ABSTRACT
Several scientific researchers have focused on biological activities of edible plants such as radish. However, there are no researches on the anticancer effect of this plant in human glioblastoma. Thus, aims of this study were to evaluate the phytochemical composition, antioxidant and anticancer activities of Raphanus sativus bulbs aqueous and methanol extracts against U-87 MG cells. Five phenol compounds were found in aqueous extract using HPLC-DAD with syringic acid followed by protocatechuic acid, gallic acid, p-coumaric acid and cirsilineol. The DPPH, ABTS, OH radical, iron (II) chelation and FRAP assays exhibited that aqueous extract shows high antioxidant activity. The chemical composition analysis of the methanol extract using GC-MS showed that 4-methylthio-3-butenyl isothiocyanate (raphasatin) was the most abundant compound (20%). Cytotoxicity assessment by MTT method showed that methanol extract exhibited a significant cytotoxicity to U-87 MG cells with IC50 at 241.35 ± 15.21 µg/mL after 72 h while no effect was noted for aqueous extract. Dis-adhesion assay of the extracts as well as of raphasatin was assessed using different protein matrices (fibronectin, fibrinogen and poly-L-lysine) and the anti-proliferative effect was performed using doxorubicin as positive control. Methanol extract showed a high ability to inhibit cell adhesion to the used protein matrices with IC50 of 20.46 ± 2.52; 12.23 ± 1.41 and 16.21 ± 1.92 µg/mL, respectively. Moreover, raphasatin strongly inhibited tumor cell adhesion with IC50 of 0.63 ± 0.09; 1.27 ± 0.18 and 0.95 ± 0.12 µg/mL, respectively. In addition, it totally blocked the proliferation of cancer cells at a dose of 0.5 µg/mL after 4 days of incubation. These data demonstrate, for the first time, the protective effect of R. sativus methanol extract and its major compound, 4-methylthio-3-butenyl isothiocyanate against U-87 MG cancer cells. Raphasatin may represent an innovative and promising tool for human glioblastoma treatment.
Introduction
The incidence of brain tumors is relatively rare compared with other cancers. Nevertheless, malignant brain tumors have a very high mortality rate. The American Cancer Society reported an estimated 23,770 cases of brain and other nervous system cancers in 2016.[Citation1] Glioblastoma is the most common type of primary central nervous system tumor. Due to the high rate of recurrence, the survival rate of patients diagnosed with glioblastoma in the last 5 years was only 4.5% and the median survival was only 14.2%,[Citation2] and recurrent tumors are more aggressive, infiltrative, and therapy-resistant than the original malignancy.[Citation3,Citation4] Glioma cells express a diverse set of proteins, including metalloproteinase and integrins, which serve as biomarkers for tumor detection and as therapeutic targets. In particular, integrins are involved in all the carcinogenesis process[Citation5] and are able to bind to the extracellular matrices and provide critical cellular functions such as transduction of intracellular signals, adhesion, cytoskeleton organization, cell differentiation, apoptosis, cell proliferation as well as cell diffusion.[Citation6] The diffusing glioma cells cause treatment failure by surgery, radiotherapy, chemotherapy, and enhance drug resistance. On the other hand, there are chronic side effects associated with chemotherapy, including nausea, bone marrow failure, and the development of multidrug resistance (MDR).[Citation7,Citation8] Hence, it is of great importance to discover natural compounds that could serve as alternatives for cancer treatment.
Radish (Raphanus sativus), belonging to the Brassicaceae family, is used in indigenous Asian herbal medications[Citation9,Citation10] and has dietary nutritional values.[Citation11] Radish has been reported to possess a wide range of pharmacological activities such as gut stimulatory,[Citation12] hepatoprotective,[Citation13] cardioprotective,[Citation14] antioxidant,[Citation15] antitumor, and anti-inflammatory activities,[Citation16,Citation17] These biological activities are due to its richness in glucosinolates such as glucoraphasatin (GRH) and phenolic compounds,[Citation15] but to the best of our knowledge, there is no report available on the effect of radish and its constituents against human glioblastoma. Thus, the current study aims to[Citation1] identify natural compounds of R. sativus bulb methanol and water extracts,[Citation2] to evaluate their antioxidant activity and[Citation3] to study for the first time the protective effect of methanol extract and its compounds against human glioblastoma cells (U-87 MG).
Materials and methods
Chemicals and reagents
All chemicals and reagents were either analytical or HPLC grade. Ultrapure deionized water was obtained from a Milli-QVR Integral water purification system with a Q-pod purchased from Millipore (Bedford, MA, USA). Acetic acid and acetonitrile were purchased from VWR International S.r.l. (Milan, Italy); methanol HPLC gradient grade was obtained from Merck (Darmstadt, Germany). Phenol standards were from HWI Analytic GmbH (Rulzheim, Germany). The human fibrinogen, fibronectin and doxorubicin were from Sigma Aldrich (Mannheim, Germany).
