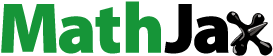
ABSTRACT
The objective of this experiment was to select D. hansenii isolates that are stable in dry fermented sausage (DFS) production and can positively affect the quality. D. hansenii isolates were examined for production of biogenic amines, β-glucuronidase, β-glucosidase, proteolytic, and lipolytic enzymatic activities. The acid and salt tolerance of D. hansenii isolates were also examined. DFS inoculated with selected D. hansenii isolates were analyzed for microorganisms, weight loss, Aw, and pH. DFS was also subjected to analyses of color, texture profile, reducing sugar, volatile basic nitrogen (VBN), lipid oxidation, and free fatty acid. Twenty-two D. hansenii isolates were isolated. None of these D. hansenii isolates produced biogenic amines, and all strains were negative for β-glucuronidase and β-glucosidase activities. After examining these strains for proteolysis, lipolysis, and salt and acid tolerance, D. hansenii isolates SMFM2021-C14, SMFM2021-S8, and SMFM2021-SL3 were selected to be used in DFS. During the processing of DFS, the groups treated with D. hansenii isolate SMFM2021-S8 or SMFM2021-SL3 showed lower (p < .05) hardness values of DFS. The sample treated with D. hansenii SMFM2021-S8 showed lower (p < .05) VBN and lipid oxidation contents than the others. The ratio of ω-6/ω-3 was lower (p < .05) in D. hansenii SMFM2021-SL3 treated group than in the other treatments. These results indicate that D. hansenii isolates SMFM2021-S8 and SMFM2021-SL3 might be useful in producing DFS to improve its quality.
Introduction
Fermentation occurs by microorganisms and enzymes in diverse fermented foods, and the microorganisms in fermented foods are mold, yeasts, and bacteria[Citation1,Citation2]. Thus, selecting and securing desired isolates from various microbial floras in fermented foods is important. Microorganisms generate the unique flavor and texture of dry fermented sausage (DFS) during fermentation and aging processes. The inoculation of microorganisms is essential for the production of DFS. Microorganisms used in a starter include lactic acid bacteria (LAB) and fungi. The main role of LAB in fermented sausage is to lower the pH of food through lactic acid production by sugar fermentation.[Citation3,Citation4] Fibrillar protein can coagulate in decreased pH, thus improving firmness and cohesiveness.[Citation5,Citation6] In addition, acetic acid, formic acid, and succinic acid produced by LAB can give sausages a unique flavor.[Citation7] Due to the production of antibacterial metabolites such as organic acids, hydrogen peroxide, diacetyl, and bacteriocin, pathogenic bacteria are suppressed.[Citation8]
Kim and Ahn[Citation9] reported that fermented sausages prepared by mixing grapefruit extract and kimchi-derived LAB showed significantly lower numbers of Escherichia coli and lipid oxidation. Yoon and Kim[Citation10] showed that Listeria monocytogenes counts decreased by about 1 Log CFU/mL in whole milk 9 h after the inoculation of the isolated Lactococcus lactis and a commercial starter. This result may suggest that a mixed culture of microorganisms effectively inhibits the growth of harmful bacteria by the antagonistic effect.[Citation11] Kim et al.[Citation12] showed that lipid oxidation was the lowest when sausages were inoculated with encapsulated Bifidobacterium longum isolated from infant feces. Yoo et al.[Citation13] selected starter isolates from traditional fermented foods and natural products with excellent growth, pH lowering, total acid production, and nitrite scavenging.
Although fungi are known to play roles in producing flavor-related fatty acids and amino acids, studies applying fungi to fermented meat products are still insufficient.[Citation14] Thus, whether fungi can improve the quality of DFS needs to be studied. Yeast is prominently present among fermented foods, and microorganisms from fermented foods might be expected to be more stable under fermentation conditions. Previous studies have reported the isolation of D. hansenii from fermented foods[Citation15,Citation16] and D. hansenii is one of the most abundant yeasts in dried meat products.[Citation17] Yeasts in fermented sausages can protect sausages from oxidation and humidity changes and facilitate proper drying.[Citation18] They may enhance the flavor of sausages through lipolysis or protein degradation.[Citation19] They can also produce ethyl ester compounds to create a unique flavor.[Citation20] Andrade et al.[Citation21] have reported that using D. hansenii in meat products can shorten the aging period and positively affect color and flavor. D. hansenii is listed in the Qualified Presumption of Safety (QPS) status by the European Food Safety Authority (EFSA).[Citation22,Citation23] Unlike molds, it did not produce mycotoxins and inhibited the biosynthesis of mycotoxin at the transcriptional level by absorbing ochratoxin A on the cell wall.[Citation24] Accordingly, Andrade et al.[Citation25] mentioned that D. hansenii can be used as a biopreservative to inhibit the growth of ochratoxigenic molds in dry-cured products. However, some D. hansenii isolates have been found to produce biogenic amines, and properties such as enzyme activity vary according to the isolates; it is important to select an appropriate D. hansenii isolate for the DFS application.[Citation26] Therefore, the objectives of this study were to isolate D. hansenii from fermented foods and select isolates to improve the quality of DFS, which may contribute to selecting appropriate microbial resources in DFS.
Materials and methods
Isolation and identification of D. hansenii
Forty-three fermented food samples [Meju (4), Nuruk (5), soybean paste (7), Gochujang (3), Makgeolli (1), wine (1), fermented sausage (7), tenderloin of dry-aged beef (3), and cheese (12)] were used for the isolation. The sample (25 g) was transferred aseptically into a sample bag containing 50 mL peptone water (Becton, Dickinson and Company, Sparks, MD, USA) and homogenized in a pummeler (BagMixer, Interscience, St. Nom, France) for 120 sec. The homogenates were serially diluted, and the diluents were spread-plated onto yeast media. The yeast media used for the isolation were potato dextrose agar (PDA; Becton, Dickinson and Company), maltose extract agar (MEA; Becton, Dickinson and Company), yeast extract peptone dextrose agar [YPD agar; yeast extract (1% w/v), peptone (2% w/v), dextrose (2% w/v), and agar (1.5% w/v)], YPD agar with 10% NaCl, and YPD agar with chloramphenicol (0.1 mg/mL). Plates were incubated at 25°C for 2–7 days. D. hansenii, which formed morphologically smooth white colonies, was isolated from the medium and streaked onto the PDA with a sterile loop. Plates were incubated at 25°C for 2–3 days. Isolated colonies on the plates were then sequenced and identified by Cosmo Genetech (Seoul, Korea) with primers NL1 (GCATATCAATAAGCGGAGGAAAAG) and NL4 (GGTCCGTGTTTCAAGACGG).[Citation27] Polymerase chain reaction conditions were as follows: an initial denaturation step at 94°C for 5 min; 35 cycles at 94°C for 30 sec, 58°C for 30 sec, and 72°C for 1 min and 30 sec; and a final extension step at 72°C for 10 min.
Safety analyses of D. hansenii isolates
Biogenic aine (BA) production
According to a method by Niven et al.,[Citation28] activated D. hansenii culture (1 mL) was inoculated into yeast mold broth (YM broth; Becton, Dickinson and Company) supplemented with cresol purple (0.006%, w/v), glycerol (0.35%, w/v), and precursor amino acid (0.1%, w/v) and cultured at 25°C for 3 days. After 3 days of incubation, the culture was homogenized and centrifuged at 15,814×g at 4°C for 5 min. The supernatant (200 μL) was placed into 96 well-microtiter plates (SPL, Pocheon, Gyeonggi-do, Korea), and absorbance was measured at 590 nm with EpochTM Microplate Spectrophotometer (BioTek Instruments, Winooski, VT, USA). Potato dextrose broth (PDB; Becton, Dickinson and Company) was used as a negative control.
β-glucuronidase and β-glucosidase activities
API ZYM kit (BioMérieux, Marcy-l’Etoile, Auvergne-Rhône-Alpes, France) was used to examine productions of β-glucuronidase and β-glucosidase in the isolates. This experiment was conducted according to the manufacturer’s instructions and a method by Ramos et al.[Citation29] The optical density (OD) of the isolated D. hansenii cultures was adjusted to 0.5 at 600 nm. After pouring about 5 mL of sterile distilled water into a tray to maintain moisture, 65 μL of the diluted D. hansenii culture was dispensed into the cupule and incubated at 30°C for 3 h. Distilled water was used as a negative control, and the results were then read according to the manufacturer’s protocol.
