ABSTRACT
In the current study, wheat varieties Inqulab-91 and AS-2002 were milled from a roller mill and analyzed for chemical characteristics through Near Infra-Red spectroscopy. Friabilin was extracted with Triton X-114 buffer and evaluated on sodium dodecyl sulfate polyacrylamide gel electrophoresis against known molecular weight protein standards. Current results show that the chemical constituents and friabilin revealed significant variation and uneven distribution among flour streams. In both wheat varieties, break streams showed higher protein, moisture, gluten, Zeleny and ash values. Inqualb-91 break streams had a higher lipid content than AS-2002 flour streams, but no clear trend was identified. The 15 kDa band was absent in M3, T2, sizing and low-grade flour of Inqulab-91 while the faint band was present in the remaining streams. Among the remaining AS-2002 mill streams, B2, B4, M3, M5, T1, and sizing showed a prominent 15 kDa band, while the rest showed a faint band. Friabilin depicted a positive correlation with all constituents except fat in AS-2002 while a negative correlation with gluten, Zeleny and fat contents for Inqulab-91.
Introduction
Wheat is the world’s principal cereal grain in terms of production, consumption and trade. It plays a significant role in global food security. Pakistan is one of the leading wheat-producing countries in the world with a 2% contribution to production.[Citation1] Grain texture is an imperative character in assessing wheat quality and one of the most significant physiochemical traits universally accepted in the wheat trade.[Citation2,Citation3] Kernel hardness refers to whether the grain is physically hard or soft and is a quantitative measurement of the force resisting grain deformation.[Citation4] Hardness is a grading factor for the differentiation of hard and soft wheat, determines milling characteristics and defines end-product quality.[Citation5,Citation6] It affects milling yield, damaged starch and baking characteristics.[Citation7] The main factor determining grain hardness is the presence of an endosperm-specific friabilin (15 kDa) protein associated with the surface of starch granules. It was named friabilin because soft wheat are more friable than harder ones.[Citation8] Friabilin protein is a biochemical marker determining grain texture and its quantitative level depicts inverse relation with kernel softness.[Citation8,Citation9] Friabilin acts as a softening agent by inhibiting the strong adhesion between starch and protein matrix.[Citation5]
Kernel hardness is a decisive factor for milling quality.[Citation4] Starchy endosperm in wheat grain is comprised of various cell types differing in composition as well as size. Sub-aleurone tissues are rich in protein and contain fewer irregularly shaped starch granules than cells of the starchy endosperm. Milling separates kernel components with maximum extraction of white flour having desired quality as the ultimate objective and enhancing the palatability of wheat grain.[Citation10] It involves a series of different operations, i.e., cleaning, conditioning, size reduction and separation. Size reduction facilitates the enzymatic and cooking processes whereas separation reduces the level of indigestible bran and removes high oil germ to make the flour shelf stable.[Citation11,Citation12] The quality of grain received at the mill is not consistent all the time owing to variable climatic conditions during growth. In a country like Pakistan, wheat grain is not traded as an individual cultivar but marketed as a mixture. In such a situation, millers use stream splitting process in roller flour milling as an option to produce diversified flours specified for each bakery product from a single grain lot due to variations in chemistry, rheology and functionality of flours based on its origin from different portions of the grain. Different baked items require flour individually suited for each product. Numerous important nutrients are lost during the milling process to obtain white flour. So, mixing of certain flour fractions produced at different mill stages is necessary to comply with nutritional, qualitative and economic constraints. Stream blending ensures the supply of standardized flours having consistent quality with relatively constant protein and ash contents from fractions with inferior and superior quality best suited for the desired baked product in subsequent processing.[Citation12,Citation13] Technical know-how about the quality and rheological attributes of millstreams is therefore necessary for efficient commercial milling, high-quality streams with more yield, precise selection, and monitoring of streams with quality attributes suitable for the desired final product to satisfy consumer demand.[Citation14–16]
Near Infra-Red Spectroscopy (NIRS) is a rapid quality control tool in the modern wheat milling industry for the measurement of the main constituents of grain-like moisture, protein, fat, starch and cellulose. Time and cost saving, no sample preparation and eco-friendly due to no chemical use make it a versatile tool and equally effective for all stakeholders, i.e. plant breeders, food technologists, traders and processors in wheat production and trade.[Citation17] Miralbes[Citation18] used it to estimate protein and moisture in both whole wheat and flour. NIR technique envisages functionality based on the relationship between different physicochemical attributes and some chemical compounds, especially moisture, protein, starch and oil in cereal grains. Different researchers standardized NIR for estimating whole wheat and flour protein,[Citation19] gliadin and glutenin proteins[Citation20] and ash content.[Citation21] Online quality monitoring of flour mill streams with NIRS improves mill performance.[Citation22]
Millers produce diversified flours for different baked products, and each type has its specifications. Quality regulation by standard tests is much slow, arduous, expensive and requires chemicals.[Citation23] The flour industry thus demands rapid and accurate analytical techniques requiring less amount of samples for measuring different chemical and physical attributes easily performed daily in cost-effective and well in time to meet these specifications. NIR is extensively used due to its ease of operation, output, precise and objective nature in analyzing cereal grains.[Citation18] It is nondestructive and requires no or minimum sample preparations and is used for the authenticity and traceability of cereals.[Citation23] Its benefits include the analysis of many samples due to the rapid nature of the technique, the prediction of several parameters from a single attempt and the small sample size.[Citation18] Results are based on absorption responses due to molecular vibrations by overtone and combination frequencies by functional groups like O-H, C-H, N-H and S-H and variability in bonding energy upon exposure to the infrared rays.[Citation17]
Information about the precise relationship between grain hardness and the content of friabilin protein on the surface of starch granules of endosperm in different mill streams is valuable for wheat millers and bakers. Parameters of the mill stream, particularly protein, color, ash and lipid contents, starch damage, distribution of enzymes and glutenin proteins have previously been explored by Yahata et al., [24]. However, the presence and distribution of starch granule-specific protein (friabilin) in mill streams has not yet been studied. The present study was therefore undertaken to explore the biochemical markers for grain hardness at the molecular level and the distribution of different chemical constituents in mill streams.