Plant material and extracts preparation
Fresh Raphanus sativus was obtained from a local market in Beja (geographical coordinates 36°43′32′′N/9°10′54”E) in January 2017. It was identified according to the flora of Tunisia by Professor Hichem Sebai. Bulbs were air dried for 7 days at room temperature (20 ± 2°C), ground into a fine powder using a Retsch blender mill, sifted through a 0.5 mm mesh screen to obtain a uniform particle size, and stored in the Laboratory of Functional Physiology and Valorization of Bioresources, High Institute of Biotechnology of Beja, Tunisia with the Voucher specimen number # RS-BL-20-01-17 and subsequently used for extraction. Sample (100 g) of ground bulb were extracted separately with 400 mL of water or methanol, sonicated for 15 min, and macerated for 24 hours at room temperature. The extracts were centrifuged for 15 min at 13,000 rpm after being filtered through a Whatman no. 40 filter paper. Extraction yields were 19 and 11% respectively using water and methanol. We did not use a rotary evaporator to dry the extracts to avoid the loss of volatile compounds such as isothiocyanates. The extracted samples were kept at 4°C until further analysis. Plant material was also dried at 105°C for 24 h, and the moisture was found to be 62.51%.
Phytochemical analysis
Total phenols and flavonoids contents analysis
The phytochemical screening of total phenol, flavonoids was evaluated according to the procedure reported by Albouchi et al.[Citation18] The total phenolic content (TPC) of extracts was assessed by spectrophotometry using the “Folin Ciocalteau” (FC) reagent assay. Briefly, 20 µL of the diluted extract solution was mixed with 100 µL of FC reagent (0.2 N). The mixture, prepared in a 96-well plate, was agitated for 30s and rested in the darkness for 5 min. 80 µL of Na2CO3 (75 g/L) was added. After agitation and incubation for 15 min, the absorbance was measured at 765 nm. Gallic acid (GA) (0–30 µg/mL) was used as a standard for the calibration curve. The total phenol content was expressed as micrograms of GA equivalent per milligram of dry weight (µg GAE/mg dw). To determine the total flavonoid content (TFC), 100 µL of each extract were mixed with an equal volume of AlCl3 solution diluted in methanol to 2%. After incubation for 15 min, the absorption was measured at 415 nm. Quercetin (2–10 µg/mL) was used as a standard for the calibration curve. The amount of total flavonoids in extracts is expressed as micrograms of quercetin equivalent per milligram of dry weight (µg QE/mg dw).
HPLC analysis
Phenol compounds of radish bulb aqueous extract were determined using a modification of a previously described HPLC technique.[Citation18] An Agilent 1100 series HPLC system, provided with a diode array detector (DAD) model G 1315 A, a G 1313 A Autosampler, and a G 1311A pump, was used. The separation was achieved using a Water Spherisorb 5 mm (ODS2250mm x 4.6 mm) at 40°C. The mobile phase consisted of (A) aqueous phosphoric acid (0.22 M) and (B) acetonitrile. The solvent gradient started at 10% (A) and reached 35% (A) in 20 min, and 100% (A) in 60 min followed by post-time isocratic conditions for 10 min at 10% (A) before the next injection. The flow rate was 1 mL/min, and the injection volume was 10 µL. The monitoring wavelengths were 280 and 350 nm, with a scan from 200 to 600 nm. The identification of each compound was based on a combination of retention time and spectral matching with the reference compound. Quantification of the identified phenol compounds was performed by correlating the measured peak area with the calibration curves obtained with the reference compounds (0.5, 5, 20, 50, 100 and 1000 µg/mL).
GC-MS analysis
Chromatographic separation and identification of components of methanol extract of R. sativus were performed on a gas chromatograph Varian model 3800 equipped with a Varian 7800 autosampler, a split/splitless injector Varian 1079, and an ion trap mass detector (ITMS) 2000. The analytical column was a Varian VF5m s (30 m × 0.25 mm i.d. × 0.25 μm film thickness) (Varian, Milan, Italy). Helium was the carrier gas at 1 mL/min. 1 μl sample, previously dehydrated with sodium sulfate and filtrated, was injected in splitless mode with a purge valve on for 1 min. The injector temperature was set at 200°C. The mass spectrometer detector was operated in the electron ionization-positive mode. Trap, manifold, and transfer-line temperatures were at 200, 80, and 200°C, respectively. The oven was programmed as follows: 50°C (1 min), raised to 100°C (5°C/min), and held for 1 min, then raised to 180°C (20°C/ min), and held for 4 min. Qualitative analysis was performed in the scan mode (50 − 550 amu). Peak identification was made by comparing full mass spectra and retention times from authentic standards and the NIST MS Spectra Library (The NIST Mass Spectral Search Program for the NIST\EPA\NIH mass Spectral Library, version 2.0, build 12/ 2000).
Antioxidant activity evaluation
The DPPH, ABTS, and hydroxyl radical scavenging activities of methanol and aqueous extract of R. sativus were determined by a previously described method.[Citation19] The antioxidant activity was expressed as the amount of extract necessary to scavenge the initial ABTS, DPPH, or OH radical by 50% (IC50). Ferric reducing antioxidant power (FRAP) was evaluated by the method described in the same previous study of Albouchi et al., 2018.(19) Briefly, FRAP reagent was freshly prepared as follows: 12.5 mL of acetate buffer (0.3 mol/L, pH = 3.6) was mixed with the same volume of methanol. 2.5 mL of 10 mmol/L TPTZ in 0.04 mol/L HCl and 2.5 mL of FeCl3 · 6H2O (0.02 mol/L) were added to such a solution. Then, 225 µL of each extract were added to the 2.25 mL of FRAP reagent. The absorbance was measured at 593 nm and the results were expressed as FeSO4_7H2O-equivalent mM per gram of dry weight (mM/g dw), using a calibration curve.
