ABSTRACT
Fresh rhizome extracts of four elite Zingiber species, viz., Zingiber kangleipakense, Zingiber montanum, Zingiber officinale, and Zingiber zerumbet, prepared in 60% aqueous ethanol/60% aqueous methanol solutions were used for assessing their comparative free radical scavenging and radioprotective properties. Various methods, such as ferric ion reducing power assay, DPPH ion scavenging assay, nitric oxide scavenging assay, metal chelating assay, thiyl/sulfur free radical reactivity assay, and hydroxyl ion scavenging assay, were employed for radical scavenging capabilities. Bio-active principles, such as total phenolic, flavonoid, and ascorbic acid contents, were also assessed. Z. officinale showed the highest TPC, TFC, and ascorbic acid content of 650 mg GAE/100 g, 889 mg QE/100 g, and 3.19 mg/100 g, respectively, as well as the greatest efficacy in ferric ion reducing and DPPH scavenging ability having lowest IC50 value of 0.22 mg/mL. Z. montanum showed the lowest IC50 value of 0.91 mg/mL in NO scavenging assay and also exhibited the highest curcumin protection in thiyl free radical scavenging assay. Z. kangleipakense showed the lowest IC50 value of 0.24 mg/mL and 0.25 mg/mL in metal ion chelating assay and hydroxyl radical assay, respectively. 60% aqueous ethanol extracts of Z. officinale and Z. kangleipakense were used for radioprotective assays both for in vitro and in vivo using plasmid pBR322 DNA and bone marrow cells of albino rats, respectively, based on their significant free radical scavenging properties. At the same concentration of 0.25 mg/mL, Z. officinale and Z. kangleipakense showed 71.50% and 56.54% protection, respectively, of DNA from exposure to 6 Gy gamma radiation. Also, Z. officinale and Z. kangleipakense exhibited 51.16% and 38.96% protection from radiation-induced chromosomal damages in rats, respectively, when exposed to 2 Gy. Reduction in the DNA open circular form as well as the chromosomal aberrations in treatments with plant extracts compared to the controls confirmed the radioprotective potentials of the extracts. The results showed that the extracts possessed potential antioxidant compounds having capacities to scavenge radiation-induced free radical species. The accessibility of the extracts in radiation countermeasures can provide immense nutraceutical potentials.
Introduction
In recent years, there has been an intense and great deal of attention toward free radical and antioxidant research. During normal metabolic process as well as exposures to environmental pollutants, cosmic and ionizing radiations, free radicals are generated in the cellular milieu. However, increased production of free radicals, in particular reactive oxygen species (ROS), leads to unfavorable balancing with antioxidant defense molecules, such as superoxide dismutase (SOD), catalase (CAT), glutathione peroxidase (GPx) and glutathione reductase (GRx), thereby generating oxidative stress,[Citation1,Citation2] which is associated with a destruction in a wide range of biological molecules including lipids, proteins, and nucleic acids,[Citation3,Citation4] eventually leading to the development of a variety of human diseases, such as cancer, coronary heart diseases, diabetes, atherosclerosis, premature aging, acquired immunodeficiency syndrome, hypertension, gastric ulcers, Alzheimer’s disease, Parkinson’s disease, muscular dystrophy, inflammatory diseases, alcoholism, smoking related diseases, and so on.[Citation5,Citation6] A balance between the antioxidant pool and the free radicals generated is necessary to have a well-maintained physiological function.
Plants are regarded as natural sources of antioxidants. Various synthetic antioxidant compounds, such as butylated hydroxyanisole (BHA) and butylated hydroxytoluene (BHT) used in food, therapeutic, and cosmetic industries, are reported to have toxic and carcinogenic effects.[Citation7] Indiscriminate use of synthetic drugs and antibiotics leads to acute toxicity and health hazards. Various plant secondary metabolites act as antioxidant compounds. Several bio-active molecules or active principles found in Zingiber species include gingerols cassuminins, cassuminarins, cassuminoids, etc. 6-gingerol is the major bioactive constituent present in ginger. It can effectively scavenge hydroxyl radicals and protect DNA from severe oxidative damage.[Citation8] One important polyphenolic compound present in Curcuma longa and some of the other Zingiber species is curcumin, which is a potent free radical scavenger reported to have chain-breaking ability or inhibit lipid peroxidation.[Citation9] It can protect cells from gamma radiation-induced damage by regulating the expression of p53 gene[Citation10] and by promoting transcription and expression of Nrf2 gene.[Citation11] Radiation-induced inflammatory responses can be suppressed using dietary flavonoids, pyranoanthocyanins, and other poylphenols.[Citation12,Citation13]
Plants play important roles in the introduction of new therapeutic medicines and drugs for prevention and treatment of various dreaded diseases and anomalies. A variety of plants including the medicinal ones are luxuriantly grown in Manipur, which lies within the Indo-Burmese mega-biodiversity ‘hot spot’ region.[Citation14] Among them, members of the ginger family are widely and wildly grown in Manipur, which are popularly known for their medicinal values since times immemorial. An attempt has been made to evaluate free radical scavenging activities of four Zingiber species in the present investigation focusing on the screening of free radical scavenging abilities of Z. kangleipakense Kishor & Škorničk, Z. montanum (J.König) A. Dietr., Z. officinale Rosc. and Z. zerumbet (Linn.) Rosc. ex Smith, and radioprotective properties of the most efficient free radical scavengers.
Materials and methods
Chemicals and reagents
Ascorbic acid, agarose, curcumin, DPPH (2,2-diphenyl-1-picrylhydrazyl), ethidium bromide, Folin-Ciocalteau reagent, gallic acid, glutathione, pyrogallol, quercetin, trichloroacetic acid (TCA), and trizma base were purchased from Sigma Aldrich, St. Louis, USA. Ferrous sulfate, ferric chloride, ferrozine, sodium salicylate, metaphosphoric acid, potassium hexacyanoferrate, Na2EDTA, and microscopic slides were obtained from Hi-media, India. Plasmid pBR322 DNA and other chemicals and reagents of analytical grade were procured from Merck, India.
Plant samples
The four plant samples, viz., Zingiber kangleipakense Kishor & Škorničk (holo CAL, iso SING, iso Herbarium of Institute of Bioresources & Sustainable Development, Imphal, Kishor 9), Zingiber montanum (J.König) A. Dietr. (MU/LSD/Herb.32), Zingiber officinale Rosc. (MU/LSD/Herb. 33), and Zingiber zerumbet (Linn.) Rosc. ex Smith (MU/LSD/Herb. 34) as shown in were collected from their natural habitats from various parts of Manipur. They were planted in the Experimental Garden of Manipur University, Imphal, India. Healthy and fresh rhizomes of the plant samples were collected during winter season when their leaves were all shed. They were used for the preparation of crude extracts.
