratory facilities. Mulu Hagos is thankful to Ethiopian Police University, Ethiopia, for sponsoring her study.
Disclosure statement
No potential conflict of interest was reported by the author(s).
Additional information
Funding
References
- Hussain, A.; Kausar, T.; Din, A.; Murtaza, M. A.; Jamil, M. A.; Noreen, S.; Rehman, H.; Shabbir, H.; Ramzan, M. A. Determination of Total Phenolic, Flavonoid, Carotenoid, and Mineral Contents in Peel, Flesh, and Seeds of Pumpkin (Cucurbita Maxima). J. Food Process. Preserv. 2021, 45(6), 1–8. DOI: 10.1111/jfpp.15542.
- Verla, A. W.; Verla, E. N.; Adowei, P.; Briggs, A.; Awa, E.; Jnr, M. H.; Spiff, A. I. Preliminary Chemical Profile of Telfairia Occidentalis Hook. F (Fluted Pumpkin) Seed Shell. Merit Res. J. Environ. Sci. Toxicol. 2014, 2(4), 64–70.
- Kwiri, R.; Winini, C.; Musengi, A.; Mudyiwa, M.; Nyambi, C.; Muredzi, P.; Malunga, A. Proximate Composition of Pumpkin Gourd (Cucurbita Pepo) Seeds from Zimbabwe. Int. J. Nutr. Food Sci. 2014, 3(4), 279–283. DOI: 10.11648/j.ijnfs.20140304.17.
- Leffingwell, J. C.; Alford, E. D.; Leffingwell, D. Identification of the Volatile Constituents of Raw Pumpkin (Cucurbita Pepo L.) by Dynamic Headspace Analyse. Leffi. Rep. 2015, 7(1), 1–14.
- Republic of South Africa. Department of Agriculture, Forestry and Fisheries. Crops and Markets, Second Quarter. 2011, 92(948), 1–43.
- Kefale, B. Evaluation of Injera Prepared from Composite Flour of Teff and Barley Variety. J. Food Sci. Nutr. Ther. 2020, 6(1), 38–40.
- Badr, S. E. A.; Shaaban, M.; Elkholy, Y. M.; Helal, M. H.; Hamza, A. S.; Masoud, M. S.; El Safty, M. M. Chemical Composition and Biological Activity of Ripe Pumpkin Fruits (Cucurbita Pepo L.) Cultivated in Egyptian Habitats. J. Nat. Prod. Lett. 2011, 25(16), 1524–1539.
- Nourmohammadi, E.; Mahoonak, A. S.; Alami, M.; Ghorbani, M. Amino Acid Composition and Antioxidative Properties of Hydrolysed Pumpkin (Cucurbitapepo L.) Oil Cake Protein. Int. J. Food Prop. 2017, 20(12), 3244–3255. DOI: 10.1080/10942912.2017.1283516.
- Uba, B.; Muhammad, C. Determination of Fatty Acid Composition and Physicochemical Properties of Pumpkin (Cucurbita Maxima) Seed Oil Cultivated in Northeast Nigeria. East. Afr. Sch. Multi. Bull. 2019, 2(7), 230–234.
- Veličković, D. T.; Ristić, M. S.; Karabegović, I. T.; Stojičević, S. S.; Nikolić, N. C.; Lazić, M. L. Volatiles and Fatty Oil of Pumpkin (Cucurbita Maxima). Adv. Technol. 2015, 4(2), 43–48. DOI: 10.5937/savteh1502043V.
- Parra, J. G.; Cebrino, F. G.; Ramírez, R. Volatile Compounds of a Pumpkin (Cucurbita Moschata) Purée Processed by High Pressure Thermal Processing. Soc. Chem. Ind. 2020, 1–8. DOI: 10.1002/jsfa.10485.
- Rowan, D. D. Volatile Metabolites. Metabolites. 2011, 1(2011), 41–63. DOI: 10.3390/metabo101004.
- Olabode, O. O.; Arueya, G. L. Analysis and Identification of the Volatile Compounds in Melon-Bitter Leaf Soup. Afr. J. Food Sci. 2016, 10, 302–312. DOI: 10.5897/AJFS2016.1460.
- Bicas, J. L.; Molina, G.; Dionísio, A. P.; Barros, F. F. C.; Wagner, R.; Maróstica, M. R.; Pastore, G. M. Volatile Constituents of Exotic Fruits from Brazil. Food Res. Int. 2011, 44, 1843–1855. DOI: 10.1016/j.foodres.2011.01.012.
- Kyung, K. H. Antimicrobial Activity of Volatile Sulfur Compounds in Foods. Am. Chem.l Society. 1068, 2011, 323–338. DOI: 10.1021/bk-2011-1068.ch016.
