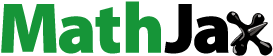
ABSTRACT
The objectives of this study were to determine the prevalence of Listeria monocytogenes in ready-to-eat (RTE) foods, and the growth boundaries of two strains of L. monocytogenes isolated from RTE foods and one ATCC7644 strain under different temperature (4, 20, and 37°C), salt (0, 1, 2, 3, and 4% w/v), and nisin (0, 50, 100, 200, and 400 µg/mL) levels with different inoculum sizes (3, 4, 5, and 6 log10 CFU/mL). One hundred thirty-three retail RTE foods were examined and a total of 39 isolates were identified. The positive Listeria spp. and L. monocytogenes samples were 29.3% and 12.8%, respectively. Dairy and fruit-based RTE foods were L. monocytogenes negative whereas seafood-based (26.7%), meat-based (19.4%), poultry-based (16%), and vegetable-based (10%) RTE foods contained L. monocytogenes. It was shown that nisin was more effective than NaCl to avoid growth. Moreover, protective effect of NaCl on nisin activity was observed. As inoculum size increased probability of growth also increased. Suppressing the growth by using temperature, NaCl and nisin was more difficult in RTE-isolates strains (strains 120 and 137) than that of ATCC7644 strain. A mathematical model based on logistic regression could be used to determine growth limits of L. monocytogenes strains with a concordance of > 91%.
Introduction
Listeria monocytogenes is a Gram positive, facultative anaerobe, psychrotrophic, motile at least at 30°C, rod-shaped (0.5 μm wide and 1–2 μm long), halotolerant, non-encapsulated, non-spore-forming food-borne pathogen responsible for human listeriosis.[Citation1–4] Listeriosis is a serious illness that occurs after the consumption of contaminated foods with L. monocytogenes, mainly in pregnant women, neonates, immunocompromised individuals, the elderly, and humans with underlying illness such as kidney disease, cancer, diabetes, and HIV AIDS patients.[Citation5,Citation6] Listeriosis can be classified into two different forms: i) severe invasive listeriosis and ii) noninvasive febrile gastroenteritis. Invasive listeriosis is commonly seen in immunocompromised individuals. More severe symptoms like sepsis, meningitis, endocarditis, encephalitis, meningoencephalitis, septicemia, and brain infection can occur in this type of listeriosis. Invasive listeriosis is responsible for 90% of hospitalization and about 20–30% case fatality rate .[Citation7]
L. monocytogenes may persist for long periods in food processing areas, and different environments such as soil, water, silage, sewage, and the intestinal tract of many mammals and humans.[Citation1] Additionally, different food types such as vegetables, raw milk, and dairy products especially soft cheeses made from raw milk, poultry, raw meat, and processed meat products, raw fish, seafood products, ready-to-eat (RTE) foods and juices can be contaminated with L. monocytogenes .[Citation8] In recent times, a large number of L. monocytogenes outbreaks have been linked to RTE foods since RTE foods may be a suitable substrate for the pathogen to grow. Moreover, L. monocytogenes can grow at refrigeration temperatures, low pH, and high salinity until consumption.[Citation9] In general, RTE foods may not be subjected to further cooking or processing to destroy L. monocytogenes before consumption.[Citation10] The regulatory agencies have identified that only three conditions have been described where RTE foods do not favor the growth of L. monocytogenes. These are i) pH≤ 4.4, ii) aw≤ 0.92, and iii) pH≤ 5.0 and aw≤ 0.94.[Citation11] Although there are several legislations in many countries to limit the prevalence of L. monocytogenes in RTE foods, there is no common consensus on the acceptable levels of L. monocytogenes in foods.[Citation12,Citation13] While EU regulation prohibits L. monocytogenes in 25 grams RTE foods for infants and RTE foods for special medical purposes, they follow a policy that states the number of L. monocytogenes should be less than 100 CFU/g of food placed on the market during its shelf-life.[Citation14] The U.S. Food and Drug Administration (FDA) does not allow the presence of L. monocytogenes in 25 g of RTE foods.[Citation13] Similarly, Turkey enforces no detectable level of viable L. monocytogenes cells in 25 g of RTE foods.[Citation15]
Nisin is the most common bacteriocin used in processed foods such as meat products, dairy products, and vegetables.[Citation16] Effective use of nisin against bacteria depends on different factors especially if it is used in combination with other hurdles such as pH, aw, and preservatives.[Citation17] Salt (NaCl), on the other hand, is used not only to control the bacterial growth but also to improve the flavor, especially in processed meat products.[Citation18] Growth boundary determination of foodborne pathogens based on probabilistic modeling offers detailed information on the growth limits under various conditions such as temperature, pH, aw, etc.[Citation19] Although many modeling studies on L. monocytogenes exist, new ones are required to better understand under which conditions L. monocytogenes could potentially be able to grow. Therefore, the objectives of this study were to determine (i) the prevalence of L. monocytogenes in RTE foods, (ii) growth boundaries of selected strains under different temperature, salt, and nisin levels with different inoculum sizes.
Materials and methods
Sample collection
In this study, a total of 133 retail RTE foods were randomly purchased from various supermarkets, patisseries, restaurants, open bazaars, and street hawkers in Ankara, Turkey between November 2020 and September 2021. RTE foods were classified into six categories: 20 were dairy-based, 31 meat-based, 25 poultry-based, 30 vegetable-based, 12 fruit-based and 15 fish-based. All samples except for vegetable- and fruit-based ones had undergone heat treatment. Each RTE food samples was stored at 4°C during the sale. RTE foods sold by the street hawkers and restaurants were unpackaged, while samples collected from other places were packaged and nonfrozen. All RTE foods were checked for expiry dates and all samples were placed in a cold box at 4°C and processed immediately on the sampling day.
Isolation and biochemical identification of Listeria monocytogenes
Detection of the L. monocytogenes was carried out in accordance with the International Organization for Standardization standard protocol[Citation20] with two-stage enrichment. L. monocytogenes ATCC7644, L. innocua ATCC12612, Escherichia coli ATCC25922, Staphylococcus aureus ATCC6538 and Rhodococcus equi ATCC6939 were used as references strains. Briefly, 25 g of each food sample were added to 225 mL ½ Fraser broth (Merck) and homogenized in a stomacher (Seward 400, USA) for 3 min. After incubation at 30 ± 1°C for 24 ± 2 h, 0.1 mL of the primary enrichment culture was transferred into 10 mL full Fraser broth and incubated at 37°C for 48 ± 2 h. To isolate Listeria spp., a loopful each of the ½ and full Fraser broth were streaked on both ALOA agar (Merck) and PALCAM agar (Merck), and the plates were incubated at 37°C for 24–48 h. Then, five suspected colonies each from ALOA and PALCAM agar plates were picked and subjected to morphological and biochemical tests including by Gram staining, catalase reactions, oxidase tests, carbohydrate utilization, CAMP tests, and motility at 20–25°C. Bacterial isolates were stored in Brain Heart Infusion (BHI) broth (Merck) with 30% sterile glycerol (Merck) at – 80°C.
