ABSTRACT
Tap water treated in air with low-temperature and low-pressure glow plasma of low frequency was tested for its either stimulation or inhibition of the growth of the selected microorganisms commonly colonizing human organism. The growth of chosen microorganisms was monitored by estimation of optical density of their colonies. The fairly linear growth against time of all microorganisms under study accelerated after 12 h from the beginning of the experiment. Colonies of E. coli and S. cerevisiae breed in the plasma treated water had an approximately 20% stimulation of the growth which was observed between 12 and 24 h. Neither stimulation nor inhibition of the growth could be noted for colonies of Aspergillus niger, Candida albicans, Yarrowia lipolytica, and Enterococcus faecalis, in whole period of observation. The plasma-treated water had no effect upon the growth of Mycobacteria. Independently of the water tested, M. tuberculosis started proliferating on the 14th day of the experiment, M. intercellulare and M. kansai after 9 days, and the growth of M. fortuitos could be observed after 3 days.
Introduction
All known kinds of plasma are capable of breaking chemical bonds. They generate either/and atomic oxygen, hydrogen peroxide, and ozone. Hence, they can initiate chemical reactions.[Citation1–25] In contrast to them, a treatment of water with invented by Oszczęda et al.[Citation26,Citation27] low-temperature, low-pressure glow plasma of low frequency (LPGP) machine solely declusterizes the water macrostructure into smaller structural units. In this manner, the latter plasma provides a liquid with unique physical, chemical, biochemical, and functional properties.[Citation28] Since LPGP cannot break valence bonds, it does not initiate chemical reactions and it generates neither atomic oxygen radical nor perhydrol and ozone.
Water treated with LPGP in the air (LPGPA) better dissolves several compounds, among them also mineral salts. Simultaneously, LPGPA more readily penetrates cells of living organisms. For that sake LPGPA should be a good vector transporting such compounds to the cells. It has been proven for entomopathogenic fungi employed as biopesticides, fermentative microorganisms, and in animal breeding. Application of LPGPA appeared beneficial in agriculture and in cosmetology.[Citation29–37] Potentially, LPGPA could be used for preparation of various soft drinks and as media useful in human prophylaxis and therapy. However, thus far, nothing is known about the effect of LPGPA upon bacteria, fungi, and yeast-colonizing the human body. It induced these studies on the effect of LPGPA on selected microorganisms commonly colonizing the human organism and beging delivered to it. The group of selected bacteria included strains of Escherichia coli, Staphylococcus aureus, Enterococcus faecalis, Klebsiella pneumoniae, Mycobacterium tuberculosis, M. intracellulare, M. kansasii, and M. fortuitum.
Although a majority of E. coli strains are harmless, some strains cause harmful food poisoning.[Citation38,Citation39] The harmless strains constitute the normal microbiota of the gut, producing K2 vitamin and benefiting their hosts, helping blood to clot, and hindering their intestines from colonization with pathogenic bacteria of a symbiotic relationship.[Citation40]
S. aureus, another Gram-positive bacterium, a facultative anaerobe that constitutes the microbiota of the body. It occupies the upper respiratory tracts and the skin. Turning into an opportunistic pathogen, it becomes a common cause of skin infections including abscesses and respiratory infections. Antibiotic-resistant strains of S. aureus have become a problem in clinical medicine.[Citation41]
E. faecalis, a Gram-positive, commensal bacterium, colonizes gastrointestinal tracts. It can be responsible for life-threatening infections, especially in hospitals,[Citation42] and reinfection of root canal-treated teeth.[Citation43] K. pneumoniae, a Gram-negative, non-motile facultative anaerobic bacterium, resides in the normal flora of the mouth, skin, and intestine. It causes destructive changes to the human lungs. It is also an important pathogen in nosocomial infections.[Citation42]
