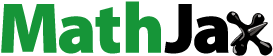
ABSTRACT
Carrot pomace, a byproduct of juice extraction from the widely cultivated root vegetable, contains micronutrients and carotenoids that are often discarded as agricultural waste. To address this issue, the present study aimed to create a stable carrot pomace-based emulsion (CPBE) suitable for use in butter production and to evaluate its impact on the physicochemical, microbiological, thermal, oxidative, and fatty acid properties of butter. The CPBE was made with a natural emulsifier and a dispersion medium made of a mixture of vegetable oils in a certain ratio to get the most components, like carotenoids, probiotic culture, and omega-3 fatty acids. The emulsion was selected based on particle size, zeta potential, and color values. Compared to the control sample, the addition of CPBE altered the content of moisture, carbohydrate, protein, and fat, resulting in a higher calorie count. The addition of CPBE also improved the oxidative stability of butter and inhibited the growth of coliforms, Staphylococcus aureus, and psychotropic bacteria during storage, and its biological value increased while its spreadability decreased. Although the color scores were impacted by CPBE, overall acceptability was unaffected. The study shows that the use of CPBE in butter production has potential applications in the functional food industry.
Introduction
The rising interest in healthier eating has prompted the food sector to innovate with the help of functional foods and ingredients, yielding new value-added products, and their availability in stores and online marketplaces has increased.[Citation1] An upside of this progress is that it enables food producers to increase the value of items that consumers are already familiar with. Variations impacting processing conditions, sensory attributes, and nutritional value of the finished product are the primary considerations.[Citation2] Therefore, waste products from the processing of fruits and vegetables can be used as a sustainable supply of nutritional fiber due to their low production costs and desirable properties, such as their ability to bind water and increase viscosity. Many regularly consumed foods and drinks, including baked goods, dairy products, and beverages, can be improved by including them in the new formulations.
Milk and dairy products are beneficial sources of protein, calcium, potassium, and vitamin D (if the product is fortified with it) and they contribute to a nourishing diet. In recent years, there has been an increase in the popularity of “functional foods,” which can be made by combining dairy products with fruits, vegetables, probiotic culture, and a blend of plant oils.[Citation3] Therefore, with addition of plant oils butter’s fatty phase can be enhanced in both fatty acid composition and biological value by the addition of polyunsaturated fatty acids, such as ω-3 fatty acids[Citation4] To reduce the amount of fat in the butter and make it more nutritious, vitamins, minerals, plant components, or dietary fibers are added to the formulation. Herbal ingredients with elevated levels of crude antioxidants (vitamins C and E) can extend the shelf life of butter. Abid et al.[Citation5] has disclosed that the storage life of Tunisian butter is prolonged by up to two months due to a tomato by-product extract. To obtain functional butter, Denisov[Citation6] proposed the introduction of unrefined vegetable oils from camellia and macadamia. Butter acquires distinct properties when lactic acid bacteria are used in its fermentation. Ewe and Loo[Citation7] conducted a study to compare the proportion of unsaturated fatty acids in butter produced from cream fermented with L. helveticus (LH-butter) to a control sample. Their findings indicated that LH-butter had a higher percentage of total unsaturated fatty acids (55%) compared to the control (36%). The researchers proposed that this result could be attributed to the presence of enzymes in L. helveticus that break down milk fat in the cream, resulting in the release of free fatty acids. In another study by Złoch et al.,[Citation8] the authors showed that the addition of L. paracasei increased the ratio of unsaturated to saturated fatty acids, suggesting that probiotic bacteria can synthesize unsaturated fatty acids during incubation in dairy products. The presence of monounsaturated fatty acids (MUFA) in cream has the potential to prolong the shelf life of butter and improve its health-promoting properties. Gundogdu et al.[Citation9] conducted a study to investigate the impact of specific starter cultures (Lactococcus lactis subsp. lactis biovar. diacetylactis or Leuconostoc mesenteroides subsp. cremoris) on the volatile characteristics and sensory quality of yogurt butter and cream butter. The researchers found that both types of butter containing starter cultures had better sensory qualities and contained 61 types of volatiles, such as esters, aldehydes, ketones, alcohols, and acids. The combination of Leu. mesenteroides subsp. cremoris and L. lactis subsp. lactis biovar diacetylactis was identified as the most suitable starter culture for producing yogurt and cream butter with optimal volatile characteristics. Butter samples containing mixed cultures were also found to have the highest general acceptability scores at the end of storage.
