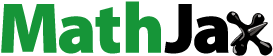
ABSTRACT
Sibiraea laevigata (L.) Maxim (SLM) is a kind of traditional Tibetan food homologous plant with health-protection function. In this paper, PEG-UAEE method was used to extract SLMPs. The effects of different experimental parameters on the extraction efficiency of SLMPs were optimized by RSM. The results showed that the optimal extraction conditions were as follows: PEG-400 concentration of 30%, E/S ratio of 21 U/g, ultrasonic time of 2 h, extract pH value of 4.0, ultrasonic power was kept at 400 W, and ultrasonic temperature of 80°C. The SLMPs yield and content could reach at 10.95% and 21.82%, respectively. Then, the preliminarily structure of SLMPs was characterized by HPLC, FT-IR, and SEM. It was found that the SLMPs were typical hetero polysaccharides considering the main constitutive monosaccharides including galactose (13.34%), glucose (46.76%), rhamnose (8.78%), fructose (2.89%), and arabinose (11.79%). The FT-IR analysis indicated that SLMPs are pyran-type polysaccharides containing α-glycosidic bonds, β-glycosidic bonds, and the SEM analysis showed that SLMPs have a prominent non-crystal structure. Moreover, SLMPs exhibited significant antioxidant activities, the IC50 values of the SLMPs for DPPH, hydroxyl, and superoxide radicals scavenging activities were 0.42, 2.08, and 0.28 mg/mL, respectively. The results suggest that SLMPs have potential applications as natural antioxidants and food ingredients in functional foods.
Introduction
Maxim (SLM) is also named Tibetan Sibiraea angustata, which belongs to Rosaceae (Genus Xianbei), and because of the similar shape with willow leaves, it is commonly known as “willow tea.” There are five described species of Sibiraea plants all over the world, three of which were described from China, including Sibiraea angustata, Maoye Sibiraea and Sibiraea, and only Sibiraea angustata can be used as medicine and tea with its twigs in Tibetans. The potential distribution areas are mainly concentrated in southern Qinghai, western Sichuan, southeastern Gansu, northern Yunnan, Tibet, and other high-altitude areas in China. As Tibetan people’s traditional precious folk medicine,[Citation1] SLM was used as tea with its young leaves and branches. Modern pharmacological studies have shown that the major effective ingredients of SLM include triterpenoids,[Citation2] flavonoids,[Citation3] and polysaccharides.[Citation4] Because of the unique physiological activities and the safety of natural sources, SLM has strong application potential in health care products and functional food. Polysaccharide, a natural pharmaceutical component composed of many similar or different monosaccharides with α-glycosidic or β-glycosidic bonds,[Citation5] is a primary energy material for maintaining life activities.[Citation6] As the primary bioactive substance of SLM, Sibiraea laevigata (L.) Maxim polysaccharides (SLMPs) have various medicinal values because of their various biological activities, such as clearing heat,[Citation7] helping digestion, regulating lipid metabolism,[Citation8] antioxidant,[Citation9] anti-tumour,[Citation10] and enhancing immune activity.[Citation11] Recently, a great diversity of extraction methods have been developed and employed to improve the extraction efficiency of polysaccharides from plants, including ultrasonic-assisted extraction,[Citation2,Citation3,Citation12,Citation13] microwave-assisted extraction,[Citation2] enzyme-assisted extraction,[Citation14] and solvent extraction.[Citation15] Recently, polyethylene glycol (PEG) has received increased attention as a “green” solvent because of its biodegradability and stability in high temperature and strong oxidants. PEG was used more and more as a solvent to extract crude polysaccharides from different kinds of plant leaves, such as superfine ground Auricularia auricular,[Citation16] Pericarpium granati,[Citation17] Ginkgo biloba leaves,[Citation18] and Lonicerae japonica leaves[Citation19] etc.
PEG-based ultrasonic-assisted enzymatic extraction (PEG-UAEE) method has developed a kind of efficient extraction technique to extract polysaccharides from various biomaterials As expected, compared to ultrasonically assisted, PEG reagent provides -OH groups to enhance the interaction of polysaccharides that could improve polysaccharides yield under the double action of PEG and enzyme. However, previous studies mainly focused on extensive phytochemical investigations of SML, while no studies have been reported to date on the extraction of polysaccharides from Sibiraea laevigata (L.) Maxim leaves by PEG-UAEE method and their antioxidant effects.
In this paper, PEG-UAEE was used for the first time to improve the extraction efficiency of SLMPs, and the response surface methodology (RSM) was employed to optimize the extraction parameters. Then, the preliminary structural characterization of SLMPs was analyzed by HPSEC, HPLC, FT-IR, nuclear magnetic resonance (NMR), and scanning electron micrograph (SEM). Moreover, antioxidant activities of SLMPs were also evaluated to expand its application in the function food industries.
Materials and methods
Materials
SLM was obtained from the grassland in the outskirts of Hezuo City, Gannan Tibetan Autonomous Prefecture, Gansu Province (102°54ʹE, 34°58ʹN). The materials were dried at 80°C and ground in a BJ-400 high disintegrator (Yongkang Boou Instrument Co., Ltd., Zhejiang, China), sieved (80-mesh stainless steel screen), and stored in closed desiccators at 4°C before use. Cellulose and pectinase were purchased from Soulebao Biological Reagent Co., Ltd. (Beijing, China). 1,1-Diphenyl-2-trinitrophenylhydrazine (DPPH), trifluoroacetic acid (TFA), and monosaccharide standard products were purchased from Sigma-Aldrich Chemical Co., Ltd. (Louis, USA). The PEG solvents and other reagents used in the experiments were all analytically pure and purchased from Sinopharm Chemical Reagent Co., Ltd. (Beijing, China).
Extraction of polysaccharides
Dry SLM leaves were subjected to degrease by petroleum ether (boiling point: 60–90°C), with help of 85% ethanol to remove some potential impurities, including flavonoids, colored materials, and lipids, etc. Then, the pretreated sample power was filtrated and dried in a DHG-9030A circulating air oven (Shanghai Grows Instrument Co., Ltd., Shanghai, China) overnight at 45°C followed by PEG-based ultrasonic-assisted enzymatic extraction.[Citation20]
Accurately weighted sample powder (3.0 g) was immersed in a 45 mL aqueous PEG complex enzyme solution (cellulose: pectase = 1:2) to improve the efficiency of wall-breaking. Through SB-500DTY ultrasonic extraction (Ningbo Xinzhi Biotechnology Co., Ltd., Zhejiang, China), the suspension was centrifuged (5000 r/min, 10 min) (Heraeus Multifuge X1R, Thermo Co., America), while the residue was re-extracted three times using the same method as mentioned before. The supernatants were combined and concentrated up to one-third of the initial volume using a RE52CS-1 vacuum distillation (Shanghai Yarong Biochemical instrument Co., Ltd., Shanghai, China). The concentrated solution was sufficiently mixed with three times volumes of anhydrous ethanol and stored at 4°C overnight. At last, the precipitate was dried by a vacuum freezing dryer (LGJ-100 F, Thermo Co., USA) at −80°C for 36 h to gain the crude SLMPs.[Citation21]
Determination of the content of SLMPs
The content of SLMPs was measured by the phenol–sulfuric acid method,[Citation22] with D-glucose used as the standard substance. Briefly, accurately weigh 25.00 mg of D-glucose standard into a 250 mL volumetric flask, distilled water was added to dubbed the concentration of 100 μg/ml of standard D-glucose solution. The solution was diluted to 20, 40, 60, 80, and 100 mg/mL D-glucose standard solution with distilled water. Then, 0.2 mL of the standard solution at different concentrations was taken, 0.1 mL of phenol (50 mg/mL) was added, 5 mL of concentrated sulfuric acid was added, the mixture was reacted in a 100°C water bath for 3 min, and the absorbance was determined at 490 nm; the standard curve was drawn through the absorbance and the content of D-glucose. The regression equation was Y =0.6291x-0.0059, R2 = 0.9992, and the formula extraction yield (%) of SLMPs was calculated as follows:
where W0 (g) is the dried SLMPs weight and W (g) is the dried powder of Sibiraea laevigata (L.) Maxim leaves weight.