M. tuberculosis, an acid-fast (Ziehl-Neelsen stain), weakly Gram-positive bacterium, is an etiological agent of tuberculosis. It belongs to the family Mycobacteriaceae as a part of the group of mycobacteria.[Citation44] Tubercle bacillus is an aerobic (or microaerophilic) immobile, anaerobic bacterium. It does not form spores or toxins. M. tuberculosis is a complex of at least nine members. The cell wall of mycobacteria contains about 60% of lipids such as mycolic acids, lipoarabinomannan, and waxes, which determine its acid resistance. M. tuberculosis attacks lungs, kidney, spine, and brain. The disease caused by M. tuberculosis can be fatal.[Citation42]
The effect of LPGPA on a group of fungi was studied involving an Aspergillus niger strain producing ochratoxin A and other mycotoxins. It is one of the most abundant food-contaminating mycotoxin. The consumption of this fungus produces chronic neurotoxic, immunosuppressive, genotoxic, carcinogenic, and teratogenic effects.[Citation45] Its airborne spores evoke asthma in children and lung diseases.[Citation46]
Strains of Candida albicans, Yarrowia lipolytica, and Saccharomyces cerevisiae were employed to study the effect of LPGPA on yeasts. C. albicans is an opportunistic pathogenic yeast[Citation47] considered as a dimorphic fungus since it grows both as yeast and filamentous cells. It consists of several morphological phenotypes.[Citation48] It is common in the human gut flora and biofilms formed either on implanted medical devices or on human tissues.[Citation49] C. albicans is usually a commensal organism, but it can become pathogenic in immunocompromised individuals under a variety of conditions.[Citation50,Citation51] S. cerevisiae, a eukaryotic model organism, is a single-celled fungus microorganism. S. cerevisiae can cause invasive, life threatening infections.[Citation52] Y. lipolytica yeast species synthesize a wide group of lipases and other hydrolytic enzymes, microbial oil, citric acid, erythritol, and γ-decalactone. Although that yeast is nonpathogenic, it can induce infections in immunocompromised and critically ill patients.[Citation53]
In this study, the influence of LPGPA on the growth of the aforementioned microorganisms was examined. The results provided an evidence for consumers, infected with those microorganisms, whether they can safely drink LPGPA and drinks prepared on its basis. Additionally, it was evaluated for its possibility to apply LPGPA for prophylaxis and therapy.
Materials and methods
Materials
Test strains of E. coli ATCC 25922, S. aureus ATCC 25923, E. faecalis ATCC 19433, and K. pneumoniae ATCC 13883, originated from the American Type Culture Collection (Manassas, VA, USA) and wild environmental strains, were taken from own microorganisms collection of the Cracow University of Technology. M. tuberculosis strains, M. tuberculosis H37Ra ATCC 25177, M. tuberculosis with resistance to Rifampicin and Isoniazid, M. intracellulare ATCC 13950, M. kansasii ATCC 12478, and M. fortuitum ATCC 6841, were obtained from the Malopolska Central Laboratory of Tuberculosis Diagnostics, Cracow. Strain of C. albicans ATCC 90028 originated from the American Type Culture Collection (Manassas, VA, USA), and wild environmental strain of C. albicans was taken from the collection of the British National Collection of Yeast Cultures. S. cerevisiae DSM 1333 and Y. lipolytica DSM 1345 were taken from DMZS German Collection of Microorganisms and Cell Cultures GmbH in Braunschweig Germany.
Substrates
Bacteria were raised on TSA medium (trypticase soy agar) from Biomaxima, Lublin, Poland. The medium was sterilized for 15 min in an autoclave at 121°C at 2 bar. For the agar well diffusion method, TSA medium with 5 vol% ram blood was applied (Biomaxima, Lublin, Poland) according to Budzyńska et al.[Citation54] Fungi were raised on the Sabouraud agar medium (Biomaxima, Lublin, Poland). The medium was sterilized for 15 min in an autoclave at 121°C and pressure equal to 2 bar. For yeasts, cells were grown on the YPD medium supplemented with 2% agar (Yeast Peptone Dextrose; Merck, Germany).[Citation55] That medium was sterilized as above. Commercially available spring water containing 335 mg minerals/l (41 mg Ca2+/L, 24 mg Mg2+/L, 9 mg Na+/L, 2 mg K+/L, 220 mg HCO3−/L, 36 mg SO42-/L, 3 mg Cl−/L) was used as a control.
Water treatment
Deionized water (200 mL) in 250 mL polyethylene bottles was placed in the reactor chamber[Citation26] and exposed to LPGP for 30 min. Plasma of 38°C was generated at 5 × 10–3 mbar, 600 V, 50 mA, and 280 GHz frequency. Treated water (LPGPA) was stored at room temperature in closed Teflon containers for no longer than one week prior to the experiments.