Genomic DNA extraction and PCR identification of Listeria monocytogenes
Following the biochemical tests, L. monocytogenes isolates were confirmed by polymerase-chain reaction (PCR) analysis. Bacterial cultures were grown in Tryptic Soy Broth supplemented with 0.6% of yeast extract (TSB-YE) (Merck) for both the 16S rRNA gene and listeriolysin (hlyA) gene determination. Genomic DNA extraction and PCR amplifications were used as described by Sanlibaba et al.[Citation3] The primer pairs including forward U1 (5′–CAGCMGCCGCGGTAATWC–3′) and reverse LI1 (5′–CTCCATAAAGGTGACCCT–3′) were used to amplify a 938 bp region in the 16S rRNA gene of the Listeria genus.[Citation21] Additionally, the primer pairs 5′–CATTAGTGGAAAGATGGAATG–3′ (forward) and 5′–GTATCCTCCAGAGTGATCGA–3′ (reverse) were used to amplify a 730 bp region of the hlyA gene of L. monocytogenes strains.[Citation22] Escherichia coli ATCC25922 and L. monocytogenes ATCC7644 were used as negative and positive controls, respectively. Amplification conditions were slightly modified and were as follows: initial denaturation at 94°C for 3 min, followed by 35 cycles of denaturation at 95°C for 45 s, annealing at 55°C for 30 s, and extension at 72°C for 2 min, then final extension step at 72°C for 5 min, as described previously.[Citation22] Amplified DNA was separated using 1% agarose gel electrophoresis (w/v) in 1X TBE buffer stained with ethidium bromide solution (2.5 µL/100 mL), and then, the gel was visualized under a UV transilluminator (SYNGENE, Biosystems UK). A 1000 bp DNA ladder (Thermo Scientific) was used as a reference marker.
Preparation of nisin solution and determination of minimum inhibitory concentration
Nisin stock solutions were prepared with Nisaplin (2.5% nisin A, Product Number: N5764, Sigma) by adding 0.020 g or 0.200 g to 10 mL of 0.02 N HCl. These solutions were kept at 4°C for a maximum of 24 h.[Citation23] Minimum inhibitory concentration (MIC) assay was used to determine the antimicrobial activity of nisin against L. monocytogenes strains. MIC assays were carried out in 96 well microtiter plates in triplicate as described by Lynch et al. .[Citation24]
Experimental design for determination of growth rate of L. monocytogenes
To determine the growth rates of 4 strains of L. monocytogenes isolated from RTE meals and an ATCC7644 strain, a microplate reader (BioTek Elisa Reader, Biotek Inc., USA) with 96 wells were used. The optical density (OD) was measured at 600 nm and growth rates were determined by using the formula below[Citation25]:
where tOD is the time (h) when OD value is equal to 0.15, that is, time to detection (TTD), λ is the lag time (h), log10NOD is the number of L. monocytogenes (log10CFU/mL) at OD = 0.15, log10N0 is the initial number of L. monocytogenes (log10CFU/mL) and µ is the specific growth rate (h−1). To verify the growth rate obtained from EquationEq.(1(1)
(1) ), colony counts were also performed and three growth rate models were fitted to the data – see below. For the microbiological plate counts, at appropriate time intervals 1 mL of sample was added to 9 mL of sterile peptone water (0.5%) and mixed for about 15 s. Serial 10-fold dilutions were prepared in peptone water and 0.1 mL of aliquots from each dilution were spread plated in duplicate. All the experiments were repeated twice with duplicate measurements (n = 4).
ÖK-BUZ GRoFiT, an Excel freeware file in which Baranyi,[Citation26] modified Gompertz,[Citation27] and three phase linear model[Citation28] is inserted, was downloaded from https://mmf.gidatarim.edu.tr/en/ok-buz-grofit and used for modeling the microbial count.[Citation29] There are two versions of this tool. Second version of the tool is available both in Turkish (https://mmf.gidatarim.edu.tr/ok-buz-grofit) and in English. The Baranyi model in ÖK-BUZ GRoFiT is a 4-parameter alternative to the one in DMFit which has six parameters. Nevertheless, almost identical fits for these Excel tools could be obtained with the growth data having lag, exponential, and stationary phases. Two strains with the highest growth rates and the ATCC7644 strain were used for growth/no growth studies.
Experimental design for determination of growth boundaries of L. monocytogenes
A full factorial design was used to test the growth ability of L. monocytogenes in TSB with different combinations of temperature (4, 20, and 37°C), sodium chloride (0, 1, 2, 3, and 4% w/v), nisin (0, 50, 100, 200, and 400 µg/mL), and initial concentration (3, 4, 5, and 6 log10CFU/mL). Three replications were done for each combination which resulted in 900 data points (3 temperature levels × 5 salt levels × 5 nisin levels × 4 initial concentration levels × 3 replications). Selected temperature levels represented the refrigeration, ambient, and abuse (optimum growth) temperatures. Salt and nisin concentrations were the ones that are generally used or allowed levels (except 400 µg/mL for nisin) in foods. It should be noted that the inoculum sizes of 5 and 6 log10CFU/mL were higher than the expected contamination of L. monocytogenes in foods and higher growth probability with these inoculum sizes should be expected.[Citation30] Inoculum sizes of 3 and 4 log10CFU/mL were also used to determine growth boundaries under more realistic conditions.
Preparation of broth and growth evaluation
Uninoculated samples were served as negative controls and broths (TSB) with no salt or nisin inoculated with 103, 104, 105, and 106 CFU/mL bacteria were used as the positive control. Microbial growth was monitored weekly by measuring OD at 600 nm up to 8 weeks. Same microplate reader with 96 wells were used for growth evaluation. At higher initial inocula (105 and 106 CFU/mL), 0.1 increase in the OD values were considered as growth.[Citation31] Growth was also verified by plate counts for some wells. At lower inocula (103 and 104 CFU/mL), growth was monitored both by checking the OD values and by plate counts.
