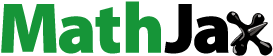
ABSTRACT
The current study aimed to investigate the phenolic and flavonoid contents, cytotoxicity, genotoxicity, and mutagenicity of ethanolic and aqueous extracts of A. roseum (Camb.). The MTT assay was used to determine cytotoxicity, Comet assay was used to determine genotoxicity and mutagenicity of extracts was assessed through Ames test. The results showed that total phenolic contents were higher in aqueous extract (538.26 mg GAE/100 g); however, total flavonoid contents were significantly higher in ethanolic extract (6553.29 mg Eq. of Quercetin/100 g). The cells survival percentage was significantly (P <.05) decreased as concentrations of ethanolic extract increased. However, no significant toxicity was observed in groups treated with 0.031–0.25 mg/ml of ethanolic extract of A. roseum compared to negative control (P > .05). IC50 of ethanolic and aqueous extract of A. roseum was 5.54 mg/ml and 11.39 mg/ml, respectively. The results indicated that all concentration groups of ethanolic and aqueous extract of A. roseum significantly (P < .05) lowered DNA damage than positive control group. The lowest DNA damage was observed at 0.031 and 0.062 mg/ml in both aqueous and ethanolic extracts. Moreover, at higher concentrations of 4, 8, and 16 mg/ml of both ethanolic and aqueous extract observed 100% DNA damage cells (comet cells). Moreover, Ames mutagenicity showed that no significant (P > .05) changes were observed in revertant colonies of both bacterial strains (T 98; T 100) at 0.25, 0.5, and 1 mg/ml concentrations of both aqueous and ethanolic extract of A. roseum as MI index was less than 2. The research concluded that A. roseum extracts exhibited no cytotoxicity, genotoxicity, and mutagenicity at lower concentrations (≤2 mg/ml).
Introduction
Many chemical and physical agents facilitate human life, but on the other hand, all these agents may cause various health issues due to their genotoxic, cytotoxic, and mutagenic properties. Therefore, medicinal plants have been used as antimutagenic and anticytotoxic agents but unfortunately, these medicinal plants have also some mutagenic and cytotoxic effects on human life as well as other organisms. Various types of medicinal plant extracts have been used previously to overcome the mutagenic and cytotoxic effects of agents such as Ziziphora. clinopodioides Lam., a medicinal plant extensively used as an antibacterial[Citation1,Citation2], antifungal, and antioxidant agent,[Citation3,Citation4] but this plant extract had strong mutagenic and genotoxic effects on human lymphocytes.[Citation5] Limonium mill has also been used as an antibacterial and anti-inflammatory agent but this plant had also highly cytotoxic and mutagenic for various cell lines.[Citation6] Therefore, in this study, we worked on one another medicinal plant to limit these issues.
Argyrolobium roseum (Camb.) is a herbal medicinal plant belonging to the family Fabaceae. It grows in tropical and temperate regions of Pakistan, India, Bangladesh, Nepal, Madagascar, South Africa, and Arabia.[Citation7] A. roseum (Camb.) has been traditionally used to treat hepatitis,[Citation8] jaundice,[Citation9] to relieve stomach and bladder inflammation[Citation10] and various skin diseases such as boils and scabies.[Citation11] Scientifically antihyperglycemic and immune-suppressant effects of this plant had been previously documented.[Citation5]
There are many constituents including flavonoids, furocoumarins, tannins, and anthraquinones in the herbal medicinal plant extracts at various dosage that is potentially teratogenic, mutagenic, and carcinogenic.[Citation12–14] The main challenge which is faced in herbal extract therapy is that no given dosage has been authorized.[Citation12,Citation13,Citation15] The process of genotoxicity, mutagenicity, and cytotoxicity assessment of plant extracts is a compulsory step during its development for human safety in relation to the potential induction of carcinogenesis and hereditary defects.[Citation16] Despite that A. roseum (Camb.) is the wide application in folk medicine and food, but unfortunately, its prejudicial aspects have not been previously documented. Therefore, the purpose of the current study was to investigate the safety profile of A. roseum (Camb.) extracts through in vitro cytotoxicity, genotoxicity, and mutagenicity assessment. In addition, the chemical composition of these plant extracts was characterized as well
Materials and methods
Plant material identification and preparation of extracts
This study was performed in the Department of Pharmacology & Toxicology, and WTO Laboratory, the University of Veterinary and Animal Sciences, Lahore, Pakistan. A. roseum (Camb.) is grown in the vicinity of District Jhelum, Punjab, and was identified by voucher No 3659, deposited at the Herbarium of Department of Botany, Government College University, Lahore, Pakistan. The plant material was washed under running tap water followed by sterile distilled water. Afterward, the aforementioned plant was dried and pulverized to a fine powder using a sterilized domestic grinder (Moulinex, Paris, France). The obtained powder was then used for further analysis. For ethanolic extraction and percolation were performed using 500 mg of crude powder of A. roseum (Camb.) in 95% ethanol. The process was performed at ambient temperature within the percolator for 15 h. Afterward, the solvent was drained and the process was repeated four 4 times to improve the extraction efficacy. The drained-off solvent portion was combined and dried under diminished pressure at 55 ºC. The process was carried out until the final amount of 36 mg ethanolic extract was obtained. For aqueous extraction, 500 g powder material was dissolved in 10 mL of pure and refined water then this solution was centrifuged at 10,000 rpm for 10 min. The solution was filtered by using Whatman filter paper, and the supernatant was drying it in the oven. This extract of A. roseum (Camb) Jaub&Spach was preserved for further use.[Citation10]
Phytochemical analysis of extracts
Total phenolic content (TPC) and total flavonoids content (TFC) of ethanolic and aqueous extract of A. roseum
Using the Folin-Ciocalteu reagent, the phenolic contents of ethanolic and aqueous extracts of A. roseum were determined. After 45 min storage at room temperature, the absorption was estimated at 765 nm and gallic acid was used for phenolic content measurement. According to the findings, the gallic acid equivalent in the samples was expressed as mg per 100 g.[Citation17] Total flavonoid contents in the ethanolic and aqueous extracts of A. roseum were measured using a spectrophotometric method based on the formation of a complex between flavonoids and aluminum. The maximum absorbance of this complex occurs at 430 nm.[Citation17]
Cytotoxicity assays
Materials and chemicals for cell culture
Materials required for the performance of cytotoxicity assay were syringes 5 ml and 10 ml, Petri dishes, cell culture medium M-199, glass filtration assembly, trypsin, DMSO, fetal bovine serum, bicarbonate buffer, gentamicin, streptomycin, amphotericin B, and BHK-21 cell line.
