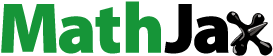
ABSTRACT
This study attempted to optimize the extraction conditions (liquid‒solid ratio, temperature and time) of phenolic yield from Cleome (Cleome arabica L.) fruits extract by the surface response methodology “Box‒Behnken plan” to identify and quantify polyphenolic compounds and test their antioxidant activity. A molecular docking method was developed to clarify the anti-apoptotic effects of bioactive molecules of cleome fruits. Optimal conditions were found at a ratio of 8 ml/g, 4°C and 2 h when the maximum yield was 27.24%. The total phenolic and flavonoid content assessed by the Folin-Ciocalteu and Quercetin methods were 195.20 mg gallic acid equivalent/g dry weight (mg GAE/g DW) and 51.20 mg quercetin equivalent/g dry plant weight (mg QE/g DW), respectively. HPLC and FT-IR protocols revealed the presence of five polyphenolic compounds of which catechin was found to be the most abundant. The antioxidant activity of the extract was quantified by DPPH and FRAP tests, and the IC50 values reached 6.125 mg/ml and 2.9 mg/ml, respectively. In silico analysis confirmed the beneficial effects of phenolic compounds. The results revealed significant antioxidant and antiapoptotic activities of phenolic compounds of cleome fruits and may be useful in the pharmaceutical and food industries with appreciable human health-promoting properties.
Introduction
The use of plant capital in each country of the world based on species diversification, especially to treat all types of diseases, is seriously encouraged and increasing. This strategic choice can help minimize the frequent side effects of synthetic drugs. Many plants can synthesize numerous secondary metabolites (commonly named bioactive compounds) such as phenolic compounds and essential oils with beneficial effects.[Citation1] In traditional use, the strategic applications or physiological mechanisms of action are usually incompletely elucidated and scientifically unapproved. Indeed, these secondary metabolites exhibit diverse chemical structures and a broad spectrum of biological activities. Numerous studies have evaluated these activities.[Citation2–6]
The phytotherapeutic potentials (antioxidant, anti-inflammatory, antinociceptive and hepatoprotective activities) of the genus Cleome were evaluated in previous studies.[Citation7–9] In this regard, Cleome arabica L. has attracted a great deal of interest in antioxidant research. This plant belongs to the Capparaceae family, which includes several species and is widely cultivated in the tropics and subtropics of the world, especially in North Africa.[Citation10]
To achieve optimal extraction conditions of bioactive compounds, an optimization study is needed. Numerous factors such as the liquid‒solid ratio, temperature, time and extraction solvent can have a significant influence on the efficiency of extraction. In general, the optimization of extraction procedures is carried out by an empirical or statistical method.[Citation11] The traditional approach to process optimization is long and expensive. In addition, interaction effects include simultaneous effects of two or more variables that falsify the process output or response. Unlike the conventional empirical method, response surface methodology (RSM) can take into account the possible correlation among the test variables while minimizing the number of experiments. Indeed, RSM is a powerful tool that can provide a complete optimal state to improve an extraction process.[Citation12]
To better understand the mechanism of action and the drug-receptor interaction, a new “in silico simulation” approach was explored.[Citation13] The ability of some drugs to generate apoptosis is believed to be cytotoxic. Initiation of caspases appears to be a common intracellular pathway in many apoptotic pathways. However, to our knowledge, little information is available on the antioxidant properties of phenolic compounds of Cleome arabica L. fruits and on the relationship between these molecules and apoptotic receptors of HEK293 cells.
Therefore, our study was undertaken with the aim to optimize the extraction conditions of the polyphenolic yield of Cleome (Cleome arabica L.) fruits extract by the surface response methodology “Box‒Behnken plan,” and to identify and quantify the phenolic compounds and evaluate their antioxidant activities. Molecular docking was employed to characterize the structures of the ligand protein complexes and to estimate the free binding energies of the docked ligands. This work constitutes a proof of concept to revalorize this plant and increase the economic value of this valuable product as a source of bioactive molecules with properties that might confer benefits to human health.
Material and methods
Plant material
Cleome fruits were randomly collected in the fructification phenological stage (March-April 2019) from Sbeitla-Kasserine (35°14’ N, 9°08’ E). The fruits were dried at room temperature for 15 days, ground to obtain a fine powder, and stored in glass cans at 4°C until use.
Optimization of extraction conditions phenolic yield
Phenolic compounds were extracted from Cleome fruits according to the different parameters shown in . Three variables were picked: the ratio of solvent to plant material (A) was 2–8 ml/g, the extraction temperature (B) was 4–40°C, and the extraction time (C) was 2–6 h to optimize the extraction conditions. After incubation by maceration, the supernatant of each condition was filtered using filter paper and dried in an oven at 40°C. Each parameter was tested between two levels, coded by the lower level (−1), high level (+1) and a central coded value considered zero (0). A composite model central generated 17 treatments with 5 repetitions at the center points (0, 0, 0) to estimate the pure error. The coding of the program proceeds in the following manner according to Diwani et al.[Citation14]
Table 1. Experimental points of Box‒Behnken design and experimental data.