Cytotoxicity
This experiment was conducted as described by Gerlier and Thomasset.[Citation30] Raw 264.7 cells (mouse macrophages) were cultured in Dulbecco’s modified Eagle’s medium (DMEM; Welgene, Gyeongsan, Gyeongsangbuk-do, Korea) supplemented with 1% penicillin–streptomycin (Gibco BRL, Life Technologies, Grand Island, NY, USA) and 10% fetal bovine serum (Gibco BRL) at 37°C with 5% CO2. These cells were seeded into wells of 96 well-microtiter plates at a density of 104 cells/mL and incubated at 37°C with 5% CO2. When the cells were attached, the supernatant was removed, and a mixture of isolated D. hansenii culture (20 μL; 7–8 Log CFU/mL) with 180 μL DMEM was inoculated to Raw 264.7 cells.[Citation31] Raw 264.7 cells treated with D. hansenii isolates were incubated at 37°C with 5% CO2 for 48 h. After the incubation, the supernatant was removed, and Raw 264.7 cells were washed twice with Dulbecco’s phosphate buffered saline (DPBS; Welgene). Then, 20 μL of 3-(4,5-Dimethyl-2-thiazolyl)-2,5-diphenyl-2 H-tetrazolium bromide (MTT, Sigma-Aldrich, St. Louis, MO, USA) solution (5 mg/mL) was added to each well. After the microtiter plate was incubated at 37°C with 5% CO2 for 4 h, the MTT reagent was removed, and Raw 264.7 cells were washed twice with DPBS to remove residual MTT reagent. Each well was filled with 200 μL of dimethylsulfoxide (Samchun, Gangnam-gu, Seoul, Korea) and mixed by pipetting. The absorbance was then measured at 540 nm with EpochTM Microplate Spectrophotometer. The absorbance was compared to the absorbance of the negative control (PBS) to determine the survival rate.[Citation32]
Hemolysis
The D. hansenii cultures were streaked onto Columbia agar (BioMérieux) supplemented with 5% sheep blood and incubated at 25°C for 48 h. Clear zones around colonies on the medium were determined as positive for hemolysis.[Citation33]
Analyses of D. hansenii isolates for factors-related dry fermented sausage production
Proteolysis activity
After adjusting the OD of D. hansenii cultures to 1.0 at 600 nm, the diluted D. hansenii cultures were then spotted onto a proteolysis medium prepared by adding 5% skim milk to yeast extract (5 g/L) and agar (15 g/L). The plates were incubated at 25°C for 4 days to observe a clear zone around the spot, indicating proteolysis.[Citation34]
Lipolysis activity
The diluted D. hansenii cultures (OD600 = 1.0) were streaked onto a lipolysis medium. The medium was composed of tryptone (8 g/L), yeast extract (4 g/L), NaCl (3 g/L), olive oil (15 mL/L), rhodamine B (2 mg/L), and agar (20 g/L) in 1 L of distilled water. The plates were then incubated at 25°C for 4 days. After the incubation, the fluorescence was visualized under UV light. The fluorescence indicates that the D. hansenii isolate has lipolysis.[Citation35]
Acidity and salt tolerance
A model system of the dry fermented sausage was prepared with beef extract (12 g/L), glucose (10 g/L), NaCl (20 g/L), dipotassium hydrophosphate (2 g/L), MgSO4·7 H2O (0.15 g/L), and nitrite (0.15 g/L)Citation13] The pH was adjusted to 3.5 with 1 N HCl. Culture (1 mL) of D. hansenii isolate was inoculated into the model system at 6–7 Log CFU/mL. Then, 0.1 mL of this mixture was immediately plated onto PDA to enumerate cell counts of D. hansenii isolate on day 0. Subsequently, the inoculated model system was incubated at 25°C for 13 days, and 0.1 mL of the sample was then plated on PDA. Plates were then incubated at 25°C for 48 h. After the incubation, colonies on the PDA were counted manually, and survivals were estimated by comparing cell counts of day 13 to those of day 0. To evaluate salt tolerance, D. hansenii culture (OD600 = 1.0) was serially diluted, and 10 μL of each diluent was spotted onto YPD agar supplemented with 2 M NaCl.[Citation36] Plates were incubated at 25°C for 4 days to observe the growth of colonies on the plates, and isolates formed colonies at the highest dilution were selected for DFS production.
Application of D. hansenii isolate to dry fermented sausages
Microbial analysis
Preparation of DFS and inoculation of D. hansenii: DFS was prepared according to the procedure described in astudy by RDA[Citation37] with brief modification. Visible connective tissues, subcutaneous fat, and intramuscular fat of pork were trimmed. Lean meat of pork leg and pork back fat were minced through a3-mm plate of amincer machine (PM-70, Mainca, Barcelona, Spain). Minced meat (80%), fat (20%), starter culture [0.025%; Pediococcus pentosaceus and Staphylococcus xylosus (T-SPX, Chr. Hansen. HHørsholm, Denmark)], D. hansenii isolates (7 Log CFU/mL; SMFM2021-C14, SMFM2021-S8, and SMFM2021-SL3), and other ingredients [salt (2%), sucrose (1.5%), black pepper (0.2%), sodium polyphosphate (0.2%), and ascorbate (0.03%)] were mixed in asilent cutter (Cutter C4 VV, SIRMAN, Venezia, Italy) for 6 min. Meat batters were stuffed into 26-mm collagen casings (NIPPI Inc., Tokyo, Japan) with astuffer (IS-8, SIRMAN, Marsango, Italy). Thereafter, the prepared sausages were dried at 20°C for 48 h with humidity of 85% or at 15°C for 19 days under 80% humidity in adry ager (Venix Co., Ltd., Fornace, Resena, Italy). Control was not inoculated with D. hansenii. The experiment was repeated three times, and 2 kg of DFS was prepared for each experimental group.
The DFS sample (10 g) was aseptically transferred into a sample bag containing 20 mL of 0.1% buffered peptone water (BPW; Becton, Dickinson and Company). The sample was then homogenized in a pummeler for 1 min. To enumerate foodborne pathogens, the homogenate was plated onto 3 M™ Petrifilm™ E. coli/Coliform Count Plates (3 M, St. Paul, Minnesota, USA) for E. coli and xylose lysine deoxycholate (XLD; Becton, Dickinson and Company) plates for Salmonella. These plates were incubated at 37°C for 24 h. Homogenates were also spread-plated onto Palcam agar (Oxoid Ltd., Basingstoke, Hampshire, England) plates for L. monocytogenes and incubated at 30°C for 48 h. The homogenates were spread-plated onto Baird-Parker agar (BPA; MBcell, Kisan Bio, Seocho-gu, Seoul, Korea) for S. xylosus and on De Man, Rogosa, and Sharpe agar (MRS agar; Becton, Dickinson and Company) for P. pentosaceus. These plates were incubated at 37°C for 24 h. The homogenates were also spread-plated onto PDA for yeast and incubated at 25°C for 48 h. Typical colonies on each plate were manually counted.
Weight loss
The weight of DFS was measured every week. The weight of the sample at different time points was compared to the weight at week 0 to calculate the weight loss with the following equation;
Water activity (Aw)
DFS (5 g) was ground weekly and placed in a plastic shell up to 70%. Aw of the sample was measured with a water activity meter (AQS-31-TC, NAGY, Siedlerstrabe, Gaufelden, Germany).
pH
DFS (10 g) was transferred into a sample bag containing 20 mL of 0.1% BPW. It was then homogenized in a pummeler for 60 sec. The pH of the homogenate was measured with a pH meter (Thermo Fisher Scientific Inc., Waltham, MA, USA).
Reducing sugar
This experiment was conducted as described by Jayasena et al.[Citation38] After adding 5 mL of 80% ethanol (50°C) to 1 g of the sample in a sample bag, the sample was homogenized in a pummeler for 1 min. The homogenate was transferred to a 15-mL conical tube and then centrifuged at 2,265×g for 10 min. After centrifugation, the upper layer was filtered with filter paper (No.1, Whatman plc, Maidstone, Kent, England). The filtrate was concentrated with nitrogen. Distilled water (2 mL) was added to the concentrated sample, and the sugar component was extracted by centrifuging the concentrated sample at 10,000×g at 4°C for 10 min. After transferring 1 mL of the extract to the test tube, 2 mL of DNS reagent (150 g Rochelle salt, 8 g NaOH, and 0.5 g dinitrosalicylic acid/500 mL distilled water) was added to 1 mL extractant. This mixture was heated in a water bath at 90°C for 15 min. It was then cooled in ice water to stop the reaction, followed by measuring OD550 with Epoch™ Microplate Spectrophotometer. The OD550 value was used to estimate the amount of reducing sugar with glucose (Samchun) standard curve. The standard curve was prepared by adding a DNS reagent (2 mL) to 1 mL of a glucose solution at 0–1 mg/mL.