Model development
Two models were proposed:
or
where, p is the probability of growth in the range of 0–1 (growth = 1, no growth = 0), logit(p) = ln[p/(1–p)] a0–a4, b0–b10 are the coefficients of the model to be estimated, T is the temperature (°C), NaCl is the salt concentration (% w/v), nisin is the nisin concentration (µg/mL), and IC is the initial concentration (log10CFU/mL) of L. monocytogenes.
SPSS (Version 22, Chicago, IL, USA) was used for logistic regression and goodness-of-fit of the models were compared by using (i) – 2 · ln L with L the likelihood in its optimum, (ii) Hosmer–Lemeshow (H-L) statistic (iii) maximum rescaled R2 statistic and (iv) percent concordant.[Citation32] The fitted growth/no growth boundaries for p= .1, 0.5, and 0.9 were calculated by using Microsoft® Excel.
The first model was the simplest possible model with the main effects (temperature, salt, nisin, and initial concentration). The second one was more complex one with bilateral interaction terms. If the interaction terms were insignificant (P > .05), they were removed from the model and regression was repeated without those terms, that is, remaining coefficients in the model were all significant (P ≤ .05).
Results
Prevalence of Listeria monocytogenes in RTE food samples
The identification of the 39 isolates was made according to the 16S rRNA sequence results (data not shown). The positive Listeria spp. and L. monocytogenes samples were 39/133 and 17/133, respectively. All L. monocytogenes strains showed a positive result for the presence of the virulence-associated hlyA gene. Results of the prevalence of Listeria spp. and L. monocytogenes in the positive samples are shown in . In particular, L. monocytogenes isolated from RTE foods was highlighted in 4/15 seafood-based, 6/31 meat-based, 4/25 poultry-based, 3/30 vegetable-based. However, dairy and fruit-based RTE foods were L. monocytogenes negative.
Table 1. Presence of Listeria spp. and L. monocytogenes in RTE food products.
MIC results
Seventeen L. monocytogenes strains isolated in this study were tested for susceptibility to nisin using MIC assays. Nisin can inhibit L. monocytogenes; MIC ranging from 128 to 4096 μg/mL were obtained. Four L. monocytogenes strains were selected among the strains with the highest, middle and lowest MIC values (). Among these selected strains, 120 and 151 was isolated from meat-based RTE foods, 137 from vegetable-based RTE foods, and also 241 from seafood-based RTE foods. While L. monocytogenes 120 was the most resistant strain to nisin (4096 μg/mL), strains 137 and 241 exhibited the lowest MIC values of 512 μg/mL. Strain 151 also displayed low susceptibility to nisin (2048 μg/mL). However, L. monocytogenes ATCC7644 was sensitive to nisin with an observed MIC value of 128 μg/mL.
Table 2. Minimum inhibitory concentration (MIC) values of nisin against L. monocytogenes strains*.
Growth rate determination
Value of NOD was determined by enumerating the number of L. monocytogenes in each well when the OD600 value was about 0.15. Although NOD values changed with respect to strains, they were between 8.0 and 8.5 log10. shows the fit of EquationEq.(1)(1)
(1) that is, how the growth rates (µ) were calculated for three strains (120, 137, and ATCC7644) and lists the growth rates of each strain. The highest and lowest growth rates were obtained as 2.21 and 2.04 h−1 for strains 120 and 151, respectively.
Table 3. Growth rates of L. monocytogenes strains in TSB calculated by using EquationEq.(1)(1)
(1) from OD measurements.
Figure 1. Time-to-turbidity as a function of logarithm of the inoculum size (gray circles) and the fit of EquationEq.(1(1)
(1) ) (black lines) to determine the growth rates of L. monocytogenes strains 120 (a), 137 (b) and ATCC7644 (c) in TSB.
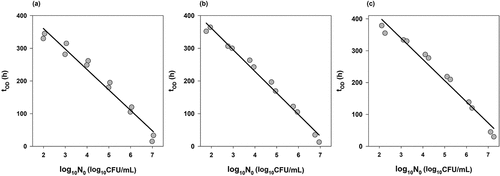
Plate count method was also applied for three strains (120, 137, and ATCC7644) to compare the growth rates of time-to-detection (TTD) data with colony counts. The fit of three models (modified Gompertz, Baranyi, and three-phase linear) used to describe the growth data of L. monocytogenes 137 is shown in . All models produced reasonable fits with R2adj>0.97 for the other strains and closest growth rates to TTD experiments were the ones obtained from the fit of three-phase linear model (). According to the model fit, highest growth rate was 2.25 h−1 for the strain 137, followed by 2.10 h−1 for the strain 120 and 2.04 h−1 for the ATCC7644 strain. The growth rates obtained for the Baranyi and Gompertz models were given in . The information obtained from MIC study combined with the growth rate study, and the strains with higher MIC and growth rates (120, 137, and ATCC7644) were further used for growth/no growth study.
Table 4. Growth rates of L. monocytogenes strains in TSB calculated by using three phase linear model from colony count data.
Table 5. Growth rates of L. monocytogenes strains in TSB calculated by using Baranyi and Gompertz models from colony count data.
Effects of temperature, salt, nisin, and initial inoculum on the growth of L. monocytogenes
Growth responses of three strains with the inoculum levels of 103 and 106 CFU/mL are shown in , respectively. In general, same response (growth or no growth) was observed for all three replicates; however, there were some exceptions. For example, at 4°C, without nisin and salt two out of three responses for the strain 120 were 1 (growth) and the one was 0 (no growth) meaning that growth probability was 67% (). For the strain 137, one out of three responses was 1 (growth) and two were 0 (no growth) indicating the probability of growth was 33% at 4°C without nisin and with 3% salt (). Differences between the replicates could also be seen in .