= 1,2,3,4, … . …,k
where xi is the dimensionless measure of an independent changeable, and Xi is the real assessment of an independent variable. Xz is the actual worth of a freelance variation at the middle point. ΔXi is the gradual change in the real value of the variable i corresponding to a change of one unit in the dimension minus the value of the variable (i). summarizes the levels of each factor.
Characterization of plant extract
Preparation of plant extract: Under the Box‒Behnken extraction conditions, the second test yielded the best extraction yield (). According to this condition, dried samples (1 g) were macerated in 8 ml of hydromethanolic solvent (80%). The extract was filtered and dried in an oven at 40°C. The yield of the extract was expressed in terms of percent (%). The final extract was kept in vials at 4°C until the corresponding analyses were conducted.
Total phenolic and flavonoid content: The total phenolic content (TPC) was determined by the Folin-Ciocalteu method.[Citation15] The reaction mixture contained 100 μl of sample and 500 μl of Folin-Ciocalteu reagent (10%). The mixture reacted for 3 min before 400 μl of Na2CO3 (2%) was added. The absorbance was measured at 750 nm after 30 min of incubation of the mixture in the dark. The amount of TPC is expressed in mg of gallic acid equivalent per g of dry weight (mg GAE/g DW).
The total flavonoid content was measured spectrophotometrically in the optimized extract.[Citation16] This procedure consists of the formation of the aluminum-flavonoid complex. A total of 125 μl of the sample was mixed with 37.5 μl NaNO2 (5%) and incubated for 5 min. Then, 37.5 μl AlCl3 (10%) was added to the mixture. After 6 min of incubation, 250 μl NaOH (1 M) and 300 μl H2O were added to the mixture. The maximum absorbance was measured at 510 nm. The results were expressed as mg of quercetin equivalent per g of dry weight (mg QE/g DW). All measurements were performed in triplicate.
HPLC analysis: Phenolic compounds in the cleome fruits extract were identified using HPLC analysis according to the Zheng and Wang[Citation17] method consisting of a vacuum degasser, autosampler, binary pump with a maximum pressure of 400 bar and an Agilent 1260, Agilent Technologies, Germany, equipped with a reversed-phase C18 analytical column of 4.6 × 100 mm and 3.5-μm particle size (Zorbax Eclipse XDB C18). The DAD detector was set to a scanning range of 200–400 nm. The column temperature was maintained at 25°C. The injected sample volume was 2 μl, and the flow rate of the mobile phase was 0.4 mL/min. Mobile phase B was Milli-Q water consisting of 0.1% formic acid, and mobile phase A was methanol. The optimized gradient elution was illustrated as follows: 0–5 min, 10–20% A; 5–10 min, 20–30% A; 10–15 min, 30–50% A; 15–20 min, 50–70% A; 20–25 min, 70–90% A; 25–30 min, 90–50% A; 30–35 min, return to initial conditions. Identification analysis was performed by comparing their retention time with those obtained from the extract. For the quantitative analysis, a calibration curve was obtained by plotting the peak area against different concentrations for each identified compound at 280 nm. Phenolic compound content was expressed in mg of compounds per gram of dry weight (mg/g DW).
Fourier transform infrared spectroscopy (FT-IR): FT-IR analysis of the cleome fruits extract fractions was conducted using a Universal ATR Sampling Accessory infrared spectrophotometer at room temperature. The IR spectrum of the hydromethanolic extract was recorded in the region 400–4500 cm−1.
DPPH• free radical scavenging activity: The antioxidant activity of cleome fruits extract was estimated by the 1,1-diphenyl-2-picrylhydrazyl (DPPH) method according to Ordonez et al.[Citation18] Briefly, the mixture containing 0.5 ml of sample and 1 ml of DPPH (0.4 mM) solution was kept in the dark at room temperature for 30 min. The absorbance was measured at 517 nm. The absorbance of the control consisted of 0.5 ml of hydromethanolic and 1 ml of DPPH• solution. The inhibition percentage (%I) of DPPH* in the steady state was calculated using the following equation:
where A0 is the absorbance of the control, and As is the absorbance of the samples. The results were expressed as the inhibitory concentration of the extract needed to decrease DPPH• absorbance by 50% (IC50). Concentrations are expressed as mg/ml.