Breeding of microorganisms
Investigated bacteria, except M. tuberculosis strains, were passed onto Petri dishes with the TSA solid medium (Tryptic Soya Agar, Biomaxima, Lublin, Poland) and stored for 24 h at 44°C and 37°C. Fungi were raised for 120 h on the Petri dishes with Sabouraud solid medium (Biomaxima, Lublin, Poland) at 28°C, whereas yeasts were bread at 28°C for 72 h on the Petri dishes using solid YPD medium (Merck, Germany).
Microorganism growth
Microorganisms were sieved under a laminar flow chamber into sterilized 250 mL conical flasks containing 40 mL of liquid medium (formulated with LPGPA). The cultures were then incubated at appropriate temperature. The growth liquid medium was prepared with 1 g of peptone, 1 g of yeast extract, and 8 g of sucrose which were weighed successively into a glass screw capped bottle. Mineral salt solution was prepared by dissolving 6 g MgSO4, 10 g K2HPO4, 2.4 CaCl2, and 60 g (NH4)2SO4 in 1 L distilled water. Twenty-five milliters of that solution was added to a glass bottle containing a previously prepared liquid medium. The whole was diluted with 500 mL LPGPA and sterilized in an autoclave at 121°C for 15 min at 2 MPa.
Optical density measurements – growth evaluation
Spectrophotometric optical density (OD) measurements with a Rayleigh model UV 1800 spectrophotometer at 540 nm were carried out. The growth of microorganisms was evaluated based on the changes in the optical density in 1 mL suspensions at a given time. An increase in the solution cloudiness was measured.
Sample preparation for measuring the effect of LPGPA on M. tuberculosis growth
Suspensions of 0.5 McFarland density were prepared from colonies of microorganisms. Inoculum of each strain was transferred onto Lowenstein-Jensen solid media with calibrated inoculation loop. Colonies ground in a glass microbial mortar were suspended in 0.5 mL LPGPA and transferred into a sterile tube and supplemented with LPGPA to 5 mL. The density of those suspensions was measured using a densitometer and, if necessary, more LPGPA was added to achieve the desired 0.5 McFarland density. The resulting bacterial suspensions (10 µL) after calibration with inoculation loop were inoculated onto Lowenstein-Jensen solid media. The seeded media were placed in a plastic tray and incubated at 37°C for 16 days, taking a reading every 24 h.
Statistical analysis
The experiments were conducted in triplicates. The obtained results were averaged. In all of the experiments, the observed values are given with ±5% standard deviations from the mean.
Results and discussion
Within the 32 h period of growth of all microorganisms some stages could be distinguished. The most frequent, occurring after a relatively fast 0–5th step growth slowed down until 24th h in order to accelerate after to the end of the experiment. Within the 32 h incubation the growth kinetics of bacterial strains of E. coli ATCC 25922, S. aureus ATCC 25923, E. faecalis ATCC 19433, and K. pneumoniae ATCC 13883 in LPGPA closely resembled that observed in the non-treated, control water ().
Figure 1. Comparison of prokaryotic microorganism cell growth measured as optical density (OD) in control medium (C) and plasma water-based medium (PL): A – Escherichia coli, B – Staphylococcus aureus, C – Enterococcus faecalis, D – Klebsiella pneumoniae. The tests were carried out in triplicates and averaged. Standard errors were below 5%.
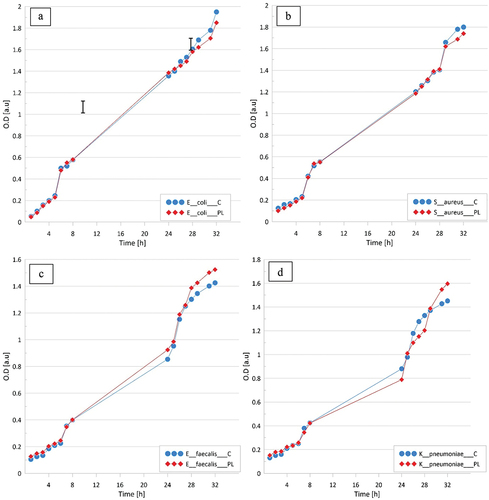
In case of E. coli and S. aureus, some subtle effects of the treatment with LPGPA could be observed after 28 h LPGPA suppressed the bacteria growth to a negligible extent. The insignificant effect of LPGPA upon the growth of E. faecalis and K. pneumonia could be observed already between 10th and 15th h. It then rose up until the 32nd h of experiment, still remaining subtle. Contact with LPGPA subtly either inhibited or stimulated the growth, respectively, depending on the test duration. LPGPA did not stimulate the growth of M. tuberculosis ().