The Iron (II) chelation assay was determined as previously described.[Citation20] A 675 μl of extract dissolved in acetate buffer (10 mM, pH 3.6) was added to 75 μl of a 1 mM FeSO4_7H2O solution. After 20 min, 750 μl of 1 mM ferrozine solution was added. The absorbance was measured at 562 nm and the data were expressed as EDTA equivalent mM of gram of dry weight (mM/g dw).
Anticancer activity evaluation
Cell culture
The human U-87 MG Glioblastoma cells were purchased from the American Type Culture Collection (ATCC; Manassas, VA, USA). Then they were first cultured in modified Eagle’s medium (MEM) supplemented with 10% fetal calf serum (FCS) containing 100 IU/mL of penicillin. Afterward they were incubated in 5% CO2 at 37°C.[Citation5,Citation21]
Cell viability test
The cytotoxicity of aqueous and methanol extracts against U-87 MG cells was assessed by the MTT assay as previously described.[Citation21] Briefly, MTT solution was added to the culture medium 4 h before the end of treatment. Cells were counted, plated in 96-well plates, either treated or not with different concentrations of methanol or aqueous extract of R. sativus (50, 100, 200, and 300 µg/mL). After 72 hours of incubation, cells were fixed with 1% glutaraldehyde, stained with a solution of 0.1% crystal violet, and lyzed with 1% SDS. Then, the absorbance was measured at 560 nm. Doxorubicin (Dox) was used as positive control.
Cell adhesion assay
In order to determine the effect of R. sativus extracts on U-87 MG cell adhesion, 96-well plates were coated with one of the following purified extracellular matrix proteins: fibronectin (10 µg/mL), fibrinogen (10 µg/mL) or poly-L-lysine (50 µg/mL) and were covered with 50 µL of BSA-PBS. In EMEM containing 0.2% BSA, 106 cells/mL were treated for 90 min in the absence or presence of different concentrations of aqueous or methanol extracts (10, 20, 50 and 100 µg/mL) or water as control. Then, they were added to extracellular matrix-coated wells and allowed to adhere for 60 min at 37°C. Unattached cells were eliminated by washing three times with adhesion buffer. The adherent cells were fixed, stained with 0.1% crystal violet and lyzed with 1% SDS. Absorbance was then measured at 560 nm.[Citation21] The same experiment was repeated using 4-methylthio-3-butenyl isothiocyanate and positive control doxorubicin individually at 1.25; 1.0; 0.5 and 0.25 µg/mL.
Cell proliferation assay
U-87 MG cells (5000 cells/well) were plated in a microtiter plate and incubated overnight in a humid atmosphere of 5% CO2 at 37°C. Then, 0.5 µg/mL of 4-methylthio-3-butenyl isothiocyanate or doxorubicin were added. After 4 days of incubation, cells were washed with PBS, fixed with 1% of glutaraldehyde, stained with 0.1% crystal violet and quantified by absorbency at 560 nm.[Citation5]
Statistical analysis
Data are from at least three independent experiments. All values are expressed as mean ± standard deviation (SD), and the statistical significance of differential findings between experimental groups was determined by a one-way ANOVA test using GraphPad Prism six software and SPSS. Differences in p values below 0.05 were considered significant (p < .05).
Results
Phytochemical analysis
Total phenol and flavonoid content analysis: Total phenol and flavonoid content characterization showed that aqueous extract is richer in phenols and flavonoids than methanol extract with 180,33 ± 9.61; 42,12 ± 4.28 [µg GAE/mg dw] and 63.34 ± 5.27; 14.21 ± 2.13 (µg QE/mg dw) respectively ().
Figure 1. Total phenols and flavonoid content of R. sativus aqueous and methanol extracts. Values are expressed as means ± SD. Radish aqueous extract is richer on phenols and flavonoids than methanol extract. All experiments were made in triplicates.
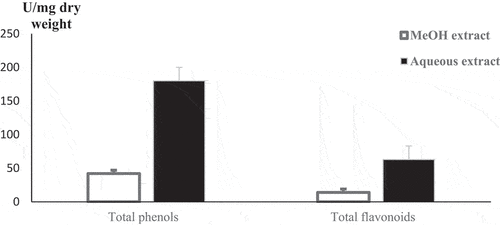
HPLC analysis
The phenol compounds of aqueous extract of radish bulb were analyzed using HPLC-DAD. After comparison of the retention times and UV/visible spectra with available standards corresponding peaks, five phenol compounds were found with syringic acid as the most abundant followed by protocatechuic acid, gallic acid, p-coumaric acid and cirsilineol with 9.15 ± 2.03; 6.23 ± 1.64; 4.62 ± 0.98; 1.24 ± 0.54 and 0.51 ± 0.06 mg/g respectively (, ).
Figure 2. HPLC profiles of the obtained compounds. Five phenolic compounds were identified in radish aqueous extract.
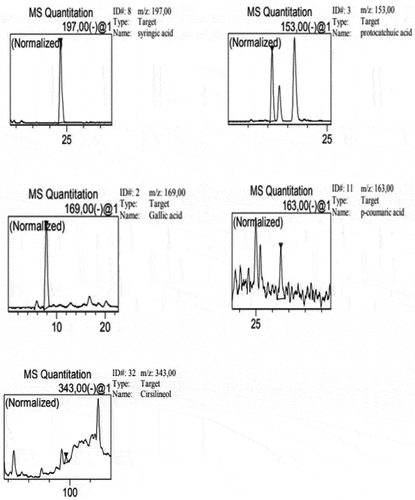
Table 1. Retention time (min) and concentration of phenolics identified in R. sativus bulb aqueous extract bu HPLC DAD.