Purification of crude SLMPs
The crude SLMPs were dissolved in distilled water at a concentration of 0.1 g/mL, and three times Sevag reagent (chloroform: n-butanol = 5:1) was added into the solution and mixed using a ZWYR-2401 shaker (Shanghai Zhicheng Analytical Instrument Manufacturing Co., Ltd., Shanghai, China) for 30 min at room temperature to remove the protein. The operation was repeated for 3 times to purify the polysaccharide. Adding activated carbon (0.06 g) and stirring until the solution is basically colorless under heating. After filtering activated carbon, the solution was first filtered through a microporous membrane (0.45 μm) and purified by 100, 30, and 10k Da ultrafiltration membrane successively using a KM-1812 G ultrafiltrater (Guangzhou Como Instrument Equipment Co., Ltd., Guangdong, China). Finally, a polysaccharide solution with relative molecular weight <10 k Da was obtained and lyophilized before further use.
Molecular weight distribution of SLMPs
The molecular weights (Mw) of SLMPs were measured by a Waters 1260 infinity HPLC system (ARC, Waters Co., USA) with 2410 differential refractive index detector and Ultrahydroge1TM-inear (300 × 7.8 mm, 8 μm, Agilent Co., USA) column. Dextrans (MWs: 1, 5, 10, 21, 40, and 84 kDa) were used as standards for calibration. The column temperature was maintained at 40°C, with a flow rate of 0.8 mL/min and a mobile phase of 0.1 mol/L NaNO2 solution .[Citation23] A linear relationship of the standard curve was used to obtain the regression equation was logMw = −0.6493x+6.1564 (RCitation5 = 0.9987); where Mw is the molecular weight of the polysaccharide; x is the retention time of the glucan standard (min).[Citation6]
Monosaccharide composition analysis of SLMPs
The monosaccharide compositions of the purified SLMPs were analyzed according to the method of Chen et al. with slight modifications.[Citation24] A 10.00 mg of dry purified SLMPs was treated with trifluoroacetic acid (2 mol/L, 5 mL) at 110°C for 5 h. After cooling, adjusted the pH of the solution to 7.0 using 3 mol/L NaOH and centrifuged to obtain polysaccharide hydrolyzates. Then, a 0.2 mL 1-phenyl-3-methyl-5-pyrazolone (PMP) methanol solution (0.5 mol/L) and a 0.2 mL NaOH solution (0.3 mol/L) were mixed with 0.2 mL SLMPs hydrolyzate, and the mixture was incubated at 70°C for 1 h. Finally, the reaction system was neutralized by adding 1 mL trichloromethane and HCl solution (0.3 mol/L, 0.2 mL) and extracting for 3 times. The supernatant was centrifuged by 0.22 μm filter for monosaccharide composition analysis by HPLC.
The determination process was carried out with an Agilent 1260 HPLC system with a DAD detector. The chromatographic conditions were as follows: Agilent ZORBAX Eclipse XDB-C18 column (4.6 × 250 mm, 5 μm, Agilent Co., USA) with a temperature of 28°C, and detection wavelength of 250 nm. The mobile phase was a mixture of phosphate buffer (0.02 mol/L PBS, pH 6.8) and acetonitrile (81:19, v/v)) with a flow rate of 0.8 mL/min; the injection volume was 5 μL. The monosaccharide standards including Rha, Glu, Gal, Fru, and Ara were PMP-labeled and analyzed in the same way as above.
FT-IR spectrometric analysis of SLMPs
The purified SLMPs were mixed with spectroscopic-grade KBr powder (Sigma Aldrich, Shanghai, China), ground, and pressed into pellets for spectral measurement over the frequency range of 4000–400 cm−Citation4 using a 650 FT-IR spectrometer (Tianjin Port East Technology Co., Ltd., Tianjin, China).[Citation25]
Nuclear magnetic resonance analysis of SLMPs
The NMR sample was prepared by mixing the 20 mg freeze-dried SLMPs with 0.5 mL of deuterated water (D2O), and NMR spectra were acquired using a Bruker AVANCE III HD 400 spectrometer (Bruker Co., Germany) operating at 300 MHz for 1H using a double-tuned BBO probe equipped for 5 mm sample tubes. The experiment was performed at 80°C using a single pulse experiment, a recycling delay of 5 s, an acquisition time of 1.66 s, and a spectral width of 10 kHz. The spectra were referenced at 0.0 ppm.[Citation26]
Scanning electron micrograph analysis of SLMPs
A Zeiss EVO18 field emission scanning electron microscope under 20.00 kV (Carl Zeiss AG, Bruker Co., Germany) was used to investigate the morphological features of purified SLMPs. To render the power conductive, the purified SLMPs were fixed on the sample table with conductive adhesive, and then vacuum gold spraying was carried out and the appearance morphology was observed under different multiples.[Citation13]
In vitro antioxidant activity assay
DPPH radical scavenging assay: The DPPH radical scavenging activity of the SLMPs was determined by the method of previously reported method[Citation27] with slight modifications. Briefly, SLMPs was dissolved to afford a series of concentrations (0.0, 0.2, 0.4, 0.6, 0.8, and 1.0 mg/mL). Then, 50 μL of SLMPs solution, 25 μL of DPPH-ethanol solution (0.4 mM) and 100 μL of distilled water were mixed in a 96-well plate. The mixture was kept at 25°C for 30 min in the dark, and the absorbance (Abs) was then measured at 517 nm by a microplate reader (Fisher FC, Thermo Co., USA). For comparison, ascorbic acid (VC) (0.0, 0.2, 0.4, 0.6, 0.8, and 1.0 mg/mL) was used as positive control. The scavenging activity of DPPH radical was calculated by the following formula:
where A0 is the Abs of DPPH solution without SLMPs; A1 is the Abs of SLMPs mixed with DPPH solution, and A2 is the Abs of SLMPs without DPPH solution.
Hydroxyl (·OH) radical scavenging assay: The ·OH radical scavenging activity of the SLMPs was determined according to the reported method[Citation28,Citation29] with a few modifications. First, the reaction mixture contained 1 mL of the SLMPs solution with different concentrations (0.0, 0.2, 0.4, 0.6, 0.8, and 1.0 mg/mL), 1 mL of 9 mM salicylic acid-ethanol, 1 mL of 9 mM FeSO4 solution, and 1 mL of 9 mM H2O2 were mixed well and incubated at 37°C for 30 min in the dark. The Abs of the mixture was measured at 510 nm by a microplate reader (Fisher FC, Thermo Co., USA). The VC (0.0, 0.2, 0.4, 0.6, 0.8, and 1.0 mg/mL) was served as positive control and distilled water was used as control. The capability of scavenging ·OH was calculated using the following equation:
where Ac was the Abs of control (distilled water instead of sample), As was the Abs of the SLMPs solution and Ab was the Abs of the sample without hydroxyl radical.