Materials and methods
Procurement and milling of wheat varieties
Grains from two different wheat varieties, Inqulab-91 (hard wheat) and AS-2002 (soft wheat), were obtained from the Wheat Research Institute in Faisalabad, Pakistan. The wheat grains were subjected to a tempering process according to the method specified by the AACC[Citation24] in method No. 26–95. The tempered wheat grains were then milled using a Roller Flour Mill manufactured by Moore Machine Manufacturer, Inc. in Wichita, Kansas. The milling process resulted in the production of different break and reduction flour streams, following the procedure described by Iqbal et al.[Citation11] in a previous study. To obtain the first break stream (B1), the wheat variety was passed through the first roll of the mill with a gap of 0.25 inches between the rolls. The milled wheat was then sifted using a gyrating sifter manufactured by Great Western Co. in Leavenworth, KS, USA. The sifting process was carried out for 2 min, using a sequence of sieves including 20W (with 1041 µm openings), 50GG (with 375 µm openings), 70GG (with 240 µm openings), 10XX (with 136 µm openings), and a pan sieve for particles smaller than 136 µm. This methodology was adapted from the work of Pasikatan et al.[Citation25] providing a standardized and reproducible procedure for obtaining different flour streams from the milled wheat.
The material that remained on sieve 20W was subjected to milling by the first set of rollers with a nip adjustment of 0.12 inches. This milling process resulted in the production of the second break flour (B2), which was then sieved using the same procedure mentioned earlier. The material collected from sieve 20W of the first break roll, with a nip adjustment of 0.12 inches, was transferred to the second set of break rolls. The gap between these rolls was adjusted to 0.06 inches. The material was then sifted as described previously to collect the third break fraction (B3). Similarly, the fourth break stream (B4) was obtained by adjusting the gap between the rolls to 0.003 inches (as shown in ). It is important to note that all the reduction streams were milled using smooth rolls with a nip adjustment of 0.003 inches. Fractions of wheat retained on 50GG from each break roll were combined to get sizing, which was milled from reduction rolls and sifted with the same arrangement of sieves as in all break and first reduction fraction.[Citation11]
Chemical analysis of wheat flour mill streams
Protein content (39–11.01), fat content (39–20), moisture content, ash content (08–21), gluten content and Zelney value of mill stream flours were determined following the AACC[Citation24] NIR method on Infratec 1241 Grain Analyzer (Foss Tecator, Denmark) having flour module with small ring cup cells. NIR transmittance spectra of the flour were recorded at 850 to 1048.2 nm after calibration AACC 2010[Citation24] method 39–00).
Friabilin extraction and SDS-PAGE
The friabilin proteins were isolated from wheat flour with the Triton X-114 buffer, analyzed on SDS-PAGE using Coomassie brilliant blue for band fixation and image was documented following Pasha et al.[Citation26]
Statistical analysis
The data obtained for each parameter was subjected to statistical analysis by using completely randomized design and mean values thus obtained were compared following Tuckey’s Honestly Significant Difference test as described by Montgomery.[Citation27]
Results and discussion
Chemical analysis of mill streams of wheat varieties
Protein content
The effect of different mill streams was found to be highly significant on protein content. Means for protein content varied from 8.6% to 16.0% and 10.57% to 16.30%, respectively, among different flour fractions of AS-2002 and Inqulab-91 (). Highest protein content was recorded in B4 in both wheat varieties while lowest in M2 and sizing, respectively, for millstreams of AS-2002 and Inqulab-91.