Ferric Reducing Antioxidant Power (FRAP): Reducing power determines the antioxidant ability of cleome fruits extract to reduce the ferric iron in the ferricyanide complex Fe3+ to ferrous iron Fe2+. This reduction results in a green color whose intensity is proportional to the reducing power.[Citation19] A total of 2.5 ml phosphate buffer (0.2, pH 6.6) and 2.5 ml K3[Fe(CN)6] (1%) were added to different concentrations of sample (0–1 mg/ml). The mixture was incubated for 20 min at 50°C. Then, 2.5 ml TCA (10%) was added to the reaction and centrifuged for 10 min at 3000 rpm. Finally, 2.5 ml distilled water and 0.5 ml of FeCl3 were added to the 2.5 ml aliquots. The absorbance was read at 700 nm. The tested compound concentration, which provides 50% inhibition (IC50, expressed in mg/ml), was calculated from the graph plotting the inhibition percentage against the extract concentration and confirmed by IBM SPSS software.
In vitro cytotoxicity assay
Cell viability was determined according to the method of Athmouni et al.[Citation20] with some modifications. Cell viability was examined by the 3-(4,5-dimethylthiazol-2-yl)-2,5-diphenyl tetrazolium bromide (MTT) assay. Cells were seeded in 96-well plates (80.000 cells/ml) and incubated overnight in full media (DMEM, RPMI containing 10% FBS). HEK 293 cells were exposed to various concentrations of hydromethanolic extract. A range of cleome concentrations, i.e., 0.3, up to 100 μg.ml−1 was used to test their effects on HEK293 cell viability. The media were eliminated after 48 h, and MTT solution (5 mg/ml) was added to each well containing 100 µl fresh media. After 4 h, the media were removed, and 100 µl of 10% SDS was added to dissolve the Formazan precipitate. The absorbance of solubilized formazan was read at 570 nm using an ELISA reader (Varioskan Thermo Fisher). The IC50 values were then calculated using the following formula:
Molecular docking process
A molecular docking simulation was carried out by the AutoDock 4.2 program packet.[Citation21] The X-ray crystallographic structures of caspase3 (6CKZ), caspase9 (1JXQ) and Bcl-xl (5MFJ) were downloaded from the RSCB protein data bank (PDB). Chain A selected for the docking studies for all receptors. The optimization of all geometries of compounds was carried out using ACD (3D viewer) software (http://www.filefacts.com/acd3d-viewer-freeware-info). The visualization and analysis of interactions were performed using Discovery Studio 2017R2 (https://www.3dsbiovia. com/products/collaborative-science/biovia-discovery-studio/).
Statistical analysis
Data are expressed as the mean ± standard error (ESM). Statistical analyses were processed by IBM SPSS 20 and Minitab 16 software.
Results and discussion
Optimizing extraction conditions for phenolic yield of Cleome
The advantage of using RSM is to understand and estimate the effect of various parameters and their interactions with each other to bring out the response(s). Indeed, it is considered an appropriate approach to optimize a process with one or more responses.[Citation22] The phenolic yields of different experimental conditions are given in . According to tests, this plant showed a significant amount of phenolic yield, which was explained by yields ranging from 1.04% to 27.24%. The Pareto chart and 3D structure indicate all of the parameter effects and their interactions ().
Figure 1. a. Standardized Pareto chart for efficiency and response surface plots of liquid/solid ratio and time extraction interaction on phenolic yield (%). Bb. Standardized Pareto chart for efficiency and response surface plots of liquid/solid ratio and temperature extraction interaction on phenolic yield (%). Cc. Standardized Pareto chart for efficiency and response surface plots of temperature and time extraction interaction on phenolic yield (%).
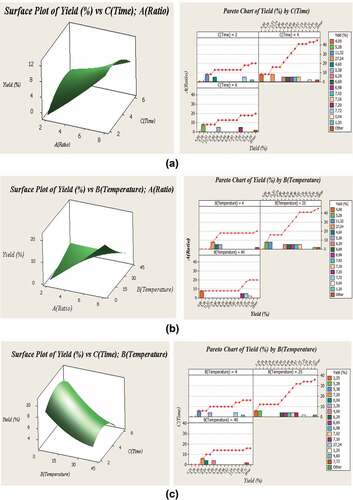
Our data reported that the strengthening in the time values from 4 h generated a decrease in phenolic yield (). Similar to the present study, Mokrani and Madani[Citation23] showed that the increased extraction time after 180 min did not enhance the recoveries of phenolic yield, and with a rise time of 340 min, the yield of the phenolic decreased. In addition, shows the effects of temperature on the phenolic yield of Cleome fruits extract. In this study, the Cleome phenolic yield was significantly enhanced with increasing temperature extraction from 4 to 22°C. Furthermore, the rise in temperature beyond 22°C caused a significant reduction in phenolic yield. A further increase in temperature beyond 22°C resulted in a significant reduction in the phenolic yield. M’hiri et al.[Citation24] reported that improvement of extraction temperature decreases the extraction phenolic yield. The results may be explained by the degradation of phenolic compounds. a and b show the effect of the ratio on the phenolic yield of cleome fruits extract. In this work, the phenolic yield significantly improved with increasing extraction ratio.
Total phenolic and flavonoid content
shows the calibration curves of the gallic acid and quercetin polyphenol standards. A good linear response over a concentration range of 0.1–0.8 mg/ml for gallic acid and 0.005–0.05 mg/ml for quercetin standards was noted.