Preparation of plant extracts
Fresh, healthy, and matured rhizomes were used for the preparation of crude extracts. One gram dry weight equivalent of fresh rhizomes relative to moisture content was ground using mortar and pestle. Then, the pastes were treated with 10 mL of 60% aqueous methanol or 60% aqueous ethanol and homogenized separately for each of the extracts. The homogenates were collected and centrifuged at 3000 x g for 10 min to get a clear supernatant. Finally, the clear supernatant was decanted and filtered through Whatman No. 1 filter paper and stored at 4°C for experimental procedures. Fresh rhizome extracts, of which dry weight equivalent relative to moisture content was taken into account, were used in the present study because drying leads to the loss of antioxidant quality.[Citation15]
Screening of antioxidant properties
Antioxidant properties of the plant extracts were evaluated through their ability to inhibit and scavenge free radicals using various in vitro test systems (ferrous ion reducing power assay, DPPH free radical scavenging assay, metal chelating assay, nitric oxide scavenging assay, thiyl free radical and hydroxyl radical scavenging assay, and antioxidant components, such as total phenol, total flavonoid, and ascorbic acid contents) by the following methods.
Ferric ion reducing power assay
Ferric ion reducing power of rhizome extracts was determined according to the method of Oyaizu with slight modification.[Citation16] Equal volumes each of 0.75 mL of various concentration of the 60% aqueous methanol extracts of plant samples (1.66, 3.33, 6.33, 8.33 and 10 mg/mL) were mixed with 0.75 mL of phosphate buffer (0.2 M, pH 6.6) and 0.75 mL of potassium hexacyanoferrate (1%, w/v), followed by incubation at 50°C for 20 min in a water bath. Then, the reaction was made to stop by adding 0.75 mL of trichloroacetic acid (TCA) solution (10%) followed by centrifugation at 3000 x g for 10 min. Then, each of 1.5 mL of the supernatants was mixed with 1.5 mL of distilled water and 0.1 mL of ferric chloride (FeCl3) solution (0.1%, w/v) and kept for 10 min at room temperature. The absorbance of the resulting solutions was measured at 700 nm, and higher the absorbance value, the greater is the reducing power. The experiments were carried out three times, and the mean of absorbance was plotted against the concentration followed by linear regression analysis.
DPPH free radical scavenging activity
DPPH assay was carried out to assess the free radical scavenging capacity of the plant extracts with slight modificatio.[Citation17] Each reaction mixture consisted of 100 µM DPPH (2, 2-diphenyl-1-picrylhydrazyl) in absolute methanol with different concentrations of 60% methanol extracts of the four sample. Absorbance was read against a blank at 515 nm after incubation of the reaction mixtures for 30 min in dark at room temperature. The percentage of DPPH decoloration was calculated as follows:
DPPH decoloration (%) = ([Abscontrol-Abssample] /Abscontrol) x 100
where Abscontrol was absorbance value of control and Abssample was absorbance value in the presence of extract. The assay was carried out in triplicates and the results were expressed as mean ± standard error. The degree of decoloration of purple colored DPPH to yellow color signifies the free radical scavenging capacity of the extract. Ascorbic acid was used as standard compound. The IC50 value was determined from the DPPH decoloration curve.
Nitric oxide scavenging assay
Determination of nitric oxide scavenging activity was done according to the method reported by Garratt with minor modification.[Citation18] Different concentrations of 0.5 mL of 60% aqueous methanol extracts were mixed with 2 mL of 10 mM sodium nitroprusside in 0.5 mL phosphate buffer saline (pH 7.4). The reaction mixture was then incubated at 25°C for 150 min and from that, and 0.5 ml was taken out and added into 1 mL sulfanilic acid reagent (0.33% in 20% gacial acetic acid). Then, they were kept at room temperature for 5 min. Finally, 1 ml naphthylethylenediamine dihydrochloride (0.1% w/v) was mixed and incubated at room temperature for another 30 min. Absorbance was measured at 540 nm using a spectrophotometer. Nitric oxide radical scavenging activity was calculated using the following formula:
Inhibition (%) = [Abscontrol-Abssample] /Abscontrol x 100
where Abscontrol was absorbance of the control (blank) and Abssample was absorbance of plant extract. The IC50 value was determined from the inhibition curve of NO scavenging assay.
Metal chelating assay
The ferrous ion chelating ability of plant extracts were monitored by measuring the formation of the ferrous ion-ferrozine complex.[Citation19] The reaction mixture containing 1 mL of various concentrations of the 60% aqueous methanol extracts (in triplicates) was mixed with 1 mL of 0.1 mM ferrous sulfate and 1 mL of 0.4 mM ferrozine to initiate the reaction. The reaction mixtures were shaken vigorously and left to stand for 10 min at room temperature. Absorbance was measured at 562 nm against a blank containing 60% methanol in place of ferrozine and control consisted of 60% methanol in place of extract. The ability of sample to chelate ferrous ion was calculated as follows:
Chelating effect (%) = [Abscontrol-Abssample] /Abscontrol x 100
where Abscontrol was absorbance value of control and Abssample was absorbance value in the presence of extract. The IC50 value was determined from the metal chelating curve.
Thiyl free radical reactivity assay
Thiyl free radical reactivity assay was carried out by methods described elsewhere,[Citation20] and glutathione was used for the in vitro generation of thiyl free radicals following gamma radiation and curcumin as a reference for assessing the reactivity with free radicals.[Citation21,Citation22] The reaction solution contained 20 µM curcumin in methanol and 15 mM glutathione in distilled water. In this experiment, different volumes of 0.4 and 0.8 mL of the 60% methanol extracts (Z. kangleipakense: 8 and 16 mg/mL; Z. montanum 15x dilution: 0.528 and 1.056 mg/mL; Z. officinale: 8 and 16 mg/mL and Z.zerumbet 2x dilution: 4 and 8 mg/mL), respectively, were added to the working solution and final volumes were adjusted at 5 mL with 60% methanol. The control solution contains 20 µM curcumin and 15 mM glutathione without sample. Then, the solutions were irradiated with 0, 50, 100, 150, 200, 250, and 300 Gy, respectively, at a dose,rate of 0.84 Gy/sec using a 60Co Gamma Irradiator GC-5000 (BRIT, Bhabha Atomic Research Center, Mumbai). The whole reaction mixtures were kept away from light. The thiyl free radicals generated by glutathione following gamma radiation could deplete the curcumin molecules in the control. Curcumin protection by different samples was determined by observing the absorbance at 425 nm using Beckman DU-640 UV/VIS Spectrophotometer against methanol as blank.
Curcumin depletion (µM) = (Initial curcumin content – Final curcumin content)
Hydroxyl free radical scavenging assay
Hydroxyl free radical scavenging activity of plant extracts were assessed using the method of Smirnoff and Cumbes[Citation23] with slight modification. In this method, 1 mL of 1.5 mM FeSO4, 0.7 mL of 6 mM hydrogen peroxide, 0.3 mL of 20 mM sodium salicylate, and varied concentrations of plant extracts prepared in 60% aqueous methanol were mixed together, and then the total volume was adjusted at 3 mL, the mixture was incubated at 37°C for 1 h, and at 526 nm, absorbance value of the hydoxylated salicylate complex was measured. The hydroxyl radical scavenging effect was calculated using the following formula:
Inhibition (%) = [1-(Abs1-Abs2 /Abs0)]x 100
where Abso was absorbance of the control without extract, Abs1 was absorbance of plant extract, and Abs2 was absorbance of the control without sodium salicylate. The IC50 value was determined from the inhibition curve of hydroxyl radical scavenging assay.