Revival and maintenance of BHK-21 cells
All solutions used in the process of revival were thawed at 37°C. Properly labeled cryovial having BHK-21 cells were removed from the container of liquid nitrogen, and 70% alcohol was used as decontaminant then cells were thawed at 37°C in a water bath by gentle swirling. The cell suspension was shifted to a 20 ml tube, containing 10 ml of cell culture medium supplemented with fetal bovine serum. The suspension of cells was centrifuged for 10 min at room temperature. The supernatant was discarded and after that cells were re-suspended in 5–10 ml of growth medium. Cells were transferred to tissue culture flask and incubated at 37°C for 72 h along with 5% CO2. Cells were monitored daily. As the cells got confluency of 80–90% they were subcultured.
Preparation of stock solution
A. Roseum ethanolic and aqueous fractions were tested for cytotoxicity assay for making stock solution (16 mg/ml) and were further diluted in cell culture media to make two-fold dilutions starting from 16 mg/ml up to 0.0313 mg/ml. These ten dilutions for every sample were tested in triplicate. All test samples were filtered by syringe filters (Sartorius Minisart) of 0.22 µm pore size, for the purpose of avoiding contamination. The whole process was done in a safety cabinet hood.
Cytotoxicity assay
After exposure to BHK-21 cells, all test samples were placed in an incubator at 37°C. After 48 h, cells were treated with MTT dye and incubation was done for 4 h. All the plates were examined under an inverted microscope. BHK-21 cells, cell culture media and DMSO were taken as negative control and for positive control cell line of BHK-21 was used in this part of the trial. Cytotoxicity was examined based on cell survival percentage (CSP) that was calculated by optical density (OD) of test and control samples at 570 nm using an ELISA reader by using the following formula, as describe previously.[Citation5]
Genotoxicity assessment by comet assay
Single-cell gel electrophoresis (SCGE) commonly known as Comet assay has acquired tremendous acceptance and is known as a standard experiment for assessing DNA damage. The principle of this technique is that the damaged DNA was moved away from the undamaged part and resulting in a comet-like image which is why known as comet assay. Fluorescent dye like ethidium bromide was used to visualize the damage under a fluorescent microscope for quantifying DNA damage based on tail formation.
Chemicals required
Sodium Chloride (NaCl), Ethidium Bromide, Trizma Base, Methanol, Dimethylsulfoxide (DMSO), Normal Melting Agarose, Low Melting Agarose, Disodium EDTA, Phosphate Buffer Saline, Sodium Hydroxide (NaOH), Triton X-100, Lymphocyte Separating Medium and Boric acid.
Preparation of slides
All the cavity slides were dipped in alcohol and then blazed over the flame to remove any dust particles or machine oil, etc. Slides were labeled according to test chemical, dipped in hot NMPA and after wiping the underside, got air dried and refrigerated at 4ºC temperature overnight.
Blood was collected from the human vein and poured into two test tubes. Each test tube was filled with 3 ml of blood and 4 ml of Histo-paque media. Media was poured on the side walls of the test tubes. The mixture was centrifuged at 800× for 45 min at 25 ºC. Three layers were formed including plasma at the top while red blood cells at the bottom and the middle layer of lymphocytes called buffy coat. The Buffy coat was separated and mixed in 3–5 ml of RPMI-1640 and centrifuged at 250× for 10 min so that lymphocytes pellet formed. A Pellet of lymphocytes was resuspended in RPMI-1640. Then, electrophoresis buffer was filled in the electrophoresis apparatus and all the slides were set in a way that all were equally distant from the electrodes. The power supply was set to 24 V and the current was set to 300 milliamperes. Electrophoresis was run for 30 min.