GC-MS analysis: Using GC-MS at these experimental conditions, we were able to identify and quantify the most abundant metabolite of methanol extract, 4-methylthio-3-butenyl isothiocyanate (raphasatin) at 20% ().
Antioxidant activity of R. sativus extracts
The ability of methanol and aqueous extracts of R. sativus bulb to reduce DPPH, ABTS, OH radicals besides, ferric reducing (FRAP) and Iron (II) chelation power are presented in . Both extracts showed a concentration-dependent scavenging activity. The aqueous extract proved to be the highest DPPH, ABTS and OH radical scavenger, with IC50 values of 121.03 ± 5.71; 31.46 ± 4.72 and 10.65 ± 1.88 µg/mL, respectively. Ferric-reducing power of aqueous extract was also significantly higher (p < .05) than methanol extract (91.27 ± 5.21 mM FeSO4/g dw and 35.29 ± 3.93 mM FeSO4/g dw respectively).
Table 2. IC50 of DPPH, ABTS, hydroxyl radical scavenging activities, ferric-reducing power and iron chelation of R. sativus bulb extracts.
In addition, the aqueous extract was able to significantly chelate the iron (II) better than the methanol extract (IC50 = 382.74 ± 10.72 and 56.16 ± 8.55 mM/g dw, respectively). Thus, the aqueous extract of radish bulb can be able to afford protection to susceptible reactive oxygen species-mediated damage via a chelation mechanism.
Anti-tumor activity
Cells viability
Both methanol and aqueous extracts of R. sativus bulb were tested on the viability of glioblastoma U-87 MG cell line using the MTT assay. Methanol extract showed a significant cytotoxicity with IC50 at 241.35 ± 15.21 µg/mL after 72 h while no effect was noted for aqueous extract for the tested doses (IC50 > 300 µg/mL). Positive control, doxorubicin showed cytotoxcicity with an IC50 of 0.64 ± 0.15 µg/mL after the same time of incubation. At a concentration of 200 µg/mL, methanol does not affect the viability of U-87 MG cell lines after 24 h, 48 h and 72 h of incubation time (). Thus, the activities of our extracts are not due to the toxic effect of solvents. Therefore, for the further tests, we operated under this concentration to study the specific effects of extracts.
Figure 5. Effect of R. sativus extracts on U-87 MG cells viability.. U-87 MG cells were incubated for 24, 48 and 72 h with different concentrations of R. sativus extracts (A) Methanol extract; (B) Aqueous extract. Doxorubicin (Dox) was used as positive control and water as negative one. All experiments were made in triplicates. Values were expressed as means ± SD, *Indicates significant differences (p < .05).
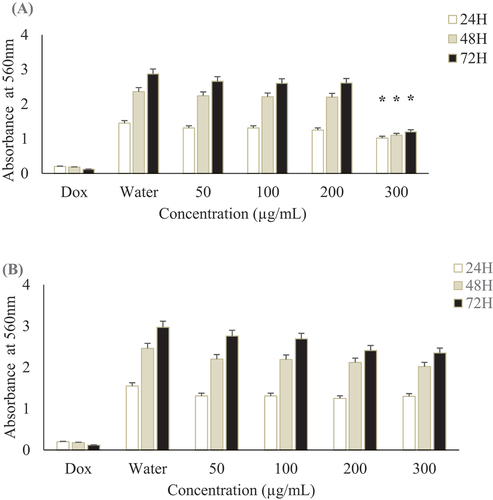
Cell adhesion inhibition
In order to investigate the effects of radish extracts, we performed cell adhesion assays using purified ECM proteins (fibronectin (Fn), fibrinogen (Fg)) and the nonspecific poly-L-Lysine (PLL). Methanol extract blocked notably the adhesion of human glioblastoma cells U-87 MG to fibronectin, fibrinogen, and the nonspecific poly-L- Lysine with IC50 values of 20.46 ± 2.52, 12.23 ± 1.41; and 16.21 ± 1.92 µg/mL. Meanwhile, no effect was observed for the aqueous extract compared to water control (, ). In the same way, the use of 4-methylthio-3-butenyl isothiocyanate, the major compound of methanol extract, alone strongly inhibited the tumor cell adhesion to ECM proteins fibronectin, fibrinogen, and poly-L-Lysine with an IC50 of 0.63 ± 0.09; 1.27 ± 0.18 and 0.95 ± 0.12 µg/mL respectively. Doxorubicin inhibited the adhesion of cancer cells to the same ECM proteins at an IC50 of 0.42 ± 0.06; 0.98 ± 0.015 and 0.69 ± 0.11 µg/mL (, ).
Figure 6. Radish methanol extract effect on various ECM in cell adhesion assays. Glioblastoma cells were preincubated with 10, 20, 50 and 100 µg/mL in radish methanol extract for 30 min at room temperature and then added to wells coated with 10 µg/mL fibronectin (Fn), 10 µg/mL fibrinogen (Fg) or 50 µg/mL (PLL) and allowed to attach for 1 h at 37°C. Values were expressed as means ± SD, *Indicates significant differences (p < .05).
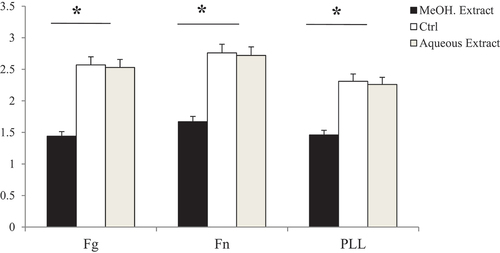
Figure 7. Cell adhesion assay of (A) 4-methylthio-3-butenyl isothiocyanate and (B) positive control doxorubicin (Dox) on different ECM proteins. 4-methylthio-3-butenyl isothiocyanate and posititive control doxorubicin inhibited with the same manner the adhesion of U-87 MG to fibronectin (Fn), fibrinogen (Fg) and poly-L-Lysine (PLL).