Figure 3. Growth/no growth interfaces of L. monocytogenes (103 CFU/mL) strains (a and b: strain 120, c and d: strain 137, e and f: ATCC7644 strain) in TSB after 8 weeks. White circles: no growth in all three replications, black circles: growth in all three replications, gray circles: two growth-one no growth observations and crossed circle: one growth-two no growth observations. Short dashed line, solid line and long dashed line represent the growth probabilities of 0.1, 0.5 and 0.9, respectively according to .EquationEq.(3)(3)
(3) with the coefficient values given in
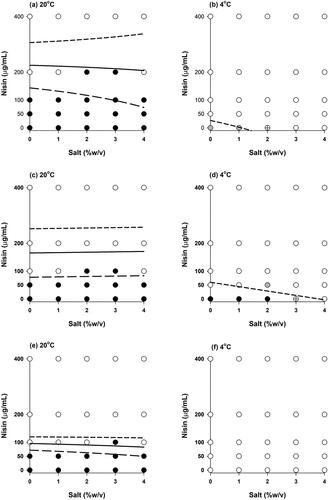
Figure 4. Growth/no growth interfaces of Listeria monocytogenes (106 CFU/mL) strains (a and b: strain 120, c and d: strain 137, e and f: ATCC7644 strain) in TSB after 8 weeks. White circles: no growth in all three replications, black circles: growth in all three replications, gray circles: two growth-one no growth observations and crossed circle: one growth-two no growth observations. Short dashed line, solid line and long dashed line represent the growth probabilities of 0.1, 0.5 and 0.9, respectively according to .EquationEq.(3)(3)
(3) with the coefficient values given in
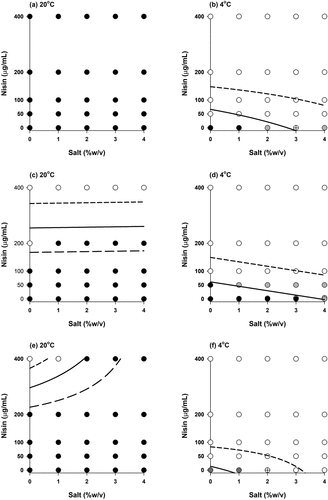
It was observed that the temperature had a significant impact on the growth of L. monocytogenes: at 37°C, the use of high salt and nisin concentration was not enough to avoid the growth even at the lowest inoculum (103 CFU/mL) – results not shown. On the other hand, high nisin concentration (≥200 µg/mL) was very effective at 20°C in the absence or presence of salt when the initial inoculum was less than 106 CFU/mL for all strains in general. Refrigeration temperature (4°C) changed the results drastically: even at the lowest nisin (50 µg/mL) concentration, growth was not observed for the strains 120 and 137 () whereas no growth was observed for the ATCC7644 strain without nisin and salt during storage of 8 weeks ().
Growth boundary determination
Models proposed [EquationEq.(2)(2)
(2) and (Equation3
(3)
(3) )] were applied to the data, and coefficient estimates, their standard errors and P values for EquationEq. (2)
(2)
(2) and (Equation3
(3)
(3) ) are all given in and , respectively. The simplest model with only the main effects [EquationEq.(2
(2)
(2) )] had an insignificant coefficient (P > .05) of NaCl terms [a2 in EquationEq.(2
(2)
(2) )] for the 137 and ATCC7644 strains (). On the other hand, for the complex model [EquationEq.(3
(3)
(3) )] all main effects retained in the equation when the insignificant coefficients were removed from the model. All goodness-of-fit indices were better for the second model ( and ) and therefore, EquationEq.(3)
(3)
(3) was further used to plot the predicted growth/no growth boundaries of L. monocytogenes. Boundaries for likely to grow probability (p= .9), equal probability of growth and no growth (p= .5), and unlikely to grow probability (p= .1) are also displayed in .
Table 6. Estimated coefficients of the simple model [EquationEq.(2(2)
(2) )].
Table 7. Estimated coefficients of the complex model [EquationEq.(3(3)
(3) )].
Table 8. Goodness-of-fit indices of the simple model [EquationEq.(2(2)
(2) )].
Table 9. Goodness-of-fit indices of the complex model [EquationEq.(3(3)
(3) )].
Discussion
In this study, the prevalence of L. monocytogenes in RTE foods was as 12.8%. Previous studies reported that the percentage of positive samples of L. monocytogenes in RTE foods was 36.73% in Nigeria[Citation33] and 33.3% in Italy.[Citation34] On the other hand, our results showed higher prevalence of L. monocytogenes strains in RTE foods compared to some other studies conducted in different regions, such as 2.6% in Estonia,[Citation35] 6.87% in China,[Citation36] 1.7% in Japan,[Citation37] 0.4% in USA,[Citation38] 0.1% in Poland,[Citation39] and 3.1% in Chile.[Citation40] Previous studies in Turkey[Citation3,Citation41,Citation42] documented that the prevalence of L. monocytogenes in RTE foods was in the range of 4.0–19.7%.
Higher prevalence in seafood products (26.7%) than other RTE foods were observed and this result was even higher than the previous reports from Iran,[Citation5] Greece,[Citation43] Estonia,[Citation44] and Nigeria.[Citation45] The prevalence of L. monocytogenes RTE seafood products in Turkey was found as 12% by Sanlibaba et al.[Citation3] The higher prevalence obtained in this study may indicate poor hygiene level by the food handlers. Incidence of L. monocytogenes was slightly higher in meat-based samples (19.4%) than in poultry-based samples (16%). Available data from literature indicated that the prevalence of L. monocytogenes was between 3.6% and 27.9%.[Citation22,Citation39,Citation46] Additionally, Awaisheh[Citation22] and Sanlibaba et al.[Citation3] found the prevalence of L. monocytogenes on RTE poultry foods at the level of 15% and 10.5%, respectively. The reason for the high prevalence of L. monocytogenes in the RTE meat and poultry-based foods may be inadequate heat treatment to eliminate of L. monocytogenes or inadequate physical separation between the raw and cooked food processing areas .[Citation39]
L. monocytogenes have been widely associated with vegetable products.[Citation47] In this study, although L. monocytogenes was not detected in fruit-based RTE foods, the prevalence of this pathogen was 10% in vegetable-based RTE foods. Fruit- or vegetable-based RTE foods which may be considered as minimally or moderately processed foods, can be great health risk for consumers since they are consumed fresh without applying heat treatment.[Citation48] The main reason of contamination of vegetable-based RTE foods by L. monocytogenes may be processing, packing, or poor sanitary conditions of food handlers.[Citation8,Citation9] It is worth noting that L. monocytogenes was not isolated from dairy-based RTE in this study. This pathogen can be controlled in dairy-based RTE by the appropriate implementation of good manufacturing practices and hazard analysis and critical control point systems. Many studies have published that the prevalence in dairy-based RTE foods ranged from 5.90 to 36%.[Citation21,Citation49,Citation50] Moreover, consumption of soft cheeses named Queso Fresco caused Listeria outbreak in 2021 according to the report published by Centers for Disease Control (CDC), and 12 persons became ill, of whom 1 died.[Citation51] Post-pasteurization contamination by L. monocytogenes is the main problem in dairy products .[Citation2]
Our results revealed that the specific growth rates of L. monocytogenes strains isolated from RTE foods obtained by the absorbance method and the plate count method were similar ( and ) which had been also observed in different studies.[Citation52–54] Therefore, it may be possible to determine the specific growth rate by performing two instead of multiple plate count experiments with 2-fold dilution method[Citation54] since the initial value of each strain was also determined by enumeration. Strain 120 was the most resistant one to nisin according to MIC results (), it had also the highest growth rate with respect to 2-fold dilution method () and second highest growth rate according to colony count data (). Hence, it was included in the growth/no growth study. On the other hand, although strain 137 had middle susceptibility to nisin () it had the second highest growth rate with respect to 2-fold dilution method () and the highest growth rate with respect to colony count data (). Therefore, it was also included in growth boundary study together with ATCC7644 strain.