Staining and Interpretation of results
Ethidium bromide stain solution already prepared was dropped in 85 µl over cavity slides. After 5 min, slides were placed in chilled water to remove an extra stain. Slides were immediately visualized under the fluorescent microscope for scoring them at 40× objective lenses. Slides not scored immediately were preserved by spraying with 100% methanol. Images of slides were captured by the camera and scored by image J software based on the length of the DNA head and tail. Results were interpreted based on tail length by applying the formula given below
DI stands for damage index, C1 stand for class 1, C2 means class 2 and C3 means class 3. All the undamaged cells were put in C1 and less tail or head equal to tail is placed in C2 and C3 head is 2 times less than the tail
Mutagenicity evaluation of extracts
Chemicals, apparatus, and media required for the assay
All chemicals used in the experiment were of analytical grade. Nutrient broth, Yeast extract, phosphate buffer saline (PBS) NaCl, K2Cr2O7, NaN3, glucose, and histidine were the chemicals required for the test. Equipment required for AMES assay included Autoclave, Incubator, Refrigerator, Laminar flow hood, Hot air oven, Spectrophotometer, and Weighing balance. Syringes (1 mL, 20 mL), Flasks, beakers, Wire loops, Petri dishes, and Screw cap test tubes were used in the experiment. There were the following media used in this assay; Vogel Bonner Salt Solution, Histidine/biotin solution 0.5 mM and LB Broth solution (100 mL).
Bacterial strains
TA98 and TA100 (S. typhimurium) strains were taken from the Biochemistry Department, University of California, Berkeley, USA. Glycerol stocks of TA100 and TA98 strains of S. typhimurium were preserved at −80°C. These strains were refreshed by inoculating 100 μl of bacterial culture into the autoclaved nutrient broth (25 ml) and placed on a shaker incubator overnight at 37°C.
Plate incorporation method for assessment of mutagenicity
The standard plate incorporation method was performed for the mutagenicity test (Maron and Ames 1983). Glycerol stocks of bacterial strains were refreshed in nutrient broth. Culture flasks were placed on a shaker overnight. Top agar was prepared and 2.5 ml was added to each test tube. Test tubes having top agar were autoclaved and allowed to stay in the water bath at 45°C. The solution of biotin and histidine of 0.5 mM was prepared and then filtered with the help of the millex ® syringe. Two-fold serial dilutions of samples were prepared in distilled water. Overnight grown culture pellet was made by centrifuging at 6000 rpm for 7 min at 24°C temp. Washing was done with normal saline and O.D was set to 0.7–0.8 at 599 nm with a spectrophotometer. In the top agar test tube, different concentrations of samples, 200 μl of the histidine-biotin solution, and 100 μl of test strain containing approximately 3 × 107 cells/ml were added. The test tube containing all mixture was mixed by placing it on a vortex machine for 3 s and was poured onto VB minimal medium plates. Plates were set in an incubator at 37°C for 72 h. Distilled (autoclaved) water (100 μl) was used as negative control while sodium azide (10 µg per plate) for TA 100 and potassium dichromate (10 µg per plate) for TA 98 were used as a positive control. The number of revertant colonies was counted and if the revertant colonies were found two folds higher than negative plate then the substance is considered mutagenic. Each experiment was performed in a triplicate manner along with controls and then the mutagenic index was calculated by the following formula:
If the mutagenic index is 2 for the TA98 strain and 1.8 for strain TA100, the substance or tested chemical is considered mutagenic.
Statistical analysis
The data were analyzed by using SPSS version 21. The treatment groups of both extracts were assessed by one-way ANOVA proceeded by Tukey’s test. Significance was assumed when p < .05.
Results
Total phenolic content (TPC) and total flavonoids content (TFC) of ethanolic and aqueous extract of A. roseum
Total phenolic content (TPC) and total flavonoids content (TFC) results are presented in . The results showed that total phenolic contents (TPC) were higher in quantity in aqueous extract of A. roseum (Camb.) than ethanolic extract (538.26 mg vs 15.39 mg GAE/100 g). However, the total flavonoid contents (TFC) were significantly higher in ethanolic extract (6553.29 mg Eq. of Quercetin/100 g) as compared to the aqueous extract of A. roseum (Camb.) (1600.26 mg Eq. of Quercetin/100 g).
Table 1. Determination of phenolic contents (TPC) and total flavonoid content (TFC) of ethanolic and aqueous extract of A. roseum.
Cytotoxicity evaluation of A. roseum (Camb.) extracts
The cytotoxicity potential of ethanolic and aqueous extract of A. roseum (Camb.) was evaluated by MTT assay. The BHK-21 cell line was used to evaluate the cytotoxicity and is presented in . The cells survival percentage was significantly decreased in the groups treated with 0.5–16 mg/ml ethanolic extract of A. roseum compared to negative control (P < .05). However, no significant toxicity was observed in the groups treated with 0.031–0.25 mg/ml of ethanolic extract of A. roseum compared to negative control (P > .05). The results showed that the highest cell survival percentage was 84.71% at the lowest dose (0.031 mg/ml) and more toxicity was observed at 52–42% at 4–16 mg/ml. The IC50 of the ethanolic extract of A. roseum was 5.54 mg/ml.
Figure 1. Cytotoxicity of (a) ethanolic and (b) aqueous extract of A. roseum measured by MTT assay. Columns with different superscript letter (a-d) are significantly different (p < .05). Negative control (DMSO 5%).