Total phenolic content
The amount of total phenolics in the plant extracts were determined using Folin-Ciocalteu phenol reagent,[Citation24] with slight modification. Equal quantities of 0.4 mL of 60% aqueous methanol extract were mixed with 0.3 mL of Folin-Ciocalteu reagent (10 x dilutions). Then, 0.25 mL of sodium carbonate (7.5% w/v) was added after 3 min and total volume was made up to 4 mL with distilled water. The reaction solution was kept in dark for 30 min. Absorbance was measured at 765 nm against a blank. The experiment was carried out in triplicates, and the amount of total phenolic content was calculated as gallic acid equivalent (GAE) in mg/100 g. The concentration range of gallic acid used for standard curve was 2–10 µg/mL. Equation of the gallic acid curve was found to be y = 0.095x + 0.004, R2 = 0.998.
Total flavonoid content
Total flavonoid content of the plant extracts were determined using the colorimetric method[Citation25] with minor modification. Equal quantities of 0.5 mL of rhizome extracts prepared in 60% aqueous methanol were mixed with 2 mL of 30% methanol and 0.15 mL of 5% NaNO2, and then after 6 min, 0.15 mL of 10% Al (NO3)3 was added. Subsequently, 2 mL of 4% NaOH and 0.2 mL of 30% methanol were added to the reaction mixture again after 6 min and mixed thoroughly. Absorbance was recorded at 510 nm following an incubation of 12 min against a blank. All experiments were carried out in triplicates and expressed as quercetin equivalent (QE) in mg/100 g of the extracts. The equation of calibration curve was y = 0.008x – 0.0094, R2 = 0.998.
Ascorbic acid content
Ascorbic acid content of the plant sample was estimated as described by the method of Klein and Perry[Citation26] with slight modification. First, 100 mg rhizome extracts were treated with 2 mL of 1% metaphosphoric acid and filtered. Then, the filtrates were mixed with 9 mL of 2, 6-dichloroindophenol (0.15 mg/mL), and absorbance was measured at 515 nm. Ascorbic acid content of the plant sample was calculated on the basis of the calibration curve and was expressed in mg/100 g. The concentration range of ascorbic acid used for standard curve was 0.3–3.5 µg/mL.
Radioprotection assays
Experiments for both in vitro protection of DNA damage induced by gamma radiation and in vivo protection of whole body irradiated albino rats (Rattus norvegicus albus, 2 n = 42) using 60% ethanol rhizome extracts of Z. kangleipakense and Z. officinale were carried out. Cytogenetic analysis was done to assess the radioprotective properties. Radioprotection assay in plasmid pBR322 DNA was done by following the methods published elsewhere.
Assessment of radiation-induced DNA damage and protection
Plasmid pBR322 DNA was used to evaluate protection of gamma radiation-induced DNA damage.[27] The DNA (300 ng) was exposed to 6 Gy at a dose rate of 0.01 Gy/sec in the absence and presence of plant extracts at different concentration. DNA solution without the sample and radiation was taken as control. After irradiation, the supercoiled (ccc) and open circular (oc) forms of DNA were separated by agarose gel electrophoresis in 1% gel agarose in Tris acetic acid EDTA buffer, pH 8 at constant voltage. The DNA bands stained with ethidium bromide were documented using Vilber Lourmat Gel Documentation Unit and bands were analyzed using PerkinElmer-Gene Tool Software, Version-3.07. Radiation-induced DNA damage was estimated as an increase in percentage of oc form of DNA.
Establishment of radioprotection in vivo
Animal model for assessment of radioprotection
Albino rat (Rattus norvegicus albus) was used as an in vivo model for assessing the radioprotective properties of Z. kangleipakense and Z. officinale. In vivo experiments were carried out with the approval of the Institutional Animal Ethics Committee, Manipur University (No. MU/3-37/IACE/Aca/14 dated 18.02.2016) and well maintained as per the guidelines. Male rats weighing about 120–150 g, aged 3–4 months were kept in polypropylene metabolic cages bedded with sanitized rice husk and maintained at about 25°C ± 2 in the animal house under 12-hr light and dark cycles. The animals were allowed to acclimatize in the laboratory environment for at least seven days before starting the experiment. Water was provided ad libitum and standard rat feed pellets from the Adilaid Foods & Feeds Pvt. Ltd. Mumbai, containing soyabean meal, wheat, grown corn as major ingredients, were given. At the time of dosing, rats were made to fast overnight but provided free access to water. Oral administration of crude rhizome extracts prepared in 60% ethanol was made.
Acute toxicity test of 60% ethanol extracts of Z. kangleipakense and Z. officinale
For the determination of acute toxicity of rhizome extracts of Z. kangleipakense and Z. officinale, animals aged 3–4 months, weighing 120–150 g, were divided into five groups with five animals each. The experimental and control groups were maintained with or without extract dose of 0.5, 1.5, 3, and 4.5 g/kg body weight, respectively. The animals were kept under constant observation for 30 days and mortality was observed every 24 hr for calculation of LD50 and MTD. Dose administration to rat was done by selecting a suitable dose for both plant extracts to be used in radioprotection experiments. In the present acute toxicity test, there was no mortality of animals with oral administration of up to 4.5 g/kg body weight of the plant extracts within 30 days of observation due to the toxicity of the extracts. There were no significant changes in the body weight and food intake. From the observation, it can be assumed that there was no toxicity at the administered doses of the plant extracts and LD50 would be higher than 2 g/kg body weight of animals. Based on the result, a single extract dose of 1 g/kg body weight was used as safe dose for determination of radioprotective property of the elite plant extracts.
Dose administration of crude rhizome extracts and radiation exposure
The crude rhizome extracts of Z. kangleipakense and Z. officinale prepared in 60% ethanol at a dose of 1 g/kg body weight was used for oral administration to experimental rats before 24 hr of radiation exposure. The rats were divided into seven different groups of five animals each weighing 120–150 g. The control groups were neither administered rhizome extract nor irradiated. One group was only irradiated with 2 Gy. The remaining four groups comprising of two groups of animals corresponding to each dose of plant extracts of 1 g/kg body weight (with or without irradiation at dose of 2 Gy) at a dose rate of 0.01 Gy/sec using GC-4000A 60Co-Gamma Irradiator (Bhabha Atomic Research Center, Mumbai, India). After irradiation, animals were maintained for 7 days and euthanized.
Metaphase chromosome preparation and cytogenetic analysis
Animals were sacrificed by cervical dislocation at the end of day 7. Femurs were removed properly and was induced at one end, and bone marrow cells were aspirated out with normal saline (0.9% sodium chloride) followed by hypotonic treatment with 0.56% potassium chloride for 30 min at 37°C in a water bath. The bone marrow cells were centrifuged at 800 g for 10 min. Then, the supernatant was discarded carefully and the pellet was fixed in chilled Carnoy’s fluid (methanol: glacial acetic acid:: 3:1) by thorough aspiration using a glass Pasteur pipette. The process of fixation and centrifugation was done 3–4 times. Slides were prepared by dropping 3–4 drops of bone marrow cell suspension through a glass Pasteur pipette on clean chilled slides stored in 90% alcohol and heat fixed. Slides were air dried and stained in 10% Giemsa stain. The stained slides were rinsed with distilled water. Chromosomal aberrations per 200 cells were scored for each animal under a light microscope (Leitz DIALUX 22) at 600-fold magnification. Chromosomal aberrations such as chromatid breaks, chromosome breaks, acentric chromosomes, translocations, centric fusions, dicentrics, rings, and cells showing endoreduplications were scored by following the method.[Citation28] Chromosome images were captured using Leica D-LUX 3 digital camera attached to the microscope with oil immersion at 100-fold magnification.
