ABSTRACT
In the present study, four waxy rice varieties (two japonica and two indica) with contrasting hardening rates were used for physicochemical properties investigation. The results showed that the hardness of cooked rice grains from zhenzhunuo and zhennuo29 increased slowly after cool storage, whereas those from zaonuo5 and yj98 raised rapidly. The peak gelatinisation temperatures for yj98, zaonuo5, zhennuo29, and zhenzhunuo flours were 78.60°C, 73.55°C, 69.00°C, and 67.25°C, respectively. The retrogradation enthalpies of stored flours (4°C, 48 h) followed the order: yj98 (3.876 J/g) > zaonuo5 (2.202 J/g) > zhennuo29 (1.684 J/g) > zhenzhunuo (1.370 J/g). Scanning electron microscopy (SEM) results exhibited that after cool storage, the cooked rice grains of zhenzhunuo and zhennuo29 had no obvious changes in morphology, whereas zaonuo5 presented increased cavities and yj98 occurred new fissures. Among intra-subspecies varieties, the rapid hardening rice accessions had a lower ratio of A chains (DP 6–12) but larger proportion of B3 chains (DP ≥ 37) than that of the corresponding genotypes with slow hardening. The physicochemical characteristics disclosed here will facilitate the application of the four cultivars in food processing industry.
Introduction
Waxy rice (Oryza sativa), also called glutinous rice, sticky rice, or sweet rice, is characterized mainly by its extremely low amylose content (<5%),[Citation1] because of a loss-of-function mutation in the Waxy gene.[Citation2] It has a milky-white, opaque appearance and a soft, sticky texture after cooking. Waxy rice flour presents the advantages of smoothness, elasticity, possessing a high water retention capacity, and having a low degree of retrogradation.[Citation3] Owing to these unique characteristics, it has been widely utilized in food industry in various forms, like snacks, wines, puddings, rice dumplings (Zong-zi), glutinous rice balls (Tang-yuan), and sauces.[Citation4] Gelatinisation, pasting, and retrogradation properties constitute the major physicochemical components of starch-based products. The three properties determine rice cooking, eating, and processing qualities,[Citation5] among which, retrogradation is the key determinant of food processing quality.[Citation6] The effects of retrogradation on starchy foods can be desirable or undesirable. In some applications (e.g., noodles, soups, and sauces), starch retrogradation is satisfying because of alterations in sensorial, structural, and mechanical properties.[Citation7] More usually, retrogradation is undesirable as it profoundly affects the textural attribute, eating quality, storage stability, and nutritional value. This can reduce not only the shelf-life of a prepared food but also consumers’ acceptance, and thereby cause food waste and economic loss.[Citation8]
Retrogradation, also referred to as aging, is an ongoing change that occurs in starchy foods during cooling or preservation, in which gelatinised starch automatically rearranges from a disordered state to an ordered state.[Citation9] An ordered structure, including short-range of double helices and long-range of crystallites formed from aggregates of double helices, is mainly developed through the formation of hydrogen bonds between hydroxyl groups on inter- and intra-starch molecules.[Citation10] It was reported that inhibiting the packing of double helices into crystallites could suppress retrogradation.[Citation11] Retrogradation usually accompanies with a series of physiological changes, including increased hardness, turbidity, fragmentation, decreased springiness, digestion, and water-holding ability.[Citation12] In terms of storage time, retrogradation can be classified as short-term and long-term retrogradation.[Citation8] For the short-term aging, it is dominated by amylose and occurs mostly within 24 h because of the fast recrystallization of amylose.[Citation13] In contrast, the long-term retrogradation is predominated by amylopectin and can continue for several days, weeks, or even months due to the low mobility and branching steric hindrance of amylopectin.[Citation14] It is generally recognized that the retrogradation degree of waxy rice starches is lower than that of non-waxy rice starches, since waxy rice starch is almost entirely composed of amylopectin, while not for non-waxy rice starch.[Citation15,Citation16] Despite that, retrogradation of some waxy rice varieties proceeds faster than that of non-waxy rice for uncertain reasons during conservation. Similar to the gelatinisation property, retrogradation is partly determined by the fine structure of amylopectin: starch with a shorter amylopectin exterior chain length (ECL) presents a lower extent of retrogradation, while a longer ECL displays a greater degree of retrogradation.[Citation17]
It is generally believed that physicochemical property is one of the determinators for the quality of a prepared food.[Citation18] A better knowledge concerning physicochemical properties, especially retrogradation, is of great importance for waxy rice flour applications and industrial process optimization. Given this, we characterized the physicochemical attributes of four waxy rice accessions with varying hardening rates in the present study.
Materials and methods
Rice sample preparation
Four waxy rice accessions including two long grain indica varieties (zhenzhunuo and zaonuo5) and two round grain japonica varieties (zhennuo29 and yj98) were used as materials. When the cooked grains were stored at 4°C, zhenzhunuo and zhennuo29 showed extremely slow rate of hardening, whereas zaonuo5 and yj98 were characterized by rapid hardening. These rice genotypes were planted in May 2020 at the experimental fields of our research team in Jurong, Jiangsu Province, China (119°10′44″ E and 31°47′51″ N). Plants from each variety were grown under conventional management. Grains were harvested at maturity and dried until reaching a moisture content of 12–14%. Subsequently, the grains were dehulled with a rice huller (THU-35A, Satake Ltd, Tokyo, Japan) and polished with a Pearlest grain polisher (Kett Electric Laboratory, Tokyo, Japan). Intact grains were manually selected and retained for further experiment. Rice flours were prepared through a milling process: polished grains were first ground into fine powder in liquid nitrogen with a Mixer Mill MM 400 (Retsch, Haan, Germany) and then passed through a 0.25 mm mesh screen.