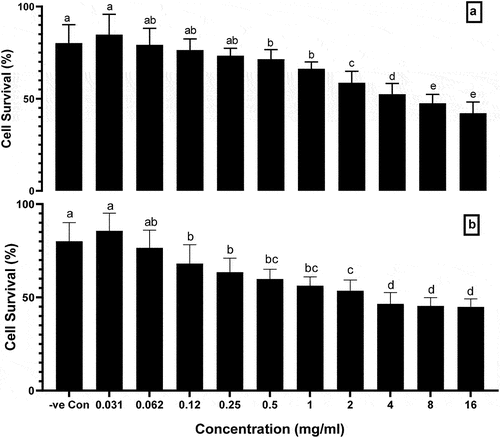
The results showed that the cells survival percentage was at the highest level (85.68%) in the 0.031 mg/ml treated group (P < .05) as compared to 0.12–16 mg/ml treated groups. The cell’s survival was significantly decreased when the BHK-21 cell line was treated with 0.12-16 mg/ml aqueous extract of A. roseum as compared to negative control. The IC50 of the aqueous extract of A. roseum was 11.39 mg/ml, which was higher than the IC50 of the ethanolic extract of A. roseum.
Genotoxicity assessment of A. roseum (Camb.) extracts
The genotoxicity of A. roseum (Camb.) extracts was evaluated by using the comet assay. The affected comet-shaped lymphocyte cells with different concentrations of ethanolic and aqueous extract are shown in . The rating of genotoxicity is based on the head and tail length of DNA damage. Higher the tail length and lower the head length more the DNA damage. The results of the head and tail length of damaged DNA by different concentrations of the ethanolic extract are presented in . The results showed that the highest concentration of ethanolic extract of A. roseum (16 mg/ml) was far lowered DNA tail length as compared to a positive control (1.2 ± 0.35 vs 11.2 ± 0.34 µm respectively; P < .05). The lower concentrations of ethanolic extract (0.031 and 0.062 mg/ml) showed the lowest tail length (0.08 ± 0.001 and 0.12 ± 0.04 µm respectively; P < .0001) with higher head length (3.8 ± 0.43 and 3.5 ± 0.97 µm respectively; P < .0001) as compared to the positive control, which indicated lower DNA damage. Moreover, the results indicated that all concentration groups of ethanolic extract of A. roseum significantly (P < .05) lowered DNA damage than positive control group.
Figure 2. The effect of (a) ethanolic and (b) extract of A. roseum on tail length and head length of human lymphocytes evaluated by comet assay, Columns with different superscript letter (a-f) are significantly different (p < .05). Negative control (DMSO 5%), Positive control (H2O2 200 μM).
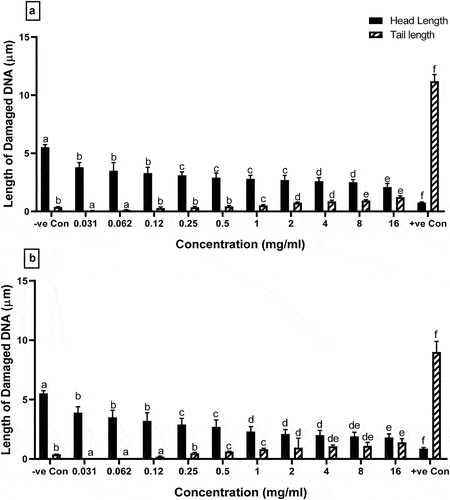
Figure 3. Visual DNA damage human lymphocytes treated with different concentrations of ethanolic extract of A. roseum. (a) 16 mg/ml: (b) 8 mg/ml: (c) 4 mg/ml: (d) 0.061 mg/ml and (e) 0.03 mg/ml.
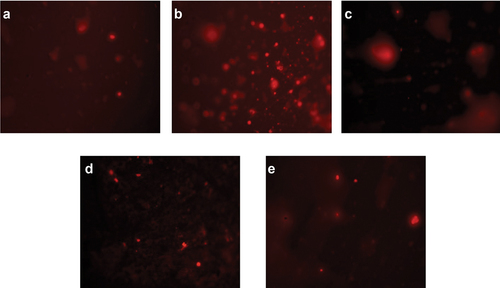
The result of the head and tail length of damaged DNA by aqueous concentrations of A. roseum is presented in . The result showed that all concentrations of aqueous extract of A. roseum significantly (P < .0001) lowered tail length and higher head length as compared to positive control group. The lowest tail length was observed at 0.031, 0.062, and 0.12 mg/ml as compared to a positive control (0.01 ± 0.0001, 0.02 ± 0.09, and 0.19 ± 0.07 vs 9 ± 0.34 µm, respectively; P < .0001), which indicated lower DNA damage than a control group.
Figure 4. Visual DNA damage human lymphocytes treated with different concentrations of aqueous extract of A. roseum. (a) 16 mg/ml: (b) 8 mg/ml: (c) 4 mg/ml: (d) 0.061 mg/ml and (e) 0.03 mg/ml.