Volatile basic nitrogen (VBN)
VBN of DFS was measured by a method based on MFDS.[Citation39] Distilled water (27 mL) was added to 3 g of DFS in a sample bag. The sample was then homogenized for 30 sec in a pummeler. The homogenate was left at room temperature for 30 min and filtered through filter paper (Advantec No.131, Chiyoda, Tokyo, Japan). One milliliter of 0.01 N sulfuric acid solution was placed in the inner chamber of the Conway dish, and 1 mL of the filtrate and 1 mL of saturated potassium carbonate solution were placed in the outer chamber of the Conway dish. Conway dish was sealed and left at 25°C for 1 h. After 1 h of reaction, the cover of the Conway dish was opened and 10 µL of Brunswik’s solution was placed into the inner chamber. The VBN captured in the sulfuric acid solution of the inner chamber was titrated with 0.01 N NaOH. The VBN content (mg%) was calculated according to the following equation[Citation39]:
where b is blank, a is a sample, f is a factor of 0.01 N NaOH, and W is the sample weight.
Lipid oxidation
Thiobarbituric acid reactive substances (TBARS) were measured according to a method by Witte et al.[Citation40] to analyze lipid oxidation. After adding 9 mL of distilled water to 3 g of DFS in a sample bag, the sample was homogenized in a pummeler for 1 min. The homogenate was filtered through filter paper (No. 1, Whatman). The filtrate (1 mL) was mixed with 2 mL reaction reagent. The reaction reagent was made by mixing 20 mM 2-thiobarbituric acid (Sigma-Aldrich) and 20% trichloroacetic acid (Daejung Chemicals & Metals, Siheung, Gyeonggi-do, Korea). The reactant was placed in a 100°C water bath for 15 min. It was then placed in cold water to stop the reaction. The reaction mass was centrifuged at 15,814×g for 5 min. The supernatant was collected, and its absorbance was measured at 531 nm with Epoch™ Microplate Spectrophotometer. The result was expressed as the amount of mg malonaldehyde per kg (mg MAD/kg) of the sample by multiplying the absorbance by a constant of 5.2.
Color
After cutting 20 g of DFS out with a knife decontaminated with 70% ethanol, it was left at room temperature for 1 h. Lightness (L*), redness (a*), and yellowness (b*) values of the sample were then measured with a colorimeter (Chroma meter Model CR-200b, Minolta Camera Co., Chiyoda, Tokyo, Japan). The standard white plate was measured after standardizing it to Y = 84.6, x = 0.3167, and y = 0.3232 values according to the manual of the instrument.
Texture profile analysis
The DFS sample (20 g) was cut with a knife, decontaminated with 70% ethanol, into a cylindrical shape with a height of 20 mm, and analyzed with a texture analyzer (TA-XT Express, Stable Micro System LTD., Haslemere, Surrey, England). The analyzer was programmed with the following parameters; 5 mm/sec of pretest speed, 5 mm/sec of test speed, 5 mm/sec of posttest speed, 5 mm of distance, 5 sec, and 10 g of trigger force. The hardness, gumminess, springiness, and chewiness of the sample were measured with a cylindrical probe (Ø75 mm) in the texture analyzer.
Free fatty acid analysis
Free fatty acids in DFS were analyzed according to the procedure of the Food Code.[Citation39] Briefly, 1 g of each sample was placed into a Mojonnier tube. Then, 2 mL of pyrogallol (50 mg/mL in ethanol), a boiling chip, and an internal standard solution were added to the sample. After washing the sample on the stopper of the Mojonnier tube with 10 mL of 8.3 M hydrochloric acid solution, the tube was sealed, stirred with an appropriate speed, and placed at 70–80°C for 40 min. After cooling the Mojonnier tube at room temperature for 1 h, the lower part of the Mojonnier tube was filled with ethanol and mixed gently to facilitate separation during ether extraction. After adding 25 mL of diethyl ether to the Mojonnier tube and shaking the tube for 5 min, 25 mL of anhydrous petroleum ether was added, followed by shaking for 5 min. Thereafter, the ether layer was separated and concentrated with nitrogen at 35–40°C. Concentrated fat was transferred to a 15-mL test tube with the Pasteur pipette. The remaining fat was washed with 3 mL of diethyl ether and added to the test tube. After concentrating the solution in the test tube with a nitrogen concentrator at 40°C, 2 mL of 7% BF3-methanol and 1 mL of toluene were added to the test tube. It was heated at 100°C for 45 min and cooled at room temperature. Distilled water (5 mL), 1 mL of isooctane, and 1 g of anhydrous sodium sulfate were added to the concentrate. After centrifuging this mixture at 374×g for 5 min, the supernatant was filtered and used as a test solution. Thirty-seven fatty acid standards (FAME MIX C4-C-24 2, 100 mg/mL) were dissolved in isooctane and used as a standard solution. Triundecanoin (5 mg/mL in chloroform) was used as the internal standard solution. The content of each fatty acid for the test solution was analyzed using a gas chromatography analyzer (HP 7890; Agilent Technologies, Santa Clara, CA, USA) with a split ratio of 100:1. A capillary column (SP-2650; 100 m × 0.25 mm × 0.2 µm, Agilent Technologies) was used to analyze samples for individual fatty acid. The injector and detector temperatures were maintained at 225°C and 282°C, respectively. Column oven temperatures were set as follows: 100°C for 4 min and increased to 240°C at 3°C/min for 20 min.
Statistical analysis
All experiments were repeated three times. The data were analyzed with the general linear procedure of SASⓇ version 9.4 (SAS Institute Inc., Cary, NC, USA). Significant differences among the least square means of treatment were determined with pairwise t-test at α = 0.05.
Results
Isolation and identification of yeasts
Seventy-one yeast colonies were isolated from 43 fermented food samples. Of these colonies, 22 D. hansenii isolates were identified by 26s rRNA sequencing, and they were isolated from eight cheeses, four fermented sausage, one soybean paste, and one dry-aged meat (). Other Debaryomyces species, Wickerhamomyces anomalus, Zygosaccharomyces rouxii, Saccharomyces cerevisiae, and Candida zeylanoides were also identified from yeast colonies ().
Table 1. Information of Debaryomyces hansenii isolates from fermented foods.
Table 2. Information of non-Debaryomyces hansenii isolates from fermented foods.
Analysis and selection of D. hansenii isolates
Isolates of D. hansenii that are negative for biogenic amine production and β-glucuronidase and β-glucosidase activity exhibited high enzyme activity related to the improvement of fermented sausage quality and are stable in the fermented sausage environment were selected.
Biogenic amine production, and β-glucuronidase and β-glucosidase activity
Biogenic amine production, and β-glucuronidase and β-glucosidase activity: For 22 D. hansenii isolates, there was no difference in absorbance between groups treated with D. hansenii and the negative control (PDB) (data not shown). In addition, all D. hansenii isolates did not show a violet color in the API ZYM kit. Thus, they were negative for β-glucuronidase and β-glucosidase activity (data not shown).
Proteolysis and lipolysis activity
All D. hansenii isolates did not form clear zones in the proteolysis medium (data not shown), indicating that no D. hansenii isolates have proteolysis activity. Eleven isolates (D. hansenii isolates SMFM2021-C3, SMFM2021-C5, SMFM2021-C8, SMFM2021-C9, SMFM2021-C12, SMFM2021-C14, SMFM2021-S3, SMFM2021-S5, SMFM2021-SL1, SMFM2021-SL3, and SMFM2021-D1) of the 22 D. hansenii isolates showed relatively strong luminescence in the lipolysis medium and 6 isolates (D. hansenii SMFM2021-C10, SMFM2021-C15, SMFM2021-S2, SMFM2021-S6, SMFM2021-S8, and SMFM2021-SL2) showed moderate luminescence (). Thus, it was shown that D. hansenii isolates SMFM2021-C3, SMFM2021-C5, SMFM2021-C8, SMFM2021-C9, SMFM2021-C10, SMFM2021-C12, SMFM2021-C14, SMFM2021-C15, SMFM2021-S2, SMFM2021-S3, SMFM2021-S5, SMFM2021-S6, SMFM2021-S8, SMFM2021-SL1, SMFM2021-SL2, SMFM2021-SL3, and SMFM2021-D1 had the lipolysis activity.