Polyphenols are mainly accountable for the antioxidant potential of medicinal plants. The total phenolic content can be regarded as an important indicator of the antioxidant properties of plant extracts. The total phenolic and flavonoid amounts in the hydromethanolic extracts were evaluated (). Cleome fruit extract revealed important content of polyphenols and flavonoids (195.20 ± 2.75 mg GAE/g DW and 51.20 ± 1.49 mg QE/g DW, respectively). Compared with previous reports,[Citation25] our results showed the highest amount of TPC. The total phenolic and flavonoid content revealed in the present study are much higher than the values of previous studies of Cleome iberica (39.6 mg/g and 90.7 mg GAE/g) and Cleome amblyocarpa (44.95 mg GAE/g and 23.33 mg CE/g).[Citation26,Citation27]
Table 2. Total phenolic and flavonoid content of cleome fruits extract.
HPLC analysis of phenolic compounds
HPLC analysis was used to determine the composition of the phenolic compounds in the Cleome fruits extract. Peak identification was based on their retention times, their UV–Vis and mass spectra together with data from the standard chromatogram ().
Five phenolic compounds (quercetin, catechin, kaempferol, rosmarinic acid and naringenin) were identified (). The major content was picked for catechin 5.45 mg/g followed by quercetin 3.45 mg, kaempferol 3.23 mg/g and naringenin 2.84 mg/g. Our results showed that rosmarinic acid was the minor compound (0.12 mg/g) among the phenolic compounds mentioned.
These data were correlated with a previous study reporting that Cleome arabica L. was rich in phenolic compounds such as kaempferol and quercetin.[Citation10,Citation28] Several studies determined the potential therapeutic effect of the phenolic compounds cleome, hepatoprotective,[Citation29] antihypercholesterolemic[Citation28] and anti-inflammatory.[Citation30]
FT-IR spectroscopy analysis
The FT-IR spectra related to cleome fruits extract are presented in . The bands observed at 3336.42 cm−1 are related to the stretching vibration of hydroxyl groups (OH phenolic compounds).[Citation31] The absorption bands at 2949.05 cm−1 and 2837.38 cm−1 presented the C-H stretch and O-H stretch, which corresponded to alkanes and carboxylic acids, respectively.[Citation32] This band is related to the vibration of CH2, CH3 groups and flavonoid components.[Citation33] A band was detected at 2130.08 cm−1. This band is related to the presence of C–C stretching of alkynes.[Citation34] The presence of amines/alkenes was determined to peak at 1649.89 cm−1. The band at 1450.02 cm−1 was attributed to the C-C stretching vibration. The absorption band at 1408.10 cm−1 was attributed to C-N stretching amides. The band at 1112.08 cm−1 revealed the presence of C-O band esters. The band at 1015.28 cm−1 must be assigned to C-N stretch or C-O stretch aliphatic amines and alcohols, respectively.
Antioxidant activity
The antioxidant capacity of extracts was studied with two assays: free radical scavenging (DPPH) and reducing power (FRAP). The results are summarized in . The hydromethanolic extract exhibited an IC50 of DPPH (IC50 = 6.125 ± 0.05 mg/ml) and FRAP (IC50 = 2.9 ± 0.05 mg/ml) compared to vitamin C, which was used as a positive control. The results of the FRAP test of the hydromethanolic extract revealed an IC50 = 2.9 ± 0.05 mg/ml higher than the IC50 of vitamin C (IC50 = 0.154 ± 0.002 mg/ml), which is able to scavenge superoxide radicals. The reducing power of Cleome fruits extract is important to reduce the Fe3+/ferricyanide complex to the ferrous form. Therefore, hydromethanolic extract is able to scavenge free radicals at low concentrations. Several studies have shown that cleome possesses strong antioxidant activity.[Citation10,Citation28] Our FT-IR analysis indicated the presence of active groups in this extract, such as O-H groups. In this regard, the antioxidant capacity of phenolic compounds depends on the structure, in particular the number and position of hydroxyl groups.[Citation35] These findings are consistent with the observations of Chen et al.[Citation36] who mentioned that the highest antioxidant capacity of phenolic compounds is related to the lower bond dissociation energies (BDE) of OH groups. In addition, Erdogan Orhan et al.[Citation37] found that catechin exhibited a strong ability to scavenge DPPH radicals.
Table 3. Antioxidant activity of Cleome fruits extract.