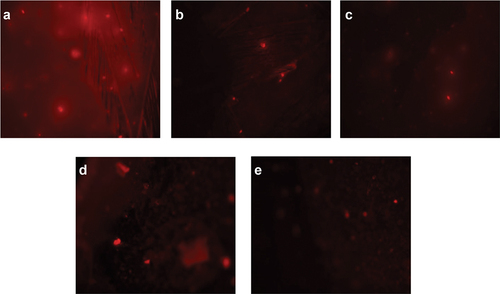
The affected comet-shaped cells were divided into four classes from undamaged to higher damaged, as shown in . The ratio of comet cells was increased from 8% to 100% as the concentrations of both ethanolic and aqueous extract increasing 0.031 to 16 mg/ml, respectively. Moreover, at higher concentrations of 4, 8, and 16 mg/ml of both ethanolic and aqueous extract observed 100% DNA damage cells (comet cells). The GDI value was calculated for all concentrations of both ethanolic and aqueous extracts of A. roseum and presented in . The GDI value of all the concentrations of both ethanolic and aqueous extract was greater than the negative control (0.08) and lowered than the positive control (2.12).
Table 2. Distribution of cell count (%) with different comet grades induced by treatment with different concentrations of ethanolic and aqueous extract of A. roseum evaluated by comet assay.
Mutagenicity assessment
The mutagenicity of the extracts of A. roseum was assessed by using the Ames assay, and its results are presented in . The results of the Ames mutagenicity showed that no significant (P > .05) changes were observed in the revertants colonies of both bacterial strains (T 98 and T 100) at 0.25, 0.5, and 1 mg/ml concentrations of the ethanolic extract of A. roseum as the MI index was less than 2 in all concentrations. However, significant changes were observed in revertant colonies of T 98 and T 100 bacterial strains at 2–8 mg/ml concentrations as its MI index was more than 2, which indicated mutagenic potential at specific concentrations.
Table 3. The number (mean ± SD) of revertant colonies and mutagenicity index (MI) of S. typhimurium TA98 and TA100 exposed to ethanolic and aqueous extract of A. roseum.
The results showed that for aqueous extract, there was no statistically significant increase in the revertant colonies in both strains of bacteria at 0.25–4 mg/ml concentrations, which indicated no potential mutagenic effect. However, at the highest concentration (8 mg/ml) significant (P < .01) increase was observed in both strains than the control group and its MI index was higher than 2.
Discussion
Nowadays, in the modern world, the major concerns are about the packaging materials of food because it is a possible factor for the migration of constituents into the food.[Citation18] Therefore, many researchers worked on medicinal plants to overcome the constituents of packaging materials.[Citation8,Citation19] Natural essential oils or other products, such as additives, have antimicrobial, antifungal, and antioxidant properties.[Citation5,Citation15] But, previous reports showed mutagenicity and carcinogenicity of some plants and essential oils.[Citation13,Citation20] For this reason, the detection of gene mutation in bacteria is the core of toxicological tests for the assessment of materials used in food safety.[Citation21] To prove that some of these products have a mutagenic effect on in-vitro systems, several laboratory studies have been conducted.[Citation5,Citation8,Citation22]
In the current study, the phytochemical composition of A. roseum extracts was analyzed. The phytochemical analysis showed that total phenolic contents were higher in aqueous extract and total flavonoid contents were higher in ethanolic extract. In literature, the lipophilic flavonoid contents were the major components in Z. clinopodiodes extracts.[Citation1,Citation2] In another report, phenolic acid and derivatives of benzyl alcohol were the major contents of Limonium globuliferum aqueous extracts and Ziziphora clinopodioides Lam. ethanolic extract.[Citation5,Citation6] Due to high phenolic and flavonoid contents, the extract of A. roseum (Camb.) Jaub&Spach can be used as a medicine for hepatitis and jaundice.[Citation23]
In the literature review, many scientists worked on various medicinal plant extracts regarding the determination of cytotoxicity and genotoxicity. Ghazanfari et al. determined cytotoxicity of Z. clinopodioides aqueous extract by the MTT test and found 88% cytotoxic effect at 5 mg/ml after 72-h exposure to gastric cancerous cell line [Citation24]. Another researcher worked on an ethanolic extract of an Iranian medicinal plant with and slight cytotoxic effect on cell lines.[Citation24] Furthermore,[Citation3,Citation25] Sharifzadeh et al. and Vijayarathna et al. found the cytotoxic effect of Bangladeshi medicinal plant extracts on pancreatic cancer cells. Although previous reports showed a toxic effect of medicinal plants on cell lines, this is the first study that determined the cytotoxicity of A. roseum (Camb.) extracts by using an MTT assay. In the present study, lymphocyte treatment was done by various aqueous and ethanolic extracts of A. roseum (Camb.). The MTT results indicated that the cell survival rate was significantly higher in both aqueous and ethanolic extracts than in control, which showed no toxicity of extract on BHK-21 cell lines. However, Z. clinopodioides extracts showed a highly toxic effect on lymphocytes at a concentration of 10 mg/ml on MTT assay.[Citation5,Citation26] Furthermore,[Citation27] Yousefbeyk et al. observed that n-hexane of Z. clinopodoides induced acute toxicity effects on T-47D and K-562 cell lines. The difference in cytotoxicity between the current study with previous reports may be the concentrations of phenolic and flavonoid contents.[Citation12,Citation13] Another possible reason for the cytotoxicity difference is the presence of metals in medicinal plants.[Citation16,Citation28] It was determined that Limonium species contain copper (Cu), zinc (Zn), manganese (Mn), chromium (Cr), and iron (Fe) (Eren and Özata 2014). So, their toxic effects may be related to these elements. In one report, the toxicity could be affiliated with a myriad of parameters and concentrations.[Citation20,Citation29] Additionally, acacetin, diosmetin, and daucosterol induced cytotoxicity in different cancer cell lines,[Citation29,Citation30] these may be low in the extracts of A. roseum (Camb.). According to Yousefbeyk et al.,[Citation27] naphthoquinone induced toxicity due to the formation of reactive oxygen species, mitochondrial disorder, and inhibition of thymine binding to DNA.