Table 1. Comparison of growth of M. tuberculosis strains in the presence and absence of LPGPA in the culture mediuma,b
In the control tests, the colonies of M. fortuitum, M. intracellulare, and M. kansasii and both strains of M. tuberculosis started rising on the 3rd, 9th, and 14th day, respectively. The contact with LPGPA did not influence that sequence. Similar responses from A. niger, C. albicans, and Y. lipolytica fungi showed that LPGPA was neither mycostatic nor mycocidal (), although between 9th and 24th h of the experiment, an approximately 10% inhibition growth could be observed for A. niger and Y. lipolytica.
Figure 2. Comparison of eukaryotic cell growth measured as optical density (OD) in control medium (C) and plasma water-based medium (PL). A – Saccharomyces cerevisiae, B – Candida albicans, C – Yarrowia lipolytica, D – Aspergillus niger. The tests were carried out in triplicates and averaged. Standard errors were below 5%.
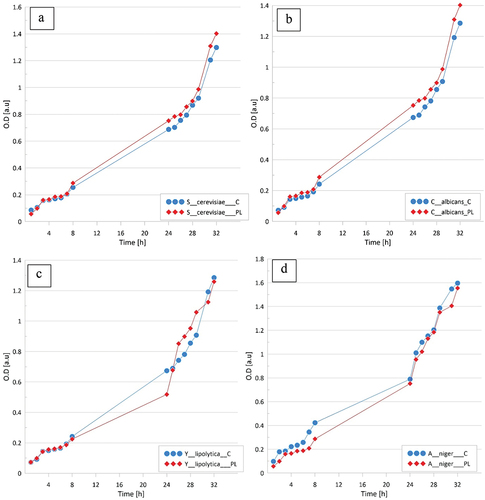
C. albicans appeared insensitive to LPGPA and slight stimulation of S. cerevisiae was noted from the 8th day. The growth of Y. lipolytica and A. niger fungi was inhibited. Also, the growth of eukaryotic cells did not change. In order to check whether LPGPA would influence the growth of chosen microorganisms in less favorable conditions, experiments were carried out with a medium containing only mineral salts. The results were identical.
Differences in the kinetics of growth of E. coli and S. cerevisiae in the water-based minimal medium () revealed that LPGPA facilitated the transport of substances across the cell membranes and simultaneously, an approximately 20% stimulation of the growth could be noted in the period between 9th and 24th h. Inspection of revealed that LPGPA did not influence the growth of Mycobacteria. Regardless, these microorganisms were maintained in 0.9% aq. solution of physiological salt or in LPGPA, and the observable increase in microorganisms of M. fortuitum took place on the third day of experiment, propagation of M. intracellulare and M. kansai could be seen on the 9th day, and M. tuberculosis developed just in the 14th day of experiment ().
Figure 3. Comparison of cell growth in control medium (C) and plasma water-based minimal medium (PL). A – Escherichia coli, B – Saccharomyces cerevisiae. The tests were carried out in triplicates and averaged. Standard errors were below 5%.
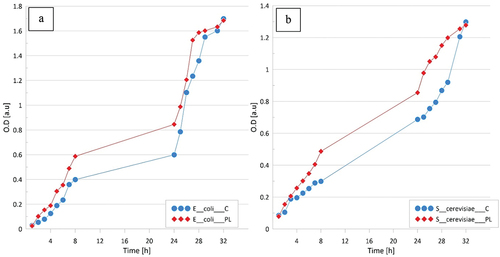
The results showed that LPGPA did not increase the bacterial population although it facilitated the transport of substances across the cell membranes.[Citation29,Citation30] Gram positive and Gram negative bacteria showed no difference in response to the contact with LPGPA. Additional experiments were carried out with the use of a medium containing only mineral salts. Differences in kinetics of the growth of E. coli and S. cerevisiae were observed () supporting an assumption that LPGPA may facilitate the transport of substances across the cell membrane. Therefore, LPGPA can be safely used as potable water and for preparation of various soft drinks.