Table 3. Lipolytic enzyme activity of Debaryomyces hansenii isolates.
Acid and NaCl tolerance
All D. hansenii isolates grew at pH 3.5 (). Ten (D. hansenii SMFM2021-C9, SMFM2021-C10, SMFM2021-C12, SMFM2021-C14, SMFM2021-D1, SMFM2021-S2, SMFM2021-S8, SMFM2021-SL1, SMFM2021-SL2, and SMFM2021-SL3) of D. hansenii isolates showed excellent growth rates in the model system of DFS. Thus, they were further analyzed for NaCl tolerance. Among the 10 D. hansenii isolates, 6 (SMFM2021-C9, SMFM2021-C12, SMFM2021-C14, SMFM2021-S8, SMFM2021-SL1, and SMFM2021-SL3) of D. hansenii isolates showed better growth than the other isolates in YPD agar supplemented with 2 M NaCl (). Then, the top three isolates (D. hansenii SMFM2021-C14, SMFM2021-S8, and SMFM2021-SL3) in the tolerance were selected, and cytotoxicity and hemolysis were analyzed.
Figure 1. Growth rates of Debaryomyces hansenii isolates at 25°C under acidic environment at pH 3.5.
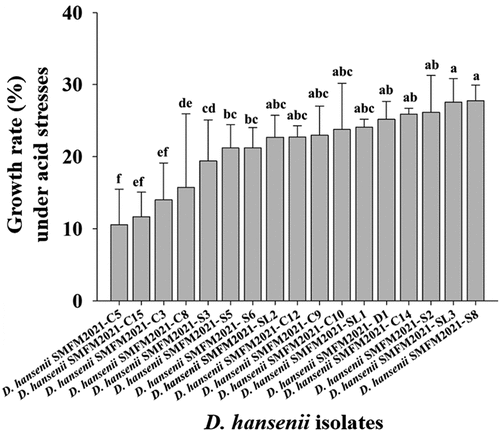
Figure 2. Tolerance of Debaryomyces hansenii isolates to 2 M NaCl stresses (C9: D. hansenii SMFM2021-C9; C10: D. hansenii SMFM2021-C10; C12: D. hansenii SMFM2021-C12; C14: D. hansenii SMFM2021-C14; SL1: D. hansenii SMFM2021-SL1; SL2: D. hansenii SMFM2021-SL2; SL3: D. hansenii SMFM2021-SL3; D1: D. hansenii SMFM2021-D1; S2: D. hansenii SMFM2021-S2; S8: D. hansenii SMFM2021-S8).
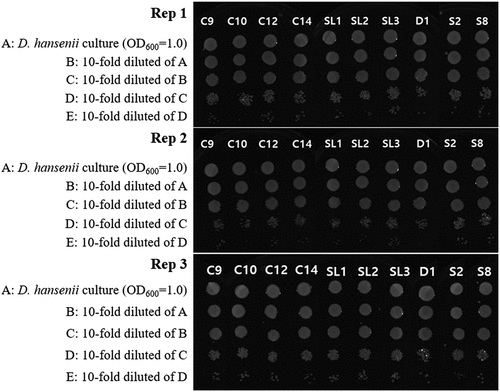
Cytotoxicity
The cytotoxicity of D. hansenii isolates was determined by measuring the survival rate of Raw 264.7 cells treated with D. hansenii isolates SMFM2021-C14, SMFM2021-S8, and SMFM2021-SL3. Treated groups showed no significant differences in cell survival compared to the negative control (data not shown). Our results indicate that D. hansenii isolates SMFM2021-C14, SMFM2021-S8, and SMFM2021-SL3 might not have cytotoxicity.
Hemolysis
Hemolysis is categorized into alpha (α) hemolysis, in which red blood cells and hemoglobin are partially dissolved; beta (β) hemolysis, in which red blood cells and hemoglobin are completely dissolved; and gamma (γ) hemolysis, which does not show hemolysis.[Citation41] D. hansenii isolates SMFM2021-C14, SMFM2021-S8, and SMFM2021-SL3 did not form clear zones in Columbia agar, indicating that these isolates had no hemolysis activity (data not shown). Therefore, D. hansenii isolates SMFM2021-C14, SMFM2021-S8, and SMFM2021-SL3 were selected to be used in DFS.
Analyses of dry fermented sausage
Microbial analysis
On week 0, P. pentosaceus and S. xylosus counts in sausage samples were similar among the treatments, which were mainly from the starter culture. However, yeast cell counts were lower (p<.05) in the control than in the other treatments. This difference was caused by the inoculation of D. hansenii isolates SMFM2021-C14, SMFM2021-S8, and SMFM2021-SL3 into sausages. These cell counts gradually increased by up to week 2 at 15°C, regardless of the treatment. During fermentation and aging, critical foodborne pathogens such as E. coli O157:H7, L. monocytogenes, and Salmonella cell counts were below the detection limit (0.5 Log CFU/g). However, coliform was detected at 0.6–1.6 Log CFU/g (). The number of coliforms was the highest on week 0. It was less than 1 Log CFU/g after week 1. This inhibition of coliform growth might be caused by P. pentosaceus and S. xylosus growth during fermentation and aging.
Table 4. Microbiological cell counts (Log CFU/g; mean ± standard deviation) in dry fermented sausages inoculated with Debaryomyces hansenii isolates SMFM2021-C14, SMFM2021-S8, and SMFM2021-SL3 during fermentation and aging at 15–20°C for 3 weeks.
Weight loss
Weight loss can be a parameter for evaluating the drying process. This is an important economic indicator in the production of DFS. On week 2 of the fermentation and aging, the control group and D. hansenii SMFM2021-C14 treated group had higher (p < .05) weight loss than D. hansenii SMFM2021-S8 and D. hansenii SMFM2021-SL3 treatment groups (). The weight loss of the control group increased to 51.9% at the end of the fermentation and aging, and there were no significant differences in weight loss among the treatments at the end of the fermentation ().
Aw and pH
Aw of the DFS decreased (p < .05) to 0.773–0.797 in all treatment groups when the fermentation and aging period increased (). These Aw values were the usual Aw for DFS.[Citation42] Various physicochemical or microbial phenomena can induce changes in pH. The decreased pH of fermented sausages is related to the accumulation of organic acids produced by LAB and Micrococcaceae.[Citation43] A decrease in pH at the beginning of fermentation is essential for the sausage manufacturing process. It can inhibit the growth of pathogens and contribute to color, texture, and nitrite reduction.[Citation44] On week 0, the pH of the DFS was approximately 5.98. The pH decreased to 5.21–5.33, depending on the treatment (). These pH values are the usual pH for DFS.[Citation44]
Table 5. Water activity (Aw) and pH (mean ± standard deviation) of dry fermented sausages inoculated with Debaryomyces hansenii isolates SMFM2021-C14, SMFM2021-S8, and SMFM2021-SL3 during fermentation and aging at 15–20°C for 3 weeks.
Reducing sugar
Reducing the sugar content in sausages was the highest on week 1. These values gradually decreased until week 3 of fermentation and aging, regardless of the treatment. It might have occurred because microorganisms decompose glucose generated by the hydrolysis of sugar after week 1. On week 3 of fermentation and aging, reducing sugar contents in D. hansenii SMFM2021-S8 and D. hansenii SMFM2021-SL3 treated groups were significantly lower (p < .05) than that of the control group (). Thus, D. hansenii SMFM2021-S8 and D. hansenii SMFM2021-SL3 showed high reducing sugar utilization rates.
VBN
VBN is an important indicator for evaluating spoilage from the production of amines/ammonia by microorganisms in meat.[Citation45,Citation46] VBN contents in DFS increased (p < .05) in all groups during fermentation and aging (). D. hansenii SMFM2021-S8 treatment group had lower (p < .05) VBN values than the control and other treatments on week 3. Our results indicate that D. hansenii SMFM2021-S8 might be an appropriate isolate to produce less VBN in sausages during dry fermentation. Thus, it might help improve the quality of DFS.
Lipid oxidation
Lipid oxidation is one of the main causes of meat product deterioration, producing harmful compounds to human health.[Citation47] Thus, controlling lipid oxidation for high-quality DFS is necessary. Measuring TBARS is considered a standardized method to evaluate secondary lipid oxidation products in meat products.[Citation48] TBARS values in DFS gradually decreased during fermentation. TBARS values of D. hansenii treated groups were lower (p < .05) than those of the control on week 1 (). Among the D. hansenii treatment groups, D. hansenii SMFM2021-S8 treatment group generally had lower (p < .05) TBARS values than those of the others on week 3. Thus, D. hansenii SMFM2021-S8 might positively affect the quality of fermented sausage due to its ability to decrease lipid oxidation during fermentation and aging.