Statistical analysis
Data were analyzed using one-way analysis of variance (ANOVA) followed by Duncan’s post hoc test. Values were presented as mean ± standard error (SE), and p values < .05 were considered significant.
Results and discussion
In vitro screening of antioxidant properties
In ferric ion reducing assay, the presence of reductants in the tested samples resulted in the reduction of the potassium ferricyanide (Fe3+) to the potassium ferrocyanide (Fe2+), which reacts with chloride to form Fe3+/ Fe2+ complex (Perl’s Prussian blue) that can be measured at 700 nm. At the same concentration of 10 mg/mL of 60% methanol extracts of different plant samples, absorbances were 1.8322 for Z. officinale, 1.6434 for Z. montanum, 0.8932 for Z. kangleipakense, and 0.8245 for Z. zerumbet, respectively (). The reducing power of plant extracts increased in a dose-dependent pattern with increasing concentration, thereby indicating stronger reducing power.
DPPH scavenging assay has been widely applicable and accepted method for evaluating antioxidant properties in a number of studies because of its ease, sensitivity, and short duration required for analysis.[Citation29] It bears a deep purple color with maximum absorbance at 515 nm. The percentage decoloration of DPPH increased with increase in the concentration of standard compound, ascorbic acid, and different plant extracts used. Methanol extract of Z. officinale had the highest efficiency of scavenging free radical with IC50 value of 0.22 mg/mL and that of Z. montanum had 0.43 mg/mL. Extracts of Z. zerumbet and Z. kangleipakense showed higher IC50 values than that of Z. montanum and Z. officinale. (). Lower IC50 values of Z. officinale and Z. montanum indicated higher capability of counteracting DPPH free radical. IC50 value of ascorbic acid used as a standard compound was 3.42 µg/mL.
Table 1. Comparative free radical scavenging activity of 60% aqueous methanol rhizome extracts through different antioxidant assays expressed in terms of IC50 value.
In the nitric oxide scavenging assay, sodium nitroprusside (SNP) in phosphate buffer saline is used for the spontaneous generation of nitric oxide, which reacts with oxygen to produce nitrite ions that can be measured using Griess reagent.[Citation30] The nitric oxide scavengers present in the rhizome extracts compete with oxygen, leading to the reduction of NO production. Z. montanum extract shows the lowest IC50 value of 0.91 mg/mL followed by Z. officinale with 1.81 mg/mL, Z. kangleipakense with 4.65 mg/mL and Z. zerumbet with 6.71 mg/mL (). IC50 value of standard compound, ascorbic acid is 45.75 µg/mL. Lowest IC50 value of Z. montanum indicates its high efficacy in nitric oxide radical scavenging.
It may be mentioned here that nitric oxide (NO) is a signaling molecule and plays important role in smooth, cardiac, and skeletal muscle relaxation, inhibition of platelet aggregation, neuronal signaling, increase blood flow, alter memory and behavior, decrease blood clotting, regulation of cell mediated toxicity, etc.[Citation31] Interaction of free radicals, nitric oxide, and superoxide lead to the formation of a highly reactive peroxynitrite, which then causes serious toxic reactions with biomolecules, such as proteins, lipids, and nucleic acids.[Citation32–35] Scavenging these radicals, therefore, would offer significant decrease in cellular damages.
In ferrous ion chelating assay, a purple color ferrozine-ferrous complex is formed with maximum absorbance at 562 nm. With the supplementation of the extract and standard compound, reduction in the purple coloration is observed, indicating the interruption of further complex formation. Metal chelating activities of the elite samples have been shown in terms of IC50 values of which Z. kangleipakense showed the lowest IC50 of 0.24 mg/mL. This shows that the rhizome extracts of Z. kangleipakense have the highest metal chelating ability than the other three samples even though they all possess considerably good iron chelating power. From the observation, there is no significant correlation between metal chelating ability and total phenol content. Z. kangleipakense, with the second lowest TPC, demonstrates the highest metal chelating ability compared to the other three samples tested in agreement with the earlier report.[Citation36] Previous studies also reported very poor or no positive correlation between metal chelating activities and total phenol content or total flavonoid content among some medicinal herbs and mushrooms.[Citation37] Comparative metal ion chelating abilities of different plant samples in different solvent system in terms of IC50 values are shown in .
In the thiyl/sulfur free radical scavenging assay, generated thiyl free radicals cause curcumin oxidation, thereby depleting the color of curcumin. In the control solution, depletion of curcumin content increases with increase in radiation dose.[Citation21] A substantial decrease in the depletion of curcumin is exhibited when the rhizome extracts (60% methanol) are supplemented with the reaction mixtures (). Inhibition of curcumin depletion increases with increasing extract concentrations. Z. montanum (60% methanol) extract exhibits substantial curcumin protection at low concentrations (15x dilution, i.e. 0.4 mL = 0.53 mg/mL and 0.8 mL = 1.06 mg/mL concentration) and also performs better in inhibiting nitric oxide compared to other plant samples. Z. zerumbet (60% methanol) also shows comparatively high degree of inhibiting curcumin depletion at low concentrations (2x dilution, i.e. 0.4 mL = 4 mg/mL and 0.8 mL = 8 mg/mL concentration). However, Z. officinale and Z. kangleipakense showed a relatively lower curcumin protection from thiyl free radicals compared to Z. montanum and Z. zerumbet. The inhibiting property of the curcumin depletion or the protective ability of curcumin by the supplementation of the crude rhizome extract of the four plant samples suggests that they possess bioactive molecules that render antioxidant properties and have thiyl free radical scavenging potentials.
Figure 3. Graphical representation of curcumin protection by 60% methanol extract of Z. kangleipakense (i.e. 0.4 mL = 8 mg/mL and 0.8 mL = 16 mg/mL concentration) against thiyl free radical attack.
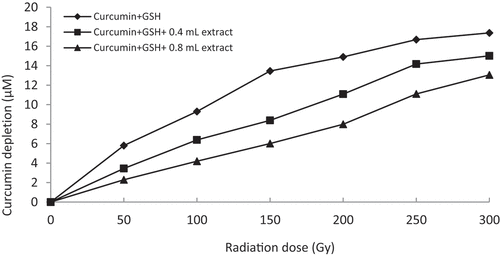
Figure 4. Graphical representation of curcumin protection by 60% methanol extract of Z. montanum (15x dilution, i.e. 0.4 mL = 0.53 mg/mL and 0.8 mL = 1.06 mg/mL concentration) against thiyl free radical attack.