- Melino, S.; Sabelli, R.; Paci, M. Allyl Sulfur Compounds and Cellular Detoxification System: Effects and Perspectives in Cancer Therapy. Amino Acids. 2011, 41, 103–112. DOI: 10.1007/s00726-010-0522-6.
- Antony, M. L.; Singh, S. V. Molecular Mechanisms and Targets of Cancer Chemoprevention by Garlic-Derived Bioactive Compound Diallyl Trisulfide. Indian J. Exp. Biol. 2011, 49(11), 805–816.
- Ohkubo, S.; Dalla Via, L.; Grancara, S.; Kanamori, Y.; García-Argáez, A. N.; Canettieri, G.; Arcari, P.; Toninello, A.; Agostinelli, E. The Antioxidant, Aged Garlic Extract, Exerts Cytotoxic Effects on Wild-Type and Multidrug-Resistant Human Cancer Cells by Altering Mitochondrial Permeability. Int. J. Oncol. 2018, 53, 1257–1268. DOI: 10.3892/ijo.2018.4452.
- Vanitha, V.; Vijayakumar, S.; Nilavukkarasi, M.; Punitha, V. N.; Vidhya, E.; Praseetha, P. K. Heneicosane A Novel Microbicidal Bioactive Alkane Identified from Plumbago Zeylanica L. Ind. Crop Prod. 2020, 154, 1–8. DOI: 10.1016/j.indcrop.2020.112748.
- Varsha, K. K.; Devendra, L.; Shilpa, G.; Priya, S.; Pandey, A.; Nampoothiri, K. M. 2,4-Di-Tert-Butyl Phenol as the Antifungal, Antioxidant Bioactive Purified from a Newly Isolated Lactococcus Sp. Int. J. Food Microbiol. 2015, 211, 44–50.
- Dehpour, A. A.; Yousefian, M.; Kelarijani, S. A. J.; Koshmoo, M.; Mirzanegad, S.; Mahdavi, V.; Bayani, M. J. J. Antibacterial Activity and Composition of Essential Oils of Flower Allium Rotundum. Adv. Environ. Biol. 2012, 6, 1020–1025.
- Dharni, S.; Sanchita, A. M.; Srivastava, S. K.; Srivastava, S. K.; Patra, D. D.; Patra, D. D.; Patra, D. D. Purification, Characterization, and in Vitro Activity of 2,4-Di- Tert -butylphenol from Pseudomonas Monteilii PsF84: Conformational and Molecular Docking Studies. J. Agric. Food Chem. 2014, 62, 6138–6146. DOI: 10.1021/jf5001138.
- Rangel-Sánchez, G.; Castro-Mercado, E.; García-Pineda, S. Avocado Roots Treated with Salicylic Acid Produce Phenol‑2,4‑Bis(1,1‑Dimethylethyl), a Compound with Antifungal Activity. J. Plant Physiol. 2014, 171, 189–198. DOI: 10.1016/j.jplph.2013.07.004.
- Sang, M. K.; Kim, J. D.; Kim, B. S.; Kim, K. D. Root Treatment with Rhizobacteria Antagonistic to Phytophthora Blight Affects Anthracnose Occurrence, Ripening, and Yield of Pepper Fruit in the Plastic House and Field. Phytopathology. 2011, 101, 666–678. DOI: 10.1094/PHYTO-08-10-0224.
- Sathuvan, M.; Vignesh, A.; Thangam, R.; Palani, P.; Rengasamy, R.; Murugesan, K. In Vitro Antioxidant and Anticancer Potential of Bark of Costus Pictus D. Don. Asian Pac. J. Trop. Biomed. 2012, 2, S741–S749. DOI: 10.1016/S2221-1691(12)60307-4.
- Engel, E.; Baty, C.; Corre, D. L.; Souchon, I.; Martin, N. Flavor Active Compounds Potentially Implicated in Cooked Cauliflower Acceptance. J. Agric. Food Chem. 2002, 50, 6459–6467. DOI: 10.1021/jf025579u.
- Xie, Z.; Liu, Q.; Liang, Z.; Zhao, M.; Yu, X.; Yang, D.; Xu, X. The Gas Chromatography Mass Spectroscopy Analysis of Volatile Components Extracted by Different Methods from Exocarpium Citri Grandis. J. Anal. Methods Chem. 2013, 1–8. DOI: 10.1155/2013/918406.
- Farouk, A.; Ali, H.; Al-Khalifa, A. R.; Mohsen, M.; Fikry, R. Aroma Volatile Compounds of Parsley Cultivated in the Kingdom of Saudi Arabia and Egypt Extracted by Hydrodistillation and Headspace Solid-Phase Microextraction. Int. J. Food Prop. 2017, 20(3), 2868–2877. DOI: 10.1080/10942912.2017.1381707.