Cytotoxicity assay
Our results revealed that up to 200 μg.mL−1 did not significantly inhibit the viability of HEK293 cells. The results showed that the concentrations of cleome <100 μg mL−1 did not show a significant effect (p < .05) on the viability of the cells compared to untreated cells (). Accordingly, the prosurvival effect observed with this extract can be explained by the stimulation of the AKT/PI3K and ERK1/2 pathways. In addition, the AKT pathway enhances cell proliferation/survival and is the prime mediator of a cytoprotective effect by preventing apoptosis. Our findings showed that the MTT test indicated that cleome<100 µg.ml−1 did not impose a significant effect on HEK293 cell viability. This effect is due to the presence of bioactive molecules in cleome, which were identified by HPLC. Similar studies have shown that phenolic compounds have no significant effect on cell viability in HEK293 cells. In agreement with these results, Wu et al.[Citation38] reported that phenolic compounds showed no significant (P < .05) effect on human cell HEK293 viability but did enhance the sensitivity of MRP1–HEK293 cells to topside.
Molecular docking analysis
Molecular docking is a valuable tool to study interactions between proteins and ligands.[Citation39] Autodock vina was supplemented to explore the anti-apoptotic potential of five natural compounds extracted from Cleome fruits against human caspase-3 (PDB code: 6CKZ), caspase-9 (PDB code: 1JXQ) and Bcl-xL (PDB code 5FMJ). The obtained results of binding energies, the number of H-bonds, and visualizations of interactions between proteins in complex with the natural compounds are shown in . All phenolic compounds interact at different receptors with high binding energy, showing their anti-apoptotic effects against the targets. Interestingly, catechin and rosmarinic acid showed potential anti-apoptotic properties due to the highest binding scores with Bcl-xL (−8.0 and −8.2 kcal/mol, respectively). Moreover, these natural compounds have the highest number of H-bonds (). A’ shows that catechin is bound to Bcl-xL through four hydrogen bonds with Ala 142, Asp 107, Thr 109 and Glu 98 together with Pi-sigma interactions with Tyr 101 and Pi-alkyl with Ala149. In summary, catechin offers excellent anti-apoptotic properties.
Figure 7. Representation of 3D structure of catechin with highest docking scores bound to pocket region of Bcl-xl (A-A’), caspase-3 (B-B’) and caspase-9 (C-C’). Micrographs of pocket region with hydrogen bonds and corresponding 2D diagram of interactions.
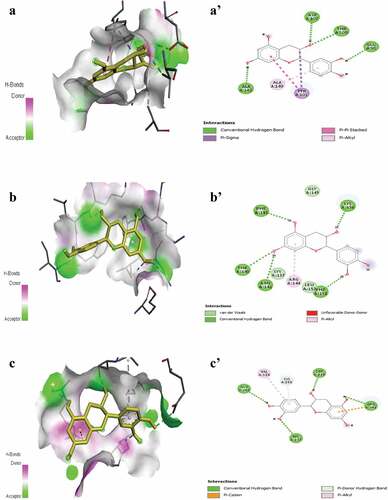
Table 4. Phenolic compounds and human apoptotic receptor interactions: binding affinity and a number of conventional hydrogen bonds.
The next issue is caspase-3 interactions with ligands. However, the highest binding score was found with catechin (−5.9 kcal/mol), followed by rosmarinic acid (−5.6 Kcal/mol). The latter exhibited five hydrogen bonds. It was bound to Lys 156, Phe 143, Thr 140, Asn 141 and Phe 158. Furthermore, this compound has electrostatic interactions encompassing a pi-alkyl bond with Arg 144 and three van der Waals bonds with Leu 157, Lys 137 and Gly 145. There are no unfavorable bonds that may strengthen the potential against caspase-3 ( B’).
The various compounds estimated by Cleome expressed diverse binding affinities with caspase-9, but all exhibited negative binding energies ranging from −6.1 to −6.8 kcal/mol. The highest binding score was found with catechin (−6.8 Kcal/mol) followed by quercetin (−6.5 Kcal/mol). The latter had four hydrogen bonds. It was bound to Glu 288, Ser 339, Arg 341 and His 237 ( C’). It has other electrostatic interactions including a Pi-cation bond with Arg 341, a Pi-donor hydrogen bond with Cys 285 and a Pi-alkyl bond with Val 338. The 3D structure and 2D diagram of interactions show that the selected compounds are bound to caspase-3, caspase-9 and Bcl-XL through a network of electrostatic and hydrogen bonds. shows that Cleome-selected compounds occupied almost the same region. This finding suggests that a synergistic impact is possible. As a consequence, it was argued that the use of whole plant extract could be much better.[Citation40]
Conclusion
The polyphenolic extraction process of Cleome fruits optimized by the response surface methodology “Box‒Behnken plan” is considered an appropriate approach to achieve optimal extraction conditions of bioactive compounds. Cleome fruits have proven an effective potential source of polyphenols as natural antioxidants. The positive correlation between the polyphenolic content and antioxidant capacity confirmed that the polyphenolic constituents are responsible for the antioxidant activity of Cleome. Molecular docking analysis confirmed the beneficial effects of phenolic compounds. The calculated binding energies of the compounds revealed that all identified compounds are beneficial against apoptosis. Cleome fruits extract can be useful in replacing or even decreasing synthetic antioxidants in foods, cosmetics and pharmaceutical products. Thus, further experiments are needed to confirm the safety and efficacy of Cleome extract as a preservative and therapeutic agent.