The detection of genotoxicity of medicinal plant extract is the critical step and one of the most important steps due to its relevancy to human safety via induction of carcinogenesis and hereditary defects.[Citation9,Citation22] Therefore, in the present study, genotoxicity of A. roseum (Camb.) extracts was analyzed using comet assays because of their high sensitivity, cell quantity demand is low and the simplest protocol assay, which has been applied extensively for the assessment of genomic lesions toxicity.[Citation21] But unfortunately, no such type of study reported on A. roseum (Camb.). The results of the current study showed that dose-dependent genotoxicity was present following the various concentrations of A. roseum (Camb.) extracts exposure; the genotoxicity via DNA damage had higher at a concentration higher than 1 mg/ml and the 100% comet cells were damaged at a concentration of 4–16 mg/ml with highest GDI. The results indicated that A. roseum (Camb.) had mild genotoxicity potency at higher concentrations, however no genotoxicity at lower concentrations. When our results were compared with previous literature, the carvacrol and thymol induced DNA damage at a very low concentration (0.05–0.2 mM).[Citation4,Citation31] In another study, these plant compounds induced DNA strand break of lymphocytes and hepatocytes in rats by feeding 20 mg/kg.[Citation24] Furthermore,[Citation5] Ahmadi et al. found that Ziziphora clinopodioides Lam. ethanolic extract induced genotoxicity at a concentration of 1 mg/ml or higher and concluded strong genotoxicity. All researchers found similar results as in our study that below the DNA damaging concentrations, the major compounds and constituents of extracts protected lymphocytes against DNA damage. In one study, the scientist found that galactosamine in the herbal extract showed a protective effect due to free radical scavenging.[Citation7] Furtado et al. found that flavonoid contents possess in-vitro antioxidant properties, therefore the A. roseum (Camb.) extracts possess low genotoxicity than other medicinal plant extracts[Citation21]. However, in contrast to this, we found that flavonoids have pro-oxidants in-vivo and result from damaged DNA via oxidative stress. In previous reports, DNA is damaged due to the production of a high quantity of ROS or other damaging agents.[Citation4] In contrast to our findings, a significant increase in oxidative DNA breaks has been previously reported in various medicinal plants extract.[Citation4,Citation11] One reason for DNA damage in comet cells is elevated production of H2O2 level, as previously reported that quercetin breaks DNA strands by overproduction of hydroxyl radicals.[Citation19]
To evaluate the mutagenicity of A. roseum (Camb.) extracts in this study, S. typhimurium strains TA98 and TA100 were used in the Ames test. The results of the Ames mutagenicity showed that no changes were observed in the revertants colonies of both bacterial strains (T 98 and T 100) at 0.25, 0.5, and 1 mg/ml concentrations of the ethanolic and aqueous extract of A. roseum as the MI index was less than 2 in all concentrations, which indicated as antimutagenic. The possible reason for the antimutagenic properties of A. roseum (Camb.) extracts is flavonoid contents in the extracts, as previously reported in other plants.[Citation1,Citation2] However, according to[Citation13] and[Citation5] some medicinal plant extracts and essential oils at high dose have been pro-mutagenic or direct mutagenic. Similarly, in the current study, significant changes were observed in revertant colonies of T 98 and T 100 bacterial strains at 2–8 mg/ml concentrations as its MI index was more than 2, which indicated mutagenic potential at specific concentrations or dose-dependent.[Citation3]
Conclusion
Finally, A. roseum extracts exhibited no cytotoxicity, genotoxicity, and mutagenicity at lower concentrations (≤2 mg/ml). However, at higher concentrations they exhibited cytotoxicity, genotoxicity, and mutagenicity in lymphocytes. So this fact suggested that A. roseum extracts application be done under the provision because usage of this medicinal plant extract is a challenge and is not clear among people using. Therefore, for determination of an acceptable dose of A. roseum extracts required an animal model study and further research is needed to investigate the concentration in blood, also performing other techniques and bacterial strains. Therefore, comprehensive risk assessments are needed for these plant extracts.
Acknowledgments
This research was funded by Princess Nourah bint Abdulrahman University Researchers Supporting Project number (PNURSP2023R110), Princess Nourah bint Abdulrahman University, Riyadh, Saudi Arabia
Disclosure statement
No potential conflict of interest was reported by the author(s).
Additional information
Funding
References
- Mohammadhosseini, M. The Ethnobotanical, Phytochemical and Pharmacological Properties and Medicinal Applications of Essential Oils and Extracts of Different Ziziphora Species. Ind. Crops Prod. 2017, 105, 164–192. DOI: 10.1016/j.indcrop.2017.05.009.