Growth response data (1: growth, 0: no growth) of three strains of L. monocytogenes were monitored at different temperature, salt, nisin, and inoculum concentrations for 8 weeks (56 days), and no increase in OD values of negative controls during this period was observed indicating that no contaimination was occurred during the preparation of the liquid media. On the other hand, OD values of the positive control (without salt and nisin) increased at each temperature as expected. Growth/no growth results also indicated that the nisin was more effective than NaCl to avoid growth. Moreover, protective effect of salt on nisin activity was also observed. For example, at 20°C with 2% and 3% of salt concentrations, and with 200 µg/mL of nisin growth were observed for the strain 120 but, the responses were no growth at the same temperature and nisin concentration with 0%, 1%, and 4% salt concentrations (). Protective effect of salt on nisin activity against L. monocytogenes was also observed by Boziaris et al.[Citation55] However, contradictory results on this subject were also found in the literature. For example, it was observed that the presence of salt (NaCl) improves the action of nisin against Listeria.[Citation56,Citation57] On the other hand, the use of 2–4% NaCl has a protective effect of nisin against L. monocytogenes.[Citation58,Citation59] Form a hurdle concept point of view, this phenomenon should be further investigated at a microscopic level.
Initial inoculum was also an important parameter. Comparison of revealed this fact. Probability of growth increased as the initial inoculum increased and this was in agreement with the work of Koutsoumanis and Sofos[Citation60] who studied the combined effect of temperature, pH, water activity, and inoculum size of L. monocytogenes. Furthermore, suppressing the growth by using temperature, NaCl, and nisin was more difficult in RTE-isolates strains (120 and 137) than that of ATCC7644 strain ().
While the simple model [EquationEq.(2(2)
(2) )] had only five coefficients (with an insignificant main effect), the complex model [EquationEq.(2
(2)
(2) )] had six coefficients for the strains 120 and 137, and 8 coefficients for ATCC7644 strain after the removal of the insignificant terms and repetition of the regression ( and ). Therefore, the second model was preferable over the first one and support for this statement also comes from and which compared the goodness-of-fit of the models. Furthermore, HL statistics were poor for the first model with P < .05 for the strains 137 and ATCC7644. The model is rejected if P is below 0.05.[Citation31] The concordance and maximum rescaled R2 values were all >91% and >0.83, respectively, indicating good predictive power of EquationEq.(3)
(3)
(3) . Growth/no growth boundaries were in general consistent with the experimental data () and it is possible to estimate the growth probability of Listeria using EquationEq.(3)
(3)
(3) for any combination of temperature, salt, and nisin levels within the experimental range.
The strains 120 and 137 were isolated from RTE foods, however, this study was conducted in laboratory medium (broth). Use of broth can present a quick estimation of growth boundaries nevertheless, deviations from the results could be obtained in the laboratory medium compared to a real food system.[Citation30]
Conclusion
Prevalence of L. monocytogenes in RTE foods sold in Turkish market was determined in this study. It was observed that some RTE foods (dairy and fruit-based) did not contain any Listeria. On the other hand, seafood-based, meat-based, poultry-based, and vegetable-based RTE foods had L. monocytogenes showing a possible cross-contamination or poor hygiene during preparation. Growth/no growth study with different levels of temperature, NaCl, nisin, and inoculum size revealed that avoiding the growth of RTE-isolated strains were more difficult than ATCC7644 strain. Furthermore, nisin was more effective than NaCl for suppressing the growth of L. monocytogenes and protective effect of NaCl on nisin activity was observed. This issue should be taken into consideration by the researchers and food processors before the simultaneous use of salt and nisin as chemicals in RTE foods. Probabilistic model used in this study showed high concordance and it was possible to determine the growth limits of L. monocytogenes accurately.
Authors contributions
Conceptualization, S.B. and P.Ş.; methodology, E.Ş., S.B., and P.Ş.; data analyses and modeling, S.B.; writing original draft, S.B. and P.Ş.; reviewing and editing, E.Ş., S.B., and P.Ş.; supervision, P.Ş. All authors have read and agreed to the published version of the manuscript.
Acknowledgments
Authors thank Dr. Başar Karaca for his experimental support.
Disclosure statement
No potential conflict of interest was reported by the author(s).
Data availability statement
Data are available upon request from the corresponding authors.
Additional information
Funding
References
- Dufailu, O. A.; Yaqub, M. O.; Owusu-Kwarteng, J.; Addy, F. Prevalence and Characteristics of Listeria Species from Selected African Countries. Tropical Diseases, Travel Med and Vaccines. 2021, 7(1), 26. DOI: 10.1186/s40794-021-00151-5.
- Kasalica, A.; Vuković, V.; Vranješ, A.; Memiši, N. Listeria Monocytogenes in Milk and Dairy Products. Biochem in Animal Husbandry. 2011, 27(3), 1067–1082. DOI: 10.2298/BAH1103067K.
- Sanlibaba, P.; Tezel, B. U.; Çakmak, G. A. Prevalence and Antibiotic Resistance of Listeria Monocytogenes Isolated from ready–to–eat Foods in Turkey. Hindawi J Food Qual 2018, 9. DOI:10.1155/2018/7693782. Article ID 7693782.
- Şanlıbaba, P.; Buzrul, S. Control of Listeria Monocytogenes in Milk by Using Phage Cocktail. Sci. Agropecu. 2022, 13(1), 7–14. DOI: 10.17268/sci.agropecu.2022.001.
- Fallah, A. A.; Saei-Dehkordi, S. S.; Mahzounieh, M. Occurrence and Antibiotic Resistance Profiles of Listeria Monocytogenes Isolated from Seafood Products and Market and Processing Environments in Iran. Food Control. 2013, 34(2), 630–636. DOI: 10.1016/j.foodcont.2013.06.015.