Rice cooking
Twenty grams of polished rice grains were placed in an aluminum box, rinsed three times in deionized water, and mixed with water (rice mass to deionized water mass ratio = 1:1). Then, the prepared samples were transferred to a steamer and steamed with boiling water. After 40 min of steaming, the heating was stopped and the rice samples were maintained for an additional 10 min before being removed from the steamer.
Hardness measurement
To explore the hardness properties, the cooked rice grains of different rice varieties were subjected to 4°C for different storage time intervals (0, 12, 24, 36, and 48 h). The hardness of the stored rice was assayed by a TA-XT2i® Texture Analyzer (Stable Microsystems Ltd., Surrey, UK) using a two-cycle, force-versus-distance compression program. Instrument parameters were set as follows: Texture profile analysis mode; Pre-Test Speed, 0.5 mm/s; Test Speed, 0.5 mm/s; Post-Test Speed, 2.00 mm/s; Strain, 90%; Time, 5.00 s; Trigger Force (auto), 0.05 N. Twelve texture measurements were performed for each rice variety. After fulfilling the detection, hardness values were outputted by the supplier’s software.
Thermal and retrogradation properties
The thermal and retrogradation characteristics of rice flours were determined using a differential scanning calorimeter (DSC) instrument (Netzsch DSC 214 Polyma; Netzsch-Geratebau GmbH, Selb, Germany). For gelatinisation, 3 mg of rice flour was precisely weighed into an aluminum pan and mixed with a 6 μL of distilled water. Subsequently, all the samples were tightly sealed and left for equilibration at room temperature (~25°C) overnight. After equilibration, the prepared samples were heated from 20°C to 100°C at a rate of 10°C/min and then cooled to room temperature (25°C). For retrogradation, completely gelatinised flour gels were stored at 4°C for different time intervals (12, 24, 36, and 48 h) and the storage samples were scanned with a DSC instrument, according to the same thermal cycle as gelatinisation. For all the measurements, a sealed empty pan was used as the reference. The thermal and retrogradation parameters including onset temperature (To), peak temperature (Tp), conclusion temperature (Tc), gelatinisation endothermic enthalpy (ΔHgel), and retrogradation enthalpy (ΔHret) were computed using DSC software (TA instruments, New Castle, USA).
Pasting characteristics
The pasting properties of rice flour were evaluated by a rapid visco-analyzer (RVA; Newport Scientific, Warriewood, Australia). For each RVA run, 3 g of rice flour was weighed directly into an RVA canister and then 25 g of distilled water was added. The suspension was stirred manually by an RVA paddle prior to detection. Instrument setting was the following: equilibrating the slurry at 50°C for 1 min, heating the temperature to 95°C within 4 min, holding the temperature at 95°C for 5 min, reducing the temperature to 50°C within 4 min, and maintaining at 50°C for 2 min. During the measurement, the paddle speed was set at 960 rpm for the initial 10 s and then kept at 160 rpm until the termination of the experiment. Pasting parameters including peak viscosity, through viscosity, final viscosity, breakdown, setback, and pasting temperature were recorded from the pasting curves.
Scanning electron microscopy (SEM) analysis
Based on the hardness analysis, the cooked rice grains of zaonuo5 and yj98 varieties showed remarkable retrogradation after 24 h of storage at 4°C, as represented by the obvious increase in hardness. Therefore, we selected the gelatinised rice grains before (0 h) and after 24 h of 4°C storage for SEM. The collected samples were first freeze-dried using a Labconco FreeZone 6 freeze drier (Labconco Corporation, Kansas, USA) and cut horizontally into two halves in the middle. Followingly, a half of the grain was attached to a brass disk and coated with gold-palladium. Finally, microstructure was examined in a Hitachi-S-3000N scanning electron microscope (Hitachi, Co., Ltd. Japan) under 15 kV acceleration and 1500× magnification.
Starch isolation and debranching
Rice starch was isolated from rice flour using a protease method as described by Han.[Citation19] Briefly, rice flour (20 g) was dispersed in 0.45% (w/v) sodium metabisulfite solution (20 mL) at 4°C overnight and then centrifuged at 4000 rpm for 10 min. After centrifugation, the supernatant was discarded and the starch precipitate was dissolved in 40 ml of ethyl alcohol tricine buffer (250 mM, pH 7.5) with protease at 37°C overnight to remove protein. The mixture was then centrifuged at 4000 rpm for 10 min, and the precipitate was washed orderly with deionized water (twice) and ethanol, so as to remove soluble impurities and lipids. The extracted starch powders were finally dried at room temperature.