Table 6. Changes in the thiobarbituric acid reactive substance (malondialdehyde/kg; mean ± standard deviation) in dry fermented sausages inoculated with Debaryomyces hansenii isolates SMFM2021-C14, SMFM2021-S8, and SMFM2021-SL3 during fermentation and aging at 15–20°C for 3 weeks.
Color
As the fermentation period increased, lightness (L*) values of all treatments decreased (p < .05), whereas redness (a*) values increased (p < .05). Hence, there were no obvious differences in color among the treatments ().
Table 7. Color (mean ± standard deviation) of dry fermented sausages inoculated with Debaryomyces hansenii isolates SMFM2021-C14, SMFM2021-S8, and SMFM2021-SL3 during fermentation and aging at 15–20°C for 3 weeks.
Texture profile
In our study, hardness, springiness, chewiness, and gumminess were examined during fermentation. Hardness is a characteristic related to the tenderness of the meat. D. hansenii SMFM2021-S8 and D. hansenii SMFM2021-SL3 treatment groups showed lower (p < .05) hardness values compared to the control group on week 2 (). Proteolysis and lipolytic activities are associated with tenderness.[Citation49] The lower tenderness in D. hansenii SMFM2021-S8 and D. hansenii SMFM2021-SL3 treatment groups might be due to their lipolytic activities. However, D. hansenii SMFM2021-C14 treated group showed a high hardness value, although D. hansenii SMFM2021-C14 had high lipolysis activities. On week 2, the weight loss (%) of D. hansenii SMFM2021-C14 treatment group was higher (p < .05) than those of D. hansenii SMFM2021-S8 and D. hansenii SMFM2021-SL3. This high weight loss in the D. hansenii SMFM2021-C14 treated sausage might cause the high hardness of the sausage, even though the isolate had high lipolysis activity. On week 2, D. hansenii SMFM2021-S8 treatment group had a higher (p < .05) springiness value than the control group (). D. hansenii SMFM2021-S8 and D. hansenii SMFM2021-SL3 treatment groups had lower (p < .05) chewiness and gumminess than the control and D. hansenii SMFM2021-C14 treatment groups on week 2 (). Texture analysis was not conducted on week 3 because the appearance of DFS on week 3 seemed excessively dry.
Table 8. Texture profile (g; mean ± standard deviation) of dry fermented sausage inoculated with Debaryomyces hansenii isolates SMFM2021-C14, SMFM2021-S8, and SMFM2021-SL3 during fermentation and aging at 15–20°C for 3 weeks.
Free fatty acids
Free fatty acids are important factors affecting storage stability and causing unique flavor changes in fermented sausage products. This study investigated the effects of selected D. hansenii isolates on fatty acid compositions in DFS. Oleic acid, palmitic acid, and linoleic acid were present in high proportions of approximately 42%, 22–23%, and 13–14%, respectively, in DFS on week 3 (). Stearic and palmitoleic acid contents were approximately 11% and 2%, respectively. Other fatty acids showed contents close to or less than 1% on week 3. The compositions of the total fatty acids in DFS showed an order of monounsaturated fatty acids > saturated fatty acids > polyunsaturated fatty acids (). The ratio of unsaturated fatty acids/saturated fatty acids increased (p < .05) in the D. hansenii SMFM2021-SL3 treatment group. The compositions of ω-3 fatty acids were higher (p < .05) in the D. hansenii SMFM2021-SL3 treatment group than the other groups, and the compositions of ω-6 fatty acids were lower (p < .05) in the D. hansenii SMFM2021-SL3 treatment group compared to the control group (). Accordingly, the ratio of ω-6/ω-3 was lower (p < .05) in the D. hansenii SMFM2021-SL3 treated group than in the other treatments ().
Table 9. Free fatty acids composition (%; mean ± standard deviation) of dry fermented sausages inoculated with Debaryomyces hansenii isolates SMFM2021-C14, SMFM2021-S8, and SMFM2021-SL3.
Discussion
For the selection of D. hansenii isolates, biogenic amine production, β-glucuronidase, and β-glucosidase activities, proteolysis activity, lipolysis activity, acid tolerance, NaCl tolerance, cytotoxicity, and hemolysis were examined. Tyramine and histamine are biogenic amines frequently detected in cheese and fermented meat products such as fermented beef sausages and Italian salamis because of lactic acid bacteria used as a starter for fermented sausages.[Citation50] These biogenic amines have toxicological effects on blood pressure, the nervous system, and the intestinal system.[Citation51,Citation52] Thus, they are not desired for fermented meat products. In this study, all D. hansenii isolates did not produce tyramine or histamine.
All D. hansenii isolates in this study did not show β-glucuronidase and β-glucosidase activity. It is well known that β-glucuronidase can break the bond between fat-soluble toxic substances and hydrophilic substances and then provide toxic substances.[Citation53] β-glucosidase hydrolyzes glycosides in the colon to produce anticancer substances and simultaneously form carcinogenic aglycones.[Citation54] It was reported that the proteolytic ability of D. hansenii was related to the production of peptides and free amino acids.[Citation55] In this study, all D. hansenii isolates did not show proteolysis in 5% skim milk agar, but they may show a different degree of proteolysis in the actual fermented sausage environment, which is rich in protein substrates. Proteins can be degraded by protease into umami amino acids.[Citation43] Umami can increase consumer accessibility to meat products, and the umami taste can also be an important factor in a fermented sausage.[Citation56] Jeong et al.[Citation3] confirmed that the fermented sausage produced by inoculating D. hansenii SMFM2021-S8 showed a higher umami flavor than the control using a commercial starter. It can help improve the flavor of fermented meats along with lipid degradation[Citation55]; however, these 22 D. hansenii isolates might not have proteolysis activity. Lipolysis activity affects the sensory properties of fermented sausage by changing the composition of free fatty acids in fermented sausages. It is known that lipolysis activity in fermented sausage can reduce lipid oxidation and toxic aldehyde components.[Citation57,Citation58] Free fatty acids produced by triglyceride hydrolysis can form a complex with rhodamine B and exhibit a fluorescent group upon UV irradiation. Seventeen isolates (D. hansenii isolates SMFM2021-C3, SMFM2021-C5, SMFM2021-C8, SMFM2021-C9, SMFM2021-C10, SMFM2021-C12, SMFM2021-C14, SMFM2021-C15, SMFM2021-S2, SMFM2021-S3, SMFM2021-S5, SMFM2021-S6, SMFM2021-S8, SMFM2021-SL1, SMFM2021-SL2, SMFM2021-SL3, and SMFM2021-D1) showed relatively strong or moderate luminescence. In fermented sausages, volatile compounds may be produced as lipid degradation occurs by lipolysis of inoculated microorganisms. Andrade et al.[Citation21] found that only fermented sausages inoculated with D. hansenii showed cured-ham-like flavor compounds (nonanal, heptanal, and octanal). These results suggest that inoculated D. hansenii can enhance flavor by increasing free fatty acid content and generating volatile compounds. It was also observed in a study by Hwang et al.[Citation59] Various physicochemical or microbial phenomena can induce changes in pH, and pH is decreased to approximately 3.5 during fermented sausage production.[Citation13,Citation60] Hence, D. hansenii should be able to grow at a low pH condition to play a role in enhancing the flavor of sausages during fermentation. Ten isolates (D. hansenii isolates SMFM2021-C9, SMFM2021-C10, SMFM2021-C12, SMFM2021-C14, SMFM2021-S2, SMFM2021-S8, SMFM2021-SL1, SMFM2021-SL2, SMFM2021-SL3, and SMFM2021-D1) showed relatively high growth rate. Furthermore, because of the salt used in fermented sausages, three (SMFM2021-C14, SMFM2021-S8, and SMFM2021-SL3) of D. hansenii isolates with better growth in YPD agar supplemented with 2 M NaCl were selected, and they might not have cytotoxicity and hemolysis. Kunz and Lee[Citation61] have reported that nitrate, competitive microflora, and Aw affect microbial safety and storage stability of fermented sausages. In this experiment, an increase in microbiological safety was observed through an increase in starter isolates during fermentation. The number of P. pentosaceus and S. xylosus increased after week 0, whereas the number of coliforms was the highest on week 0 and decreased after week 0.