- Šmejkal, K.; Malaník, M.; Zhaparkulova, K.; Sakipova, Z.; Ibragimova, L.; Ibadullaeva, G.; Žemlička, M. Kazakh Ziziphora Species as Sources of Bioactive Substances. Molecules. 2016, 21(7), 826. DOI: 10.3390/molecules21070826.
- Sharifzadeh, A.; Javan, A. J.; Shokri, H.; Abbaszadeh, S.; Keykhosravy, K. Evaluation of Antioxidant and Antifungal Properties of the Traditional Plants Against Foodborne Fungal Pathogens. Journal de Mycologie Médicale. 2016, 26(1), e11–e17. DOI: 10.1016/j.mycmed.2015.11.002.
- Shokri, H.; Sharifzadeh, A.; Tamai, I. A. Anti-Candida Zeylanoides Activity of Some Iranian Plants Used in Traditional Medicine. Journal de mycologie médicale. 2012, 22(3), 211–216. DOI: 10.1016/j.mycmed.2012.04.006.
- Ahmadi, A.; Gandomi, H.; Derakhshandeh, A.; Misaghi, A.; Noori, N. Phytochemical Composition and in vitro Safety Evaluation of Ziziphora Clinopodioides Lam. Ethanolic Extract: Cytotoxicity, Genotoxicity and Mutagenicity Assessment. J. Ethnopharmacol. 2021, 266, 113428. DOI: 10.1016/j.jep.2020.113428.
- Eren, Y.; Özata, A. Determination of Mutagenic and Cytotoxic Effects of Limonium Globuliferum Aqueous Extracts by Allium, Ames, and MTT Tests. Rev. Bras. Farmacogn. 2014, 24, 51–59. DOI: 10.1590/0102-695X20142413322.
- Sharma, N.; Verma, M. K.; Gupta, D. K.; Satti, N. K.; Khajuria, R. K. Isolation and Quantification of Pinitol in Argyrolobium Roseum Plant, by 1H-NMR. J. Saudi Chem. Soc. 2016, 20(1), 81–87. DOI: 10.1016/j.jscs.2014.07.002.
- Abbas, M.; Ali, A.; Arshad, M.; Atta, A.; Mehmood, Z.; Tahir, I. M.; Iqbal, M. Mutagenicity, Cytotoxic and Antioxidant Activities of Ricinus Communis Different Parts. Chem. Cent. J. 2018, 12(1), 1–9. DOI: 10.1186/s13065-018-0370-0.
- Obafemi, T. O.; Onasanya, A.; Adeoye, A.; Falode, J. A.; Daniel, D. J.; Irefo, E. F.; Ojo, A. O.; Fadaka, A.; Afolabi, O. B.; Awe, J. O. Protective Effect of Methanolic and Flavonoid-Rich Leaf Extracts of Synsepalum Dulcificum (Danielli) on Lead-Acetate-Induced Toxicity in Wistar Albino Rats. J. Appl. Pharm. Sci. 2019, 9(5), 065–072.
- Chauhan, P. S.; Gupta, K. K.; Bani, S. The Immunosuppressive Effects of Agyrolobium Roseum and Pinitol in Experimental Animals. Int. Immunopharmacol. 2011, 11(2), 286–291. DOI: 10.1016/j.intimp.2010.11.028.
- Ahmad, A.; Husain, A.; Mujeeb, M.; Khan, S. A.; Najmi, A. K.; Siddique, N. A.; Damanhouri, Z. A.; Anwar, F. A Review on Therapeutic Potential of Nigella sativa: A Miracle Herb. Asian Pac. J. Trop. Biomed. 2013, 3(5), 337–352. DOI: 10.1016/S2221-1691(13)60075-1.
- De Wet, H.; Ramulondi, M.; Ngcobo, Z. The Use of Indigenous Medicine for the Treatment of Hypertension by a Rural Community in Northern Maputaland, South Africa. South African J. Of Bot. 2016, 103, 78–88. DOI: 10.1016/j.sajb.2015.08.011.
- Kharchoufa, L.; Merrouni, I. A.; Yamani, A.; Elachouri, M. Profile on Medicinal Plants Used by the People of North Eastern Morocco: Toxicity Concerns. Toxicon. 2018, 154, 90–113. DOI: 10.1016/j.toxicon.2018.09.003.
- Mounanga, M. B.; Mewono, L.; Angone, S. A. Toxicity Studies of Medicinal Plants Used in Sub-Saharan Africa. J. Ethnopharmacol. 2015, 174, 618–627. DOI: 10.1016/j.jep.2015.06.005.
- Elisha, I. L.; Botha, F. S.; McGaw, L. J.; Eloff, J. N. The Antibacterial Activity of Extracts of Nine Plant Species with Good Activity Against Escherichia coli Against Five Other Bacteria and Cytotoxicity of Extracts. BMC Complementary Altern. Med. 2017, 17(1), 1–10. DOI: 10.1186/s12906-017-1645-z.
- Bose, S.; Datta, R.; Kirlin, W. G. Toxicity Studies Related to Medicinal Plants. In Evidence Based Validation of Traditional Medicines; Springer: Singapore, 2021; pp. 621–647. DOI: 10.1007/978-981-15-8127-4_30.