Purified starch was debranched with isoamylase using the following steps: 4 mg of isolated starch was first dispersed with acetate buffer (0.01 M, pH 4.2) and then the solution was placed in a boiling water bath with shaking for 1 h, cooled to room temperature (25°C). After cooling, 5000 U of isoamylase was added and the mixture was incubated at 50°C overnight. The debranching process was terminated by adding 0.1 M NaOH, and the debranched samples were freeze-dried for further analysis.
Measurement of amylopectin chain-length distributions (CLDs) by fluorophore-assisted carbohydrate electrophoresis (FACE)
About 0.3 mg of debranched starch powders were 1.5 μL of labeled with 8-aminopyrene-1,3,6,trisulfonic acid (APTS) as fluorophore following a previously described procedure.[Citation20] The labeled starch was subjected to a FACE micro-vial and then analyzed using a P/ACE MDQ plus system (Ab Sciex, Framingham, USA). After detection, FACE system directly provides the number, Nde(X), which is the number of chains with degree of polymerisation (DP) X. The number and weight of CLDs can be inter-converted with the following mathematical model: w (logX) = X2 Nde(X).[Citation19]
Statistical analysis
All experiments were performed in triplicate unless otherwise stated. Analysis of variance (ANOVA) of means was performed by using SPSS17.0 software (IBM Corporation, Armonk, USA). Statistically significant differences of the mean values were determined with Duncan’s multiple tests at P < .05.
Results and discussion
Hardness properties of waxy rice
To dissect the hardness property of the waxy rice varieties, we performed a texture profile analysis (TPA). As shown in , the cooked rice grains of zhenzhunuo did not display statistically significant differences in the hardness throughout the storage period, while those of the other three varieties were generally elevated with increasing storage time, with valley values occurring at 0 h. During storage, the growing hardness is the main indicator of retrogradation for starch-based products.[Citation21] Since there were no changes for zhenzhunuo hardness, it can be inferred that this variety did not retrograde and had an extremely slow rate of hardening. Despite the fact that the hardness of zhennuo29 was generally increased over time, it did not exhibit evident retrogradation, as its values at the end of the treatment (48 h) were similar to the starting levels of zaonuo5. Given these, zhennuo29 was also recognized to have a slow rate of hardening, similar to zhenzhunuo. As for zaonuo5 and yj98, their hardness levels were always higher than zhenzhunuo and zhennuo29 after storage. At the end of storage, zaonuo5 varieties showed a sharp increase in hardness, being 4.47 times larger than that at 0 h. For yj98, its hardness level at 36 h post storage was 9.51 times larger than that at 0 h, and the values at 48 h were even higher than the instrument’s detection limit. As anticipated, zaonuo5 and yj98 both presented an extremely fast hardening rate and could be retrograde within 2 days of storage. Generally, the retrogradation of waxy rice is long-term recording because of the lack of amylose.[Citation22] The hardness performance of zaonuo5 and yj98 in our study seemed that there were exceptions.
Table 1. The hardness levels of cooked rice grains at different storage (4°C) times.
Thermal and retrogradation properties of rice flours
DSC measurements can provide the endothermic energy and gelatinisation temperature changes during the dissociation of starch molecules from a double-helical structure to an amorphous state.[Citation15] summarizes the gelatinisation temperatures (To, Tp, Tc), gelatinisation temperature range (ΔT), and gelatinization enthalpy (ΔHgel) of the four waxy rice varieties. Overall, there was significant variation in gelatinisation temperatures (GT) among the genotypes. Flour from zhenzhunuo had the lowest To, Tp, and Tc, followed by zhennuo29, zaonuo5, and yj98. Based on the Tp, rice varieties can be divided into three classes: low (Tp < 70°C), intermediate (Tp within the range of 70 ~ 73°C), and high (Tp > 73°C) GT groups.[Citation23] According to these criteria, zhenzhunuo (Tp = 67.25°C) and zhennuo29 (Tp = 69.00°C) were the low-GT genotypes, while zaonuo5 (Tp = 73.55°C) and yj98 (Tp = 78.60°C) were the high-GT lines. On the basis of DSC and TPA results, we found that low-GT waxy rice varieties exhibited softer texture than the high-GT genotypes following a period of cooling. This seemed to be a common phenomenon in rice.[Citation24] The reason might be ascribed to the fact that starches showing lower GT generally harbor smaller proportion of initially perfect crystalline structures and would retrograde slower after gelatinisation.[Citation25] Gelatinisation temperature range (ΔT) is an indicator of the structural heterogeneity within starch granules.[Citation26] The average ΔT values of zhenzhunuo, zaonuo5, zhennuo29, and yj98 were 12.5°C, 9.15°C, 10°C, and 9.6°C, respectively. Zhenzhunuo possessed an obviously higher ΔT than the other three varieties, suggesting that this variety had the most heterogenous crystalline regions. Gelatinisation enthalpy (ΔHgel) reflects the amount of energy needed for unraveling of double helices during gelatinisation.[Citation27] Among the tested varieties, zaonuo5 had the highest ΔHgel, suggesting that this variety needed more energy to be gelatinised. The ΔHgel of zhennuo29 flour was the lowest, indicating that it required less energy to be fully gelatinised.
Table 2. Gelatinisation properties of waxy rice flours.