- Khen, B. K.; Lynch, O. A.; Carroll, J.; McDowell, D. A.; Duffy, G. Occurrence, Antibiotic Resistance and Molecular Characterization of Listeria Monocytogenes in the Beef Chain in the Republic of Ireland. Zoonoses Public Health. 2015, 62(1), 11–17. DOI: 10.1111/zph.12106.
- Matle, I.; Mbatha, K. R.; Madoroba, E. A Review of Listeria Monocytogenes from Meat and Meat Products: Epidemiology, Virulence Factors, Antimicrobial Resistance and Diagnosis. Onderstepoort J Vet Res. 2020, 87(1), 1–20. DOI: 10.4102/ojvr.v87i1.1869.
- Simonetti, T.; Peter, K.; Chen, Y.; Jin, Q.; Zhang, G.; LaBorde, L. F.; Macarisin, D. Prevalence and Distribution of Listeria Monocytogenes in Three Commercial Tree Fruit Packinghouses. Front. Microbiol. 2021, 12, 652708. DOI: 10.3389/fmicb.2021.652708.
- Townsend, A.; Strawn, L. K.; Chapman, B. J.; Dunn, L. L. A Systematic Review of Listeria Species and Listeria Monocytogenes Prevalence, Persistence, and Diversity Throughout the Fresh Produce Supply Chain. Foods. 2021, 10(6), 1427. DOI: 10.3390/foods10061427.
- Coroneo, V.; Carraro, V.; Aissani, N.; Sanna, C.; Ruggeri, A.; Succa, S.; Meloni, B.; Sanna, C.; Sanna, C. Detection of Virulence Genes and Growth Potential in Listeria Monocytogenes Strains Isolated from Ricotta Salata Cheese. J. Food Sci. 2016, 81(1), 114–120. DOI: 10.1111/1750-3841.13173.
- Ryser, E.T. 2021. Listeria. In: Foodborne Infections and Intoxications. Ed: Morris, J .G.Jr., and Vugia, D.J., Chapter 11, page: 201-220 Academic Press, ISBN 978-0-12-819519-2, https://doi.org/10.1016/C2018-0-02948-5
- Castrica, M.; Andoni, E.; Intraina, I.; Curone, G.; Copelotti, E.; Massacci, F. R.; Terio, V.; Colombo, S.; Balzaretti, C. M. Prevalence of Listeria Monocytogenes and Salmonella Spp. in Different Ready to Eat Foods from Large Retailers and Canteens over a 2-year Period in Northern Italy. Int. J. Environ. Res. Public Health. 2021, 18(20), 10568. DOI: 10.3390/ijerph182010568.
- Farber, J. M.; Zwietering, M.; Wiedmann, M.; Schaffner, D.; Hedberg, C. W.; Harrison, M. A.; Hartnett, E.; Chapman, B.; Donnelly, C. W.; Goodburn, K. E., et al. Alternative Approaches to the Risk Management of Listeria Monocytogenes in low-risk Foods. Food Control. 2021, 123, 107601. DOI: 10.1016/j.foodcont.2020.107601.
- European Commission Regulation (EC) No 2073/2005. On Microbiological Criteria for Foodstuffs. Official Journal of the European Union, Accessed Date: July 2, 2022 https://www.fsai.ie/uploadedFiles/Reg2073_2005(1).pdf.
- TFC (Turkish Food Codex), 2011. Microbiological Criteria Regulation. Republic of Turkey Ministry of Food, Agriculture and Livestock. Official Gazette: 29.December.2011. Issue: 28157, 33 pages, Ankara.
- Yamaki, S.; Shirahama, S.; Kobayashi, T.; Kawai, Y.; Yamazaki, K. Combined Effect of Nisin and Commercial Pectin-hydrolysate Treatment on Survival and Growth of Listeria Monocytogenes in Soy-seasoned Salmon Roe Products. Food Sci. Technol. Res. 2015, 21(5), 751–755. DOI: 10.3136/fstr.21.751.
- Boziaris, I. S.; Nychas, G.-J. E. Effect of Nisin on Growth Boundaries of Listeria Monocytogenes Scott A, at Various Temperatures, pH and Water Activities. Food Microbiol. 2006, 23(8), 779–784. DOI: 10.1016/j.fm.2006.03.003.
- Gwak, E.; Oh, M.-H.; Park, B.-Y.; Lee, H.; Lee, S.; Ha, J.; Lee, J.; Kim, S.; Choi, K.-H.; Yoon, Y. Probabilistic Models to Predict Listeria Monocytogenes Growth at Low Concentrations of NaNO 2 and NaCl in Frankfurters. Korean J. Food Sci. Animal Resour. 2015, 35(6), 815–823. DOI: 10.5851/kosfa.2015.35.6.815.
- Yoon, H.; Lee, J.-Y.; Suk, H.-J.; Lee, S.; Lee, H.; Lee, S.; Yoon, Y. Modeling to Predict growth/no Growth Boundaries and Kinetic Behavior of Salmonella on Cutting Board Surfaces. J. Food Prot. 2012, 75(12), 2116–2121. DOI: 10.4315/0362-028X.JFP-12-094.
- ISO 11290-1 (International Standardization Organization). Microbiology of the Food chain-horizontal Method for the Detection and Enumeration of Listeria Monocytogenes and of Listeria spp.-Part 1: Detection Method.
- Usman, U. B.; Kwaga, J. K. P.; Kabir, J.; Olonitola, O. S. Isolation and Antimicrobial Susceptibility of Listeria Monocytogenes from Raw Milk and Milk Products in Northern Kaduna State, Nigeria. J Appl Environ Microbiol. 2016, 4(3), 46–54. DOI: 10.12691/jaem-4-3-1.
- Awaisheh, S. S.;. Incidence and Contamination Level of Listeria Monocytogenes and Other Listeria Spp. in ready-to- Eat Meat Products in Jordan. J. Food Prot. 2010, 73(3), 535–540. DOI: 10.4315/0362-028x-73.3.535.
- Martinez-Rios, V.; Pedersen, M.; Pedrazzi, M.; Gkogka, E.; Smedsgaard, J.; Dalgaard, P. Antimicrobial Effect of Nisin in Processed cheese-Quantification of Residual Nisin by LC-MS/MS and Development of New Growth and Growth Boundary Model for Listeria Monocytogenes. Int. J. Food Microbiol. 2021, 338, 108952. DOI: 10.1016/j.ijfoodmicro.2020.108952.