Superoxide (·O2−) radical scavenging assay: The ·O2− radical scavenging activity of SLMPs was measured according to the previous report[Citation24] with minor modifications. Firstly, the reaction mixture contained 1.0 mL of the SLMPs solution with different concentration (0.0, 0.2, 0.4, 0.6, 0.8, and 1.0 mg/mL) and 2.0 mL of 0.05 M Tris-HCl buffer (pH 8.2) were incubated at 25°C for 30 min in the dark. Then, 0.4 mL of 1,2,3-phentriol (5 mM) was added to keep at 25°C for 4 min. The Abs of the mixture was measured at 510 nm using a microplate reader. VC (0.0, 0.2, 0.4, 0.6, 0.8, and 1.0 mg/mL) was tested under the same conditions. The ability of scavenging ·O2− radical was calculated using the following equation:
where Ai was the rate of Abs change of blank control group and Aj was the rate of Abs change of the SLMPs solution.
Experimental design of optimization of extraction conditions: The effects of PEG molecular weight (200 Da, 400 Da, 600 Da, 800 Da, and 1,000 Da), PEG concentration (15%, 20%, 25%, 30%, 35%), E/S ratio (10.50, 15.75, 21.00, 26.25, and 31.50 U/g), pH (3.0, 4.0, 5.0, 6.0, and 7.0), ultrasonic power (200, 250, 300, 350, and 400 W), and ultrasonic temperature (40, 50, 60, 70, and 80°C), and extraction time (1.0, 1.5, 2.0, 2.5, and 3.0 h) on the SLMPs extraction yield were individually investigated by the method of single-factor experiment design.[Citation29]
Based on the results of the single-factor experimental data, Box–Behnken design (BBD) of four levels and three independent variables (E/S ratio, X1; pH, X2; ultrasonic temperature, X3; and ultrasonic time, X4) was applied to optimize the extraction conditions of SLMPs. In addition, three levels (high, medium, and low, code +1, 0, and −1, respectively) were designed for every variable condition. The experimental design levels for the response surface are exhibited in , and the whole design consisted of 29 experimental points. All trials were performed at least in triplicate. Eperimental data were analyzed by multiple regressions method for fitting the quadratic polynomial model indicated as below.[Citation30]
Table 1. Factors of response surface test design.
where Y is the response variable (SLMPs extraction yield, %); β0 is the constant; and βi, βii, and βij are the regression coefficients for intercept, linearity, square, and interaction, respectively; Xi and Xj are the different independent variables (i ≠ j).
Statistical analysis
The design expert software (Version 8.0.6, Stat-Ease, Inc., Minneapolis, MN, USA) was used for the RSM experiment design. All statistical analyses were performed using SPSS (v.19.0; Chicago, IL, USA), and the data of the results were presented as mean ± SD. In addition, differences among groups were analyzed by one-way analysis of variance, followed by Duncan’s test. P < .05 and P < .01 were considered statistically significant.
Results and discussion
Effects of peg molecular weight, peg concentration, e/s ratio, extracts ph value, ultrasonic power, temperature, and time on the SLMPs extraction yield
In the extraction process of SLMPs, PEG could penetrate the plant cell wall, dissolve the polysaccharides inside, deliver the polysaccharides out from the plant cell wall, and form sufficient hydrogen bonds with polysaccharides to strengthen the interaction, which brings viscosity and polarity to the characteristics of PEG.[Citation31] Research has shown that PEG can enhance the solubility of polysaccharides and facilitate the diffusion factor of extraction solvent so that it can be applied in the extraction of SLMPs; however, both the polarity and viscosity of PEG are greatly dependent on its molecular weight.[Citation16] In this study, their extraction abilities of aqueous PEG solutions at different molecular weights (200, 400, 600, 800, and 1000 Da) were studied. PEG 400 supplied a higher extraction yield of SLMPs with 9.85 ± 0.08%, which was higher than those of the other PEG solutions among all tested extraction solutions. This means that PEG 400 can provide a suitable solution condition to achieve the maximal extraction yield of SLMPs (). Therefore, PEG 400 was used as the extraction solvent in this study.
Figure 1. The effects of (A) PEG molecular weight, (B) PEG concentration, (C) E/S ratio, (D) extracts pH value, (E) ultrasonic power, (F) ultrasonic temperature, and (G) ultrasonic time on the SLMPs extraction yield. Different superscripts (a-e) indicate a significant difference (p < .05).
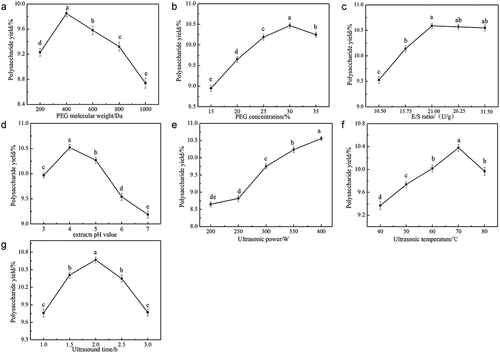
Different concentrations of PEG were tested to determine the effects of viscosity of the solution system on the yield of polysaccharides.[Citation18] It can be seen from that when the PEG concentration was 15%–30%, the polysaccharide yield of SLMPs increased significantly in a concentration-dependent manner. The polysaccharide yield reached the maximum value of 10.47 ± 0.06%, when PEG concentration was greater than 30%. The polysaccharide yield showed a decreased trend. Zhou et al. found that the maximum yield of polysaccharides (7.65 ± 0.08%) was obtained using 30% PEG 400 aqueous solution as solvent to extract polysaccharides from Pericarpium granati .[Citation17] The increase of solvent concentration changed the dissipation factor of the system, which was beneficial to the extraction efficiency of polysaccharides. High PEG concentration results in high viscosity and reduced polysaccharide yield. Thus, the PEG 400 concentration of 30% was the most proper choice in this study.
Plant cell walls consist of rigid cellulose skeletons embedded in a matrix of hemicelluloses (mainly xyloglucans), pectin, and glycoproteins. In order to enhance the extraction yield of polysaccharides, mixed enzymes have been used to catalyze the degradation of cellulose and break the plant cell wall. Chen et al. reported that the polysaccharide extraction yield was improved by increasing the E/S ratio.[Citation32] As shown in , the extraction yield of SLMPs gradually increased when the ratio of E/S ranged from 10.50 to 21.00 U/g, and the maximal extraction yield reached 1.06 ± 0.05%. After that, the extraction yield remains nearly constant. Zhang et al. used mixed enzymes to extract polysaccharides from Ginkgo biloba leaves, which was similar to the trend of extraction rate in this experiment.[Citation16] Hence, the E/S ratio of 21.00 U/g was the suitable extraction condition for this study.
Active sites or functional group regions of enzyme conformation can be altered or controlled by adjusting the pH value. Therefore, the optimal pH value can improve the yield of polysaccharides.[Citation27] As shown in , the yield of SLMPs was firstly increased and then decreased with pH. The optimal pH was 4.0, which led to a SLMPs yield of 10.52 ± 0.06%. The research that studied the effects of different extracts pH values on the yield of polysaccharides from pomegranate peel, they found that when enzymatic hydrolysis time was 20 min, enzymatic hydrolysis temperature was 50°C and pectinase dosage was 0.7%, the polysaccharide yield increased with the increase in extracts’ pH value at first, but when pH value was surpass 5, the polysaccharide yield would decrease, which should be due to the spatial structure and conformation of pectinase were destroyed in alkaline environment, causing the enzyme to lose some catalysis function.[Citation14] Therefore, pH 4.0 was considered to be the optimal value.
Cavitation caused by the continuous improvement of ultrasonic power can result in cell fragmentation under high temperature and pressure environments, and polysaccharide is effectively released and dissolved in the extracts, thus leading to the increasing polysaccharide yield.[Citation33] As shown in , the effects of different ultrasound power (200, 250, 300, 350, and 400 W) were evaluated. The extraction yield of SLMPs increased with ultrasonic power ranging from 200 to 400 W. However, with the increase in ultrasonic power, the yield of polysaccharide tended to be flat; in addition, the structure of polysaccharide was destroyed due to the excessive pressure of the system. In this study, the ultrasonic power of 400 W was ensured as the optimum condition.