Retrogradation enthalpy (ΔHret) is the quantity of energy needed for melting of recrystallized starch, and a higher ΔHret demonstrates a greater tendency to retrograde.[Citation28] To analyse the retrogradation of the four varieties, gelatinised rice flours were stored at 4°C for 48 h and the ΔHret were detected every 12 h. As shown in , the ΔHret values of all rice gels increased gradually over storage time, with the peak values present at 48 h. Among the four varieties, yj98 always showed the highest ΔHret values throughout the aging process, and zaonuo5 consistently exhibited the second highest, while zhenzhunuo and zhennuo29 presented the lowest. After 48 h of storage, the ΔHret of yj98, zaonuo5, zhennuo29, and zhenzhunuo were 3.88, 2.20, 1.68, and 1.37 J/g, respectively. These results imply that the high-GT waxy rice variety was more inclined to retrogradation than the low-GT genotypes. Our findings were consistent with a previous work, which showed that the ΔHret of high-GT waxy rice was much higher than those of low-GT ones when stored at 4°C.[Citation29]
Pasting profiles of milled rice flour
The pasting viscosity profiles and parameters of rice flours are shown in and . The pasting temperatures (PT) of zhenzhunuo, zhennuo29, zaonuo5, and yj98 were 72.0
Table 3. Pasting properties of waxy rice flours.
°C, 72.8°C, 79.9°C, and 80.7°C, respectively. It is reported that PT detected by RVA does not accurately reflect the actual GT as measured by DSC, and this may be the result of different internal applications for RVA and DSC detections.[Citation30] In our study, despite that the RVA-measured PTs were not identical with the Tp derived from DSC, they generally presented the same trends, with the PT of yj98 and zaonuo5 being higher than that of zhenzhunuo and zhennuo29 (). Zhenzhunuo rice flour exhibited the highest peak viscosity (3355 cP), trough viscosity (1720 cP), and final viscosity (1997 cP), whereas zaonuo5 displayed the lowest peak viscosity (1944 cP) and trough viscosity (1292 cP) (). Peak viscosity is influenced by the extent of amylopectin leaching, the ability of granular to swell, and the content of phosphate monoester.[Citation31] In our study, given a relatively low value of peak viscosity, zaonuo5 was deduced to have low starch leaching rate and granule swelling volume when cooking. We found that there were no apparent correlations between the GT and viscosity, which may be due to the fact that besides starch, viscosity is also influenced by lipids and proteins.[Citation32] Breakdown viscosity is an indicative of paste stability, and a low breakdown suggests a high ability to withstand heating and shear stress.[Citation25] For the four varieties, the breakdown viscosity of zaonuo5 was the lowest (702 cP) (), suggesting that this cultivar had high resistance to heat and shear. Setback indicates the short-term aging of gelatinised sample and a higher level of setback suggests a higher degree of retrogradation.[Citation33] In the present study, the setback values of yj98 and zaonuo5 were obviously greater than the other two genotypes (), in line with the results detected by DSC.
Morphological properties
The SEM of cooked rice grains before (0 h) and after 4°C storage (24 h) is illustrated in . Before subjecting to cold storage, the grains of zhenzhunuo, zhennuo29, and yj98 seemed to have similar morphology, both possessing a smooth surface. However, zaonuo5 had a distinct structure with respect to the other three genotypes, presenting a honeycomb-like structure on its surface. Likewise, a few cavities could be observed from the surface of zaonuo5. The production of an ordered honeycomb-like structure is likely due to the gelatinisation of starch and the adherence between gelatinised starch and denatured protein.[Citation34] This special appearance suggested that zaonuo5 might have lower amount of amylopectin leaching with respect to the other three accessions, as revealed by the lowest peak viscosity and breakdown values (). After cold storage, the microstructures of zhenzhunuo and zhennuo29 remained basically unchanged, having quite smooth surface. This might suggest that zhenzhunuo and zhennuo29 had low retrogradation properties, consistent with the conclusions obtained by TPA and DSC results ( and ). For both zaonuo5 and yj98, the morphologies of rice grains before and after storage were obviously different. Following cold storage, zaonuo5 showed an increase in the number of cavities, and the grains of yj98 generated an obvious fissure. When a gelatinised starchy product was placed at low temperature, it often facilitates the re-association of starch molecules, resulting in retrogradation.[Citation35] Retrogradation would contribute to the separation of water from the gelatinised starch (syneresis).[Citation9] Therefore, rice grains with a high tendency of retrogradation generally present a low water-retaining capacity and abundant syneresis under cold storage conditions. The formation of plenty of cavities in zaonuo5 and splits in yj98 might be ascribed to the massive loss of loosely bound water during starch staling. These results demonstrated that zaonuo5 and yj98 were very prone to retrogradation.
Figure 3. Scanning electron microscopy of cooked rice grains. a) zhenzhunuo gelatinised rice before storage. b) zhenzhunuo gelatinised rice stored at 4°C for 24 h. c) zaonuo5 gelatinised rice before storage. d) zaonuo5 gelatinized rice stored at 4°C for 24 h. e) zhennuo29 gelatinized rice before storage. f) zhennuo29 gelatinised rice stored at 4°C for 24 h. g) yj98 gelatinised rice before storage. h) yj98 gelatinised rice stored at 4°C for 24 h. Arrow indicates the cavity. Scale bar = 15 μm.