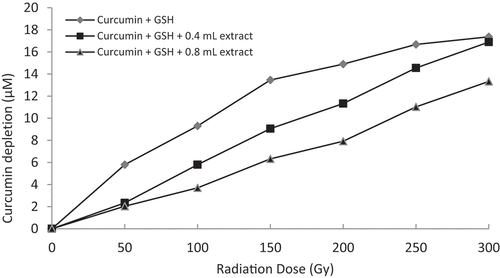
Figure 5. Graphical representation of curcumin protection by 60% methanol extract of Z. officinale (i.e. 0.4 mL = 8 mg/mL and 0.8 mL = 16 mg/mL concentration) against thiyl free radical attack.
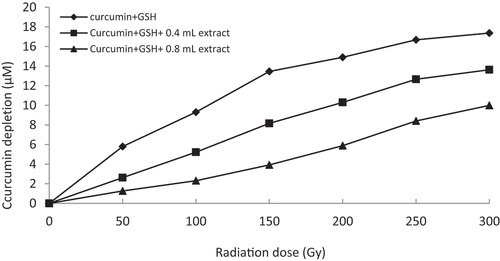
Figure 6. Graphical representation of curcumin protection by 60% methanol extract of Z. zerumbet (2x dilution, i.e. 0.4 mL = 4 mg/mL and 0.8 mL = 8 mg/mL concentration) against thiyl free radical attack.
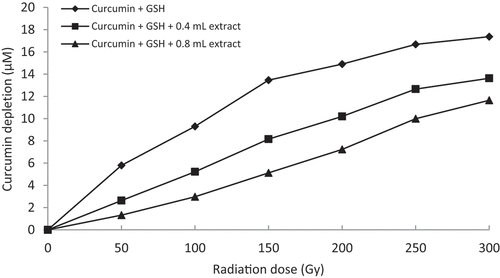
Hydroxyl radical is known to be the most reactive among the ROS and can induce oxidative damage to the living cells reacting with targets, such as proteins, carbohydrates, DNA, polyunsaturated fatty acids, and so on.[Citation38] All the four plant samples show significant concentration-dependent OH radical scavenging properties. Z. kangleipakense exhibits the lowest IC50 value of 0.25 mg/mL indicating maximum radical scavenging potential. Z. zerumbet has comparatively lower IC50 value of 0.48 mg/mL compared to Z. officinale and Z. montanum with IC50 values of 0.74 and 0.99 mg/mL, respectively (). IC50 value of ascorbic acid is observed to be 7.89 µg/mL.
Phenolic compounds are considered secondary metabolites and are potent antioxidants because of their redox properties, which play important roles as hydrogen donors, quenching of singlet and triplet oxygen, neutralizing free radicals, and metal ion chelators.[Citation39,Citation40] Flavonoids are also naturally occurring secondary metabolites in plants, and precursors of flavonoids, such as such as caffeic acid, coumaric acid, ferulic acid, sinapic acid, ellagic acid, gallic acid, vanillic acid, and hydrocoumarin (scopoletin), are reported to form complex with metal ions.[Citation41] Ascorbic acid, an important candidate for enzyme browning and an effective scavenger of free radicals, has also been estimated from the plant samples. It is a powerful water soluble antioxidant that can reduce or scavenge many free radicals and reactive species, thus preventing oxidative and DNA damage.[Citation42] Based on the experimental findings, these four plant extracts exhibit high amount of TPC, TFC, and ascorbic acid. TPC of the plant extracts is expressed in gallic acid equivalent (GAE) and TFC is expressed in quercetin equivalent (QE) present in 100 mg of the plant sample (). The TPC of 60% methanol extract of Z. officinale was found to be 650.09 mg GAE/100 g. Highest value of TFC is observed in Z. offcinale with a value of 889.03 mg QE/100 g. Rhizome extract of Z. officinale shows the highest TPC and TFC as compared with other samples. The rhizome extract of Z. montanum has 390.56 mg GAE/100 g and 656.9 mg QE/100 g TPC and TFC values, respectively. Z. kangleipakense shows TPC and TFC values of 276.25 mg GAE/100 g and 341.22 mg QE/100 g, respectively. Z. zerumbet extract shows the lowest values of TPC and TFC of 178.05 mg GAE/100 g and 184.06 mg QE/100 g compared to the other three samples. Z. officinale is found to have higher ascorbic acid content of 3.19 mg/100 g than Z. montanum (3.08 mg/100 g). Unlike the trends of TPC and TFC, Z. zerumbet shows higher ascorbic acid content of 0.86 mg/100 g than that of Z. kangleipakense with 0.19 mg/100 g ().
Table 2. Antioxidant components of plant extracts given as total phenol content expressed in gallic acid equivalent (GAE), total flavonoid content expressed in quercetin equivalent (QE) and ascorbic acid content.
In vitro screening of radioprotective properties
It is well documented that almost all the damages caused to the living cells by ionizing radiation are due to the generation of aqueous free radicals or reactive oxygen species (ROS), which attack various cellular components including DNA, proteins, and membrane lipids, leading to intense cellular damages.[Citation43,Citation44] Radioprotective agents or antioxidants play important role in counteracting such radiation damages to the living systems. The redox environment with natural defense systems in the cellular pools is of significance in mitigating the radiation risks. Therefore, enhancement of antioxidant pools using nutraceuticals or phytochemicals can contribute to a great extent in such countermeasures. In view of this, both in vitro and in vivo models are used to determine the radioprotective properties of elite Zingiber species.
A variety of lesions such as single-strand breaks, double-strand breaks, DNA-DNA cross-links along with damage to nucleotide bases are produced due to exposure of DNA to ionizing radiation.[Citation45] Evaluating such kind of lesions is considered an important parameter in the examination of the sequence of events, leading to mutagenic, carcinogenic, and lethal effects of radiation. Plasmid pBR322 DNA is predominantly supercoiled or covalently closed circular (ccc) in native form. In this experiment, DNA when exposed to the gamma radiation at doses of 6 Gy leads to the conversion of supercoiled (ccc) form to open circular (oc) form.[Citation27]). The disappearance of ccc-form of DNA can be taken as an index of DNA damage induced by the radiation exposure. Based on the potential of free radical scavenging capacities in different methods used, Z. officinale and Z. kangleipakense were selected for assessment of radioprotective properties. Treatment of plasmid DNA with different concentrations of 60% ethanol extract concentration of rhizome (0.25 to 2 mg/mL for Z.kangleipakense and 0.05 to 0.5 mg/mL for Z. officinale) before 1 h of irradiation results to a dose-dependent protection of pBR322 DNA (). At low concentration of 0.25 mg/mL, Z.kangleipakense is able to provide 56.54% protection of DNA from exposure to gamma radiation at 6 Gy (). Similarly, at same concentration of 0.25 mg/mL, Z. officinale showed 71.50% protection of plasmid when exposed to 6 Gy. (). Presence of plant extract reduces the conversion of ccc-form to oc-form of plasmid DNA, indicating the radioprotective abilities. Similar observations have been reported in experiments carried out elsewhere using curcumin, gallic acid, and Triphala.[Citation27,Citation46,Citation47] Results show that 60% ethanol extracts of Z. kangleipakense and Z. officinale can scavenge various free radicals generated and protect DNA from undergoing strand breakage due to gamma radiation exposure.