Table 1. NIR protein and moisture content of mill streams.
The protein content in break flour streams is generally higher compared to reduction flour. Among the break streams, the first break flour had the lowest protein content, while the fourth break flour had the highest protein content. In terms of reduction flours, M3 had the highest protein content among both types of wheat studied (Inqulab-91 and AS 2002), while the lowest protein content was found in sizing and M2 for Inqulab-91 and AS 2002, respectively. In the break streams, there was a gradual increase in protein content from the first to the fourth break. However, there was no such clear trend observed in the reduction streams. Overall, the protein content increased with the number of break or reduction passages, indicating that successive breaks or reductions led to higher protein content in the resulting flours.
The endosperm of wheat grain is not homogenous in composition, and there exist gradients in the level of different constituents from outer to inner layers.[Citation10] This uneven distribution in the content and chemistry of endosperm tissues results in disparity in the composition of milled flour fractions as these receive a different proportion of endosperm layers during grinding and sifting operations. The protein content of millstreams in the present study is in line with Yahata et al.[Citation28] recorded protein content from 9.24% to 18.98% in different mill streams. Protein content varied between 7.86% and 18.08% in different flour samples analyzed from NIR.[Citation18] whereas Dornez et al.[Citation29] found protein content range from 11.10% to 16.80%, 9.80% to 11.10% and 10.60% to 12.60% among different streams of break, coarse reduction and fine reduction flours, respectively. Protein content gradually increased in quantity from the first to last break streams which are analogues to the results for protein in millstreams by Gomez et al.,[Citation30] Sakhare et al.,[Citation31] and Abo-Dief et al.,[Citation13] which might be due to increasing concentration of peripheral endosperm portion from ventral and dorsal ends rich in protein. Among the break streams, B1 had the minimum protein content whereas the maximum in B4. Reduction streams exhibited less protein content than break streams and no particular trend was observed in the reduction streams. This quantitative trend is in similarity with Iqbal et al.[Citation11] who reported a higher mean for protein in break stream blend as compared to middling and sizing blends.
Moisture content
The moisture content of different mill streams was found to be highly significantly influenced by differences among flour fractions. The moisture content of different flour fractions ranged from 11.60 to 13.50% and 11.60% to 13.90% among different flour fractions of AS-2002 and Inqulab-91, respectively (). The highest moisture content was observed in B3 in both wheat varieties, while the lowest value was found in T1 and T2 for mill streams of AS-2002 and Inqulab-91, respectively. Among different flour fractions, Inqulab-91 moisture content was highest in break streams than in reduction fractions. A declining trend for moisture content was observed in break streams and reduction flour streams. Among break streams, B3 exhibited maximum mean for moisture content while minimum in B4 in both wheat varieties. Among different reduction streams, highest moisture content was observed in M3 while lowest in T1 and T2 for both wheat varieties.
Higher score for moisture among break streams is attributed to more gap and less grinding in break rolls leading to less evaporation owing to lower temperature than reduction streams, and these results are in similarity to results reported by Abo-Dief et al.[Citation13] and El-Porai et al.[Citation32] Iqbal et al.[Citation11] found similar moisture content (12.80 to 13.60%) in milled flour blends with the highest in break stream blend and minimum in sizing blend. Mirables[Citation18] reported 9% to 16.48% moisture content in different flour streams analyzed from NIR. Gomez et al.[Citation30] reported lower moisture in reduction streams than in break streams due to higher separation in break rolls and more heating in reduction rolls. However, Abo-Dief et al.[Citation13] and Sakhare et al.[Citation31] evidenced decreasing moisture among both break and reduction flour streams owing to pressure by grinding rollers and its evaporation in pneumatic transit of ground grains.
Gluten content
Mill streams exerted a highly significant impact on gluten content. Gluten content varied from 26.0% to 40.0% and 24% to 40% among different flour fractions of Inqulab-91 and AS-2002 millstreams (), respectively. The fourth break stream (B4) showed maximum mean for gluten content in both wheat varieties while lowest in M2 and T1 and M5 and LG in different fractions, respectively, for Inqulab-91 and AS-2002 (). Break flour streams exhibited higher gluten content than reduction streams. An increasing trend for gluten content was observed in break streams while no such trend in reduction streams. The highest mean value for gluten content was observed in B4 while lowest in B1 in break streams of both wheat varieties. Among reduction streams, maximum gluten content was observed in M3 in both varieties while minimum in LG in AS-2002 and M2 in Inqulab-91.
Table 2. NIR gluten and ash content of mill streams.