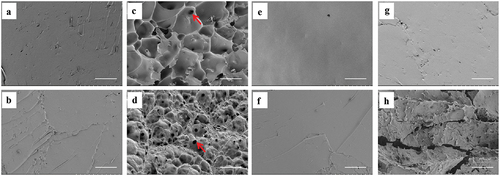
Chain-length distributions of amylopectin analyzed by FACE
In terms of waxy rice, the fine structure of amylopectin greatly affects the functional characteristics of starch.[Citation36] In our study, a FACE technique was used to dissect the precise CLD of amylopectin. To eliminate the influences of different sample concentrations, the resulting number distributions were normalized to the global maximum for qualitative comparisons.[Citation37] As shown in , all the tested samples displayed the typical CLD number with three peaks.[Citation20] The highest peak occurred at DP 12, with the shoulder ranging from DP 6 to DP 32. These regions were short amylopectin chains covering a single lamella.[Citation38,Citation39] The second peak is located at DP ~ 45, with the shoulder covering from DP 33 to DP 67. These DPs were the representatives of intermediate amylopectin chains spanning two crystalline lamellae. The third one was the global minimum peak at approximately DP 79, with the shoulder varying from DP 68 to DP 100. These DPs were the reflections of long amylopectin chains that seemed to span at least three adjacent lamellae.
Based on the amylopectin cluster model,[Citation40] amylopectin chains could be divided into four categories: A chains (DP 6–12), B1 chains (DP 13–24), B2 chains (DP 25–36), and B3 chains (DP 37–100), which represents the short, medium, long, and very long chains, respectively. The proportion of these chains in each DP type to the whole amount of chains was calculated and summarized in . The mean branch chain length was 20.49, 22.12, 22.11, and 23.02 glucose units for zhenzhunuo, zaonuo5, zhennuo29, and yj98, respectively. The ratios of A chains varied from 22.95% to 29.25% for the four varieties, with yj98 being the lowest while zhenzhunuo the highest. The proportions of DP 13–24 and DP 25–36 were similar within the two japonica varieties (zhennuo29 and yj98), but quite different between the two indica genotypes zhenzhunuo and zaonuo5. Zhenzhunuo contained a higher ratio of DP 13–24 and a lower amount of DP 25–36 than zaonuo5. The amount of starch branch chains with DP 37–100 ranged from 12.78% to 17.53%, and the highest value occurred in yj98 while the lowest level was in zhenzhunuo. Interestingly, although the indica variety zaonuo5 possessed a higher GT than the japonica genotype zhennuo29 (), they showed no significant differences for the amount of amylopectin CLDs, with the exception of DP 13–24. This might be due to the fact that the GT differentiation between the two genotypes was not large enough. For the varieties within the same subspecies, the genotype with a rapid rate of hardening always contained less short A chains and more long B3 chains than the corresponding variety with a slow rate of hardening. This trend was similar to results obtained by Chen et al.[Citation41] and Sasaki et al.[Citation42]
Table 4. Amylopectin chain length distribution of rice starches.
According to previous reports, an amylopectin chain length with at least 10 glucose units is required for crystallization (double helices formation).[Citation43] Therefore, starches with higher amount of short amylopectin chains could form weaker crystalline structure. Contrastly, starches containing higher amount of long amylopectin chains would result in stronger crystalline structure, since long chains facilitate long double helices production.[Citation44] A stronger crystalline structure needs a greater energy upon gelatinisation and allows more helices to re-align during retrogradation.[Citation45] It was reported that the gelatinisation temperature positively correlated with the ratio of amylopectin long chains (DP ≥ 37) and negatively correlated with short chains (DP6–12).[Citation44] Vamadevan et al.[Citation45] reported that amylopectin short external chains with DP 6–8 blocked retrogradation. In waxy maize and potato, no retrogradation enthalpies were detected when the external chains of amylopectin were less than 11.[Citation46] Altogether, it seemed reasonable that for each subspecies, the genotype containing a lower proportion of short A chains (DP 6–12) and higher ratio of long branch chains (DP ≥ 37) presented a faster rate of hardening and a higher temperature of gelatinisation in our study. The fine structure of amylopectin might have an impact on the physiochemical properties of waxy rice flours.
Conclusion
In our study, the physicochemical attributes of waxy rice varieties with different hardening rates were explored. The hardening rates of waxy rice flour prepared from yj98 and zaonuo5 were faster than those taken from zhenzhunuo and zhennuo29. The peak gelatinisation temperatures of rice flours were yj98 (78.60°C) > zaonuo5 (73.55°C) > zhennuo29 (69.00°C) > zhenzhunuo flours (67.25°C). The retrogradation enthalpies of stored flours were 3.876, 2.202, 1.684, and 1.370 J/g for yj98, zaonuo5, zhennuo29, and zhenzhunuo, respectively. After cool storage, zhenzhunuo and zhennuo29 cooked grains had no obvious changes in morphology, while zaonuo5 produced increased cavities and yj98 generated obvious fissures. Varieties with rapid hardening rates were found to possess high gelatinisation temperature and retrogradation enthalpy, but low water-holding capacity upon cool storage. The pasting property of rice flours seemed to have no apparent relations with the hardening attribute. Among intra-subspecies cultivars, the hardening rate of cooked rice grains was likely to be promoted by amylopectin extremely long chains (DP ≥ 37) and suppressed by amylopectin short chains (DP 6–12). Our results indicate that zhenzhuonuo and zhennuo29 are preferred for use in marking puddings, Tang yuan, and infant foods due to their slow hardening rates, while the zaonuo5 and yj98 flours are good choices for vermicelli, noodles, and sauces because of their rapid retrogradation rates. The physicochemical characteristics of the four cultivars revealed here will lay a foundation for future design of food processing operation in order to obtain high-quality products.