- Barroso, M. S. T.; Villanueva, G.; Lucas, A. M.; Perez, G. P.; Vargas, R. M. F.; Brun, G. W.; Cassel, E. Supercritical Fluid Extraction of Volatile and Non-volatile Compounds from Schinus Molle. Braz. J. Chem. Eng. 2011, 28(2), 305–312. DOI: 10.1590/S0104-66322011000200015.
- Si, L.; Chen, Y.; Han, X.; Zhan, Z.; Tian, S.; Cui, Q.; Wang, Y. Chemical Composition of Essential Oils of Litsea Cubeba Harvested from Its Distribution Areas in China. Molecules. 2012, 17, 7057–7066. DOI: 10.3390/molecules17067057.
- Mugao, L. G.; Gichimu, B. M.; Muturi, P. W.; Mukono, S. T.; Zheng, D. Characterization of the Volatile Components of Essential Oils of Selected Plants in Kenya. Biochem. Res. Int. 2020, 2020, 1–8. DOI: 10.1155/2020/8861798.8861798.
- Hasbullah, U. H.; Supriyadi, A., and Daryono, B. S. Aroma Volatile Compounds Profile of Melon (Cucumis Melo L.) Gama Melon Parfum. Ear. Enviro. Sci. 2019, 292, 012027.
- Jiang, Y.; Song, J. Fruits and Fruit Flavor: Classification and Biological Characterization. In Handbook of Fruit and Vegetable Flavors; By Hui, Y. H., Ed.; John Wiley and Sons: Hoboken, NJ, 2010; pp 3–23.
- Bauer, K.; Garbe, D.; Surburg, H. Common Fragrance and Flavor Materials: Preparations, Properties, and Uses, 4th; Wiley: New York, 2001; pp 1–299.
- Bhatia, S. P.; Letizia, C. S.; Api, A. M. Fragrance Material Review on Alpha-Terpineol. Food Chem. Toxicol. 2008, 46(11), 280–285. DOI: 10.1016/j.fct.2008.06.027.
- Sabino, C. K.; Ferreria-Filho, E. S.; Mendes, M. B.; Da-SilvaFilho, J. C.; Ponte, M. P. T. R.; Moura, L. H. P.; Oliveira, E. C. A.; Quintans-Junior, L. J.; Dos Santos, M. R. V.; de Cássia Meneses Oliveira, R. Cardiovascular Effects Induced by Alpha-Terpineol in Hypertensive Rats. Flavour Frag. J. 2013, 28(5), 333–339. DOI: 10.1002/ffj.3159.
- Ribeiro, T. P.; Porto, D. L.; Menezes, C. P.; Antunes, A. A.; Silva, D. F.; De Sousa, D. P.; Nakao, L. S.; Braga, V. A.; Medeiros, I. A. Unravelling the Cardiovascular Effects Induced by alpha-Terpineol: A Role for the Nitric Oxide–Cgmp Pathway. Clin. Exp. Pharmacol. Physiol. 2010, 37(8), 811–816. DOI: 10.1111/j.1440-1681.2010.05383.x.
- Hassan, S. B.; Muhtasib, H. G.; Goeransson, H.; Larsson, R. Alphaterpineol: A Potential Anticancer Agent Which Acts through Suppressing NF-Κb Signaling. Anticancer Res. 2010, 30(6), 1911–1920.
- Bicas, J. L.; Neri-Numa, I. A.; Ruiz, A. L.; De Carvalho, J. E.; Pastore, G. M. Evaluation of the Antioxidant and Antiproliferative Potential of Bioflavors. Food Chem. Toxicol. 2011, 49(7), 1610–1615. DOI: 10.1016/j.fct.2011.04.012.
- Souza, R. H.; Cardoso, M. S.; Menezes, C. T.; Silva, J. P.; De Sousa, D. P.; Batista, J. S. Gastroprotective Activity of alph-Terpineol in Two Experimental Models of Gastric Ulcer in Rats. DARU J. Pharm. Sci. 2011, 19(4), 277–281.
- Matsunaga, T.; Hasegawa, C.; Kawasuji, T.; Suzuki, H.; Saito, H.; Sagioka, T.; Takahashi, R.; Tsukamoto, H.; Morikawa, T.; Akiyama, T. Isolation of the Antiulcer Compound in Essential Oil from the Leaves of Cryptomeria Japonica. Biol. Pharm. Bull. 2000, 23(5), 595–598. DOI: 10.1248/bpb.23.595.
- De Sousa, D.; Raphael, E.; Brocksom, U.; Brocksom, T. Sedative Effect of Monoterpene Alcohols in Mice: A Preliminary Screening. Z. Naturforsch. C. 2007, 62(7–8), 563–566. DOI: 10.1515/znc-2007-7-816.