- Mahboubi, M.; Kazempour, N.; Nazar, A. R. B. Total Phenolic, Total Flavonoids, Antioxidant and Antimicrobial Activities of Scrophularia Striata Boiss Extracts. Jundishapur J. Nat. Pharm. Prod. 2013, 8(1), 15–19. DOI: 10.17795/jjnpp-7621.
- Metsämuuronen, S.; Sillanpää, M.; Bhatnagar, A.; Mänttäri, M. Natural Organic Matter Removal from Drinking Water by Membrane Technology. Sep. Purif. Rev. 2014, 43(1), 1–61. DOI: 10.1080/15422119.2012.712080.
- Asif, M. Chemistry and Antioxidant Activity of Plants Containing Some Phenolic Compounds. Chem. Int. 2015, 1(1), 35–52.
- LLana-Ruiz-Cabello, M.; Maisanaba, S.; Puerto, M.; Prieto, A. I.; Pichardo, S.; Jos, Á.; Cameán, A. M. Evaluation of the Mutagenicity and Genotoxic Potential of Carvacrol and Thymol Using the Ames Salmonella Test and Alkaline, Endo III-And FPG-Modified Comet Assays with the Human Cell Line Caco-2. Food Chem. Toxicol. 2014, 72, 122–128. DOI: 10.1016/j.fct.2014.07.013.
- Furtado, R. A.; de Oliveira, P. F.; Senedese, J. M.; Ozelin, S. D.; de Souza, L. D. R.; Leandro, L. F.; de Oliveira, W. L.; da Silva, J. J. M.; Oliveira, L. C.; Rogez, H. Assessment of Genotoxic Activity of Oleoresins and Leaves Extracts of Six Copaifera Species for Prediction of Potential Human Risks. J. Ethnopharmacol. 2018, 221, 119–125. DOI: 10.1016/j.jep.2018.04.002.
- Elachouri, M. Ethnobotany/ethnopharmacology, and bioprospecting: Issues on knowledge and uses of medicinal plants by Moroccan people. In natural products and drug discovery; Elsevier, 2018; 105–118. DOI: 10.1016/B978-0-08-102081-4.00005-8.
- Abbasi, A. M.; Khan, M. A.; Ahmad, M.; Zafar, M.; Khan, H.; Muhammad, N.; Sultana, S. Medicinal Plants Used for the Treatment of Jaundice and Hepatitis Based on Socio-Economic Documentation. Afr. J. Biotechnol. 2009, 8(8), 1643–1650.
- Asadi-Samani, M.; Rafieian-Kopaei, M.; Lorigooini, Z.; Shirzad, H. A Screening of Growth Inhibitory Activity of Iranian Medicinal Plants on Prostate Cancer Cell Lines. BioMedicine. 2018, 8(2), 8. DOI: 10.1051/bmdcn/2018080208.
- Vijayarathna, S.; Sasidharan, S. Cytotoxicity of Methanol Extracts of Elaeis Guineensis on MCF-7 and Vero Cell Lines. Asian Pac. J. Trop. Biomed. 2012, 2(10), 826–829. DOI: 10.1016/S2221-1691(12)60237-8.
- Božović, M.; Ragno, R. Calamintha Nepeta (L.) Savi and Its Main Essential Oil Constituent Pulegone: Biological Activities and Chemistry. Molecules. 2017, 22(2), 290. DOI: 10.3390/molecules22020290.
- Yousefbeyk, F.; Tabaside, J.; Ostad, S.; Salehi Sourmaghi, M.; Amin, G. Investigation of Chemical Composition and Cytotoxic Activity of Aerial Parts of Ziziphora Clinopodioides. Lam. Research Journal Of Pharmacognosy. 2016, 3(2), 47–51.
- Ndhlala, A. R.; Ncube, B.; Okem, A.; Mulaudzi, R. B.; Van Staden, J. Toxicology of Some Important Medicinal Plants in Southern Africa. Food Chem. Toxicol. 2013, 62, 609–621. DOI: 10.1016/j.fct.2013.09.027.
- Kim, C.-D.; Cha, J.-D.; Li, S.; Cha, I.-H. The Mechanism of Acacetin-Induced Apoptosis on Oral Squamous Cell Carcinoma. Arch. Oral Biol. 2015, 60(9), 1283–1298. DOI: 10.1016/j.archoralbio.2015.05.009.
- Ghazanfari, T.; Yaraee, R.; Shams, J.; Rahmati, B.; Radjabian, T.; Hakimzadeh, H. Cytotoxic Effect of Four Herbal Medicines on Gastric Cancer (AGS) Cell Line. Food Agric. Immunol. 2013, 24(1), 1–7. DOI: 10.1080/09540105.2011.637549.
- Piloto Ferrer, J.; Cozzi, R.; Cornetta, T.; Stano, P.; Fiore, M.; Degrassi, F.; De Salvia, R.; Remigio, A.; Francisco, M.; Quiñones, O., et al. Xanthium strumarium L. Extracts Produce DNA Damage Mediated by Cytotoxicity in in vitro Assays but Does Not Induce Micronucleus in Mice. Biomed Res. Int. 2014, 2014, 1–8. DOI: 10.1155/2014/575197.