Reducing sugar is a flavor precursor in edible meat, and the higher the reducing sugar value, the better the flavor.[Citation62] However, when fermented sausages were manufactured with three D. hansenii isolates (SMFM2021-C14, SMFM2021-S8, and SMFM2021-SL3), the amount of glucose detected in this experiment was too small for humans to be noticed. Thus, the effect of these D. hansenii isolates on producing reducing sugar in the sausages was negligible.
Decreased moisture contents in DFS might cause decreased L* values. Moon et al.[Citation63] have reported that the higher the moisture content of pork, the higher the L* value. In all treatment groups, a* values were increased (p < .05), and the increase in a* values for DFS might be due to nitrosomyoglobin through the combination of myoglobin of pork with nitric oxide from microorganisms.[Citation57]
Texture is a very important sensory factor that can significantly affect the palatability and quality of sausages. Hardness is the force required to attain a deformation, and springiness is the property to return to its original state when the force is removed from the deformation.[Citation64] Chewiness means the force required to chew meat enough to swallow it.[Citation65] Also, gumminess refers to the strength required to crush the meat before swallowing.[Citation66] The lower the hardness of the meat, the softer it is and other characteristics, such as chewiness and springiness, can be evaluated in various ways according to individual preference.[Citation67] Corral et al.[Citation68] reported that D. hansenii could affect the hardness and chewiness of sausages without affecting their springiness. Ozturk et al.[Citation55] reported that sausages inoculated with a single LAB showed significantly higher elasticity, chewiness, and gumminess values than sausages inoculated with a mixture of LAB and D. hansenii. In our study, the D. hansenii SMFM2021-S8 treatment group showed significantly lower hardness, chewiness, and gumminess values but higher springiness than other treatments. Thus, using D. hansenii SMFM2021-S8 in DFS might improve their texture profile. Casaburi et al.[Citation45] have shown a higher content of monounsaturated fatty acids than saturated and polyunsaturated fatty acids during the fermentation of sausages, as shown in our results.
Among D. hansenii isolates from fermented food, we selected isolates for DFS based on safety, effectiveness (lipolysis and proteolysis activity), and stability (acid and salt tolerance) experiments. In particular, D. hansenii SMFM2021-S8 treated group showed lower (p < .05) VBN content and lipid oxidation value than the control group. D. hansenii SMFM2021-SL3 treated group exhibited higher (p < .05) fatty acid contents than the control group, which may positively impact sensory characteristics of the DFS. Therefore, D. hansenii SMFM2021-S8 and D. hansenii SMFM2021-SL3 might be useful in producing DFS to improve the quality.
The microorganisms that can be used in fermented meat are limited. Thus, it is important to isolate the microorganism to improve the quality of DFS. This study is meaningful because it evaluated the effect of isolates from fermented food on the quality of DFS. However, to ensure the quality improvement of DFS, additional research such as further safety analysis, aroma profiling, sensory analysis, and shelf-life examination are required.
Acknowledgments
This work was carried out with the support of “Cooperative Research Program for Agriculture Science & Technology Development (Project No. PJ0155902021)” Rural Development Administration, Republic of Korea.
Disclosure statement
No potential conflict of interest was reported by the author(s).
Additional information
Funding
References
- Choi, C. Y.; Lee, C. H.; Yang, J.; Kang, S. J.; Park, I. B.; Park, S. W.; Lee, N. Y.; Hwang, H. B.; Yun, H. S.; Chun, T. Efficacies of Potential Probiotic Candidates Isolated from Traditional Fermented Korean Foods in Stimulating Immunoglobulin a Secretion. Food Sci. Anim. Resour. 2023, 43(2), 345–358. DOI: 10.5851/kosfa.2023.e2.
- Kim, S. K.; Huang, E. C.; Ji, Y. S.; Holzapfel, W. H.; Lim, S. D. Probiotic Property and Anti-Obesity Effect of Lactiplantibacillus plantarum KC3. Food Sci. Anim. Resour. 2022, 42(6), 996–1008. DOI: 10.5851/kosfa.2022.e43.
- Jeong, C. H.; Lee, S. H.; Kim, H. Y. Microbiological Composition and Sensory Characterization Analysis of Fermented Sausage Using Strains Isolated from Korean Fermented Foods. Food Sci. Anim. Resour. 2022, 42(6), 928–941. DOI: 10.5851/kosfa.2022.e56.
- Kim, S. G.; Kim, H. Y. Effect of the Types of Starter on Microbiological and Physicochemical Properties of Dry-Cured Ham. Food Sci. Anim. Resour. 2023, 43(3), 454–470. DOI: 10.5851/kosfa.2023.e9.
- Kim, D. H.; Kim, Y. J.; Shin, D. M.; Lee, J. H.; Han, S. G. Drying Characteristics and Physicochemical Properties of Semi-Dried Restructured Sausage Depend on Initial Moisture Content. Food Sci. Anim. Resour. 2022, 42(3), 411–425. DOI: 10.5851/kosfa.2022.e12.
- Shin, S. H.; Choi, W. S. Physiochemical Properties of Chicken Breast Sausage with Red Ginseng Marc Powder. Food Sci. Anim. Resour. 2022, 42(3), 486–503. DOI: 10.5851/kosfa.2022.e17.
- Zhao, Y.; Suyama, T.; Wu, Z.; Zhang, W. Characterization of Variations and Correlations Between Flavor Metabolites and Microbial Communities of Industrial Paocai Brine During Fermentation. J. Food Process Preserv. 2022, 46(10), e16859. DOI: 10.1111/jfpp.16859.
- Šušković, J.; Kos, B.; Beganović, J.; Leboš Pavunc, A.; Habjanič, K.; Matošić, S. Antimicrobial Activity–The Most Important Property of Probiotic and Starter Lactic Acid Bacteria. Food Technol. Biotechnol. 2010, 48, 296–307.
- Kim, Y.; Ahn, B. S. Study on Development of Fermented Sausage Using Grapefruit Extract and Kimchi Extracted Starter Culture [Korean]. J. East Asian Soc. Dietary Life. 2014, 24(1), 70–79. DOI: 10.17495/easdl.2014.02.24.1.70.
- Yoon, S. H.; Kim, G. B. Inhibition Of Listeria Monocytogenes in Fresh Cheese Using a Bacteriocin-Producing Lactococcus lactis CAU2013 Strain. Food Sci. Anim. Resour. 2022, 42(6), 1009–1019. DOI: 10.5851/kosfa.2022.e48.
- Yuk, Y. S.; Lee, Y. K.; Kim, G. Y. Antagonistic Effects of Lactobacillus Plantarum on Candida albicans in ME-180 Cervical Carcinoma Cell Culture. Jundishapur J. Microbiol. 2020, 13(11), e112449. DOI: 10.5812/jjm.112449.
- Kim, H. S.; Lee, S. Y.; Kang, H. J.; Joo, S. T.; Hur, S. J. Effects of Six Different Starter Cultures on Mutagenicity and Biogenic Amine Concentrations in Fermented Sausages Treated with Vitamins C and E. Food Sci. Anim. Resour. 2019, 39(6), 877–887. DOI: 10.5851/kosfa.2019.e66.
- Yoo, S. A.; Seo, S. H.; Park, S. E.; Son, H. S. Screening of Lactic Acid Bacteria as a Starter Culture in Fermented Sausage [Korean]. J. Korean Soc. Food Sci. Nutr. 2014, 43, 1289–1295. DOI: 10.3746/jkfn.2014.43.8.1289.
- Berni, E. Molds. In Handbook of Fermented Meat and Poultry; Toldrá, F., Hui, Y. H., Astiasarán, I., Nip, W. K., Sebranek, J. G., Silveira, E. T. F., Stahnke, L. H. Talon, R., Eds.; John Wiley & Sons, Ltd: Chichester, 2014; pp. 147–154.
- Song, N. E.; Lee, D. B.; Lee, S. H.; Baik, S. H. Preparation of High GABA-Enriched Yeast Extract by Non-Saccharomyces Yeasts Isolated from Korean Traditional Fermented Soybean Product. Microbiol. Biotechnol. Lett. 2021, 49, 320–328. DOI: 10.48022/mbl.2105.05003.
- Tamang, J. P.; Lama, S. Probiotic Properties of Yeasts in Traditional Fermented Foods and Beverages. J. Appl. Microbiol. 2022, 132(5), 3533–3542. DOI: 10.1111/jam.15467.