Higher content of gluten in break flour streams than reduction ones and progressive increment in gluten among break streams in current research agrees with findings by Abo-Dief et al.[Citation13] which might be attributed to dominating proportion of gluten-forming proteins in them. Iqbal et al.[Citation11] recorded the highest NIR gluten content in both AS-2002 and Inqulab-91 break blends (33%) while lowest in AS-2002 middling and sizing blends (25%) which also endorse the results of current studies. In concordance with current study results, Sakhare et al.[Citation31] revealed a rising trend in dry gluten from the first to 4th break streams. Higher gluten in M3 and M1 while lower in M2 and M5 is contrary to Sakhare et al.[Citation31] and Banu et al.[Citation33] which might be due to different milling systems, capacity of mills used in the present study (roller) and (Buhler) previous studies and probably more proportion of peripheral grain layers. Gluten content decreased in tail-end reduction streams. NIR gluten content in the current study is somewhat higher than Mirables[Citation18] who recorded 17.7–36.76% which might be due to a difference in genetic makeup or environmental and milling conditions. The quantity of gluten decreases whereas the content of glutenin polypeptide increases from the outer to inner layers of endosperm tissues.[Citation10] Brütsch et al. [34] revealed that the elevated protein level in break streams correlated with higher wet gluten and lower gluten in tail-end reduction streams higher in ash due to weakening gluten network by interference from bran which is quite supportive of the present findings.
Ash content
The results showed that the effect of mill streams was highly significant on ash content in both wheat varieties. Ash content varied from 0.23 to 0.70% and 0.25 to 0.98%, respectively, among different milled fractions of Inqulab-91 and AS-2002 (). The maximum mean for ash content was recorded for B1 in both kinds of wheat while lowest in M2 and T2 for wheat variety Inqulab-91 and AS-2002, respectively. Flour fractions obtained from the middle portion of endosperm were lower in ash content. Ash content was higher in break streams than in reduction flour streams whereas flour fractions obtained from the middle portion of endosperm were lower in ash content which is consistent with the ash content of Australian and Russian wheats studied by Abo-Dief et al.[Citation13] because of more bran contamination which is higher in ash than endosperm. The ash content exhibited an incremental trend in both break and reduction streams which is in line with results reported by Iqbal et al.[Citation11] in mill stream blends, Sakhare et al.[Citation31] and Abo-Dief et al.[Citation13] for flour streams. Mirables[Citation18]recorded NIR ash content of 0.37% to 1.54%.
Zeleny value
Data revealed that the Zelney value was found to be highly significantly influenced by variation among different mill streams of both wheat varieties. Zelney value varied from 35 to 44 mL and 37 to 41 mL among different milled fractions of Inqulab-91 and AS-2002, respectively (). The highest Zeleny score was observed in B4 in Inqulab-91 whereas in B3 and B4 for AS-2002 while lowest in T1 for both wheat varieties. Break flour streams showed a higher Zelney value than reduction. A progressive rise in Zeleny value was observed in break flour streams while the variable trend for reduction streams as its value was the same in the first four middling streams and then decreased in M5, rising gradually in tailings, sizing and low-grade flour. The results of the present investigation are in close conformity with the findings by Iqbal et al.[Citation11] who recorded mean for Zeleny number varied from 36 to 41 mL in different flour blends and higher in break blends than in reduction flour blends. Konopka[Citation34] reported Zeleny sedimentation value in the range of 29.50 to 62.00 cm3.
Table 3. NIR Zeleny value and fat content of mill streams.
Fat content
The fat content of the mill streams showed significant variations among different fractions. For the AS-2002 wheat variety, the fat content ranged from 0.43% to 1.09% among different mill fractions, while for the Inqulab-91 wheat variety, it ranged from 0.70% to 1.51% (as shown in ). In general, the break stream flours had higher fat content compared to the reduction flour streams. The mean scores for fat content in both break and reduction flour fractions exhibited an incremental trend, indicating an increase in fat content with each subsequent fraction. Among the break streams, the highest fat content was observed in B4 for both wheat varieties, while the lowest value was recorded in B1 for AS-2002 and low-grade flour for Inqulab-91. For the reduction streams, the highest mean fat content was found in T1 and sizing fractions, while the lowest values were observed in M5 and low-grade flour fractions for AS-2002 and Inqulab-91, respectively. These results suggest that the distribution of fat content varies across different mill streams, with higher fat content in certain fractions.
In general, break streams contained more fat content than reduction flour and the highest fat content was observed in B4. The results of the present investigation are in close agreement with the previous findings of Prabhasankar et al.[Citation35] who reported that the fat content of 1.30, 1.41 and 1.60% respectively in wheat milled in the mill I, II and III and increasing trend with progressive grinding from I to V break in mills I, II and III.