High temperatures will increase the enzymatic hydrolysis activity, accelerate the movement of polysaccharide molecules, and enhance the solubility of polysaccharides. Thus, high temperature will lead to an increased polysaccharide yield. As shown in , the influence of different extraction temperatures (40, 50, 60, 70, and 80°C) on the extraction yield of SLMPs (%) was investigated, respectively. When the ultrasonic temperature was set at 70°C, the SLMPs yield reached the maximum value (10.38 ± 0.06%), and higher temperatures did not benefit the extraction. The results were similar to those reported by Chen et al., who found that 80°C was optimal for the yield of polysaccharides from Rosa roxburghii tratt fruit.[Citation24] This may be explained that the increase in temperature accelerates the reaction rate of the whole reaction system.[Citation34,Citation35] When the temperature exceeds 70°C, the destruction of the polysaccharide structure is accelerated. In summary, 70°C was selected as the best ultrasonic temperature for extraction.
The plant cell wall was destroyed by providing sufficient ultrasonic time. shows the influence of ultrasonic time (1.0, 1.5, 2.0, 2.5, and 3.0 h) on the yield of SLMPs. The extraction yields increased to 10.67 ± 0.05% as ultrasonic time increased up to 2.0 h. A longer extraction time, increased viscosity of the extraction liquid, the structural destruction, and decomposition of SLMPs. Our data were similar to the records for the polysaccharides extracted from Tuber huidongense, which found that the extraction yield considerably increased during the initial 40 min and then decreased on further increase in time.[Citation36] Therefore, 2.0 h was selected as the optimum ultrasonic time for extraction.
Box-Behnken design and response surface analysis modeling
The four key parameters (E/S ratio, extraction pH value, ultrasonic time, and ultrasonic temperature) for the extraction of polysaccharides derived from SLM were further optimized by RSM with BBD. The results are presented in , which showed that the SLMPs yield varied from 8.96% to 10.95%. A quadratic polynomial equation between SLMPs yield, and the extraction variables were derived as follows:
Table 2. Response surface test results and analysis of Sibiraea angustata polysaccharide.
Y = 10.88 + 0.10X1 + 0.033X2-0.037X3 + 0.30X4 + 0.070X1X2 + 0.11X1X3-0.087X1X4 + 0.11X2X3 + 0.15X2X4 + 0.015X3X4-0.60X12-0.66X22-0.47X32-0.71X42
where Y is the SLMPs yield (%) and X1, X2, X3, and X4 are the coded values of the tested E/S ratio (U/g), extracts’ pH value, ultrasonic times (h), and ultrasonic temperature (°C), respectively. The effects of independent variables on the SLMPs yield were tested for adequacy and fitness (EquationEq. (1(1)
(1) )) by ANOVA () and identify the significant factors.
Table 3. Analysis of variance and results of regression equation.
Table 4. Relative molecular weight analysis of SLMPs.
The statistical significance of the regression model was checked by the corresponding F-value and P-value, as shown in , the extremely low p-value (p < .0001) and the high F-value (26.13) suggested that the regression model was statistically significant. The linear terms (X1 and X4), quadratic terms (X12, X22, X32, and X42) had significant effects (P < .05), as shown by the regression coefficients, while the interaction term had no significant effect on SLMPs yield. In addition, the coefficient of determination (RCitation5) and the adjusted coefficient determination (RCitation5) were used to evaluate the degree of fitness of the model. In the regression model, R2 of 0.9831 and R2adj of 0.9730 clearly demonstrated that the model was statistically significant. The coefficient of variation (C.V.) was 1.62% showed that the accuracy and the general availability of the polynomial model were adequate. RSM had been used in some studies to extraction conditions of polysaccharides from different plants, such as the extraction yield of polysaccharide from Lonicera japonica leaves,[Citation16] Agaricus bisporus,[Citation17] Pleurotus ferulae,[Citation20,Citation37,Citation26] Lenzites betulina,[Citation10] Hibiscus leaf,[Citation16] and Codonopsis pilosula,[Citation2] etc. Wang et al.[Citation7] All these statistical parameters show that the BBD model is sufficient to accurately predict the extraction rate of polysaccharides under any combination of variable values and is acceptable in terms of experimental errors and reliable for predicting experimental yield
The three-dimensional (3D) response surface was plotted using Design-Expert to analyze the effects of experimental factors and their interactions on the SLMPs yield. In shows that the extraction yield of SLMPs was a function of E/S ratio and extracted pH values when the ultrasonic time (h) and ultrasonic temperature (°C) were fixed at the central points. Results indicated that the extraction yield of SLMPs increased rapidly with increasing E/S ratio to 21.00 U/g, and the extraction yield of SLMPs was found to increase with the increase in extract pH value to 4. In , when the 3D response surface plot was developed for the extraction yield of SLMPs with varying E/S ratio and ultrasonic time at extract pH value and ultrasonic temperature (°C), fixed in the central points. It is indicated that the maximum extraction yield of SLMPs can be achieved when the E/S ratio and ultrasonic time are at the threshold level of 21.00 U/g and 2.0 h, respectively. shows the 3D response surface plot at a varying E/S ratio and ultrasonic temperature at an extract pH value and ultrasonic time (h), fixed at the central points. The extraction yield of SLMPs increased rapidly within the E/S ratio to 21.00 U/g, and the yield extraction of SLMPs increased with the increase in the ultrasonic temperature to 70°C. shows the 3D response surface plot at varying extract pH value and ultrasonic time at E/S ratio (U/g) and ultrasonic temperature (°C), fixed in the central points. It can be seen that the maximum extraction yield of SLMPs can be achieved when the extract pH value and ultrasonic time were 4 and 2 h, respectively. shows the 3D response surface plot at a variable extract pH value, the ultrasonic temperature at E/S ratio (U/g), and ultrasonic time (h), fixed at the central points. The extraction yield of SLMPs increased rapidly within the extract pH value to 4, and the yield extraction of SLMPs increased with the increase in the ultrasonic temperature to 70°C. Wang et al.[Citation38] found that the extraction rate increased with the increase in temperature and pH value but past a certain point, the rate decreased slightly with a further increase in temperature and pH value when they used response surface methodology to optimize the extraction conditions of polysaccharides from Dioscorea opposita thunb. This is consistent with the effect of ultrasonic temperature and extracted pH value in this study. shows the 3D response surface plot at varying ultrasonic temperature and ultrasonic time at E/S ratio (U/g) and extract pH value, fixed at the central points. It can be seen that the maximum extraction yield of SLMPs could be achieved when the ultrasonic temperature and ultrasonic time were 70°C and 2 h, respectively. Wang et al. used the BBD to plotted response surface and study the influence of ultrasonic temperature and ultrasonic time and their interaction on the extraction rate of polysaccharides from Hibiscus leaf under the condition of fixed extract pH value and E/S ratio. They found that when there was a perfect interaction between ultrasonic temperature and ultrasonic time, an elliptical contour could be obtained.[Citation34]
Figure 2. Response surface (3D) showing the effect of (A) E/S ratio and extracts pH value, (B) E/S ratio and ultrasonic time, (C) E/S ratio and ultrasonic power, (D) extracts pH value and ultrasonic time, (E) extracts pH value and ultrasonic temperature and (F) ultrasonic time and ultrasonic temperature on extraction yield of SLMPs.