Acknowledgments
The authors thank the Ministry of Higher Education, Scientific Research and Technology, Tunisia, for supporting this study. The authors also extend their appreciation to the Researchers Supporting Project number RSP- 2021/17 at King Saud University, Riyadh, Saudi Arabia, for funding this project.
Disclosure statement
No potential conflict of interest was reported by the author(s).
References
- Muthu, C.; Ayyanar, M.; Raja, N.; Ignacimuthu, S. Medicinal Plants Used by Traditional Healers in Kancheepuram District of Tamil Nadu, India. J. Ethnobiol. Ethnomed. 2006, 2(1), 43. DOI: 10.1186/1746-4269-2-43.
- Iqbal, J.; Abbasi, B. A.; Mahmood, T.; Kanwal, S.; Ahmad, R.; Ashraf, M. Plant-ExtractMediated Green Approach for the Synthesis of ZnONPs: Characterization and Evaluation of Cytotoxic, Antimicrobial and AntioxidantPotentials. J. Mol. Struct. 2019, 1189, 315–327. DOI: 10.1016/j.molstruc.2019.04.060.
- Itzel, F.; Baetz, N.; Hohrenk, L. L.; Gehrmann, L.; Antakyali, D.; Schmidt, T. C.; Tuerk, J. Evaluation of a Biological Post-Treatment after Full-Scale Ozonation at a Municipal Waste Water Treatment Plant. Water Res. 2020, 170, 115316. DOI: 10.1016/j.watres.2019.115316.
- Khalil, R.; Ali, Q.; Hafeez, M. M.; Malik, A. Phenolic Acid Profiling by rp-hplc: Evaluation of Antibacterial and Anticancer Activities of Conocarpus Erectus Plant Extracts. Biol. Clin. Sci. Res. J. 2020, 2020(1), 1. DOI:10.54112/bcsrj.v2020i1.10.
- Yarrappagaari, S.; Gutha, R.; Narayanaswamy, L.; Thopireddy, L.; Benne, L.; Mohiyuddin, S. S.; Vijayakumar, V.; Saddala, R. R. Eco-Friendly Synthesis of Silver Nanoparticles from the Whole Plant of Cleome Viscosa and Evaluation of Their Characterization, Antibacterial, Antioxidant and Antidiabetic Properties. Saudi J. Biol. Sci. 2020, 27(12), 3601–3614. DOI: 10.1016/j.sjbs.2020.07.034.
- Hashem, N. M.; Shehata, M. G. Antioxidant and Antimicrobial Activity of Cleome Droserifolia (Forssk.) Del. And Its Biological Effects on Redox Status, Immunity, and Gut Microflora. Animals. 2021, 11(7), 1929. DOI: 10.3390/ani11071929.
- Parimalakrishnan, S.; Dey, A.; Smith, A. A.; Manavalan, R. Evaluation of Anti-Inflammatory, Antinociceptive and AntipyreticEffects of Methanol Extract of Cleome Chelidonii. Int. J. Biol. Chem. Sci. 2007, 1(3), 223–228. DOI:10.4314/ijbcs.v1i3.39717.
- Bose, U.; Bala, V.; Ghosh, T. N.; Gunasekaran, K.; Rahman, A. A. A. Antinociceptive, Cytotoxic and Antibacterial Activities of Cleome Viscosa Leaves. Rev. Brasfarmacogn. 2011, 21(1), 165–169. DOI:10.1590/S0102-695X2011005000023.
- Phan, N. M.; Tuoi Do, T. H.; Tuyen Nguyen, L. T.; Nguyen, T. T.; Ngo, Q. L.; Tran, T. D.; Nguyen, Q. H.; Chi Huynh, B. L.; Ky Nguyen, D. X.; Bui, T. D., et al. Hepatoprotection and Phytochemistry of the Vietnamese Herbs Cleome Chelidonii and Cleome Viscosa Stems. J. Chem. 2021, 2021, e5578667. DOI: 10.1155/2021/5578667.
- Ladhari, A.; Omezzine, F.; DellaGreca, M.; Zarrelli, A.; Zuppolini, S.; Haouala, R. Phytotoxic Activity of Cleome Arabica L. and Its Principal Discovered Active Compounds. S. A. J. Botany. 2013, 88, 341–351. DOI: 10.1016/j.sajb.2013.08.016.
- Ghafoor, K.; Choi, Y. H.; Jeon, J. Y.; Jo, I. H. Optimization of Ultrasound-Assisted Extraction of Phenolic Compounds, Antioxidants, and Anthocyanins from Grape (Vitis Vinifera) Seeds. J. Agric. Food Chem. 2009, 57(11), 4988–4994. DOI:10.1021/jf9001439.