The findings of the present study indicate that the fat content follows a specific pattern in the break streams of both wheat varieties. Initially, there is an increase in fat content in the first two break streams, followed by a decrease in the third break stream, and finally an increase again in the fourth break stream. This trend is not observed in the reduction streams, where no exact pattern is identified. These differences in the results may be attributed to variations in milling conditions and fat extraction methods employed in different studies. Factors such as the gap between rolls, duration of milling, and extraction techniques can influence the fat content obtained. The tailings fractions (T1 and T2) of both wheat varieties were found to have exceptionally higher fat content compared to other reduction flour streams. This can be attributed to the pressing of the germ during the milling process, resulting in the extraction of oil. The first two-break rolls primarily open up the grains and derive flour from the central portion of the endosperm, leading to relatively lower fat content in these break streams.[Citation36] On the other hand, reduction streams tend to have a higher concentration of germ, bran, pericarp and aleurone layers, which are known to contain lipids. The pressing of the germ in a series of reduction rolls can release more lipids, thereby contributing to the higher fat content observed in reduction flour streams. Overall, the observed variations in fat content among different mill streams can be attributed to the milling process, including the distribution of grain components and the extraction of oil from the germ.
Tosi et al.[Citation10] and Abo-Dief et al.[Citation13] reported a gradient in lipids content in various parts of wheat grain. Hence, fat difference among flour streams might be attributed to variations in bran proportion rich in fat which is in concordance with Brütsch et al.[Citation36] and Abo-Dief et al.[Citation13]
Friabilin content
Friabilin protein was extracted from each mill-stream flour and analyzed through SDS-PAGE. The results regarding the presence or absence of 15 kDa protein, i.e., friabilin have been presented in , and the electrophoregrams have been shown in (a, b, c and d). Among different mill streams of wheat variety AS-2002, B2, B4, M3, M5, T1 and sizing showed a prominent band of 15 kDa while a faint band was observed in all remaining mill streams of this variety. The gel images showed the absence of a 15 kDa band in M3, T2, sizing and low-grade flour of Inqulab-91 mill streams while all remaining streams depicted the presence of a 15 kDa protein band with low intensity.
Figure 2. (a). Presence of 15 kDa band in mill streams B1, B2, B3, B4, M1, M2, M3 (b). M4, M5, T1, T2, sizing and LG of AS-2002 (c). 15 kDa band in millstreams B1, B2, B3, B4, M1, M2, M3, M4. (d). M5, T1, T2, sizing and LG of Inqulab-91.
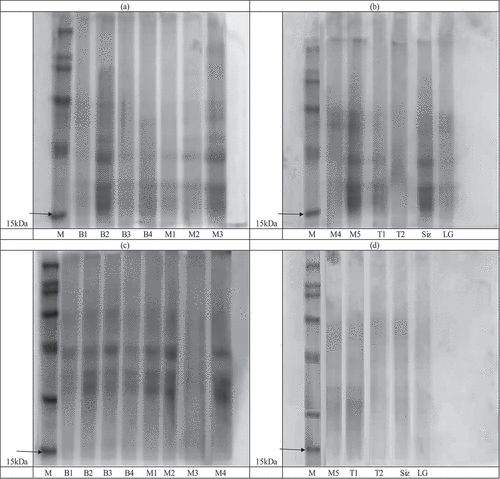
Table 4. Correlation of friabilin with different chemical constituents of flour streams.
The results of the current study, focusing on the distribution of the 15 kDa protein band, suggest that mill streams containing a prominent band may indicate a higher proportion of the endospermic portion. This portion is characterized by a significant amount of starch and the presence of a protein called friabilin on the surface of water-washed starch granules. This association between the 15 kDa protein band and the endospermic portion suggests a correlation with the texture of the grain, specifically a softer grain texture. However, the mill streams showing the absence of a 15 kDa polypeptide band may have less proportionate content of endosperm with lower starch content and hence probably least friabilin content, i.e., hard textured. The results of the present investigation are supported by Tosi et al.[Citation10] who reported that different parts of the endosperm and the flour obtained from these vary in functional attributes during food processing.
The molecular level factors that contribute to wheat grain hardness are primarily associated with the adhesion between starch granules and the protein matrix. The degree of this adhesion is regulated by a protein complex called friabilin. Geneix et al.[Citation37] have suggested that the interaction between starch and protein plays a crucial role in determining the hardness of wheat grains.
Understanding the molecular mechanisms behind wheat grain hardness is valuable for wheat breeders. This knowledge allows breeders to make informed decisions and utilize the potential of different wheat varieties in their hybridization programs. By selecting wheat varieties with specific characteristics related to grain hardness, breeders can work toward developing wheat varieties with desired textures, whether it is hard or soft, based on the specific requirements or preferences.
By studying the role of protein complexes like friabilin and the adhesion between starch and protein, breeders can gain insights into the genetic and molecular factors influencing grain hardness. This information can guide breeding strategies aimed at improving wheat quality and developing wheat varieties with desirable traits.