- Songkro, S. An Overview of Skin Penetration Enhancers: Penetration Enhancing Activity, Skin Irritation Potential and Mechanism of Action. Songklanakarin J. Sci. Technol. 2009, 31(3), 299–321.
- Patil, U. K.; Saraogi, R. Natural Products as Potential Drug Permeation Enhancer in Transdermal Drug Delivery System. Arch. Dermatol. Res. 2014, 306(5), 419–426. DOI: 10.1007/s00403-014-1445-y.
- Tong, F.; Coats, J. R. Quantitative Structure–Activity Relationships of Monoterpenoid Binding Activities to the Housefly GABA Receptor. Pest. Manag. Sci. 2012, 68, 1122–1129. DOI: 10.1002/ps.3280.
- Pandey, S. K.; Tandon, S.; Ahmad, A.; Singh, A. K.; Tripathi, A. K. Structure-Activity Relationships of Monoterpenes and Acetyl Derivatives against Aedes Aegypti (Diptera: Culicidae) Larvae. Pest. Manag. Sci. 2013, 69(11), 1235–1238. DOI: 10.1002/ps.3488.
- Liu, Z. L.; Zhao, N. N.; Liu, C. M.; Zhou, L.; Du, S. Identification of Insecticidal Constituents of the Essential Oil of Curcuma Wenyujin Rhizomes Active against Liposcelis Bostrychophila Badonnel. Molecules. 2012, 17(10), 12049–12060. DOI: 10.3390/molecules171012049.
- Liu, X. C.; Li, Y. P.; Li, H. Q.; Deng, Z. W.; Zhou, L.; Liu, Z.; Du, S. Identification of Repellent and Insecticidal Constituents of the Essential Oil of Artemisia Rupestris L. Aerial Parts against Liposcelis Bostrychophila Badonnel. Molecules. 2013, 18, 10733–10746. DOI: 10.3390/molecules180910733.
- Havaux, M. Carotenoid Oxidation Products as Stress Signals in Plants. Plant J. 2014, 79, 597–606. DOI: 10.1111/tpj.12386.
- Ansari, M.; Emami, S. Βeta-Ionone and Its Analogs as Promising Anticancer Agents. Eur. J. Med. Chem. 2016, 123, 141–154. DOI: 10.1016/j.ejmech.2016.07.037.
- Balbi, A.; Anzaldi, M.; Mazzei, M.; Miele, M.; Bertolotto, M.; Ottonello, L.; Dallegri, F. Synthesis and Biological Evaluation of Novel Heterocyclic Ionone-Like Derivatives as Anti-Inflammatory Agents. Bioorg. Med. Chem. 2006, 14, 5152–5160. DOI: 10.1016/j.bmc.2006.04.007.
- Dong, H. W.; Wang, K.; Chang, X. X.; Jin, F. F.; Wang, Q.; Jiang, X. F.; Liu, J. R.; Wu, Y. H.; Yang, C. Beta-Ionone-Inhibited Proliferation of Breast Cancer Cells by Inhibited COX-2 Activity. Arch. Toxicol. 2019, 93, 2993–3003. DOI: 10.1007/s00204-019-02550-2.
- Sharma, V.; Singh, G.; Kaur, H.; Saxena, A. K.; Ishar, M. P. S. Synthesis of β-Ionone Derived Chalcones as Potent Antimicrobial Agents. Bioorg. Med. Chem. Lett. 2012, 22, 6343–6346. DOI: 10.1016/j.bmcl.2012.08.084.
- Asokkumar, S.; Naveenkumar, C.; Raghunandhakumar, S.; Kamaraj, S.; Anandakumar, P.; Jagan, S.; Devaki, T. Antiproliferative and Antioxidant Potential of Beta-Ionone against Benzo (A) Pyrene-Induced Lung Carcinogenesis in Swiss Albino Mice. Mol. Cell. Biochem. 2012, 363, 335–345. DOI: 10.1007/s11010-011-1186-6.
- Liu, J. R.; Sun, X. R.; Dong, H. W.; Sun, C. H.; Sun, W. G.; Chen, B. Q.; Song, Y. Q.; Yang, B. F. Βeta-Ionone Suppresses Mammary Carcinogenesis, Proliferative Activity and Induces Apoptosis in the Mammary Gland of the Sprague-Dawley Rat. Int. J. Oncol. 2008, 122, 2689–2698.
- Xie, H.; Liu, T.; Chen, J.; Yang, Z.; Xu, S.; Fan, Y.; Zeng, J.; Chen, Y.; Ma, Z.; Gao, Y. Activation of PSGR with Βeta-Ionone Suppresses Prostate Cancer Progression by Blocking Androgen Receptor Nuclear Translocation. Cancer Lett. 2019, 453, 193–205. DOI: 10.1016/j.canlet.2019.03.044.