- Ramos-Moreno, L.; Ruiz-Pérez, F.; Rodríguez-Castro, E.; Ramos, J. Debaryomyces hansenii is a Real Tool to Improve a Diversity of Characteristics in Sausages and Dry-Meat Products. Microorganisms. 2021, 9(7), 1512. DOI: 10.3390/microorganisms9071512.
- Cano-García, L.; Rivera-Jiménez, S.; Belloch, C.; Flores, M. Generation of Aroma Compounds in a Fermented Sausage Meat Model System by Debaryomyces hansenii Strains. Food Chem. 2014, 151, 364–373. DOI: 10.1016/j.foodchem.2013.11.051.
- Liu, Y.; Wan, Z.; Yohannes, K. W.; Yu, Q.; Yang, Z.; Li, H.; Liu, J.; Wang, J. Functional Characteristics of Lactobacillus and Yeast Single Starter Cultures in the Ripening Process of Dry Fermented Sausage. Front. Microbiol. 2021, 11, 611260. DOI: 10.3389/fmicb.2020.611260.
- Pires, E. J.; Teixeira, J. A.; Brányik, T.; Vicente, A. A. Yeast: The Soul of Beer’s Aroma—A Review of Flavour-Active Esters and Higher Alcohols Produced by the Brewing Yeast. Appl. Microbiol. Biotechnol. 2014, 98(5), 1937–1949. DOI: 10.1007/s00253-013-5470-0.
- Andrade, M. J.; Córdoba, J. J.; Casado, E. M.; Córdoba, M. G.; Rodríguez, M. Effect of Selected Strains of Debaryomyces hansenii on the Volatile Compound Production of Dry Fermented Sausage “salchichón”. Meat Sci. 2010, 85(2), 256–264. DOI: 10.1016/j.meatsci.2010.01.009.
- EFSA. Opinion of the Scientific Committee on a Request from EFSA on the Introduction of a Qualified Presumption of Safety (QPS) Approach for Assessment of Selected Microorganisms Referred to EFSA. EFSA J. 2007, 587, 1–16. DOI: 10.2903/j.efsa.2007.587.
- Koutsoumanis, K.; Allende, A.; Alvarez-Ordóñez, A.; Bolton, D.; Bover-Cid, S.; Chemaly, M.; Davies, R.; De Cesare, A.; Correia, L.; Hilber, F., et al. Update of the List of QPS-Recommended Biological Agents Intentionally Added to Food or Feed as Notified to EFSA 12: Suitability of Taxonomic Units Notified to EFSA Until March 2020. EFSA J. 2020, 18(7), e06174. DOI: 10.2903/j.efsa.2020.6174.
- Gil-Serna, J.; Patiño, B.; Cortés, L.; González-Jaén, M. T.; Vázquez, C. Mechanisms Involved in Reduction of Ochratoxin a Produced by Aspergillus Westerdijkiae Using Debaryomyces hansenii CYC 1244. Int. J. Food Microbiol. 2011, 151, 113–118. DOI: 10.1016/j.ijfoodmicro.2011.08.012.
- Andrade, M. J.; Thorsen, L.; Rodríguez, A.; Córdoba, J. J.; Jespersen, L. Inhibition of Ochratoxigenic Moulds by Debaryomyces hansenii Strains for Biopreservation of Dry-Cured Meat Products. Int. J. Food Microbiol. 2014, 170, 70–77. DOI: 10.1016/j.ijfoodmicro.2013.11.004.
- Bäumlisberger, M.; Moellecken, U.; König, H.; Claus, H. The Potential of the Yeast Debaryomyces hansenii H525 to Degrade Biogenic Amines in Food. Microorganisms. 2015, 3(4), 839–850. DOI: 10.3390/microorganisms3040839.
- Kurtzman, C. P.; Robnett, C. Identification of Clinically Important Ascomycetous Yeasts Based on Nucleotide Divergence in the 5’ End of the Large-Subunit (26S) Ribosomal DNA Gene. J. Clin. Microbiol. 1997, 35(5), 1216–1223. DOI: 10.1128/jcm.35.5.1216-1223.1997.
- Niven, C. F., Jr; Jeffrey, M. B.; Corlett, D. A., Jr. Differential Plating Medium for Quantitative Detection of Histamine-Producing Bacteria. AEM. 1981, 41(1), 321–322. DOI: 10.1128/aem.41.1.321-322.1981.
- Ramos, J.; Melero, Y.; Ramos-Moreno, L.; Michán, C.; Cabezas, L. Debaryomyces hansenii Strains from Valle de Los Pedroches Iberian Dry Meat Products: Isolation, Identification, Characterization, and Selection for Starter Cultures. J. Microbiol. Biotechnol. 2017, 27, 1576–1585. DOI: 10.4014/jmb.1704.04045.
- Gerlier, D.; Thomasset, N. Use of MTT Colorimetric Assay to Measure Cell Activation. J. Immunol. Methods. 1986, 94(1–2), 57–63. DOI: 10.1016/0022-1759(86)90215-2.
- Hammes, W. P.; Knauf, H. J. Starters in the Processing of Meat Products. Meat Sci. 1994, 36(1–2), 155–168. DOI: 10.1016/0309-1740(94)90039-6.
- Wiegand, I.; Hilpert, K.; Hancock, R. E. Agar and Broth Dilution Methods to Determine the Minimal Inhibitory Concentration (MIC) of Antimicrobial Substances. Nat. Protoc. 2008, 3(2), 163–175. DOI: 10.1038/nprot.2007.521.
- Buxton, R. Blood Agar Plates and Hemolysis Protocols. ASM. 2005, 1–9. Available at: https://asm.org/getattachment/7ec0de2b-bb16-4f6e-ba07-2aea25a43e76/protocol-2885.pdf.
- Vermelho, A. B.; Meirelles, M. N. L.; Lopes, A.; Petinate, S. D. G.; Chaia, A. A.; Branquinha, M. H. Detection of Extracellular Proteases from Microorganisms on Agar Plates. Mem Inst Oswaldo Cruz. 1996, 91(6), 755–760. DOI: 10.1590/S0074-02761996000600020.
- Kouker, G.; Jaeger, K. E. Specific and Sensitive Plate Assay for Bacterial Lipases. AEM. 1987, 53(1), 211–213. DOI: 10.1128/aem.53.1.211-213.1987.
- Almagro, A.; Prista, C.; Castro, S.; Quintas, C.; Madeira-Lopes, A.; Ramos, J.; Loureiro-Dias, M. C. Effects of Salts on Debaryomyces hansenii and Saccharomyces cerevisiae Under Stress Conditions. Int. J. Food Microbiol. 2000, 56, 191–197. DOI: 10.1016/S0168-1605(00)00220-8.
- Rural Development Administration (RDA). Meat Product Manufacturing Method (II) [Korean]. 2015. ISBN: 978-89-480-3489-9 94520. Available at: https://www.rda.go.kr/download_file/act/bookcafe086.PDF.
- Jayasena, D. D.; Jung, S.; Kim, H. J.; Yong, H. I.; Nam, K. C.; Jo, C. Taste-Active Compound Levels in Korean Native Chicken Meat: The Effects of Bird Age and the Cooking Process. Poult. Sci. 2015, 94, 1964–1972. DOI: 10.3382/ps/pev154.
- Ministry of Food and Drug Safety (MFDS). Food Code ( MFDS Notice No. 2021-54). 2021. Available at: https://www.mfds.go.kr/eng/brd/m_15/view.do?seq=72437&srchFr=&srchTo=&srchWord=&srchTp=&itm_seq_1=0&itm_seq_2=0&multi_itm_seq=0&company_cd=&company_nm=&page=1.
- Witte, V. C.; Krause, G. F.; Bailey, M. E. A New Extraction Method for Determining 2‐Thiobarbituric Acid Values of Pork and Beef During Storage. J. Food Sci. 1970, 35, 582–585. DOI: 10.1111/j.1365-2621.1970.tb04815.x.
- Michael, T. M.; John, M. M.; Kelly, S. B.; Daniel, H. B.; David, A. S. Microbial Interaction with humans” in “Brock Biology of Microorganisms eds; Benjamin Cummings: San Francisco, 2012; pp. 787–815.
- Ameer, A.; Seleshe, S.; Kang, S. N. Effect of Modified Atmosphere Packaging (MAP) Varying in CO2 and N2 Composition on Quality Characteristics of Dry Fermented Sausage During Refrigeration Storage. Food Sci. Anim. Resour. 2022, 42(4), 639–654. DOI: 10.5851/kosfa.2022.e27.