- Ferreira, S. L. C.; Bruns, R. E.; Ferreira, H. S.; Matos, G. D.; David, J. M.; Brandão, G. C.; da Silva, E. G. P.; Portugal, L. A.; Dos Reis, P. S.; Souza, A. S., et al. Box-Behnken Design: An Alternative for the Optimization of Analytical Methods. Anal. Chim. Acta. 2007, 597(2), 179–186. DOI: 10.1016/j.aca.2007.07.011.
- Enkhtaivan, G.; Muthuraman, P.; Kim, D. H.; Mistry, B. Discovery of Berberine Based Derivatives as Anti-Influenza Agent through Blocking of Neuraminidase. Bioorg. Med. Chem. 2017, 25(20), 5185–5193. DOI: 10.1016/j.bmc.2017.07.006.
- Diwani, N.; Fakhfakh, J.; Athmouni, K.; Belhaj, D.; El Feki, A.; Allouche, N.; Ayadi, H.; Bouaziz-Ketata, H. Optimization, Extraction, Structure Analysis and Antioxidant Properties of Flavan-3-Ol Polymers: Proanthocyanidins Isolated from Periploca Angusti Folia Using Surface ResponseMethodology. Ind. Crops Prod. 2020, 144, 112040. DOI: 10.1016/j.indcrop.2019.112040.
- Singleton, V. L.; Rossi, J. A. Colorimetry of Total Phenolics with phosphomolybdic-phosphotungstic Acid Reagents. Am. J. Enol. Vitic. 1965, 16(3), 144–158. http://www.ajevonline.org/content/16/3/144.
- John, B.; Sulaiman, C. T.; George, S.; Reddy, V. R. K. Total Phenolics and Flavonoids in Selected Medicinal Plants from Kerala. Int. J. Pharm. Pharm. Sci. 2014, 6, 406–408.
- Zheng, W.; Wang, S. Y. Antioxidant Activity and Phenolic Compounds in Selected Herbs. J. Agric. Food Chem. 2001, 49(11), 5165–5170. DOI:10.1021/jf010697n.
- Ordoñez, A. A. L.; Gomez, J. D.; Vattuone, M. A.; Lsla, M. I. Antioxidant Activities of Sechium Edule (Jacq.) Swartz Extracts. Food Chem. 2006, 97(3), 452–458. DOI: 10.1016/j.foodchem.2005.05.024.
- Oyaizu, M. Studies on Products of Browning Reaction. Jpn. J. Nutr. Diet. 1986, 44(6), 307–315. DOI: 10.5264/eiyogakuzashi.44.307.
- Athmouni, K.; Belhaj, D.; El Feki, A.; Ayadi, H. Optimization, Antioxidant Potential, Modulatory Effect and Anti-Apoptotic Action in of Euphorbia Bivonae Polysaccharides on Hydrogen Peroxide-Induced Toxicity in Human Embryonic Kidney Cells HEK293. Int. J. Biol. Macromol. 2018, 116, 482–491. DOI: 10.1016/j.ijbiomac.2018.04.172.
- Trott, O.; Olson, A. J. Auto Dock Vina: Improving the Speed and Accuracy of Docking with a New ScoringFunction, Efficient Optimization, and Multithreading. J. Comput. Chem. 2010, 31(2), 455–461. DOI: 10.1002/jcc.21334.
- Yetilmezsoy, K.; Demirel, S.; Vanderbei, R. J. Response Surface Modeling of Pb(II) Removal fromAqueous Solution by Pistacia Vera L.: Box–BehnkenExperimental Design. J. Hazard. Mater. 2009, 171(1), 551–562. DOI: 10.1016/j.jhazmat.2009.06.035.
- Mokrani, A.; Madani, K. Effect of Solvent, Time and Temperature on the Extraction of Phenolic Compounds and Antioxidant Capacity of Peach (Prunus Persica L.) Fruit. Sep. Purif. Technol. 2016, 162, 68–76. DOI: 10.1016/j.seppur.2016.01.043.
- M’hiri, N.; Ioannou, I.; Ghoul, M.; Boudhrioua, N. M. Extraction Methods of Citrus Peel Phenolic Compounds. Food Rev. Int. 2014, 30(4), 265–290. DOI: 10.1080/87559129.2014.924139.
- Feriha, B.; Brahim, A.; Reguia, M.; Amar, D.; Mohamed, Y. High Antioxidant Capacities and Anti-Inflammatory Effects of Hammada Elegans Botsch Extracts: An in Vitro Assessment. Curr. Enzyme Inhib. 2019, 15(1), 55–68. DOI: 10.2174/1573408015666190225151916.
- Moridi Farimani, M.; Nazarianpoor, E.; Rustaie, A.; Akhbari, M. Phytochemical Constituents and Biological Activities of Cleome Iberica DC. Nat. Prod. Res. 2017, 31(11), 1329–1332. DOI: 10.1080/14786419.2016.1239093.