Figure 7. Agarose gel electrophoresis pattern of pBR322 DNA exposed to different doses of gamma radiation in the absence and presence of Zingiber kangleipakense (60% aqueous ethanol extract)Lane1: control; Lane 2: DNA exposed to 6 Gy; Lane 3: DNA with 0.25 mg/mL extract exposed to 6 Gy; Lane 4: DNA with 0.5 mg/mL extract exposed to 6 Gy; Lane 5: DNA with 1 mg/mL extract exposed to 6 Gy; Lane 6: DNA with 2 mg/ mL extract exposed to 6 Gy.
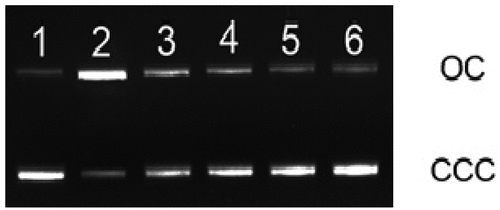
Figure 8. Agarose gel electrophoresis pattern of pBR322 DNA exposed to different doses of gamma radiation in the absence and presence of Zingiber officinale (60% aqueous ethanol extract).
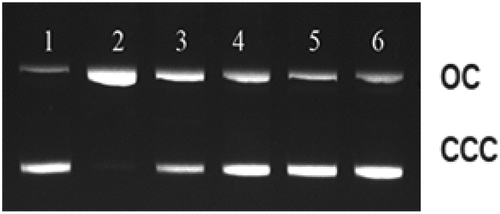
Table 3. Ability of rhizome extract (60% ethanol) of Z. kangleipakense to protect pBR322 DNA from radiation-induced damages in vitro.
Table 4. Ability of rhizome extract (60% ethanol) of Z. officinale to protect pBR322 DNA from radiation-induced damages in vitro.
Effect of Z. kangleipakense and Z. officinale rhizome extracts on chromosomal aberrations
Albino rats have been used for the in vivo experiments. In the experiments to investigate any possible acute toxicity of the two plant extracts, it was found that no significant changes were observed in parameters like behavior, growth, consumption of water, and foods of animals as compared with the control group. In the US FDA documents, ginger has been grouped in ‘Generally Recognized as Safe’ (GRAS) list. In some literatures, LD50 for the acute oral toxicity of roasted ginger in rat is 170 g/kg body weight and that of dry ginger is more than 250 g/kg body weight.[Citation48,Citation49] In the experimental results, there was no mortality in the extract treated animals at a dose of 4.5 g/kg upto a period of 30 days, and hence LD50 of both extracts may consider greater than 2 g/kg body weight. The 60% ethanol has been claimed to have no significant clastogenic effect to the rats.[Citation50] Whole-body exposure of rats at a radiation dose of 2 Gy produced different types of chromosomal aberrations. The most common chromosomal aberrations are fragments, breaks, rings, dicentrics, fusions, and endoreduplications (). Oral administration of the rhizome extracts (60% ethanol) of Z. kangleipakense and Z. officinale at a dose of 1 g/kg considerably reduces chromosomal aberrations in the animals irradiated with 2 Gy. Z. officinale shows higher radioprotective ability than Z. kangleipakense (). In the present study, 60% ethanol extract of Z. kangleipakense and Z. officinale show potential radioprotective properties and, hence, protect bone marrow cells from chromosomal aberrations by 38.95% and 51.16%, respectively, with an extract dose of 1 mg/kg at a radiation dose of 2 Gy. Z. officinale exhibited better radioprotection as compared with Z. kangleipakense.
Figure 9. (a) Normal metaphase spread of Rattus norvegicus albus chromosomes; (b) Metaphase plate of Rattus norvegicus chromosomes showing different chromosomal abberations: dicentric chromosome (DC), chromatid & centromere break (CrB, CmB) and acrocentric association (ACA); (c) Metaphase plate showing chromosome breaks (CB) and chromosome fusion (F); (d) chromosomes showing ring chromosome (R); (e): chromosomes showing endoreduplication with condensed chromosomes and (f): chromosomes showing acentric fragment (AF).
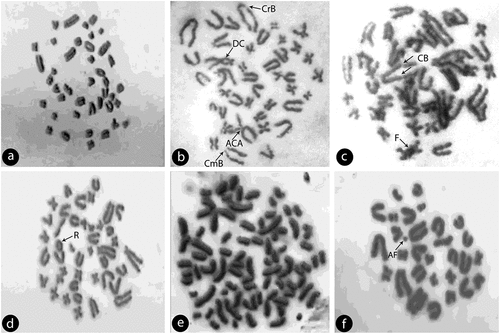
Table 5. Effect of Z. kangleipakense and Z. officinale extracts (60% ethanol) on radiation-induced chromosomal damage in whole-body irradiated albino rats at a dose of 2 Gy.
Conclusion
The present results clearly show that the rhizome extracts of the elite Zingiber species possess significant antioxidant properties and free radical scavenging potentials. With the variance in the presence of phytochemicals in different plant extracts, different capabilities in scavenging different free radicals generated are exhibited. Thus, Z. officinale has shown maximum DPPH free radical scavenging and ferric ion reducing power than other three samples. Z. montanum shows the highest thiyl free radical scavenging and nitric oxide inhibiting ability, and Z. kangleipakense has shown greater metal chelating and hydroxyl free radical scavenging ability as compared to the other three samples. Z. officinale shows the highest total phenol, flavonoid, and ascorbic acid contents among them. Considering the observed results on antioxidant potentials of these plant samples used, it can be assumed that these plants are potential sources of natural antioxidants and may be used as nutraceuticals for various therapeutic applications for the control and treatment of ROS-mediated diseases. 60% aqueous ethanol extracts of Z. officinale and Z. kangleipakense were chosen for radioprotective assays for both in vitro and in vivo using plasmid pBR322 DNA, and bone marrow cells of albino rats, respectively, are based on their highest efficacy of antioxidant and free radical scavenging properties among the four samples. From the present study, Z. officinale shows better radioprotection as compared with Z. kangleipakense for both in vitro and in vivo from radiation-induced DNA and chromosomal damages. These findings do indeed lend credence to the requirement of immediate supplementation of the antioxidant extracts as nutraceuticals in dietary intakes from the viewpoint of preventive countermeasures. At the same time, experiments as to whether combination treatments of the both the extracts provide additive or synergistic effects need to be undertaken using suitable mammalian model systems in vitro and in vivo. Experiments are under way to address and resolve some of these significant issues.
Acknowledgments
MMD is thankful to the Department of Science and Technology, Government of India, for providing financial assistance (vide Grant No. DST/INSPIRE Fellowship/ 2013/285). No conflict of interest arises. Contributions: MMD has performed experimental design, data collection, analysis, interpretation, and manuscript writing. GJS has performed conceptualization, data evaluation, interpretation, editing, and final draft preparation.
Disclosure statement
No potential conflict of interest was reported by the author(s).
Correction Statement
This article has been corrected with minor changes. These changes do not impact the academic content of the article.
Additional information
Funding
References
- Aruoma, O. I. Assessment of Potential Prooxidant and Antioxidant Actions. Journal of the American Oil Chemists’ Society. 1996, 73(12), 1617–1625. DOI: 10.1007/BF02517962.