The presence of friabilin correlated with soft while its absence is associated with a hard texture of the wheat grain. The presence or absence of friabilin is independent of environmental effects and agronomic practices. Friabilin is present on the surface of water-washed starch from soft wheat in high amounts and on hard wheat starch in small quantities while absent from durum wheat starch. Pakistani spring wheat varieties are medium hard with faint friabilin bands.[Citation26]
Correlation studies
Friabilin was a positive relationship with all attributes except fat content which is negatively associated (r=-0.30) in wheat variety AS-2002 while it exhibited a negative association with gluten (−0.15), Zelney (−0.13) and fat (−0.02) contents in Inqulab-91 (). The existence of friabilin on water-washed wheat starch was perfectly correlated with the qualitative level of endosperm hardness. The friabilin-endosperm softness relationship was confirmed in hundreds of genotypes. Giroux and Morris[Citation38] reported that the quantitative level of friabilin was highly correlated (r =-0.780) with wheat grain softness in chromosome 5D recombinant substitution lines which is confirmed by Pasha[Citation39] who found a slight correlation (r = 0.18) between friabilin with cookie score. Contrarily friabilin depicted no link between friabilin and other quality characters.
Table 5. Friabilin protein by SDS-PAGE in different mill streams of wheat varieties.
Conclusion
Uneven distribution of constituents within wheat grain inflected disparity in the composition of flour from different mill streams. Distribution of protein, moisture, gluten, Zelney, fat and ash content varied in different mill streams. Information on the distribution of various chemical constituents and friabilin protein is valuable for millers and food processors to formulate appropriate flours by blending particular flour streams having different chemical and functional properties for specified end uses. Streams with higher protein, gluten and Zelney values and lower friabilin are suitable for leavened baked products like bread and its variants roti and chapatti, naan and pizza while fractions with higher friabilin and less strong gluten are better suited for cookies, cakes and pastries.
Acknowledgments
The authors express their gratitude to Princess Nourah bint Abdulrahman University Researchers Supporting Project number (PNURSP2023R171), Princess Nourah bint Abdulrahman University, Riyadh, Saudi Arabia.
Disclosure statement
No potential conflict of interest was reported by the author(s).
Additional information
Funding
References
- Iqbal, Z.; Pasha, I.; Abrar, M.; Hanif, M. S.; Arif, A. M.; Masih, S. Protein Concentration, Composition and Distribution in Wheat Flour Mill Streams. Annals. Food Scie. Tech. 2015, 16(1), 104–114.
- Qin, H.; Ma, D.; Huang, X.; Zhang, J.; Sun, W.; Hou, G.; Wang, C.; Guo, T. Accumulation of Glycolipids in Wheat Grain and Their Role in Hardness During Grain Development. Crop. J. 2019, 7(1), 19–29. DOI: 10.1016/j.cj.2018.08.005.
- Iqbal, Z.; Pasha, I.; Abrar, M.; Masih, S.; Hanif, M. S. Physico-Chemical, Functional and Rheological Properties of Wheat Varieties. J. Agr. Res. 2015, 53, 253–267.
- Hourston, J. E.; Ignatz, M.; Reith, M.; Leubner-Metzger, G.; Steinbrecher, T. Biomechanical Properties of Wheat Grains: The Implications on Milling. J. R. Soc. Interface. 2017, 14(126), 20160828. DOI: 10.1098/rsif.2016.0828.
- Nirmal, R. C.; Furtado, A.; Wringly, C.; Henry, R. J. Influence of Gene Expression on Hardness in Wheat. PLoS. One. 2016, 11(10), e0164746. DOI: 10.1371/journal.pone.0164746.
- Ma, X.; Sajjad, M.; Wang, J.; Yang, W.; Sun, J.; Li, X.; Zhang, A.; Liu, D. Diversity and Distribution of Puroindoline Genes and Their Effect on Kernel Hardness in Diverse Panel of Chinese Wheat Germplasm. BMC. Plant. Biol. 2017, 17(1), 158. DOI: 10.1186/s12870-017-1101-8.
- Sharma, R.; Rawat, A.; Misra, B. K.; Nagarajan, S. Distribution of Grain Hardness in Indian Wheat Varieties and Landraces. Wheat Info. Ser. 2012, 114, 1–8.
- Szabo, B. P.; Veha, A.; Gyimes, E.; Horvath, Z. H. Flour Quality and Kernel Hardness Connection in Winter Wheat. Acta Univ. Sapientiae, Alimentaria. 2016, 9(1), 33–40. DOI: 10.1515/ausal-2016-0003.
- Pasha, I.; Anjum, F. M.; Morris, C. F. Grain Hardness: A Major Determinant of Wheat Quality. Food Sci. Technol. Int. 2010, 16(6), 511–522. DOI: 10.1177/1082013210379691.