- Khlifi, A.; Pecio, Ł.; Lobo, J. C.; Melo, D.; Ben Ayache, S.; Flamini, G.; Oliveira, M. B. P. P.; Oleszek, W.; Achour, L. Leaves of Cleome Amblyocarpa Barr. and Murb. and Cleome Arabica L.: Assessment of Nutritional Composition and Chemical Profile (LC-ESI-MS/MS), Anti-Inflammatory and Analgesic Effects of Their Extracts. J. Ethnopharmacol. 2021, 269, 113739. DOI: 10.1016/j.jep.2020.113739.
- Samout, N.; Bouzenna, H.; Ettaya, A.; Elfeki, A.; Hfaiedh, N. Antihyper Cholesterolemic Effect of Cleome Arabica L. on High Cholesterol Diet Induced Damage in Rats. EXCLI J. 2015, 14, 791–800. DOI: 10.17179/excli2015-169.
- Tigrine, C., and Kameli, A. Antioxidant Activity, Phenolic Content and Hematoprotective Effects of Cleome Arabica Polyphenolic Leaf Extract. Phytotherapie. 2021, 19(3), 156. DOI: 10.3166/phyto-2019-0206.
- Bouriche, H.; Selloum, L.; Tigrine, C.; Boudoukha, C. Effect of Cleome Arabica Leaf Extract on Rat PawEdema and Human Neutrophil Migration. Pharm. Biol. 2003, 41(1), 10–15. DOI: 10.1076/phbi.41.1.10.14698.
- Moyo, S. Effects of Cooking and Drying on the Total Phenolic, Total Flavonoid Content, Antioxidant and Antibacterial Activity of CleomeGynandra (Spider Plant). Technology & Natural Resources, 24–25 . 2016. DOI: 10.15242/IAE.IAE1116433.
- Pillai, L. S., and Nair, B. R. Functional Group Analysis of Cleome Viscosa L. and C. Burmanni W. & A. (Cleomaceae) Extracts by FT-IR. J. Pharmacogn. Phytochem. 2014, 2(6), 120–124.
- Silva, A. J.; Silva, J. R.; de Souza, N. C.; Souto, P. C. S. Membranes from Latex with Propolis for Biomedical Applications. Mater. Lett. 2014, 116, 235–238. DOI: 10.1016/j.matlet.2013.11.045.
- Lingegowda, D. C.; Kumar, J. K.; Prasad, A. G. D.; Gopal, S. FTIR Spectroscopic Studies on Cleome Gynandra – Comparative Analysis of Functional Group before and after Extraction. Rom. J. Biophys. 2012, 22(3–4), 137–143.
- Aberoum, A.; Deokule, S. S. Comparison of Phenolic Compounds of Some Edible Plants of Iran and India. Pak. J. Nutr. 2008, 7(4), 582–585. DOI: 10.3923/pjn.2008.582.585.
- Chen, X.; Ru, Y.; Chen, F.; Wang, X.; Zhao, X.; Ao, Q. FTIR Spectroscopic Characterization of Soy Proteins Obtained through AOT Reverse Micelles. Food Hydrocolloids. 2013, 31(2), 435–437. DOI: 10.1016/j.foodhyd.2012.11.017.
- Erdogan Orhan, I.; Küpeliakkol, E.; Suntar, I.; Yesilada, E. Assessment of Anticholinesterase and Antioxidant Properties of the Extracts and (+)-catechin Obtained from Arceuthobium Oxycedri (D.C.) MBieb (Dwarf mistletoe). S. Afr. J. Bot. 2019, 120, 309–312. DOI: 10.1016/j.sajb.2018.09.023.
- Wu, C.-P.; Calcagno, A. M.; Hladky, S. B.; Ambudkar, S. V.; Barrand, M. A. Modulatory Effects of Plant Phenols on Human Multidrug-Resistance Proteins 1, 4 and 5 (ABCC1, 4 and 5). FEBS J. 2005, 272(18), 4725–4740. DOI: 10.1111/j.1742-4658.2005.04888.x.
- Zarei, A.; Ramazani, A.; Pourmand, S.; Sattari, A.; Rezaei, A.; Moradi, S. In Silico Evaluation of COVID-19 Main Protease Interactions with Honey Bee Natural Products for Discovery of High Potential Antiviral Compounds. Nat. Prod. Res. 2021, 36(16), 4254–4260. DOI: 10.1080/14786419.2021.1974435.
- Rengasamy, K. R. R.; Khan, H.; Gowrishankar, S.; Lagoa, R. J. L.; Mahomoodally, F. M.; Khan, Z.; Suroowan, S.; Tewari, D.; Zengin, G.; Hassan, S. T. S., et al. The Role of Flavonoids in AutoimmuneDiseases: Therapeutic Updates. Pharmacology&Therapeutics. 2019, 194, 107–131. DOI: 10.1016/j.pharmthera.2018.09.009.