- Zima, T. S.; Fialova, L.; Mestek, O.; Janebova, M.; Crkovska, J.; Malbohan, I.; Stipek, S.; Mikulíková, L.; Popov, P. Oxidative Stress, Metabolism of Ethanol and alcohol-related Diseases. J. Biomed. Sci. 2001, 8(1), 59–70. DOI: 10.1007/BF02255972.
- McCord, J. M. The Evolution of Free Radicals and Oxidative Stress. The American Journal of Medicine. 2000, 108(8), 652–659. DOI: 10.1016/S0002-9343(00)00412-5.
- Patra, J. K.; Rath, S. K.; Jena, K.; Rathod, V. K., and Hrudayanath, T. Evaluation of Antioxidant and Antimicrobial Activity of Seaweed (Sargassum Sp.) Extract: A Study on Inhibition of glutathione-s-transferase Activity. Turkish J. Biol . 2008.32, (2)119–125.
- Poulsen, H. E.; Prieme, H.; Loft, S. Role of Oxidative DNA Damage in Cancer Initiation and Promotion. Eur. J. Cancer Prev. 1998, 7(1), 9–16. DOI: 10.1111/j.1365-2621.1979.tb09125.x.
- Yue-Fei, W.; Jei, W.; Jing, W. U.; Ping, X. U.; Yi-Qi, W.; Jun-Jei, G.; Danielle, H. In Vitro Antioxidant Activity and Potential Inhibitory Action against α-glucosidase of Polysaccharides from Fruit Peel of Tea (Camellia Sinensis L.). J. Zhejiang Univ. Sci. B. 2014, 15(2), 173–180. DOI: 10.1631/jzus.B1300186.
- Grillo, C. A.; Dulout, F. N. Cytogenetic Evaluation of Butylated Hydroxyl Toluene. Mutat. Res. 1995, 345(1–2), 73–78. DOI: 10.1016/0165-1218(95)90071-3.
- Lin, J.; Li, X.; Chen, L.; Lu, W.; Chen, X.; Han, H.; Chen, D. Protective Effect against Hydroxyl radical-induced DNA Damage and Antioxidant Mechanism of [6]-gingerol: A Chemical Study. Bulletin of the Korean Chemical Society. 2014, 35(6), 1633–1638. DOI: 10.5012/bkcs.2014.35.6.1633.
- Polasa, K.; Naidu, N. A.; Ravindranath, I.; Krishnaswamy, K. Inhibition of B(a) P Induced Strand Breaks in Presence of Curcumin. Mutat. Res. 2004, 557(2), 203–213. DOI: 10.1016/j.mrgentox.2003.10.016.
- Soltani, B.; Ghaemi, N.; Sadeghizadeh, M.; Najafi, F. Redox Maintenance and Concerted Modulation of Gene Expression and Signaling Pathways by a Nanoformulation of Curcumin Protects Peripheral Blood Mononuclear Cells against Gamma Radiation Chem. Chemico-Biological Interactions. 2016, 257(25), 81–93. DOI: 10.1016/j.cbi.2016.07.021.
- Khor, T. O.; Huang, Y.; Wu, T. Y.; Shu, L.; Lee, J.; Kong, A. N. Pharmocodynamics of Curcumin as DNA Hypomethylation Agent in Restoring the Expression of Nrf2 via Promoter CpGs Demethylation. Biochem. Pharmacol. 2011, 82(9), 1073–1078. DOI: 10.1016/j.bcp.2011.07.065.
- Lotito, S. B.; Zhang, W.-J.; Yang, C. S.; Crozier, A.; Frei, B. Metabolic Conversion of Dietary Flavonoids Alters Their anti-inflammatory and Antioxidant Properties. Free Radical Biology and Medicine. 2011, 51(2), 454–463. DOI: 10.1016/j.jep.2014.11.034.
- Peng, Y.; Zhang, H.; Liu, R.; Mine, Y.; McCallum, J.; Kirby, C.; Tsao, R. Antioxidant and anti-inflammatory Activities of Pyranoanthocyanins and Other Polyphenols from Staghorn Sumac (Rhushirta) in Caco-2 Cell Models. J. Funct. Foods. 2016, 20, 139–147. DOI: 10.1016/j.jff.2015.10.026.
- Myers, N.; Mittermeier, R. A.; Mittermeier, C. G.; Fonseca, G. A. B.; Kent, J. Biodiversity Hotspots for Conservation Priorities. Nature. 2000, 403(6772), 853–858. DOI: 10.1038/35002501.
- Chan, E. W. C.; Lim, Y. Y.; Wong, S. K.; Lim, K. K.; Tan, S. P.; Lianto, F. S.; Yong, M. Y. Effects of Different Drying Methods on Antioxidant Properties of Leaves and Tea of Ginger Species. Food Chem. 2009, 113(1), 166–172. DOI: 10.1016/j.foodchem.2008.07.090.
- Oyaizu, M. Studies on Products of Browing Reaction: Antioxidantive Activity of Product of Browning Reaction Prepared from Glucosamine. Jpn. J. Nutr. 1986, 44(6), 307–315. DOI: 10.5264/eiyogakuzashi.44.307.
- Miliauskas, G.; Venskutonis, P. R.; Van Beek, T. A. Screening of Radical Scavenging Activity of Some Medicinal and Aromatic Plant Extracts. Food Chemistry. 2004, 85(2), 231–237. DOI: 10.1016/j.foodchem.2003.05.007.
- Garratt, D. C. The Quantitative Analysis of Drugs, 3rd Edition. United Kingdom: Springer US. 2012; 9781461333807.
- How, Y. L.; Lau, Y. L. Evaluation of Antioxidant Activities of the Methanolic Extracts of Selected Ferns in Malayasia. Int. J. Environ. Sci. Dev. 2011, 2(6), 442–447.
- Chirangini, P.; Sharma, G. J.; Sinha, S. K. Sulfur Free Radical Reactivity with Curcumin as Reference for Evaluating Antioxidant Properties of Medicinal Zingiberales. Journal of Environmental Pathology, Toxicology and Oncology. 2004, 23(3), 227–236. DOI: 10.1615/JEnvPathToxOncol.v23.i3.60.
- Devi, M. D.; Devi, H. S.; Sharma, G. J.; James, G.; Tipping, I.; Willson, R. L. Curcumin: Visible Destruction of the Conjugated Unsaturated Compound as a Reference Indicator of Sulphur Free Radical Reactivity. Int. J. Radiat. Biol. 1992, 62, 105.
- D’ Aquino, M.; Bullion, C.; Chopra, M.; Devi, D.; Devi, S.; Dunster, C.; James, G.; Komura, E.; Kundu, S.; Niki, E., et al. Sulfhydryl Free Radical Radical Formation Enzymatically, by Sonolysis, by Radiolysis and Thermally: Vitamin A, Curcumin, Muconic Acid and Related Conjugated Olefins as References. Meths Enzymol. 1994, 233, 34–46.
- Smirnoff, N., and Cumbes, Q. J. 1989. Hydroxyl Radical Scavenging Activity of Compatible Solutes. Phytochem. 28, 1057–1060.
- Singleton, V. L., and Rossi, J. A. 1965. Colorimetry of Total Phenolics with phosphomolybdic-phosphotungstic Acid Reagents. Am. J. Enol. Vitic. 16(), 144–158.