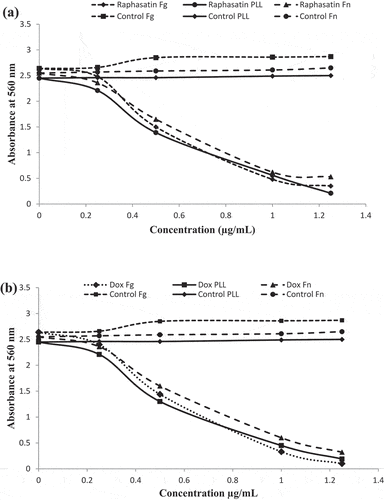
Table 3. IC50 (µg/mL) of radish extracts, raphasatin, and positive control doxorubicin against the tested ECM in dis-adhesion test.
Cell proliferation inhibition
Using 0.5 µg/mL of 4-methylthio-3-butenyl isothiocyanate for 4 days totally inhibited cell proliferation (). To our knowledge, these are the first dis-adhesion and anti-proliferation activities reported for R. sativus methanol extract and rapahsatin on U-87 MG cells.
Figure 8. 4-methylthio-3-butenyl isothiocyanate and doxorubicin (Dox) effects on U-87 MG cells proliferation. After 4 days of incubation with 0.5 µg/mL of raphasatin or doxorubicin, wells were washed with PBS and the cells were fixed with 1% glutaraldehyde, stained with 0.1% crystal violet and quantified by absorbance at 560 nm.
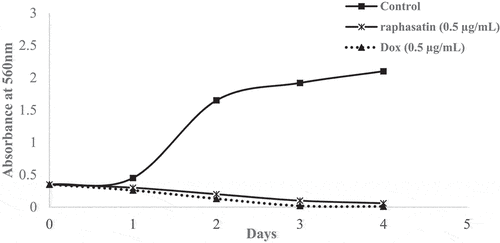
Discussion
The total phenol content of radish has been previously well investigated.[Citation22,Citation23] Many factors, including plant breeding, ontogenetic stage, post-harvest, handling, abiotic factors and polarity of solvents influenced phenol compound synthesis and extraction.[Citation24] In our study, we found three times much high phenol content in aqueous extract of Tunisian radish than in Indian one used in the study of Beevi et. al with 180,33 ± 9.61 and 63.54 ± 3.62 µg GAE/mg dw respectively.[Citation22] Generally, phenol and flavonoid content of an extract are directly proportional to the antioxidant activity present in that extract. The antioxidant property of phenols is due to the presence of a benzene ring that is characterized by the ability to donate electrons. This is in accordance with our results. In fact, the aqueous extract, rich in polar compounds such as polyphenols and flavonoids, showed higher antioxidant and antiradical power. However, in a our previous study on Petroselinum crispum aerial parts, a significantly higher total phenol, flavonoid content and antioxidant activity were found in the methanol extract.[Citation21]
The use of DAD allows peak identification and purity that generally reached 1.00 in our experiments. Five phenol compounds were found in the aqueous extract. Similar results were found by Beevi et al. with the presence of catechin in Indian radish.[Citation22] Using gas chromatography-mass spectrophotometry, we were able to identify the most abundant compound in methanol extract, 4-methylthio-3-butenyl isothiocyanate (raphasatin) at 20%. Our results are similar to those found by Abbes et al. using the same Tunisian variety of R. sativus.[Citation13] However, Beevi et al. found several isothiocyanates in hexan extract of Indian radish roots, including sulforaphene.[Citation25] This confirms that extraction yield is influenced by the origin of plants, solvent used for extraction and analysis method.
Isothiocyanates are volatile secondary metabolites that derive from the enzymatic hydrolysis of glucosinolates, in numerous plants belonging to the Brassicaceae family, by myrosinase (EC 3.2.1.147) after tissue damage.[Citation26] These components give the characteristic smell and pungent flavor of these plants. Isothiocyanates are general biocides with well-documented anticancer activity.[Citation13] They have been associated with a reduced risk of cancers of lung, stomach, breast, prostate, pancreas, colon, and rectum.[Citation27] However, no activity against human glioblastoma has been reported.