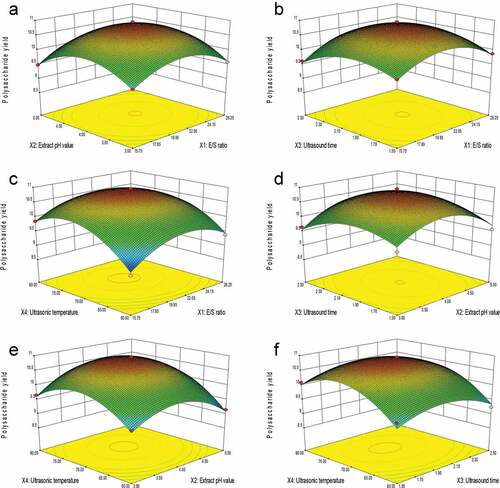
Optimization and model validation
According to the mathematical prediction of the BBD, the optimal PEG-UAEE conditions for each factor with the maximum SLMPs yield (10.95%) obtained by the Design Expert software are as follows: E/S ratio (X1) of 21 U/g, extract pH value (X2) of 4.0, ultrasonic time (X3) of 2.0 h and ultrasonic temperature (X4) at 80°C. Triplicate confirmatory experiments were carried out to validate the adequacy of the mathematical model. The average extraction SLMPs yield was 10.97 ± 0.16% and SLMPs content was 71.82%, which were higher than those reported by Wang et al.[Citation37] The results demonstrate that the RSM approach is appropriate for optimizing the extraction yield of SLMPs.[Citation23]
Molecular weight distribution of SLMPs
Crude SLMPs were purified using optimal purification conditions where the protein and colors were removed by Sevag reagent and activated carbon. Then, the polysaccharide was eluted by ultrafiltration to obtain purified SLMPs. The total carbohydrate content of the purified SLMPs was 85.31%. As shown in , purified SLMPs gave a single symmetrical peak, indicating that SLMPs are homogeneous. The molecular weight of the SLMPs was determined to be 1.5 × 103 Da on HPGPC with reference to standard glucans. The molecular weight of SLMPs was lower than the other molecular weights of published polysaccharides[Citation13,Citation39] The value of Mw/Mn was close to 1, indicating the homogeneity of the molecular weight distribution.
Monosaccharide composition of SLMPs
The monosaccharide composition of the purified SLMPs determined by HPLC-PMP is shown in . The purified SLMPs was composed of rhamnose (Rha), glucose (Glu), galactose (Gal), fructose (Fru), and arabinose (Ara). The molar ratio of Rha, Glu, Gal, Fru, and Ara for purified SLMPs was 8.78:46.76:13.34:2.89:11.79. These results indicated that the purified SLMPs were acidic polysaccharides. Wang et al. used the HPLC to analysis the monosaccharides of the polysaccharides from Epimedium koreanum Nakai treated, and found that Ara was the main monosaccharide (30.1%) in the polysaccharides purified by ion-exchange chromatography, while Glucose (Glc) was the main monosaccharide in the polysaccharides purified by gel chromatography, and the content up to 66.0%, and the monosaccharide composition of SLWPs was similar, indicating that the purification method might affect the structure and physicochemical properties of polysaccharides.[Citation1,Citation8,Citation40]
FT-IR spectrum of SLMPs
FT-IR spectra of purified SLMPs is presented in . The broad and strong absorbance band at 3366.11 cm−Citation4 represented the stretching vibration of O-H in the constituent sugar residues, while the strong band at around 2879.75 cm−Citation4 was associated with the stretching vibration of C-H in the sugar ring. These two absorption peaks are characteristic absorption peaks of sugars, which prove that the extract was polysaccharide.[Citation41,Citation6] The absorption band at 1732.76 cm−Citation4 was due to the bending vibration of bond water. Moreover, the absorption peaks at 1611.16 cm−Citation4 and 1436.25 cm−Citation4 were attributed to the stretching vibrations of the ester carbonyl groups (C = O) and carboxylic groups (COO-), respectively, which indicated that the purified SLMPs were acidic polysaccharides and might be uronic acids in the main chain, and the locations of these peaks were similar to the investigations of Atratylodes macrocephala by FT-IR.[Citation42] The results are consistent with the analytical results of monosaccharide compositions for purified SLMPs as mentioned above. In addition, the strong absorption band at 1060 cm−Citation4 in the range of 1200–1000 cm−Citation4 by C-O-C stretching and angular vibration in the sugar ring suggested that the monosaccharide of purified SLMPs had pyranose rings. Another apparent characteristic absorption at 840.82 cm−Citation4 indicated the presence of α-pyranose in the SLMPs. The absorption at 947.92 cm−Citation4 suggests the presence of a β-anomeric configuration. It can be inferred that SLMPs are α-, β- type polysaccharides according to the stronger peak value of FT-IR spectra and indicating higher polysaccharide content, and that the locations of these peaks were similar to the investigations of Trichosanthin polysaccharide by FT-IR.[Citation43]
NMR spectroscopic analysis of SLMPs
Structure identification and analysis of polysaccharides mostly use Citation4H NMR as the primary method to study the types of glycosidic bonds. The range of δ4.5–5.5 in the Citation4H NMR spectrum is the region where the proton signal mainly exists in the glycosidic bond of the polysaccharide, so there are several proton signals in this region in the Citation4H NMR spectrum, indicating that the sugar has several monosaccharide species, but also the overlap and interference between proton signals lead to the lack of monosaccharide composition analysis.[Citation39] While the other hydrogen signals are mainly concentrated in the narrow region of δ3.3–4.3, and the signal peaks overlap seriously. Among them, δ5.0 is the critical value of the proton signal to distinguish the configuration of pyranose. When the proton shift of the first carbon is greater than 5.0, it is an α-glycoside, and when it is less than 5.0, it is a β-glycoside. The one-dimensional Citation4H NMR spectra of SLMPs can be seen in . It can be seen from the figure that SLMPs have five proton signal peaks in the range of δ4.5 ~ 5.5, which is consistent with the HPLC-PMP results, and anomeric hydrogen appears in the range of δ4.2–4.4 and δ5.0–5.8 signal, indicating that there are both α-glycosidic bonds and β-glycosidic bonds in SLMPs,[Citation44] which was in agreement with the result from FT-IR analysis.
Figure 5. Citation4H NMR spectra of SLMPs.
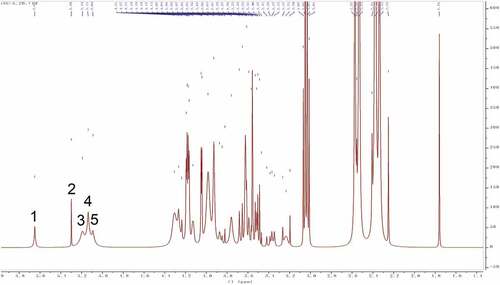
Microstructure of SLMPs
The microscopic scanning electron micrograph (SEM) structure of purified SLMPs () revealed that the surface of particles, which were mostly seen as aggregates of irregular shapes and dimensions. The typical characteristics of amorphous powders were irregular shape and rough surface and accompanied by fold structure with holes in the irregular-shaped particle similar with Macroalgae polysaccharides prepared by physical treatments.[Citation45] It can be proved that SLMPs have prominent amorphous structure and relatively complete structural morphology.
Antioxidant activity of SLMPs
Scavenging activity of DPPH radical: DPPH, as stable nitrogen-centered lipophilic free radical, is usually used for evaluating free radical scavenging activities of antioxidant materials. DPPH· becomes a stable diamagnetic molecule by accepting electron or hydrogen-donating radicals of the antioxidants.[Citation24,Citation36,Citation32]**** As shown in , in the range of 0.0–1.0 mg/mL, there was an apparent dose–response relationship between DPPH radical scavenging ability of SLMPs and VC, which gradually increased with the increase in mass concentration. However, for SLMPs, the scavenging ability was lower than that of VC. DPPH· radical scavenging ability of SLMPs and VC increased with increasing concentration in the range of 0.0–0.2 mg/mL, and all attained their respective maximum value 80.3% and 95.1% at 1.0 mg/mL, and the median inhibition concentration (IC50) of the SLMPs and VC was determined to be 0.42 and 0.21 mg/mL. Cheng et al. used hot water to extract polysaccharides from Epimedium acuminatum Franch polysaccharide (EAPs) showed a pronounced scavenging effect on DPPH radical in a dose-dependent manner. The IC50 values were 1.32 mg/mL, which is lower scavenging activity for DPPH radical than SLMPs.[Citation46] It was indicated that SLMPs could be used as natural antioxidants and could be perspective tools for food preservation and preservation. According to relevant studies, the antioxidant activity of polysaccharides is related to molecular weight, degree of sulphuration, and glycosidic bond, etc. Nevertheless, ultrasonic treatment can reduce molecular weight to improve the biological activity of polysaccharides.