Disclosure statement
No potential conflict of interest was reported by the author(s).
Additional information
Funding
References
- Wang, W.; Wei, X.; Jiao, G.; Chen, W.; Wu, Y.; Sheng, Z.; Hu, S.; Xie, L.; Wang, J.; Tang, S. GBSS-BINDING PROTEIN , Encoding a CBM48 Domain-Containing Protein, Affects Rice Quality and Yield. J. Integr. Plant Biol. 2020, 62(7), 948–966. DOI: 10.1111/jipb.12866.
- Lian, X.; Wang, C.; Zhang, K.; Li, L. The Retrogradation Properties of Glutinous Rice and Buckwheat Starches as Observed with FT-IR, 13C NMR and DSC. Int. J. Biol. Macromol. 2014, 64, 288–293. DOI: 10.1016/j.ijbiomac.2013.12.014.
- Zhang, S.; Li, Z.; Lin, L.; Zhang, L.; Wei, C. Starch Components, Starch Properties and Appearance Quality of Opaque Kernels from Rice Mutants. Molecul. 2019, 24(24), 4580. DOI: 10.3390/molecules24244580.
- Bao, J.; Corke, H.; Sun, M. Genetic Diversity in the Physicochemical Properties of Waxy Rice (Oryza Sativa L) Starch. J. Sci. Food Agr. 2004, 84(11), 1299–1306. DOI: 10.1002/jsfa.1750.
- Tian, Z.; Qian, Q.; Liu, Q.; Yan, M.; Liu, X.; Yan, C.; Liu, G.; Gao, Z.; Tang, S.; Zeng, D. Allelic Diversities in Rice Starch Biosynthesis Lead to a Diverse Array of Rice Eating and Cooking Qualities. P. Natl. Acad. Sci. 2009, 106(51), 21760–21765. DOI: 10.1073/pnas.0912396106.
- Zeng, X.; Zheng, B.; Xiao, G.; Chen, L. Synergistic Effect of Extrusion and Polyphenol Molecular Interaction on the Short/long-Term Retrogradation Properties of Chestnut Starch. Carbohyd. Polym. 2022, 276, 118731. DOI: 10.1016/j.carbpol.2021.118731.
- Ding, L.; Zhang, B.; Tan, C. P.; Fu, X.; Huang, Q. Effects of Limited Moisture Content and Storing Temperature on Retrogradation of Rice Starch. Int. J. Biol. Macromol. 2019, 137, 1068–1075. DOI: 10.1016/j.ijbiomac.2019.06.226.
- Liu, Y.; Chen, J.; Wu, J.; Luo, S.; Chen, R.; Liu, C.; Gilbert, R. G. Modification of Retrogradation Property of Rice Starch by Improved Extrusion Cooking Technology. Carbohyd. Polym. 2019, 213, 192–198. DOI: 10.1016/j.carbpol.2019.02.089.
- Chang, Q.; Zheng, B.; Zhang, Y.; Zeng, H. A Comprehensive Review of the Factors Influencing the Formation of Retrograded Starch. Int. J. Biol. Macromol. 2021, 186, 163–173. DOI: 10.1016/j.ijbiomac.2021.07.050.
- Sung, S.; Kim, H. R.; Park, C. S.; Choi, S. J.; Moon, T. W. Structure and in vitro Digestion of Amylosucrase-Modified Waxy Corn Starch as Affected by Iterative Retrogradation. Int. J. Food. Prop. 2020, 23(1), 1176–1186. DOI: 10.1080/10942912.2020.1788580.
- Zeng, X.; Zheng, B.; Li, T.; Chen, L. How to Synchronously Slow Down Starch Digestion and Retrogradation: A Structural Analysis Study. Int. J. Biol. Macromol. 2022, 212, 43–53. DOI: 10.1016/j.ijbiomac.2022.05.099.
- Li, C.; Hu, Y.; Li, E. Effects of Amylose and Amylopectin Chain-Length Distribution on the Kinetics of Long-Term Rice Starch Retrogradation. Food Hydrocoll. 2021, 111, 106239. DOI: 10.1016/j.foodhyd.2020.106239.
- Yu, S.; Xu, J.; Zhang, Y.; Kopparapu, N. K. Relationship Between Intrinsic Viscosity, Thermal and Retrogradation Properties of Amylose and Amylopectin. Czech J. Food Sci. 2014, 32(5), 514–520. DOI: 10.17221/394/2013-CJFS.
- Li, C.; Luo, J. X.; Zhang, C. Q.; Yu, W. W. Causal Relations Among Starch Chain-Length Distributions, Short-Term Retrogradation and Cooked Rice Texture. Food Hydrocoll. 2020, 108, 106064. DOI: 10.1016/j.foodhyd.2020.106064.