Using the MTT test, methanol extract of radish showed significant in vitro cytotoxicity against U-87 MG cancer cells with an IC50 at 241.35 ± 15.21 µg/mL after 72 h while no effect was noted for aqueous extract. Our results are similar to those found in our previous study using P. crispum and by Shaabani et al. using Dracocephalum kotschyi extracts.[Citation21,Citation28]
Anarchic cell proliferation and adhesion are key steps in cancer development.[Citation25] Integrins are cell surface glycoproteins that are implicated in all cell adhesion processes by linking cancer cells to ECM proteins through the formation of cell adhesion complexes, and causing activation of intracellular signaling pathways implicated in the modulation of cell proliferation and metastasis.[Citation29] The anticancer mechanisms are based on inhibition of this cell adhesion, proliferation, tumor invasion, and angiogenesis. In this context, radish methanol extract exhibited high dis-adhesion effect to fibronectin, fibrinogen and poly-L- Lysine with IC50 values of 20.46 ± 2.52; 12.23 ± 1.41 and 16.21 ± 1.92 µg/mL respectively. However, no dis-adhesion effect was noted for the aqueous extract which is rich on polyphenols and flavonoids. This disagrees with our previous results conducted on the antiglioblastoma effect of P. crispum polyphenols.[Citation21] This contradiction can be explained by the difference in the composition and concentrations of polyphenols in radish that may have antagonistic effects. In another experiment, the most abundant compound of radish methanol extract, 4-methylthio-3-butenyl isothiocyanate used individually inhibited significantly the adhesion of U-87 MG cells to the used ECM comparing to the positive control doxorubicin, with IC50 of 0.63 ± 0.09; 1.27 ± 0.18 and 0.95 ± 0.12, respectively. Raphasatin is a highly electrophile isothiocyanate capable of reacting with sulfhydryl groups and binding to cystein, altering integrin functions such as α3 integrin, which is overexpressed in glioma cells.[Citation30,Citation31] 4-methylthio-3-butenyl isothiocyanate at 0.5 µg/mL inhibited totally U-87 MG cell proliferation after 4 days of incubation compared to the positive control doxorubicin. A previous study showed that artemisinin-isothiocyanate derivates exhibited potent antitumor effects against glioblastoma multiforme U-87 MG in vitro at a dose of 7.41 mM after 24 h.[Citation32] Induction of apoptosis in glioblastoma cells by the inhibition of the PI3K/Akt-ERK signaling pathway has been observed with several isothiocyanates in many types of cancer including colorectal, breast, lung and prostate cancers.[Citation33] 4-methylthio-3-butenyl isothiocyanate has been shown to reduce prostate and esophageal cancer cell growth by interfering with signaling pathways, arresting the cell cycle via ROS production and causing apoptotic cell death.[Citation13,Citation34] In the same way, benzyl and phenethyl isothiocyanates, inhibit human brain glioblastoma multiforme GBM-8401 cell xenograft tumors in nude mice in vivo and in vitro by upregulating caspase 9 and 3 and increasing the proapoptotic protein Bax levels.[Citation35,Citation36] In addition, a disruption of cytoskeleton was observed in HT29 cells treated with allyl-isothiocyanates.[Citation37] Other intrinsic apoptotic pathways were also induced, including the release of cytochrome C, enhancement of mitochondrial membrane potential loss (MMP), stimulation of NF-kB pathway and a decrease of the anti-apoptotic protein Bcl 2.[Citation38,Citation39]
Conclusion
The present study clearly indicates that 4-methylthio-3-butenyl isothiocyanate from R. sativus methanol extract can be used to treat human glioblastoma in vitro. This anticancer activity can be related to inhibiting cell adhesion to fibronectin, fibrinogen, and poly-L-lysine with IC50 of 0.63 ± 0.09; 1.27 ± 0.18 and 0.95 ± 0.12 µg/mL, respectively, and proliferation at a dose of 0.5 µg/mL. Further studies are needed to fully understand the mode of action underlying this effect. The antioxidant properties of aqueous extract are primarily related to its phenol content.
Acknowledgments
Special thanks to Dr. Riadh Marouchi for his technical and scientific support.
Disclosure statement
No potential conflict of interest was reported by the author(s).
References
- “Cancer Facts & Figures”. American Cancer Society. 2016. Atlanta.
- Zhang, C.; Yuan, X.; Li, H.; Zhao, Z.; Liao, Y.; Wang, X.; Su, J.; Sang, S.; Liu, Q. Anti-cancer Effect of Metabotropic Glutamate Receptor 1 Inhibition in Human Glioma U-87 MG Cells: Involvement of PI3K/ Akt/mTOR Pathway. Cell. Physiol. Biochem. 2015, 35, 419–432. DOI: 10.1159/000369707.
- Bambury, R. M.; Morris, P. G. The Search for Novel Therapeutic Strategies in the Treatment of Recurrent Glioblastoma Multiforme. Expert Rev. Anticancer. Ther. 2014, 14, 955–964. DOI: 10.1586/14737140.2014.916214.
- Kamiya-Matsuoka, C.; Gilbert, M. R. Treating Recurrent Glioblastoma: An Update. CNS Oncol. 2015, 4, 91–104. DOI: 10.2217/cns.14.55.
- Khamessi, O.; Ben Mabrouk, H.; Othman, H.; ElFessi- Magouri, R.; De Waard, M.; Hafedh, M.; Marrakchi, N.; Srairi-Abid, N.; Kharrat, R. RK, the First Scorpion Peptide with Dual Disintegrin Activity on a1b1 and avb3 Integrins. Int. J. Biol. Macromol. 2018, 120, 1777–1788. DOI: 10.1016/j.ijbiomac.2018.09.180.
- Liddington, R. C.; Ginsberg, M. H. Integrin Activation Takes Shape. J. Cell. Biol. 2002, 158, 833–839. DOI: 10.1083/jcb.200206011.
- Raguz, S.; Yagüe, E. Resistance to Chemotherapy: New Treatments and Novel Insights into an Old Problem. Br. J. Cancer. 2008, 99, 387–391. DOI: 10.1038/sj.bjc.6604510.
- Zare, E.; Pourseyedi, S.; Khatami, M.; Darezereshki, E. Simple Biosynthesis of Zinc Oxide Nanoparticles Using Nature’s Source, and It’s in Vitro bio-activity. J. Mol. Struct. 2017, 1146, 96–103. DOI: 10.1016/j.molstruc.2017.05.118.