Figure 7. Scavenging effect of SLMPs on (A) DPPH· radicals compared with VC, (B) ·OH radicals compared with VC and (C) ·O2− radicals compared with VC.
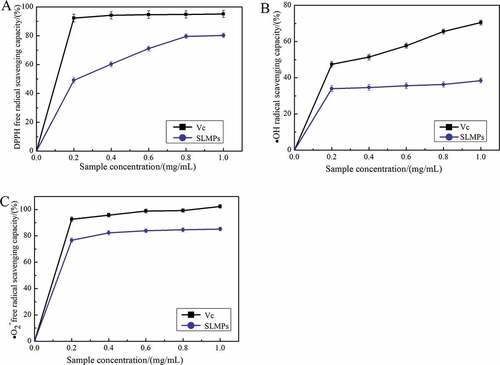
Scavenging activity of OH radicals: The ·OH free radical considered a highly potent oxidant can rapidly cause lipid peroxidation, nucleic acid rupture, and protein decomposition in the body and cause tissue damage or cell death.[Citation32] Accordingly, removing hydroxyl radical is essential for antioxidant defense in cell or food systems. shows that both SLMPs and VC showed concentration dependence on ·OH free radical scavenging ability, which increased gradually with the increase in mass concentration in the range of 0.0–1.0 mg /mL, and VC exhibited the highest ability to scavenge ·OH free radical. When the concentration was 0.4 mg/mL, the scavenging ability of SLMPs on ·OH free radical increased slightly. However, the effect of SLMPs and VC, respectively, reached 38.4% and 70.5% at 1.0 mg/mL. The IC50 value of SLMPs was approximately 2.08 mg/L, which was higher than that of VC (0.51 mg/L). The research found that the polysaccharide from Porphyra haitanensis showed a noticeable scavenging effect on ·OH scavenging ability (22.84%, 2 mg/Ml).[Citation44] The inhibition rate of polysaccharide from Porphyra haitanensis was significantly lower than that of low concentration SLMPs. All the data in ·OH radical assay indicate that the scavenging ability of hydroxyl radicals directly reflects the antioxidant activity of polysaccharides.
Scavenging activity of ·O2− radical: ·O2− free radical is an essential free radical generated by the action of a few enzymes in the body such as xanthine dehydrogenase, fibrinose oxidase, and aldehyde oxidase. Moreover, excessive ·O2− free radical generation was considered the beginning of the body’s accumulation of reactive oxygen species, which leads to a redox imbalance and related harmful physiological effects.[Citation45] As shown in , the concentration of SLMPs was positively correlated with its ·O2− free radical scavenging activity in the range of 0.0–1.0 mg/mL. In addition, the scavenging activity of SLMPs increased from 76.7% to 85.2% as concentration increased from 0.01 to 1.0 mg/mL, which is lower than VC with 102.3% scavenging rates at 1.0 mg/mL. The IC50 value of SLMPs was approximately 0.28 mg/L, which was lower than that of VC (0.20 mg/L). The reports showed that the polysaccharide from Trichosanthin exhibited the maximum ·O2− radical scavenging activity (35.33 ± 0.76%) when the concentration of samples reached 1.28 mg/mL.[Citation43] The mechanism of ·O2− free radical scavenging may be due to the presence of electrophilic groups in polysaccharides, such as aldehydes or ketones.
Although the present results indicate that SLMPs exhibited strong scavenging activities on DPPH, ·OH, and ·OCitation5− free radicals, and it could be explored as a natural antioxidant used in the functional food and pharmaceutical industries, but the structure–activity relationship and the action mechanism of antioxidant in vivo of polysaccharides derived from SLM should be explained clearly in our further study, which can provide useful research underpinnings for their further application.
Conclusion
The PEG-UAEE method was used to extract polysaccharides from Sibiraea laevigata (L.) Maxim leaves. Response surface methodology was employed to determine the optimal conditions for the PEG-UAEE of SLMPs, and the optimized parameters were determined to be a PEG 400 concentration of 30%, E/S ratio of 21 U/g, extraction pH value of 4.0, ultrasonic power of 400 W, ultrasonic times of 2.0 h and ultrasonic temperature at 80°C. Under these parameters, the experimental extraction rate of SLMPs was 10.95%, and the content of SLMPs was 21.82%. The results showed that the molecular weight of SLMPs was 1.06 kDa after purification by HPSEC. The monosaccharide composition of SLMPs was determined to be 8.78% Rha, 46.76% Glu, 13.34% Gal, 2.89% Fru, and 11.79% Ara by HPLC-PMP, and the structural characterization analysis revealed that the SLMPs had α-glycosidic bonds and β-glycosidic bonds pyranoid polysaccharides with an apparent amorphous structure. Furthermore, in vitro antioxidant activity analysis revealed that SLMPs exhibited strong DPPH, ·OH, and ·O2− free radical scavenging activities with an IC50 of 0.42 mg/mL, 2.08 mg/mL, and 0.28 mg/mL, respectively. The results suggest that SLMPs could be explored as natural antioxidants for use in functional foods or the pharmaceutical industry.
Authors contributions
Dandan Gao and Zhongren Ma designed the whole experiment, Penghui Guo. Xuhua Yang and Hong Chen prepared SLMPs, Honghai Liu, Jutian Yang, and Penghui Guo determined the structure of SLMPs. Xuhua Yang and Dandan Gao wrote the manuscript. All authors discussed the results and contributed to the article.
Ethical review
This study does not involve any human or animal testing.
Acknowledgments
This work was supported by the National Natural Science Foundation of China (NO. 31960461); The Fundamental Research Funds for the Central Universities of Northwest Minzu University (31920220024 and BELTY201901); The Young Doctor Fund of Gansu Province (2021QB-147).
Disclosure statement
No potential conflict of interest was reported by the author(s).
Additional information
Funding
References
- Liu, H.; Zhang, T.; Jiang, P.; Zhu, W.; Yu, S.; Liu, Y.; Li, F.; Li, F. Hypolipidemic Constituents from the Aerial Portion of Sibiraea Angustata. Bioorg. Med. Chem. Lett. 2020, 30(11), 127161. DOI: 10.1016/j.bmcl.2020.127161.
- Chen, Y. Y.; Xue, Y. T. Optimization of Microwave Assisted Extraction, Chemical Characterization and Antitumor Activities of Polysaccharides from Porphyra Haitanensis. Carbohydr. Polym. 2019, 206, 179–186. DOI: 10.1016/j.carbpol.2018.10.093.
- Chen, Y.; Yao, F.; Ming, K.; Wang, D.; Hu, Y.; Liu, J. Polysaccharides from Traditional Chinese Medicines: Extraction, Purification, Modification, and Biological Activity. Molecules. 2016, 21(12), 1705. DOI: 10.3390/molecules21121705.
- Shi, F. L.; Yan, X. L.; Cheong, K. L.; Liu, Y. Extraction, Purification, and Characterization of Polysaccharides from Marine Algae Gracilaria Lemaneiformis with anti-tumor Activity. Process Biochem. 2018, 73, 197–203. DOI: 10.1016/j.procbio.2018.08.011.