- Li, C.; Hu, Y. Antagonistic Effects of Amylopectin and Amylose Molecules on the Starch Inter-And Intramolecular Interactions During Retrogradation. LWT-Food Sci. Technol. 2021, 148, 111942. DOI: 10.1016/j.lwt.2021.111942.
- Varavinit, S.; Shobsngob, S.; Varanyanond, W.; Chinachoti, P.; Naivikul, O. Effect of Amylose Content on Gelatinization, Retrogradation and Pasting Properties of Flours from Different Cultivars of Thai Rice. Starch/Stärke. 2003, 55(9), 410–415. DOI: 10.1002/star.200300185.
- Wang, Y. J.; Wang, L. Structures of Four Waxy Rice Starches in Relation to Thermal, Pasting, and Textural Properties. Cereal Chem. 2002, 79(2), 252–256. DOI: 10.1094/CCHEM.2002.79.2.252.
- Zhang, C.; Narayanamoorthy, S.; Ming, S.; Li, K.; Cantre, D.; Sui, Z.; Corke, H. Rheological Properties, Structure and Digestibility of Starches Isolated from Common Bean (Phaseolus Vulgaris L.) Varieties from Europe and Asia. LWT-Food Sci. Technol. 2022, 161, 113352. DOI: 10.1016/j.lwt.2022.113352.
- Han, H.; Yang, C.; Zhu, J.; Zhang, L.; Bai, Y.; Li, E.; Gilbert, R. G. Competition Between Granule Bound Starch Synthase and Starch Branching Enzyme in Starch Biosynthesis. Rice. 2019, 12(1), 96. DOI: 10.1186/s12284-019-0353-3.
- Wu, A. C.; Li, E.; Gilbert, R. G. Exploring Extraction/Dissolution Procedures for Analysis of Starch Chain-Length Distributions. Carbohyd. Polym. 2014, 114, 36–42. DOI: 10.1016/j.carbpol.2014.08.001.
- Yang, H.; Tang, M.; Wu, W.; Ding, W.; Ding, B.; Wang, X. Study on Inhibition Effects and Mechanism of Wheat Starch Retrogradation by Polyols. Food Hydrocoll. 2021, 121, 106996. DOI: 10.1016/j.foodhyd.2021.106996.
- Abdel‐Aal, E. S.; Hucl, P.; Chibbar, R.; Han, H.; Demeke, T. Physicochemical and Structural Characteristics of Flours and Starches from Waxy and Nonwaxy Wheats. Cereal Chem. 2002, 79(3), 458–464. DOI: 10.1094/CCHEM.2002.79.3.458.
- Cuevas, R. P. O.; Domingo, C. J.; Sreenivasulu, N. Multivariate-Based Classification of Predicting Cooking Quality Ideotypes in Rice (Oryza Sativa L.) Indica Germplasm. Rice. 2018, 11(1), 56. DOI: 10.1186/s12284-018-0245-y.
- Zhang, C.; Yang, Y.; Chen, Z.; Chen, F.; Pan, L.; Lu, Y.; Li, Q.; Fan, X.; Sun, Z.; Liu, Q. Characteristics of Grain Physicochemical Properties and the Starch Structure in Rice Carrying a Mutated ALK/SSIIa Gene. J. Agric. Food. Chem. 2020, 68(47), 13950–13959. DOI: 10.1021/acs.jafc.0c01471.
- Du, C.; Jiang, F.; Jiang, W.; Ge, W.; Du, S. K. Physicochemical and Structural Properties of Sago Starch. Int. J. Biol. Macromol. 2020, 164, 1785–1793. DOI: 10.1016/j.ijbiomac.2020.07.310.
- Zhu, S.; Hu, F.; Ramaswamy, H.; Yu, Y.; Yu, L.; Zhang, Q. Effect of High Pressure Treatment and Degree of Milling on Gelatinization and Structural Properties of Brown Rice. Food Bioprocess. Tech. 2016, 9(11), 1844–1853. DOI: 10.1007/s11947-016-1770-6.
- Zhang, C.; Xu, Z.; Liu, X.; Ma, M.; Khalid, S.; Bordiga, M.; Sui, Z.; Corke, H. Removing Starch Granule-Associated Surface Lipids Affects Structure of Heat-Moisture Treated Hull-Less Barley Starch. Carbohyd. Polym. 2023, 303, 120477. DOI: 10.1016/j.carbpol.2022.120477.
- Singh, S.; Singh, N.; Isono, N.; Noda, T. Relationship of Granule Size Distribution and Amylopectin Structure with Pasting, Thermal, and Retrogradation Properties in Wheat Starch. J. Agric. Food. Chem. 2010, 58(2), 1180–1188. DOI: 10.1021/jf902753f.
- Xu, L.; Xie, J.; Kong, X.; Bao, J. Analysis of Genotypic and Environmental Effects on Rice Starch. 2. Thermal and Retrogradation Properties. J. Agric. Food. Chem. 2004, 52(19), 6017–6022. DOI: 10.1021/jf049235a.
- Madruga, M. S.; de Albuquerque, F. S. M.; Silva, I. R. A.; Do Amaral, D. S.; Magnani, M.; Neto, V. Q. Chemical, Morphological and Functional Properties of Brazilian Jackfruit (Artocarpus Heterophyllus L.) Seeds Starch. Food Chem. 2014, 143, 440–445. DOI: 10.1016/j.foodchem.2013.08.003.