- Tosi, P.; Heb, J.; Lovegrovec, A.; Gonzáles-Thuillierc, I.; Pensond, S.; Shewry, P. R. Gradients in Compositions in the Starchy Endosperm of Wheat Have Implications for Milling and Processing-A Review. Trends Food Sci. Technol. 2018, 82, 1–7. DOI: 10.1016/j.tifs.2018.09.027.
- Iqbal, Z.; Pasha, I.; Hanif, M. S.; Randhawa, M. A.; Rashid, A.; Arif, A. M. Effect of Specialty Flours on Physical and Sensorial Attributes of Cookies. Pak. J. Agric. Sci. 2015, 52, 1091–1098.
- Vukić, M. S.; Janić-Hajnal, E. P.; Mastilović, J. S.; Vujadinović, D. P.; Ivanović, M. M.; Šoronja-Simović, D. M. Application of Solvent Retention Capacity Tests for Prediction of Rheological Parameters of Wheat Flour Mill Streams. Hem. Ind. 2020, 74(1), 37–49. DOI: 10.2298/HEMIND190625001V.
- Abo‐Dief, M.; Abo‐Bakr, T.; Youssef, M.; Moustafa, A. Physicochemical and Rheological Properties of Australian and Russian Wheat Flour Mill Streams. Cereal. Chem. 2022, 99(2), 421–431. DOI: 10.1002/cche.10508.
- Pojić, M. M.; Spasojević, N. B.; Atlas, M. Đ. Chemometric Approach to Characterization of Flour Mill Streams: Chemical and Rheological Properties. Food Bioprocess. Technol. 2014, 7(5), 1298–1309. DOI: 10.1007/s11947-013-1133-5.
- Liu, Y.; Ohm, J. B.; Hareland, G.; Wiersma, J.; Kaiser, D. Sulfur, Protein Size Distribution, and Free Amino Acids in Flour Mill Streams and Their Relationship to Dough Rheology and Breadmaking Traits. Cereal Chem. J. 2011, 88(2), 109–116. DOI: 10.1094/CCHEM-06-10-0086.
- Ramseyer, D. D.; Bettge, A. D.; Morris, C. F. Distribution of Total, Water‐Unextractable, and Water‐Extractable Arabinoxylans in Wheat Flour Mill Streams. Cereal Chem. J. 2011, 88(2), 209–216. DOI: 10.1094/CCHEM-10-10-0148.
- Pojić, M. M.; Mastilović, J. S. Near Infrared Spectroscopy—Advanced Analytical Tool in Wheat Breeding, Trade, and Processing. Food Bioproc. Tech. 2013, 6(2), 330–352. DOI: 10.1007/s11947-012-0917-3.
- Miralbes, C. Analytical, Nutritional and Clinical Methods: Quality Control in the Milling Industry Using Near Infrared Transmittance Spectroscopy. Food Chem. 2004, 88(4), 621–628. DOI: 10.1016/j.foodchem.2004.05.004.
- Bramble, T.; Dowell, F. E.; Herrman, T. J. Single-Kernel Near-Infrared Protein Prediction and the Role of Kernel Weight in Hard Red Winter Wheat. Appl. Eng. Agric. 2006, 22(6), 945–949. DOI: 10.13031/2013.22241.
- Dowell, F. E.; Maghirang, E. B.; Xie, F.; Lookhart, G. L.; Pierce, R. O.; Seabourn, B. W.; Bean, S. R.; Wilson, J. D.; Chung, O. K. Predicting Wheat Quality Characteristics and Functionality Using Near-Infrared Spectroscopy. Cereal Chem. J. 2006, 83(5), 529–536. DOI: 10.1094/CC-83-0529.
- Pojić, M.; Mastilović, J.; Palić, D.; Pestorić, M. The Development of Near Infrared Spectroscopy (NIRS) Calibration for Prediction of Ash Content in Legumes on the Basis of Two Different Reference Methods. Food Chem. 2010, 123(3), 800–805. DOI: 10.1016/j.foodchem.2010.05.013.
- Fistes, A.; Rakic, D.; Takaci, A. The Function for Estimating the Separation Efficiency of the Wheat Flour Milling Process. J. Food Sci. Technol. 2013, 50(3), 609–614. DOI: 10.1007/s13197-012-0717-9.
- Cozzolino, D. Authentication of Cereals and Cereal Products. Vil. I, Pp. 441-457. In Advances in Food Authenticity Testing; Gerard, D., Ed.; Woodhead publishing: Cambridge, MA, USA, 2016; pp. 441–457. DOI:10.1016/B978-0-08-100220-9.00016-3.