- Lynch, D.; Hill, C.; Field, D.; Begley, M. Inhibition of Listeria Monocytogenes by the Staphylococcus Capitis- Derived Bacteriocin Capidermicin. Food Microbiol. 2021, 94, 103661. DOI: 10.1016/j.fm.2020.103661.
- Cuppers, H. G. A. M.; Smelt, J. P. P. M. Time to Turbidity Measurement as a Tool for Modeling Spoilage byLactobacillus. J Ind Microbiol. 1993, 12(3–5), 168–171. DOI: 10.1007/BF01584186.
- Baranyi, J.; Roberts, T. A. A Dynamic Approach to Predicting Bacterial Growth in Food. Int. J. Food Microbiol. 1994, 23(3–4), 277–294. DOI: 10.1016/0168-1605(94)90157-0.
- Zwietering, M. H.; Jongenburger, I.; Rombouts, F. M.; Van’t Riet, K. Modelling of the Bacterial Growth Curve. Appl. Environ. Microbiol. 1990, 56(6), 1875–1881.
- Buchanan, R. L.; Whiting, R. C.; Damert, W. C. When Is Simple Good Enough: A Comparison of the Gompertz, Baranyi, and three-phase Linear Models for Fitting Bacterial Growth Curves. Food Microbiol. 1997, 14(4), 313–326. DOI: 10.1006/fmic.1997.0125.
- Öksüz, H. B.; Buzrul, S. An excel-based, user-friendly Freeware Tool to Describe Microbial Growth Curves: ÖK-BUZ GRoFiT. J of Tekirdag Agric Fac. 2021, 18(3), 521–532. DOI: 10.33462/jotaf.853435.
- Leylak, C.; Buzrul, S. The Combined Effect of Temperature, pH and Lactose Concentration on the Growth Probability of Listeria Innocua. J. Microbiol. Biotechnol. Food Sci. 2020, 10(3), 474–477. DOI: 10.15414/jmbfs.2020.
- Khanipour, E.; Flint, S. H.; McCarthy, O. J.; Golding, M.; Palmer, J.; Ratkowsky, D. A.; Ross, T.; Tramplin, M. Modelling the Combined Effects of Salt, Sorbic Acid and Nisin on the Probability of Growth of Clostridium Sporogenes in a Controlled Environment (Nutrient Broth). Food Control. 2016, 62, 32–43. DOI: 10.1016/j.foodcont.2015.10.012.
- Buzrul, S.;. High Hydrostatic Pressure Inactivation of Microorganisms: A Probabilistic Model for Target log-reductions. Int. J. Food Microbiol. 2019, 309, 108330. DOI: 10.1016/j.ijfoodmicro.2019.108330.
- Ebakota, D. O.; Abiodun, O. A.; Nosa, O. O. Prevalence of Antibiotics Resistant Listeria Monocytogenes Strains in Nigerian ready-to-eat Foods. Food Saf. 2018, 6(3), 118–125. DOI: 10.14252/foodsafetyfscj.2018002.
- Cossu, F.; Spanu, C.; Deidda, S.; Mura, E.; Casti, D.; Pala, C.; Lamon, S.; Spanu, V.; Ibba, M.; Marrocu, E., et al. Listeria Spp. and Listeria Monocytogenes Contamination in ready-to-eat Sandwiches Collected from Vending Machines. Italian J. Food Safety. 2016, 5, 5500. DOI: 10.4081/ijfs.2016.5500.
- Kramarenko, T.; Roasto, M.; Meremӓe, K.; Kuningas, M.; Pöltsama, P.; Elias, T. .2013. Listeria monocytogenes prevalence and serotype diversity in various foods, Food Control, 30. DOI: 10.1016/j.foodcont.2012.06.047
- Shi, W.; Qingping, W.; Jumei, Z.; Moutong, C.; Zean, Y. Prevalence, Antibiotic Resistance and Genetic Diversity of Listeria Monocytogenes Isolated from Retail ready-to-eat Foods in China. Food Control. 2015, 47, 340–347. DOI: 10.1016/j.foodcont.2014.07.028.
- Shimojima, Y.; Ida, M.; Nakama, A.; Nishino, Y.; Fukui, R.; Kuroda, S.; Hirai, A.; Kai, A.; Sadamasu, K. Prevalence and Contamination Levels of Listeria Monocytogenes in ready-to-eat Foods in Tokyo, Japan. J. Vet. Med. Sci. 2016, 78(7), 1183–1187. DOI: 10.1292/jvms.15-0708.
- Luchansky, J. B.; Chen, Y.; Porto-Fett, A. C. S.; Pouillot, R.; Shoyer, B. A.; Johnson-DeRycke, R.; Eblen, D. R.; Hoelzer, K.; Shaw, W. K., Jr; van-Doren, J. M., et al. Survey for Listeria Monocytogenes in and on ready-to-eat Foods from Retail Establishments in the United States (2010 through 2013): Assessing Potential Changes of Pathogen Prevalence and Levels in a Decade. J. Food Prot. 2017, 80(6), 903–921. DOI: 10.4315/0362-028X.JFP-16-420.
- Maćkiw, E.; Stasiak, M.; Kowalska, J.; Kucharek, K.; Korsak, D.; Postupolski, J. Occurrence and Characteristics of Listeria Monocytogenes in ready-to-eat Meat Products in Poland. J. Food Prot. 2020, 83(6), 1002–1009. DOI: 10.4315/JFP-19-525.
- Parra-Flores, J.; Holý, O.; Bustamante, F.; Lepuschitz, S.; Pietzka, A.; Contreras-Fernández, A.; Castillo, C.; Ovalle, C.; Alarcón-Lavín, M. P.; Cruz-Córdova, A., et al. Virulence and Antibiotic Resistance Genes in Listeria Monocytogenes Strains Isolated from ready-to-eat Foods in Chile. Front. Microbiol. 2022, 12, 796040. DOI: 10.3389/fmicb.2021.796040.
- Arslan, S.; Özdemir, F. Prevalence and Antimicrobial Resistance of Listeria Species and Molecular Characterization of Listeria Monocytogenes Isolated from Retail ready-to-eat Foods. FEMS Microbiol. Lett. 2020, 367(4), fnaa006. DOI: 10.1093/femsle/fnaa006.
- Terzi, G.; Gücüklüoğlu, A.; Çadırcı, Ö.; Uyanık, T.; Alişarlı, M. Serotyping and Antibiotic Susceptibility of Listeria Monocytogenes Isolated from ready-to-eat Foods in Samsun, Turkey. Turk. J. Vet. Anim. Sci. 2015, 39, 211–217. DOI: 10.3906/vet-1407-15.