Conclusion
Water treated with low-temperature, low-pressure glow plasma of low frequency (LPGPA), regardless of the applied method of its preparation, neither inhibits nor stimulates the growth of yeast, fungi, and bacteria including Mycobacterium microorgnisms. The results provide an evidence that the water treated with low-temperature, low-pressure glow plasma of low frequency is safe in several everyday applications, that is, for preparing various drinks and also for prophylaxis and therapy. LPGPA can be safely drunk by consumers with infectious diseases. Because LPGPA is a good vector for solutes dissolved in it, the use of drinks prepared with such kind of water can be beneficial.
Disclosure statement
No potential conflict of interest was reported by the author(s).
Additional information
Funding
References
- Doughty, D. A.; Hartog, E. A. D.; Lawler, J. E. Current Balance at the Surface of a Cold Cathode. Phys. Rev. Lett. 1987, 58, 2668–2671. DOI: 10.1103/PhysRevLett.58.2668.
- Donsbach, K. W.; Cazares, R. Process for Making Highly Oxygenated Drinking Water and Drinking Water Made by the Process. U.S. Patent 5587191A, 24 December 1996.
- Zelenak, Z. M.; Berzsenyi, L.; Abramo, F. Oxygen Enriched Liquids, Method and Apparatus for Making, and Applications Thereof. U.S. Patent 581422A, 29 September 1998.
- Miichi, T.; Hayashi, N.; Ihara, S.; Satoh, S.; Yamade, C. Ozone Generation inside Bubbles in Water. IEEJ Trans. Fundam. Mater. 2001, 121, 448–452. DOI: 10.1541/ieejfms1990.121.5_448.
- DeWald, J. J. Method and Apparatus for Adding Oxygen to Drinking Water. U.S. Patent 69361179B2, 30 August 2005.
- Danone, C. G. Method for Enriching Water with Oxygen by an Electrolytic Process, Oxygen Enriched Water or Beverage and Uses Thereof. U.S. Patent 8,709,231, 19 December 2008.
- Sato, K.; Yasuoka, K.; Ishii, S. Water Treatment with Pulsed Plasmas Generated inside Bubbles. IEEJ Trans. Fundam. Mater. 2008, 128, 401–406. DOI: 10.1541/IEEJFMS.128.401.
- Verreycken, T.; Schram, D. C.; Leys, C.; Bruggeman, P. J. Spectroscopic Study of an Atmospheric Pressure Dc Glow Discharge with a Water Electrode in Atomic and Molecular Gases. Plasma Sources Sci. Technol. 2010, 19,45004. DOI: 10.1088/0963-0252/19/4/045004.
- Nemcova, L.; Nikiforov, A.; Leys, C.; Krcma, F. Chemical Efficiency of {H}_{2}{O}_{2} Production and Decomposition of Organic Compounds under Action of DCC under Water Discharge in Gas Bubbles. IEEE Trans. Plasma. Sci. 2011, 39, 865–870. DOI: 10.1109/TPS.2010.2098053.
- Takahashi, K.; Yagi, I.; Takaki, K.; Satta, N. Development of Pulsed Discharge inside Bubble in Water. IEEE Trans. Plasma. Sci. 2011, 39(11), 2654–2655. DOI: 10.1109/TPS.2011.2164095.
- Sommers, B.; Foster, J. Plasma Formation inside Deformed Gas Bubbles Submerged in Water. In: Proc. Am. Phys. Soc. 65th Annual Gaseous Electronics Conference, Austin, TX, USA, 22–26 October 2012. https://scholar.google.com/scholar_lookup?title=Plasma+formation+inside+deformed+gas+bubbles+submerged+in+water&conference=Proceedings+of+the+American+Physical+Society,+65th+Annual+Gaseous+Electronics+Conference&author=Sommers,+B.&author=Foster,+J.&publication_year=2012
- Muradia, S.; Nagatsu, M. Low-voltage Pulsed Plasma Discharges inside Water Using a Bubble self-generating Parallel Plate Electrode with a Porous Ceramic. Appl. Phys. Lett. 2013, 102(14), 144105. https://doi.org/10.1063/1.4799652
- Pranevicius, L.; Tuckute, S.; Gedvilas, K.; Gedvilas, K. Water vapor-plasma-enhanced Oxidation of Thin Titanium Films. Acta Phys. Polon. A. 2013, 123, 907–910. DOI: 10.12693/APhysPolA.123.907.