- Zhang, C.; Ma, M.; Xu, Y.; Xu, Z.; Sui, Z.; Corke, H. Octenyl Succinic Anhydride Modification Alters Blending Effects of Waxy Potato and Waxy Rice Starches. Int. J. Biol. Macromol. 2021, 190, 1–10. DOI: 10.1016/j.ijbiomac.2021.08.113.
- Fang, S.; Chen, C.; Yao, Y.; Nsor-Atindana, J.; Liu, F.; Chen, M.; Zhong, F. Study on the Pasting Properties of Indica and Japonica Waxy Rice. Foods. 2022, 11(8), 1132. DOI: 10.3390/foods11081132.
- Mao, H.; Li, J.; Chen, Z.; Yan, S.; Li, H.; Wen, Y.; Wang, J. Molecular Structure of Different Prepared Pyrodextrins and the Inhibitory Effects on Starch Retrogradation. Food. Res. Int. 2021, 143, 110305. DOI: 10.1016/j.foodres.2021.110305.
- Ma, M.; He, M.; Xu, Y.; Li, P.; Li, Z.; Sui, Z.; Corke, H. Thermal Processing of Rice Grains Affects the Physical Properties of Their Pregelatinised Rice Flours. Int J. Food Sci. Tech. 2020, 55(3), 1375–1385. DOI: 10.1111/ijfs.14417.
- Navaf, M.; Sunooj, K. V.; Aaliya, B.; Sudheesh, C.; Akhila, P. P.; Sabu, S.; Sasidharan, A.; George, J. Talipot Palm (Corypha Umbraculifera L.) a Nonconventional Source of Starch: Effect of Citric Acid on Structural, Rheological, Thermal Properties and in vitro Digestibility. Int. J. Biol. Macromol. 2021, 182, 554–563. DOI: 10.1016/j.ijbiomac.2021.04.035.
- Srichuwong, S.; Sunarti, T. C.; Mishima, T.; Isono, N.; Hisamatsu, M. Starches from Different Botanical Sources I: Contribution of Amylopectin Fine Structure to Thermal Properties and Enzyme Digestibility. Carbohyd. Polym. 2005, 60(4), 529–538. DOI: 10.1016/j.carbpol.2005.03.004.
- Li, H.; Liu, Y. Effects of Variety and Growth Location on the Chain-Length Distribution of Rice Starches. J. Cereal Sci. 2019, 85, 77–83. DOI: 10.1016/j.jcs.2018.11.009.
- Wang, K.; Wambugu, P. W.; Zhang, B.; Wu, A. C.; Henry, R. J.; Gilbert, R. G. The Biosynthesis, Structure and Gelatinization Properties of Starches from Wild and Cultivated African Rice Species (Oryza Barthii and Oryza glaberrima). Carbohyd. Polym. 2015, 129, 92–100. DOI: 10.1016/j.carbpol.2015.04.035.
- Obadi, M.; Li, C.; Li, Q.; Li, X.; Qi, Y.; Xu, B. Relationship Between Starch Fine Molecular Structures and Cooked Wheat Starch Digestibility. J. Cereal Sci. 2020, 95, 103047. DOI: 10.1016/j.jcs.2020.103047.
- Hanashiro, I.; Abe, J. I.; Hizukuri, S. A Periodic Distribution of the Chain Length of Amylopectin as Revealed by High-Performance Anion-Exchange Chromatography. Carbohyd. Res. 1996, 283, 151–159. DOI: 10.1016/0008-6215(95)00408-4.
- Chen, J.; Watanabe, M.; Nakamori, T.; Hisamatsu, M. Relationship Between Physical Properties and Amylopectin Structure of Waxy Rice Starch. J. Appl. Glyosci. 2003, 50(2), 133–137. DOI: 10.5458/jag.50.133.
- Sasaki, T.; Kawamata, K.; Okamoto, K. Comparison of Starch Physicochemical Properties of Waxy Rice Cultivars with Different Hardening Rates. Cereal Chem. 2017, 94(4), 699–704. DOI: 10.1094/CCHEM-08-16-0220-R.
- Robin, F.; Mérinat, S.; Simon, A.; Lehmann, U. Influence of Chain Length on α-1,4-D-Glucan Recrystallization and Slowly Digestible Starch Formation. Starch - Stärke. 2008, 60(10), 551–558. DOI: 10.1002/star.200800220.
- Park, I. M.; Ibáñez, A. M.; Zhong, F.; Shoemaker, C. F. Gelatinization and Pasting Properties of Waxy and Non‐Waxy Rice Starches. Starch - Stärke. 2007, 59(8), 388–396. DOI: 10.1002/star.200600570.
- Vamadevan, V.; Bertoft, E. Impact of Different Structural Types of Amylopectin on Retrogradation. Food Hydrocoll. 2018, 80, 88–96. DOI: 10.1016/j.foodhyd.2018.01.029.
- Würsch, P.; Gumy, D. Inhibition of Amylopectin Retrogradation by Partial Beta-Amylolysis. Carbohyd. Res. 1994, 256(1), 129–137. DOI: 10.1016/0008-6215(94)84232-9.