- Soultos, N.; Iossifidou, E.; Ambrahim, A.; Psomas, E.; Tzavaras, I.; Koutsopoulos, D.; Lazou, T. Listeria Monocytogenes in Mussels (Mytilus Galloprovincialis) Harvested from North Aegean Coastal Area. Turk. J. Vet. Anim. Sci. 2014, 38, 50–53. DOI: 10.3906/vet-1212-36.
- Kramarenko, T.; Roasto, M.; Keto-Timonen, R.; Maesaar, M.; Meremae, K.; Kuningas, M.; Hörman, A.; Korkeala, H. Listeria Monocytogenes in ready-to-eat Vacuum and Modified Atmosphere Packaged Meat and Fish Products of Estonian Origin at Retail Level. Food Control. 2016, 67, 48–52. DOI: 10.1016/j.foodcont.2016.02.034.
- Adeshina, I.; Abdulwahab, M.; Adewale, Y. A.; Suleiman, S. B.; Tilamiyu, L. O. Detection of Listeria Monocytogenes in Fried Fish, Processing Slab and Tools in Kwara State, Nigeria. J Vet Fac of Harran University. 2017, 6(1), 32–37. DOI: 10.31196/huvfd.325713.
- Al-Nabulsi, A. A.; Osaili, T. M.; Awad, A. A.; Olaimat, A. N.; Shaker, R. R.; Holley, R. A. Occurrence and Antibiotic Susceptibility of Listeria Monocytogenes Isolated from Raw and Processed Meat Products in Amman, Jordan. CyTA J Food. 2015, 13(3), 346–352. DOI: 10.1080/19476337.2014.982191.
- Zhu, Q.; Gooneratne, R.; Hussain, M. A. Listeria Monocytogenes in Fresh Produce: Outbreaks, Prevalence and Contamination Levels. Foods. 2017, 6(3), 21. DOI: 10.3390/foods6030021.
- Bustamante, F.; Maury-Sintjago, E.; Leal, F. C.; Acuña, S.; Aguirre, J.; Troncoso, M.; Figueroa, G.; Parra-Flores, J. Presence of Listeria Monocytogenes in ready-to-eat Artisanal Chilean Foods. Microorganisms. 2020, 8(11), 1669. DOI: 10.3390/microorganisms8111669.
- Marnissi, B. E.; Bennani, L.; Cohen, N.; Lalami, A. E. O.; Belkhou, R. Presence of Listeria Monocytogenes in Raw Milk and Traditional Dairy Products Marketed in the North-central Region of Morocco. African Journal of. Food Sci. 2013, 7(5), 87–91. DOI: 10.5897/AJFS2013.0992.
- Seyoum, E. T.; Woldetsadik, D. A.; Mekonen, T. K.; Gezahegn, H. A.; Gebreyes, W. A. Prevalence of Listeria Monocytogenes in Raw Bovine Milk and Milk Products from Central Highlands of Ethiopia. J. Infect. Developing Countries. 2015, 9(11), 104–1209. DOI: 10.3855/jidc.6211.
- CDC, 2022. https://www.cdc.gov/listeria/outbreaks/hispanic-soft-cheese-02-21/index.html Accessed Date: June 24, 2022.
- Dalgaard, P.; Ross, T.; Kamperman, L.; Neumeyer, K.; McMeekin, T. A. Estimation of Bacterial Growth Rates from Turbidimetric and Viable Count Data. Int. J. Food Microbiol. 1994, 23(3–4), 391–404. DOI: 10.1016/0168-1605(94)90165-1.
- Augustin, J.-C.; Rosso, L.; Carlier, V. Estimation of Temperature Dependent Growth Rate and Lag Time of Listeria Monocytogenes by Optical Density Measurements. J. Microbiol. Methods. 1999, 38(1–2), 137–146. DOI: 10.1016/S0167-7012(99)00089-5.
- Biesta-Peters, E. G.; Reij, M. W.; Joosten, H.; Gorris, L. G. M.; Zwietering, M. H. Comparison of Two Optical-Density-Based Methods and a Plate Count Method for Estimation of Growth Parameters of Bacillus Cereus. Appl. Environ. Microbiol. 2010, 76(5), 1399–1405. DOI: 10.1128/AEM.02336-09.
- Boziaris, I. S.; Skandamis, P. N.; Anastasiadi, M.; Nychas, G.-J. E. Effect of NaCl and KCl on Fate and growth/no Growth Interfaces of Listeria Monocytogenes Scott A at Different pH and Nisin Concentrations. J. Appl. Microbiol. 2006, 102(3), 796–805. DOI: 10.1111/j.1365-2672.2006.03117.x.
- Parente, E.; Giglio, A. M.; Ricciardi, A.; Clementi, F. The Combined Effect of Nisin, Leucocin F10, pH, NaCl and EDTA on the Survival of Listeria Monocytogenes in Broth. Int. J. Food Microbiol. 1998, 40(1–2), 65–75. DOI: 10.1016/s0168-1605(98)00021-x.
- Thomas, L. V.; Wimpenny, J. W. T. Investigations of the Effect of Combined Variations in Temperature, pH and NaCl Concentration on Nisin Inhibition of Listeria Monocytogenes and Staphylococcus Aureus. Appl. Environ. Microbiol. 1996, 62(6), 2006–2012. DOI: 10.1128/aem.62.6.2006-2012.1996.
- De Martinis, E. C. P.; Crandall, A. D.; Mazzotta, A. S.; Montville, T. J. Influence of pH, Salt, and Temperature on Nisin Resistance in Listeria Monocytogenes†. J. Food Prot. 1997, 60(4), 420–423. DOI: 10.4315/0362-028X-60.4.420.
- Bouttefroy, A.; Mansour, M.; Linder, M.; Milliere, J.-B. Inhibitory Combinations of Nisin, Sodium Chloride, and pH on Listeria Monocytogenes ATCC 15313 in Broth by an Experimental Design Approach. Int. J. Food Microbiol. 2000, 54(1–2), 109–115. DOI: 10.1016/s0168-1605(99)00171-3.
- Koutsoumanis, K.P., Sofos, J.N. 2005. Effect of inoculum size on the combined temperature, pH and a(w) limits for growth of Listeria monocytogenes. International Journal of Food Microbiology, 104(1), 83–91. https://org/j.ijfoodmicro.2005.01.010