- AACC. Approved Methods of the AACC, 11th; American Association of Cereal Chemists: St. Paul, MN, USA, 2010. Methods 26-95.01, 39-11.01, 39-20.01, 55-31.01, 56-81.03
- Pasikatan, M. C.; Haque, E.; Spillman, C. K.; Steele, J. L.; Milliken, G. A. Granulation Sensing of First-Break Ground Wheat Using a Near-Infrared Reflectance Spectrometer: Studies with Soft Red Winter Wheats. J. Sci. Food Agri. 2003, 83(3), 151–157. DOI: 10.1002/jsfa.1290.
- Pasha, I.; Anjum, F. M.; Butt, M. S. Biochemical Characterization of Spring Wheats in Relation to Grain Hardness. Int. J. Food Prop. 2009, 12(4), 910–928. DOI: 10.1080/10942910802123281.
- Montgomery, D. C. Design and Analysis of Experiments, 8th ed.; John Willy and Sons Inc: Tempe, Arizona, 2017.
- Yahata, E.; Maruyama-Funatsuki, W.; Nishio, Z.; Yamamoto, Y.; Hanaoka, A.; Sugiyama, H.; Tanida, M.; Saruyama, H. Relationship Between the Dough Quality and Content of Specific Glutenin Proteins in Wheat Mill Streams and Its Application to Making Flour Suitable for Instant Chinese Noodles. Biosci. Biotechnol. Biochem. 2006, 70(4), 788–797. DOI: 10.1271/bbb.70.788.
- Dornez, E.; Gebruers, K.; Wiame, S.; Delcour, J.; Courtin, C. M. Insight into the Distribution of Arabinoxylans, Endoxylanases, and Endoxylanase inhibitors in Industrial wheat roller mill streams. J. Agric. Food Chem. 2006, 54(22), 8521–8529. DOI: 10.1021/jf061728n.
- Gomez, M.; Ruiz-Paris, E.; Banu, O.; Liete, B. Influence of Flour Mill Streams on Cake Quality. Int. J. Food Sci. Tech. 2010, 45(9), 1794–1800. DOI: 10.1111/j.1365-2621.2010.02338.x.
- Sakhare, S. D.; Inamdar, A. A.; Indrani, D.; Madhu Kiran, M. H.; Rao, G. V. Physicochemical and Microstructure Analysis of Flour Mill Streams and Milled Products. J. Food Sci. Technol. 2015, 52(1), 407–414. DOI: 10.1007/s13197-013-1029-4.
- El-Porai, E. S.; Salama, A. E.; Sharaf, A. M.; Hegazy, A. I.; Gadallah, M. G. E. Effect of Different Milling Processes on Egyptian Wheat Flour Properties and Pan Bread Quality. Ann. Agric. Sci. 2013, 58(1), 51–59. DOI: 10.1016/j.aoas.2013.01.008.
- Banu, I. S.; Georgeta, S.; Violeta, I.; Iuliana, A. Physicochemical and Rheological Analysis of Flour Mill Streams. Cereal. Chem. 2010, 87(2), 112–117. DOI: 10.1094/CCHEM-87-2-0112.
- Konopka, I.; Fornal, L.; Abramczyk, D.; Rothkaehl, J.; Rotkiewicz, D. Statistical Evaluation of Different Technological and Rheological Tests of Polish Wheat Varieties for Bread Volume Prediction. J. Food Sci. Technol. 2004, 39(1), 11–20. DOI: 10.1111/j.1365-2621.2004.00741.x.
- Prabhasankar, P.; Sudha, M. L.; Haridas Rao, P. Quality Characteristics of Wheat Flour Milled Streams. Food Res. Int. 2000, 33(5), 381–386. DOI: 10.1016/S0963-9969(00)00059-4.
- Brütsch, L.; Huggler, I.; Kuster, S.; Windhab, E. J. Industrial Roller Milling Process Characterisation for Targeted Bread Quality Optimization. Food Bioprocess. Technol. 2017, 10(4), 710–719. DOI: 10.1007/s11947-016-1856-1.
- Geneix, N.; Dalgalarrondo, M.; Tassy, C.; Nadaud, I.; Barret, P.; Bakan, B.; Elmorjani, K.; Marion, D.; Kumar, A. Relationships Between Puroindoline A-Prolamin Interactions and Wheat Grain Hardness. PLoS. One. 2020, 15(9), e0225293. DOI: 10.1371/journal.pone.0225293.
- Giroux, M. J.; Morris, C. F. Glycine to Serine Change in Puroindoline B is Associated with Wheat Grain Hardness and Low Levels of Starch-Surface Friabilin. Theor. Appl. Genet. 1997, 95(5–6), 857–864. DOI: 10.1007/s001220050636.
- Pasha, I. Biochemical characterization of Pakistani wheats in relation to grain hardness, PhD thesis, University of Agriculture Faisalabad, Pakistan (2006).