- Hayashi, Y.; Takada, N.; Kanda, H.; Goto, M. Generation of Pulsed Discharge Plasma in Water with Fine Bubbles. Proc. APS Gaseous Conf. 2015, LW1. 123. https://jglobal.jst.go.jp/en/detail?JGLOBAL_ID=202102254752813848
- Takahashi, K.; Konno, R.; Akiyama, M.; Takaki, K.; Satta, N. Improvement of Energy Efficiency for de Colorization of Organic Dye by Discharge inside Bubble in Water, 2016. IEEE Int. Conf. Plasma Sci. (ICOPS) 2016, 1. DOI: 10.1109/PLASMA.2016.7533948.
- Shang, K.; Li, J.; Wang, X.; Yao, D.; Lu, N.; Jiang, N.; Wu, Y. Evaluating the Generation Efficiency of Hydrogen Peroxide in Water by Pulsed Discharge over Water Surface and Underwater Bubbling Pulsed Discharge. Jpn. J. Appl. Phys. 2015, 55(1S), 01AB02. DOI: 10.7567/JJAP.55.01AB02.
- Iwabuchi, M.; Takahashi, K.; Takaki, K.; Satta, N. Influence of Sodium Carbonate on Decomposition of Formic Acid by Pulsed Discharge Plasma inside Bubble in Water. Jpn. J. Appl. Phys. 2016, 55(7S2), 07LF02. DOI: 10.7567/JJAP.55.07LF02.
- Khristolubova, V. I.; Kashapov, N. F.; Shaekhov, M. F. Gas and Plasma Dynamics of RF Discharge Jet of low-pressure in a Vacuum Chamber with Flat Electrodes and inside Tube, Influence of RF Discharge on the Steel Surface Parameters. IOP Conf. Ser. Mater. Sci. Eng. 2016, 134, 012017. DOI: 10.1088/1757-899X/134/1/012017.
- Liu, J.; He, B.; Chen, Q.; Li, J.; Xiong, Q.; Yue, G.; Zhang, X.; Yang, S.; Liu, H.; Liu, Q. H. Direct Synthesis of Hydrogen Peroxide from plasma-water Interactions. Sci. Rep. 2016, 6(1), 38454. DOI: 10.1038/srep38454.
- Sharma, A.; Levko, D.; Raja, L. L.; Cha, M. S. Kinetics and Dynamics of Nanosecond Streamer Discharge in atmospheric-pressure Gas Bubble Suspended in Distilled Water under Saturated Vapour Pressure Conditions. J. Phys. D: Appl. Phys. 2016, 49, 395205. DOI: 10.1088/0022-3727/49/39/395205.
- Chen, Z.; Krasik, Y. E.; Cousens, S.; Ambujakshan, A.; Corr, C.; Dai, X. J. Generation of Underwater Discharges inside Gas Bubbles Using a 30-needles-to-plate Electrode. J. Appl. Phys. 2017, 122, 153303. DOI: 10.1063/1.4993497.
- Takahashi, K.; Takeda, M.; Konno, R.; Takaki, K.; Satta, N. Influence of Electric Parameters on Hydroxyl Radical Production by Positive Pulsed Discharge inside of a Bubble in Water. IEEE Trans. Plasma. Sci. 2018, 47, 1105–1113. DOI: 10.1109/TPS.2018.2883767.
- Hagen, R. Plasma-treated Water as a Superior Electrolyte. accessed on 3 July 2017. https://www.advancedsciencenews.com/plasma-treated-water-superior-electrolyte/
- Yui, H.; Someya, Y.; Kusama, Y.; Kanno, K.; Banno, M. Atmospheric Discharge Plasma in Aqueous Solution: Importance of the Generation of Water Vapor Bubbles for Plasma Onset and Physicochemical Evolution. J. Appl. Phys. 2018, 124, 103301. DOI: 10.1063/1.5040314.