- Shishu Kaur, I. P.; Kaur, I. P. Inhibition of Cooked food-induced Mutagenesis by Dietary Constituents: Comparison of Two Natural Isothiocyanates. Food Chem. 2009, 112, 977–981. DOI: 10.1016/j.foodchem.2008.07.019.
- Shukla, S.; Chatterji, S.; Mehta, S.; Rai, P. K.; Singh, R. K.; Yadav, D. K.; Watal, G. Antidiabetic Effect of Raphanus Sativus Root Juice. Pharm. Biol. 2011, 49, 32–37. DOI: 10.3109/13880209.2010.493178.
- Hanlon, P. R.; Barnes, D. M. Phytochemical Composition and Biological Activity of 8 Varieties of Radish [Raphanus Sativus L.] Sprouts and Mature Taproots. J. Food Sci. 2011, 76(1), 185–192. DOI: 10.1111/j.1750-3841.2010.01972.x.
- Gilani, A. H.; Ghayur, M. N. Pharmacological Basis for the Gut Stimulatory Activity of Raphanus Sativus Leaves. J. Ethnopharmacol. 2004, 95, 169–172. DOI: 10.1016/j.jep.2004.06.038.
- Salah-Abbès, J. B.; Abbès, S.; Haous, Z.; Oueslati, R. Raphanus Sativus Extract Prevents and Ameliorates zearalenone-induced Peroxidative Hepatic Damage in Balb/c Mice. J. Pharm. Pharmacol. 2009, 61, 1545–1554. DOI: 10.1211/jpp/61.11.0015.
- Zaman, R. Study of Cardioprotective Activity of Raphinus Sativus L. in the Rabbits. Pak. J. Biol. Sci. 2004, 7, 843–847. DOI: 10.3923/pjbs.2004.843.847.
- Barillari, J.; Cervellati, R.; Costa, S.; Guerra, M. C.; Speroni, E.; Utan, A.; Iori, R. Antioxidant and Choleretic Properties of Raphanus Sativus L. Sprout (Kaiware Daikon) Extract. J. Agric. Food Chem. 2006, 54, 9773–9778. DOI: 10.1021/jf061838u.
- Kim, K. H.; Moon, E.; Kim, S. Y.; Choi, S. U.; Lee, J. H.; Lee, K. R. 4-methylthio-butanyl Derivatives from the Seeds of Raphanus Sativus and Their Biological Evaluation on anti-inflammatory and Antitumor Activities. J. Ethnopharmacol. 2014, 151, 503–508. DOI: 10.1016/j.jep.2013.11.003.
- Kim, W. K.; Kim, J. H.; Jeong, D. H.; Chun, Y. H.; Kim, S. H.; Cho, K. J.; Chang, M. J. Radish [Raphanus Sativus L. Leaf] Ethanol Extract Inhibits Protein and mRNA Expression of ErbB[2] and ErbB[3] in MDA-MB-231 Human Breast Cancer Cells. Nutr. Res. Pract. 2011, 5, 288–293. DOI: 10.4162/nrp.2011.5.4.288.
- Aissani, N.; Coroneo, V.; Fattouch, S.; Caboni, P. Inhibitory Effect of Carob [Ceratonia Siliqua] Leaves Methanolic Extract on Listeria Monocytogenes. J. Agric. Food Chem. 2012, 60, 9954–9958. DOI: 10.1021/jf3029623.
- Albouchi, F.; Avola, R.; Dico, G.; Calabrese, V.; Graziano, A.; Abderrabba, M.; Cardile, V. Melaleuca Styphelioides Sm. Polyphenols Modulate Interferon gamma/histamine- Induced Inflammation in Human NCTC 2544 Keratinocytes. Molecules. 2018, 23, 2526. DOI: 10.3390/molecules23102526.
- Dorman, H. J. D.; Lantto, T. A.; Raasmaja, A.; Hiltunen, R. Antioxidant, pro-oxidant and Cytotoxic Properties of Parsley. Food Funct. 2011, 2, 237–328. DOI: 10.1039/c1fo10027k.
- Aissani, N.; Albouchi, F.; Sebai, H. Anticancer Effect in Human Glioblastoma and Antioxidant Activity of Petroselinum Crispum L. Methanol Extract. Nutr. Cancer. 2020, 73, 2605–2613. DOI: 10.1080/01635581.2020.1842894.
- Beevi, S. S.; Mangamoori, L. N.; Gowda, B. B. Polyphenolics Profile and Antioxidant Properties of Raphanus Sativus L. Nat. Prod. Res. 2012, 26, 557–563. DOI: 10.1080/14786419.2010.521884.
- Chang, H. P.; Thanislas, B. B.; Soo, Y. P.; Sun, J. K.; Mariadhas, V. A.; Naif, A. D.; Jae, K. K.; Sang, U. P. Metabolic Profiling and Antioxidant Assay of Metabolites from Three Radish Cultivars [Raphanus Sativus]. Molecules. 2016, 21, 157. DOI: 10.3390/molecules21020157.
- Bruni, R.; Sacchetti, G. Factors Affecting Polyphenol Biosynthesis in Wild and Field Grown St. John’s Wort [Hypericum Perforatum L. Hypericaceae/Guttiferae]. Molecules. 2009, 14, 682–725. DOI: 10.3390/molecules14020682.