- Jia, Z. M.; Tang, J.; Jianming, W. The Determination of Flavonoid Content in Mulberry and Their Scavenging Effects on Superoxide Radicals. Food Chem. 1999, 64(4), 555–599. DOI: 10.1016/S0308-8146(98)00102-2.
- Klein, B. P.; Perry, A. K. Ascorbic Acid and Vitamin A Activity in Selected Vegetables from Different Geographical Areas of United States. J. Food Sci. 1982, 47(3), 941–945. DOI: 10.1111/j.1365-2621.1982.tb12750.x.
- Gandhi, N. M.; Nair, C. K. K. Protection of DNA and Membrane from Gamma Radiation Induced Damage by Gallic Acid. Molecular and Cellular Biochemistry. 2005, 278(1–2), 111–117. DOI: 10.1007/s11010-005-6940-1.
- Savage, J. R. K. Classification and Relationships of Induced Chromosomal Structural Changes. J. Med. Genet. 1976, 12(2), 103–122. DOI: 10.1136/jmg.13.2.103.
- Brad-Williams, W.; Cuvelier, M. E., and Berset, C. 1995. Use of a Radical Method to Evaluate Antioxidant Activity, Lebensen-wiss A. Technol. F. Sci. Technol. 28, 25–30. DOI: 10.1016/S0023-6438(95)80008-5.
- Miller, M. J.; Sadowska-krowicka, H.; Chotinaruemol, S.; Kakkis, J. L.; Clark, D. A. Amelioration of Chronic Iletis by Nitric Oxide Synthase Inhibition. J. Pharmacol. Exptl. Ther. 1993, 264, 11–16.
- Murad, F. Discovery of Some of the Biological Effects of Nitric Oxide and Its Role in Cell Signaling. Bioscience Reports. 1999, 19(3), 133–154. DOI: 10.1023/A:1020265417394.
- Moncada, S.; Palmer, R. M.; Higg, E. A. Nitric Oxide: Physiology, Pathophysiology, and Pharmacology. Pharmacol. Rev. 1991, 43(2), 109–142.
- Radi, R.; Beckman, J. S.; Bush, K. M.; Freeman, B. A. Peroxynitrite Oxidation of Sulfhydryls. J. Biol. Chem. 1991a, 266(7), 4244–4250. DOI: 10.1016/S0021-9258(20)64313-7.
- Radi, R.; Beckman, J. S.; Bush, K. M.; Freeman, B. A. Peroxynitrite-induced Membrane Lipid Peroxidation: The Cytotoxic Potential of Superoxide and Nitric Oxide. Archives of Biochemistry and Biophysics. 1991b, 288(2), 481–487. DOI: 10.1016/0003-9861(91)90224-7.
- Yermilov, V.; Rubio, J.; Becchi, M.; Friesen, M. D.; Pignatelli, B.; Ohshima, H. Formation of 8-nitroguanine by the Reaction of Guanine with Peroxynitrite in Vitro. Carcinogenesis. 1995, 16(9), 2045–2050. DOI: 10.1093/carcin/16.9.2045.
- Wong, F. C.; Chai, T. T.; Tan, S. L.; Yong, A. L. Evaluation of Bioactivities and Phenolic Content of Selected Edible Mushroom in Malaysia. Trop. J. Pharm. Res. 2013, 12(6), 1011–1016. DOI: 10.4314/tjpr.v12i6.21.
- Rainha, N.; Lima, E.; Baptista, J.; Rodrigues, C. Antioxidant Properties, Total Phenolic, Total Carotenoid and Chlorophyll Content of Anatomical Parts of Hypericumfoliosum. J. Med. Plants Res. 2011, 5(10), 1930–1940. DOI: 10.5897/JMPR.9000227.
- Nabavi, S. M. In Vitro Antioxidant and Free Radical Scavenging Activity of Diospyros Lotus and Pyrus Boissieriana Growing in Iran. Pharmcogn. Mag. 2009, 4(18), 123–127.
- Rice-Evans, C. A.; Miller, N. J.; Paganga, G. Antioxidant Properties of Phenolic Compounds. Trends Plant Sci. 1997, 2(4), 152–159. DOI: 10.1016/S1360-1385(97)01018-2.
- Dzoyem, J. P.; Eloff, J. N. Anti-inflammatory, Anticholinesterase and Antioxidant Activity of Leaf Extracts of Twelve Plants Used Traditionally to Alleviate Pain and Inflammation in South Africa. J. Ethnopharmacol. 2015, 3(160), 194–201. DOI: 10.1016/j.jep.2014.11.034.
- Pratt, D. E.; Birac, P. M. Source of Antioxidant Activity of Soybeans and Soy Products. Journal of Food Science. 1979, 44(6), 1720–1722. DOI: 10.1111/j.1365-2621.1979.tb09125.x.
- Du, J.; Cullen, J. J.; Buettner, G. R. Ascorbic Acid: Chemistry, Biology and the Treatment of Cancer. Biochimica Et Biophysica Acta. 2012, 1826(2), 443–457. DOI: 10.1016/j.bbcan.2012.06.003.
- Valko, M.; Leibfritz, D.; Moncola, J.; Cronin, M. D.; Mazur, M.; Telser, J. Free Radicals and Antioxidants in Normal Physiological Functions and Human Disease. The International Journal of Biochemistry & Cell Biology. 2007, 39(1), 44–84. DOI: 10.1016/j.biocel.2006.07.001.
- Mansour, H. H.; Hafe, H. F.; Fahmy, N. M.; Hanafi, N. Protective Effect of N-acetylcysteine against Radiation Induced DNA Damage and Hepatic Toxicity in Rats. Biochemical Pharmacology. 2008, 75(3), 773–780. DOI: 10.1016/j.bcp.2007.09.018.
- Scholes, G. Radiation Effects on DNA. The Silvanus Thompson Memorial Lecture, April 1982. Br J Radiol. 1983, 56(664), 221–223. DOI: 10.1259/0007-1285-56-664-221.
- Naik, G. H.; Priyadarsini, K. I.; Bhagirath, R. G.; Mishra, B.; Mishra, K. P.; Banavalikar, M. M.; Mohan, H. In Vitro Antioxidant Studies and Free Radical Reactions of Triphala, an Ayurvedic Formulation and Its Constituents. Phytotherapy Research. 2005, 19(7), 582–586. DOI: 10.1002/ptr.1515.
- Singh, P. P.; Devi, K. R.; Devi, M. M.; Thokchom, D. S.; Sharma, G. J. Protection of Low LET radiation-induced DNA Damage in Rat Bone Marrow Cells by Free Radical Scavenger Curcumin. Int. J. Pharm. Sci. Res. 2016, 7(3), 1168–1178.
- Bradley, P.;. British Herbal Compendium; I Vol, British Herbal Medical AssociationBournemouth: Dorset, UK, 1990; pp 112–114.
- Langner, E.; Greifenberg, S.; Gruenwald, J. Ginger: History and Use. Advances in Therapy. 1998, 15(1), 25–44.
- Thokchom, D. S.; Sharm, T. D.; Sharma, G. J. Radioprotective Effect of Rhizome Extract of Zingiber Montanum in Rattus Norvegicus. Radiat. Environ. Biophys. 2012, 51(3), 311–318.