- Zhao, Y.; Zhao, X.; Sun-Waterhouse, D.; Waterhouse, G. I. N.; Zhao, M.; Zhang, J.; Wang, F.; Su, G. Two-Stage Selective Enzymatic Hydrolysis Generates Protein Hydrolysates Rich in Asn-Pro and Ala-His for Enhancing Taste Attributes of Soy Sauce. Food Chem. 2021, 345, 128803. DOI: 10.1016/j.foodchem.2020.128803.
- Bozkurt, H.; Erkmen, O. Effects of Starter Cultures and Additives on the Quality of Turkish Style Sausage (Sucuk). Meat Sci. 2002, 61, 149–156. DOI: 10.1016/S0309-1740(01)00176-0.
- Casaburi, A.; Di Monaco, R.; Cavella, S.; Toldrá, F.; Ercolini, D.; Villani, F. Proteolytic and Lipolytic Starter Cultures and Their Effect on Traditional Fermented Sausages Ripening and Sensory Traits. Food Microbiol. 2008, 25, 335–347. DOI: 10.1016/j.fm.2007.10.006.
- Kim, S. J.; Kim, G. H.; Moon, C.; Ko, K. B.; Choi, Y. M.; Choe, J. H.; Ryu, Y. C. Effects of Aging Methods and Periods on Quality Characteristics of Beef. Food Sci. Anim. Resour. 2022, 42(6), 953–967. DOI: 10.5851/kosfa.2022.e63.
- Broncano, J. M.; Petrón, M. J.; Parra, V.; Timón, M. L. Effect of Different Cooking Methods on Lipid Oxidation and Formation of Free Cholesterol Oxidation Products (COPs) in Latissimus Dorsi Muscle of Iberian Pigs. Meat Sci. 2009, 83, 431–437. DOI: 10.1016/j.meatsci.2009.06.021.
- Seleshe, S.; Ameer, A.; Kang, S. N. Incorporation of Selected Strains of Pediococcus Spp. on Quality Characteristics of Dry Fermented Sausage During Fermentation and Ripening. Food Sci. Anim. Resour. 2021, 41(6), 1078–1094. DOI: 10.5851/kosfa.2021.e601.
- Choe, J. H.; Kim, H. Y. Effects of Dry Aging on Physicochemical Properties of Beef Cattle Loins. Korean J. Food Sci. Technol. 2017, 49, 158–161. DOI: 10.9721/KJFST.2017.49.2.158.
- Pasini, F.; Soglia, F.; Petracci, M.; Caboni, M. F.; Marziali, S.; Montanari, C.; Gardini, F.; Grazia, L.; Tabanelli, G. Effect of Fermentation with Different Lactic Acid Bacteria Starter Cultures on Biogenic Amine Content and Ripening Patterns in Dry Fermented Sausages. Nutrients. 2018, 10(10), 1497. DOI: 10.3390/nu10101497.
- Smith, T. A. Amines in Food. Food Chem. 1981, 6(3), 169–200. DOI: 10.1016/0308-8146(81)90008-X.
- Taylor, S. L. Histamine Food Poisoning: Toxicology and Clinical Aspects. CRC Crit. Rev. Toxicol. 1986, 17(2), 91–128. DOI: 10.3109/10408448609023767.
- Ministry of Food and Drug Safety (MFDS). Guidelines for Evaluating Health Functional Foods (No. 2020-92). 2020. Available at: file:///C:/Users/User/Downloads/Health+Fuctional+Food+Code(No.+2020-92).pdf.
- Allsopp, P.; Rowland, I. Potential Protective Effects of Probiotics and Prebiotics Against Colorectal cancer” in “Prebiotics and Probiotics Science and Technology; Charalampopoulos, D.; Rastall, R.A eds., Springer: New York, 2009; pp. 997–1048. 10.1007/978-0-387-79058-9_26
- Ozturk, I.; Sagdic, O.; Yetim, H. Effects of Autochthonous Yeast Cultures on Some Quality Characteristics of Traditional Turkish Fermented Sausage “Sucuk”. Food Sci. Anim. Resour. 2021, 41(2), 196–213. DOI: 10.5851/kosfa.2020.e89.
- Dermiki, M.; Phanphensophon, N.; Mottram, D. S.; Methven, L. Contributions of Non-Volatile and Volatile Compounds to the Umami Taste and Overall Flavour of Shiitake Mushroom Extracts and Their Application as Flavour Enhancers in Cooked Minced Meat. Food Chem. 2013, 141, 77–83. DOI: 10.1016/j.foodchem.2013.03.018.
- Cavalheiro, C. P.; Piovesan, N.; Terra, L. D. M.; Lovato, M.; Terra, N. N.; Fries, L. L. M. Colorimetric and Sensory Characteristics of Fermented Cured Sausage with Brazilian Ostrich Meat Addition. Food Sci. Technol. 2013, 33(4), 660–665. DOI: 10.1590/S0101-20612013000400010.
- Karsloğlu, B.; Çiçek, Ü. E.; Kolsarici, N.; Candoğan, K. Lipolytic Changes in Fermented Sausages Produced with Turkey Meat: Effects of Starter Culture and Heat Treatment. Food Sci. Anim. Resour. 2014, 34(1), 40–48. DOI: 10.5851/kosfa.2014.34.1.40.
- Hwang, J. E.; Kim, Y. J.; Seo, Y. E.; Sung, M. S.; Oh, J.; Yoon, Y. H. Effect of Starter Cultures on Quality of Fermented Sausages. Food Sci. Anim. Resour. 2023, 43(1), 1–9. DOI: 10.5851/kosfa.2022.e75.
- Lee, J. Y.; Sung, J. M.; Cho, H. J.; Woo, S. H.; Kang, M. C.; Yong, H. I.; Choi, Y. S. Natural Extracts as Inhibitors of Microorganisms and Lipid Oxidation in Emulsion Sausage During Storage. Food Sci. Anim. Resour. 2021, 41(6), 1060–1077. DOI: 10.5851/kosfa.2021.e58.
- Kunz, B.; Lee, J. Y. Production and Microbiological Characteristics of Fermented Sausages. Food Sci. Anim. Resour. 2003, 23, 361–375.
- Khan, M. I.; Jo, C.; Tariq, M. R. Meat Flavor Precursors and Factors Influencing Flavor Precursors-A Systematic Review. Meat Sci. 2015, 110, 278–284. DOI: 10.1016/j.meatsci.2015.08.002.
- Moon, S. H.; Jeong, J. Y.; Kim, G. D.; Cho, I. C.; Jeon, J. T.; Joo, S. T.; Park, G. B. The Relationship Between Measurements of Color and Water-Holding Capacity in Pork Loin. J. Anim. Sci. Technol. 2009, 51(4), 329–336. DOI: 10.5187/JAST.2009.51.4.329.
- Marta, H.; Cahyana, Y.; Djali, M.; Arcot, J.; Tensiska, T. A Comparative Study on the Physicochemical and Pasting Properties of Starch and Flour from Different Banana (Musa Spp.) Cultivars Grown in Indonesia. Int. J. Food. Prop. 2019, 22, 1562–1575. DOI: 10.1080/10942912.2019.1657447.
- Suebsaen, K.; Suksatit, B.; Kanha, N.; Laokuldilok, T. Instrumental Characterization of Banana Dessert Gels for the Elderly with Dysphagia. Food Biosci. 2019, 32, 100477. DOI: 10.1016/j.fbio.2019.100477.
- Li, R.; Carpenter, J. A.; Cheney, R. Sensory and Instrumental Properties of Smoked Sausage Made with Mechanically Separated Poultry (MSP) Meat and Wheat Protein. J. Food Sci. 1998, 63, 923–929. DOI: 10.1111/j.1365-2621.1998.tb17928.x.
- Yi, H. C.; Cho, H.; Hong, J. J.; Ryu, R. K.; Hwang, K. T.; Regenstein, J. M. Physicochemical and Organoleptic Characteristics of Seasoned Beef Patties with Added Glutinous Rice Flour. Meat Sci. 2012, 92, 464–468. DOI: 10.1016/j.meatsci.2012.05.012.
- Corral, S.; Belloch, C.; López-Díez, J. J.; Salvador, A.; Flores, M. Yeast Inoculation as a Strategy to Improve the Physico-Chemical and Sensory Properties of Reduced Salt Fermented Sausages Produced with Entire Male Fat. Meat Sci. 2017, 123, 1–7. DOI: 10.1016/j.meatsci.2016.08.007.