- Beevi, S. S.; Mangamoori, L. N.; Subathra, M. J.; Edula, R. J. Hexane Extract of Raphanus Sativus L. Roots Inhibits Cell Proliferation and Induces Apoptosis in Human Cancer Cells by Modulating Genes Related to Apoptotic Pathway. Plant Foods Hum. Nutr. 2010, 65, 200–209. DOI: 10.1007/s11130-010-0178-0.
- Aissani, N.; Tedeschi, P.; Maietti, A.; Brandolini, V.; Garau, L. V.; Caboni, P. Nematicidal Activity of Allylisothiocyanate from Horseradish [Armoracia Rusticana] Roots against Meloidogyne Incognita. J. Agric. Food Chem. 2013, 61, 4723–4727. DOI: 10.1021/jf4008949.
- Delphy, D. S. P.; Harini, P.; Assvitha, S.; Kalairasi, A.; Ganesh, S.; Umamaheswari, A. Phytochemical Investigation and Anticancer Activity of Leaf Extract of Raphanus Sativus Var. Sativus. IJRPP. 2016, 1, 39–45.
- Shaabani, M.; Mousavi, S. M.; Azizi, M.; Ashraf Jafari, A. A. Cytotoxic and Apoptogenic Effects of Dracocephalum Kotschyi Boiss Extracts against Human Glioblastoma U-87 MG Cells. Avicenna J. Phytomed. 2020, 10, 594–603.
- Seguin, L.; Desgrosellier, J. S.; Weis, S. M.; Cheresh, D. A. Integrins and Cancer: Regulators of Cancer Stemness, Metastasis, and Drug Resistance. Trends. Cell Biol. 2015, 25, 234–240. DOI: 10.1016/j.tcb.2014.12.006.
- Nakamura, Y.; Miyoshi, M. Electrophiles in Food; the Current Status of Isothiocyanates and Their Chemical Biology. Biosci. Biotechnol. Biochem. 2010, 74, 242–255. DOI: 10.1271/bbb.90731.
- Nakada, M.; Nambu, E.; Furuyama, N.; Yoshida, Y.; Takino, T.; Hayashi, Y.; Sato, H.; Sai, Y.; Tsuji, T.; Miyamoto, K.-I., et al. Integrin α3 Is Overexpressed in Glioma stem-like Cells and Promotes Invasion. Br. j. cancer. 2013, 108, 2516–2524. DOI: 10.1038/bjc.2013.218.
- Chan Myae, N.; Xiaolin, Z.; Junfeng, L.; Huijuan, L.; Jiamin, W.; Simona, R.; Mingtao, L.; Ou-yang, Y.; Rongbiao, P.; Xixin, H. Synthesis and anti-glioblastoma Effects of Artemisinin Isothiocyanate Derivatives. Rsc. Adv. 2018, 8, 40974–40983. DOI: 10.1039/C8RA08162J.
- Atwell, L. L.; Beaver, L. M.; Shannon, J.; Williams, D. E.; Dashwood, R. H.; Ho, E. Epigenetic Regulation by Sulforaphane: Opportunities for Breast and Prostate Cancer Chemoprevention. Curr. Pharmacol. Rep. 2015, 1, 102–111. DOI: 10.1007/s40495-014-0002-x.
- Hirata, T.; Cho, Y. M.; Suzuki, I.; Toyoda, T.; Akagi, J. I.; Nakamura, Y.; Numazawa, S.; Ogawa, K. 4-Methylthio-3-butenyl Isothiocyanate [MTBITC] Induced Apoptotic Cell Death and G2/M Cell Cycle Arrest via ROS Production in Human Esophageal Epithelial Cancer Cells. Toxicol. Sci. 2019, 44, 73–81. DOI: 10.2131/jts.44.73.
- Yi-Shih, M.; Jen-Jyh, L.; Chin-Chung, L.; Jin-Cherng, L.; Shu-Fen, P.; Ming-Jen, F.; Fei-Ting, H.; Jing-Gung, C. Benzyl Isothiocyanate Inhibits Human Brain Glioblastoma Multiforme GBM 8401 Cell Xenograft Tumor in Nude Mice in Vivo. Environ. Toxicol. 2018, 33, 1097–1104. DOI: 10.1002/tox.22581.
- Yu-Cheng, C.; Meng-Ya, C.; Hsu-Tung, L.; Chiung-Chyi, S.; Tomor, H.; Yea-Jiuan, L.; Sai-Chuen, W. R.; Kuang-Chi, L.; Fei-Ting, H.; Jing-Gung, C. Phenethyl Isothiocyanate Inhibits in Vivo Growth of Xenograft Tumors of Human Glioblastoma Cells. Molecules. 2018, 23, 2305. DOI: 10.3390/molecules23092305.
- Smith, K. T.; Lund, K. E.; Parker, M. L.; Clarke, G. R.; Johnson, T. I. Allyl-isothiocyanate Causes Mitotic Block, Loss of Cell Adhesion and Disrupted Cytoskeletal Structure in HT29 Cells. Carcinogenesis. 2004, 25, 1409–1415. DOI: 10.1093/carcin/bgh149.
- Fulda, S. Cell death-based Treatment of Glioblastoma. Cell Death. Dis. 2018, 9, 9121. DOI: 10.1038/s41419-017-0021-8.
- McDonald, F. E.; Ironside, J. W.; Gregor, A.; Wyatt, B.; Stewart, M.; Rye, R.; Adams, J. H., and Potts, W. W. The Prognostic Influence of Bcl-2 in Malignant Glioma. Br. J. Cancer. 1899-1904, 2002(86), 14.