- Sun, H.; Li, C.; Ni, Y.; Yao, L.; Jiang, H.; Ren, X.; Zhao, C.; Zhao, C. Ultrasonic/microwave-assisted Extraction of Polysaccharides from Camptotheca Acuminata Fruits and Its Antitumor Activity. Carbohydr. Polym. 2019, 206, 557–564. DOI: 10.1016/j.carbpol.2018.11.010.
- Wang, D.; Fan, B.; Wang, Y.; Zhang, L.; Wang, F. Optimum Extraction, Characterization, and Antioxidant Activities of Polysaccharides from Flowers of Dendrobium Devonianum. Int. J. Anal. Chem. 2018, (2018, 3013497. DOI: 10.1155/2018/3013497.
- Zou, X.; Liu, Y.; Tao, C.; Liu, Y.; Liu, M.; Wu, J.; Lv, Z. CO2 Supercritical Fluid Extraction and Characterization of Polysaccharide from Bamboo (Phyllostachys Heterocycla) Leaves. J. Food Meas. Charact. 2017, 12(1), 35–44. DOI: 10.1007/s11694-017-9614-2.
- Liu, Y.; Tang, T.; Duan, S.; Li, C.; Lin, Q.; Wu, H.; Wu, W.; Hu, B.; Wu, D.; Li, S. The Purification, Structural Characterization and Antidiabetic Activity of a Polysaccharide from Anoectochilus Roxburghii. Food Funct. 2020, 11(4), 3730–3740. DOI: 10.1039/c9fo00860h.
- Guo, L.; Tan, D. C.; Hui, F. Y.; Gu, F.; Xiao, K. M.; Hua, Y. Optimization of the cellulase-ultrasonic Synergistic Extraction Conditions of Polysaccharides from Lenzites Betulina. Chem. Biodiversity. 2019, 16(11), e1900369. DOI: 10.1002/cbdv.201900369.
- Xu, X.;. Plant Polysaccharides and Their Effects on Cell Adhesion. In Polysaccharides (Switzerland: Springer International Publishing), 2014; pp 1–16.
- Zhu, H.; Liu, C.; Hou, J.; Long, H.; Wang, B.; Guo, D.; Wu, W.; Wu, W. Gastrodia Elata Blume Polysaccharides: A Review of Their Acquisition, Analysis, Modification, and Pharmacological Activities. Molecules. 2019, 24(13), 2436. DOI: 10.3390/molecules24132436.
- Chen, X.-Q.; Zhang, Y. Ultrasonic-associated Extraction of Water Soluble Polysaccharides from Defatted Korean Pine Kernel. J. For. Res. 2007, 18(2), 133–135. DOI: 10.1007/s11676-007-0026-8.
- Chen, X.; Ji, H.; Zhang, C.; Liu, A. Optimization of Extraction Process from Taraxacum Officinale Polysaccharide and Its Purification, Structural Characterization, Antioxidant and anti-tumor Activity. J. Food Meas. Charact. 2019, 14(1), 194–206. DOI: 10.1007/s11694-019-00281-7.
- Zhai, X.; Zhu, C.; Li, Y.; Zhang, Y.; Duan, Z.; Yang, X. Optimization for pectinase-assisted Extraction of Polysaccharides from Pomegranate Peel with Chemical Composition and Antioxidant Activity. Int. J. Biol. Macromol. 2018, 109, 244–253. DOI: 10.1016/j.ijbiomac.2017.12.064.
- Enriquez-Ochoa, D.; Sanchez-Trasvina, C.; Hernandez-Sedas, B.; Mayolo-Deloisa, K.; Zavala, J.; Rito-Palomares, M.; Valdez-Garcia, J. E. Aqueous two-phase Extraction of Phenolic Compounds from Sedum Dendroideum with Antioxidant Activity and anti-proliferative Properties against Breast Cancer Cells. Sep. Purif. Technol. 2020, 251, 117341. DOI: 10.1016/j.seppur.2020.117341.
- Zhang, L.; Wang, M. PEG-based ultrasound-assisted Extraction of Polysaccharides from Superfine Ground Auricularia Auricular. J. Food Process. Preserv. 2017, 42(2). DOI: 10.1111/jfpp.13445.
- Zhou, X. Y.; Liu, R. L.; Ma, X.; Zhang, Z. Q. Polyethylene Glycol as a Novel Solvent for Extraction of Crude Polysaccharides from Pericarpium Granati. Carbohydr. Polym. 2014, 101, 886–889. DOI: 10.1016/j.carbpol.2013.10.017.
- Zhang, L.; Guo, S.; Wang, M.; He, L. PEG-based ultrasound-assisted Enzymatic Extraction of Polysaccharides from Ginkgo Biloba Leaves. Int. J. Biol. Macromol. 2015, 80, 644–650. DOI: 10.1016/j.ijbiomac.2015.07.023.
- Wu, W.; Huang, T.; Xiang, F. Polyethylene glycol-based ultrasonic-assisted Enzymatic Extraction, Characterization, and Antioxidant Activity in Vitro and in Vivo of Polysaccharides from Lonicerae Japonica Leaves. Food Sci. Nutr. 2019, 7(11), 3452–3462. DOI: 10.1002/fsn3.1186.
- Wang, W.; Li, X.; Chen, K.; Yang, H.; Jialengbieke, B.; Hu, X. Extraction Optimization, Characterization and the Antioxidant Activities in Vitro and in Vivo of Polysaccharide from Pleurotus Ferulae. Int. J. Biol. Macromol. 2020, 160, 380–389. DOI: 10.1016/j.ijbiomac.2020.05.158.
- Deng, W.; Wu, J.; Da, Y.; Ma, Z. Effect of Temperature Treatment on Fruit Quality and Immunoregulation of Satsuma (Citrus Unshiu Marc.) during Storage. Food Sci. Nutr. 2020, 8(10), 5443–5451. DOI: 10.1002/fsn3.1771.
- Duan, S.; Huang, Q.; Shen, X.; Hu, J.; Yi, X.; Li, Z.; Ding, B. Deproteinization of Four Macroporous Resins for Rapeseed Meal Polysaccharides. Food Sci. Nutr. 2020, 8(1), 322–331. DOI: 10.1002/fsn3.1309.
- Yuan, Q.; Xie, Y.; Wang, W.; Yan, Y.; Ye, H.; Jabbar, S.; Zeng, X. Extraction Optimization, Characterization and Antioxidant Activity in Vitro of Polysaccharides from Mulberry (Morus Alba L.) Leaves. Carbohydrate Polymers. 2015, 128, 52–62. DOI: 10.1016/j.carbpol.2015.04.028.
- Chen, S.; Su, T. T.; Wang, Z. Y. Structural Characterization, Antioxidant Activity, and Immunological Activity in Vitro of Polysaccharides from Fruiting Bodies of Suillus Granulatus. J. Food Biochem. 2018, 42(3), e12515. DOI: 10.1111/jfbc.12515.
- Hammami, N.; Gara, A. B.; Bargougui, K.; Ayedi, H.; Abdalleh, F. B.; Belghith, K. Improved in Vitro Antioxidant and Antimicrobial Capacities of Polysaccharides Isolated from Salicornia Arabica. Int. J. Biol. Macromol. 2018, 120(Pt B), 2123–2130. DOI: 10.1016/j.ijbiomac.2018.09.052.
- Wang, Y. F.; Hou, G. H.; Li, J. L.; Surhio, M. M.; Ye, M. Structure Characterization, Modification through Carboxymethylation and Sulfation, and in Vitro Antioxidant and Hypoglycemic Activities of a Polysaccharide from Lachnum Sp. Process Biochem. 2018, 72, 177–187. DOI: 10.1016/j.procbio.2018.06.002.