- Messer Americas, F. A. R. M. O. X. Drop-in Oxygenation Apparatus. New, Easy-to-deploy, Highly Efficient Solution for Oxygenation of Water. accessed on 25 June 2020. https://cdn2.hubspot.net/hubfs/189660/Messer%20US%20Website_2019/Resources/MESS-3024_FARMOX_Dropln_datasheet.pdf
- Oszczęda, Z.; Elkin, I.; Stręk, W. Equipment for Treatment of Water with Plasma Polish Patent. Appl. PL 216025 B1, 20.11. 2014, .
- Reszke, E.; Yelkin, L.; Oszczęda, Z. Plasming Lamp with Power Supply, Polish Patent PL 227530 B1, 2017.
- Białopiotrowicz, T.; Ciesielski, W.; Domański, J.; Doskocz, M.; Fiedorowicz, M.; Grąż, K.; Khachatryan, K.; Kołoczek, H.; Kozak, A.; Oszczęda, Z., et al. Structure and Physicochemical Properties of Water Treated with low-temperature low-frequency Glow Plasma. Curr. Phys, Chem. 2016, 6, 312–320. DOI: 10.2174/1877946806666161118152613.
- Tomasik, P.;. Fundamentals of Food Nanotechnology, Essentials. Lambert Academic Publishing: Saarbruecken, 2017; Vol. 20017, pp 63.
- Tomasik, P.;. Fundamentals of Nanotechnology of Food and Cosmetics (In Polish). Sophia Scientific Editorial Board: Warsaw, 2019; pp 54.
- Szymanowicz, J.; Schwarz, T.; Murawski, M.; Małopolska, M.; Oszczęda, Z.; Tuz, R.; Nowicki, J.; Bartlewski, P. M. Storage, of Bear Semen at 16-18°C in the Long Term Commercial Extender Prepared with Deionized Water or Nanowater. Anim. Reprod. 2019, 164, 1–7. DOI:10.2478/aoas-2022-0008.
- Ciesielska, K.; Ciesielski, W.; Kulawik, D.; Oszczęda, Z.; Tomasik, P. Cultivation of Cress Involving Water Treated under Different Atmospheres with Low-Temperature, Low- Pressure Glow Plasma of Low Frequency. Water. 2020, 12, 2152. DOI: 10.3390/w12082152.
- Ciesielska, K.; Ciesielski, W.; Girek, T.; Kołoczek, H.; Oszczęda, Z.; Tomasik, P. Reaction of Lavandula angustifolia Mill. to Water Treated with Low-Temperature, Low-Pressure Glow Plasma of Low Frequency. Water. 2020, 12, 3186. DOI: 10.3390/w12113168.
- Ciesielska, K.; Ciesielski, W.; Girek, T.; Oszczęda, Z.; Tomasik, P. Effect of Watering of Selected Seasoning Herbs with Water Treated with Low-temperature, Low-pressure Glow Plasma of Low Frequency. Water. 2020, 12, 3526. https://doi.org/10.3390/w12123526.
- Ciesielski, W.; Gąstoł, M.; Kulawik, D.; Oszczęda, Z.; Pisulewska, E.; Tomasik, P. Specific Controlling Essential Oil Composition of Basil (Ocimum basilicum L.) Involving Low-temperature, Low-pressure Glow Plasma of Low Frequency. Water. 2020, 12, 3332. https://doi.org/10.3390/w12123332.
- Pater, A.; Zdaniewicz, M.; Satora, P.; Khachatryan, G.; Oszczęda, Z. Application of Water Treated with Low-temperature Low-pressure Glow Plasma for Quality Improvement of Barley and Malt. Biomolecules. 2020, 10, 267. DOI: 10.3390/biom10020267.
- Ciesielski, W.; Domagała, I.; Garcia, B.; Girek, T.; Oszczęda, Z.; Szczuka, E.;.; Tomasik, P. Specific Way of Controlling Composition of Cannabinoids and Essential Oil from Cannabis sativa var. Finola. Water. 2022, 14, 688. DOI: 10.3390/w14050688.
- Tenaillon, O.; Skurnik, D.; Picard, B.; Denamur, E. The Population Genetics of Commensal Escherichia coli. Nature Revs. Microbiol. 2010, 83, 207–217. DOI:10.1038/nrmicro2298.