- Jalili Safaryan, M.; Ganjloo, A.; Bimakr, M.; Zarringhalami, S. Optimization of ultrasound-assisted Extraction, Preliminary Characterization and in Vitro Antioxidant Activity of Polysaccharides from Green Pea Pods. Foods. 2016, 5(4), 78. DOI: 10.3390/foods5040078.
- Wang, J.; Lu, H. D.; Muhammad, U.; Han, J. Z.; Wei, Z. H.; Lu, Z. X.; Lu, F. X.; Lu, F. X. Ultrasound-assisted Extraction of Polysaccharides from Artemisia Selengensis Turcz and Its Antioxidant and Anticancer Activities. J. Food Sci. Technol. 2016, 53(2), 1025–1034. DOI: 10.1007/s13197-015-2156-x.
- Wang, L.; Li, T.; Liu, F.; Liu, D.; Xu, Y.; Yang, Y.; Wei, H.; Wei, H. Ultrasonic-assisted Enzymatic Extraction and Characterization of Polysaccharides from Dandelion (Taraxacum Officinale) Leaves. Int. J. Biol. Macromol. 2019, 126, 846–856. DOI: 10.1016/j.ijbiomac.2018.12.232.
- Long, X.; Yan, Q.; Cai, L.; Li, G.; Luo, X. Box-Behnken design-based Optimization for Deproteinization of Crude Polysaccharides in Lycium Barbarum Berry Residue Using the Sevag Method. Heliyon. 2020, 6(5), e03888. DOI: 10.1016/j.heliyon.2020.e03888.
- Zhang, X.; Ban, Q.; Wang, X.; Wang, Z. Green and Efficient PEG-based ultrasonic-assisted Extraction of Polysaccharides from Tree Peony Pods and the Evaluation of Their Antioxidant Activity in Vitro. Biomed Res. Int. 2018, (2018, 2121385. DOI: 10.1155/2018/2121385.
- Chen, W.; Jia, Z.; Zhu, J.; Zou, Y.; Huang, G.; Hong, Y. Optimization of ultrasonic-assisted Enzymatic Extraction of Polysaccharides from thick-shell Mussel (Mytilus Coruscus) and Their Antioxidant Activities. Int. J. Biol. Macromol. 2019, 140, 1116–1125. DOI: 10.1016/j.ijbiomac.2019.08.136.
- Sorourian, R.; Khajehrahimi, A. E.; Tadayoni, M.; Azizi, M. H.; Hojjati, M. Ultrasound-assisted Extraction of Polysaccharides from Typha Domingensis: Structural Characterization and Functional Properties. Int. J. Biol. Macromol. 2020, 160, 758–768. DOI: 10.1016/j.ijbiomac.2020.05.226.
- Afshari, K.; Samavati, V.; Shahidi, S. A. Ultrasonic-assisted Extraction and in-vitro Antioxidant Activity of Polysaccharide from Hibiscus Leaf. Int. J. Biol. Macromol. 2015, 74, 558–567. DOI: 10.1016/j.ijbiomac.2014.07.023.
- Chen, G.; Kan, J. Ultrasound-assisted Extraction, Characterization, and Antioxidant Activity in Vitro and in Vivo of Polysaccharides from Chestnut Rose (Rosa Roxburghii Tratt) Fruit. J. Food Sci. Technol. 2018, 55(3), 1083–1092. DOI: 10.1007/s13197-017-3023-8.
- Chen, G.; Zhang, S.; Ran, C.; Wang, L.; Kan, J. Extraction, Characterization and Antioxidant Activity of water-soluble Polysaccharides from Tuber Huidongense. Int. J. Biol. Macromol. 2016, 91, 431–442. DOI: 10.1016/j.ijbiomac.2016.05.108.
- Wang, Y.; Wang, C.; Guo, M. Effects of Ultrasound Treatment on Extraction and Rheological Properties of Polysaccharides from Auricularia Cornea Var. Li. Molecules. 2019, 24, 5. DOI: 10.3390/molecules24050939
- Xue, H. Y.; Li, J. R.; Liu, Y. G.; Gao, Q.; Wang, X. W.; Zhang, J. W.; Xue, Y. L.; Xue, Y.-L. Optimization of the ultrafiltration-assisted Extraction of Chinese Yam Polysaccharide Using Response Surface Methodology and Its Biological Activity. Int. J. Biol. Macromol. 2019, 121, 1186–1193. DOI: 10.1016/j.ijbiomac.2018.10.126.
- Zhang, W.; Hu, Y.; Zhao, J.; Zhang, Y.; Guo, D.; Gao, C.; Li, P.; Li, P. Immunoregulation and Antioxidant Activities of a Novel Acidic Polysaccharide from Radix Paeoniae Alba. Glycoconjugate J. 2020, 37(3), 361–371. DOI: 10.1007/s10719-020-09916-0.
- Li, B.; Zhang, N.; Wang, D. X.; Jiao, L.; Tan, Y.; Wang, J.; Jiang, D. C.; Wu, W.; Jiang, D. C. Structural Analysis and Antioxidant Activities of Neutral Polysaccharide Isolated from Epimedium Koreanum Nakai. Carbohydr. Polym. 2018, 196, 246–253. DOI: 10.1016/j.carbpol.2018.05.037.
- Tian, Y.; Zeng, H.; Xu, Z.; Zheng, B.; Lin, Y.; Gan, C.; Lo, Y. M. Ultrasonic-assisted Extraction and Antioxidant Activity of Polysaccharides Recovered from White Button Mushroom (Agaricus Bisporus). Carbohydr. Polym. 2012, 88(2), 522–529. DOI: 10.1016/j.carbpol.2011.12.042.
- Pu, J. B.; Xia, B. H.; Hu, Y. J.; Zhang, H. J.; Chen, J.; Zhou, J.; Xu, P.; Xu, P. Multi-optimization of ultrasonic-assisted Enzymatic Extraction of Atractylodes Macrocephala Polysaccharides and Antioxidants Using Response Surface Methodology and Desirability Function Approach. Molecules. 2015, 20(12), 22220–22235. DOI: 10.3390/molecules201219837.
- Ma, D.-L.; Du, H.-R.; Wen, Z.-S.; Wang, L.; Li, J.; Zheng, Y.-G. Optimization of Ultrasonic-Assisted Extraction of Polysaccharides from Trichosanthin and Antioxidant Activities in vitro. In 2018 9th International Conference on Information Technology in Medicine and Education (ITME), computer society, 2018; Paper 894–899.
- Wu, Y. T.; Huo, Y. F.; Xu, L.; Xu, Y. Y.; Wang, X. L.; Zhou, T. Purification, Characterization and Antioxidant Activity of Polysaccharides from Porphyra Haitanensis. Int. J. Biol. Macromol. 2020, 165(Pt B)), 2116–2125. DOI: 10.1016/j.ijbiomac.2020.10.053.
- Malafronte, L.; Yilmaz-Turan, S.; Krona, A.; Martinez-Sanz, M.; Vilaplana, F.; Lopez-Sanchez, P. Macroalgae Suspensions Prepared by Physical Treatments: Effect of Polysaccharide Composition and Microstructure on the Rheological Properties. Food Hydrocolloids. 2021, 120, 106989. DOI: 10.1016/j.foodhyd.2021.106989.
- Cheng, H.; Feng, S.; Shen, S.; Zhang, L.; Yang, R.; Zhou, Y.; Ding, C. Extraction, Antioxidant and Antimicrobial Activities of Epimedium Acuminatum Franch. Polysaccharide. Carbohydr. Polym. 2013, 96(1), 101–108. DOI: 10.1016/j.carbpol.2013.03.072.