- Center for Disease Control and Prevention. Escherichia coli,; Saving Lives, Protecting People. 2020. CDC 24/7. https://www.cdc.gov/ecoli/index.html
- Reid, G.; Howard, J.; Gan, B. S. Can Bacterial Interference Prevent Infection? Trends Microbiol. 2001, 9(9), 424–428. DOI: 10.1016/s0966-842x(01)02132-1.
- Tong, S. Y.; Davis, J. S.; Eichenberger, E.; Holland, T. L.; Fowler, V. G. Staphylococcus aureus Infections: Epidemiology, Pathophysiology, Clinical Manifestations and Management. Clin. Microbiol. Revs. 2015, 28, 603–661. DOI: 10.1128/cmr.00134-14.
- Ryan, K. J.; Ray, C. G. Sherris Medical Microbiology. J. Biosci. Meds. 2004, 3(7), 294–295.
- Rocas, I.; Siqueira, J.; Santos, K. Association of Enterococcus faecalis with Different Forms of Periradicular Diseases. J. Endod. 2004, 30, 315–320. DOI: 10.1097/00004770-200405000-00004.
- Virella, G.;. Microbiology and Infectious Diseases(in Polish). Urban & Partner: Wrocław, 2000.
- Ravelo Abreu, A.; Rubio Armendáriz, C.; Gutiérrez Fernández, A. J.; Hardisson, D. L. T.; Ochratoxin, A. A in Foods for Human Consumption: Review (In Spanish). Nutr. Hospital. 2011, 26, 1215–1226. DOI: 10.1590/S0212-16112011000600004.
- Reponen, T.; Lockey, J.; Bernstein, D. I.; Vesper, S. J.; Levin, L.; Khurana Hershey, G. K.; Zheng, S.; Ryan, P.; Grinshpun, S. A.; Villareal, M., et al. Infant Origins of Childhood Asthma Associated with Specific Moulds. J. Allergy Clin. Immunol. 2012, 130, 639–644.e5. DOI: 10.1016/j.jaci.2012.05.030.
- Gow, N. A. R.; Yadav, B. Microbe Profile: Candida albicans: A shape-changing Opportunistic Pathogenic Fungus of Humans. Microbiology. 2017, 163, 1145–1147. DOI: 10.1099/mic.0.000499.
- Basso, V.; d’Enfert, C.; Znaidi, S.; Bachellier-Bassi, S. From Genes to Networks: The Regulatory Circuitry Controlling Candida albicans Morphogenesis. Curr. Topics Microbiol. Immunol. 2019, 422, 61–99. DOI: 10.1007/82_2018_144.
- Kumamoto, C. A. Candida Biofilms. Curr. Opin.Microbiol. 2002, 5, 608–611. DOI: 10.1016/s1369-5274(02)00371-5.
- Martins, N.; Ferreira, I. C.; Barros, L.; Silva, S.; Henriques, M. Candidiasis: Predisposing Factors, Prevention, Diagnosis and Alternative Treatment. Mycopathologia. 2014, 177(5–6), 223–240. DOI: 10.1007/s11046-014-9749-1.
- Erdogan, A.; Rao, S. S. Small Intestinal Fungal Overgrowth. Curr. Gastroenterol. Repts. 2015, 174, 16. DOI:10.1007/s11894-015-0436-2.
- Enache-Angoulvant, A.; Hennequin, C. Invasive Saccharomyces Infection; A Comprehensive Review. Clin. Inf. Dis. 2005, 41, 1559–1568. DOI: 10.1086/497832.
- Zieniuk, B.; Fabiszewska, A. Yarrowia lipolytica: A Beneficious Yeast in Biotechnology as a Rare Opportunistic Fungal Pathogen: A Minireview. World J. Microbiol. Biotechnol. 2019, 35(1), 10. DOI: 10.1007/s11274-018-2583-8.
- Budzyńska, A.; Więckowska-Szakiel, M.; Kalemba, D.; Sadowska, B.; Różalska, B. Verification of Methodical Parameters of Determination of Bactericidal Activity of Essential Oils (In Polish). Med. Dośw. Mikrobiol. 2009, 61. 281–287. http://www.medmikro.org/weryfikacja-metodycznych-parametrow-oznaczania-przeciwbakteryjnej-aktywnosci-olejkow-eterycznych?lang=pl
- Atlas, R. M.;. Handbook of Microbiological Media, 4th ed. CRC Press: Boca Raton, 2004.