Vegetable oils are full of monounsaturated and polyunsaturated fats and are becoming more popular as people look for healthier alternatives to the unhealthy saturated and trans fats found in many processed foods.[Citation10] However, it is challenging to completely replace saturated fats with liquid vegetable oils and improve texture because of the flexibility and elasticity features of solid fats and their significance in specific foodstuffs.[Citation11] The application and functional properties of fats and oil depend on the composition and structure of triacylglycerols (TAG). As a result, lipid TAG changes can be used to synthesize tailored lipids with a broader range of applications. However, no natural edible oil is available with appropriate dietary and functional properties to meet the human recommended dietary allowances (RDA). However, there is evidence to suggest that some of the processes used to modify vegetable oils can have negative health effects. For example, partially hydrogenated oils (PHOs) produced through hydrogenation contain trans-fatty acids (TFAs), which have been linked to an increased risk of heart disease.[Citation12] A number of studies have found that the consumption of TFAs raises LDL (“bad”) cholesterol levels and lowers HDL (“good”) cholesterol levels, which can increase the risk of heart disease.[Citation12] Therefore, innovative technologies are shifting toward modifying fat and oil to improve their functionality. Bascuas et al.[Citation13] found that making semi-solid or solid fats through the preparation of emulsion gels or oleogels is a new and potentially effective way to change the structure and texture of food. And there are several advantages of the oleogels fabrication process over blending, hydrogenation, and interesterification.[Citation14]
It has been demonstrated through an analysis of the relevant scientific and technical literature that the addition of probiotics or lactic acid cultures,[Citation8] vegetable oils, fruits, and vegetables,[Citation5] berry crops, grain components, plant extracts with antioxidant properties to butter not only expands the functionality of the butter but also pro-longs the shelf life.[Citation4] The aim of this study was to ascertain how the addition of a vegetable fat supplement, which included powdered carrot pomace, pollen, dry buttermilk, the emulsifier lecithin, and a mixture of vegetable oils (including linseed, sea buckthorn, and wheat germ oil) as a source of ω-fatty acids, would affect the functional properties of butter. This would allow us to produce healthier butter by increasing its overall nutritional value.
Materials and methods
Materials
Milk to produce butter was taken from the local farm (Borodulikha district, Kazakhstan). Milk that meets the requirements of GOST (National Standard) 13264–88 was used for the production of butter. For this purpose, milk quality indicators were determined, and the related data are given in Tables S1-S3. Fresh, non-damaged whole carrots (Daucus carota L.), wheat germ oil (Triticum Vulgare), sea buckthorn oil (Hippophae rhamnoides L.), and linseed oil (Linum Usitatissimum L.) were purchased from the local market (Semey, Kazakhstan) and kept at 4°C before use. Liquid oils were used as continuous-phase medium. These oils were used due to their functional attributes toward human health and good amount of polyunsaturated fatty acids (PUFA) (). Probiotic culture, the EM-Kurunga (Baikal-Biotech, Irkutsk, Russia) was in the form of dry sour-dough powder, a multifunctional natural probiotic product with a preventive, corrective, and restorative effect. Fermented for 2–3 days at 25–28°C in 0.05% skimmed milk (this optimizes the fermentation time of milk), after which the product becomes organoleptically homogeneous, with the yeasty and light sour-wine taste. The great stability of this culture in dairy products led to its selection. An emulsifier soy-bean lecithin (Russia) was purchased from a local pharmacy. Flower pollen is obtained from the local apiary (Katon Karagay, Kazakhstan). Dry buttermilk and whey protein concentrate (KSB-60, Russia) were purchased from local suppliers. All the substances utilized in this study were of analytical quality and sourced from reputable vendors.
Table 1. Fatty acid composition of liquid oils used to formulate emulsion.
Methods
Experimental plan
In the present study, carrot pomace powder was taken into formulation of carrot pomace-based emulsion which consist of wheat germ oil (Triticum Vulgare), sea buckthorn oil (Hippophae rhamnoides L.), linseed oil (Linum Usitatissimum L.), probiotic culture, emulsifier, flower pollen, dry buttermilk, and whey protein concentrate to obtain a functional food – butter. A commercially produced butter “Buterbrodnoe” was kept as a control. The butter enriched with CPBE were stored at 4°C to perform oxidative stability tests for 60 days comparing with a control sample (butter “Buterbrodnoe”). Sampling was performed at 0, 15, 30, 45, and 60 days. The treatment was replicated three times, and the sample was at least analyzed three times.
Carrot pomace processing (CP)
The Department of Technology of Food Production and Biotechnology’s Laboratory of Operating Systems at Shakarim University of Semey (Semey, Kazakhstan) manufactured CP using a process involving 15 kg of uniformly ripe carrots (Daucus carota L.) purchased from a local farmers’ market. The carrots were cleaned and disinfected with a sodium hypochloride solution at a concentration of 70 mg/L at 10 ± 1°C. Then, the outer layer was manually removed. The cleaned carrots were processed in a juicer (MES4010, Centrifugal juicer VitaJuice 4, 1200W, Bosch) and the resulting CP was dehydrated in a tray dryer at a 60 ± 2°C for 5 h (Sedona SD-9000, Tribest, Seoul, South Korea) with a layer thickness of 0.5 cm. Following drying, the CP was ground in a grinder (Magic Bullet, Capital Brands, Los Angeles, USA) until 95% of the particles passed through a 40-mesh filter. The resulting CP was stored in a desiccator until further use in a dry, dark room at 24 ± 2°C after being vacuum-packed in polyethylene bags (300 gauge) using a vacuum baler (Boxer 42, Netherlands). For reasons of preserving the CP’s physicochemical, color, and textural features, the bags containing the CP were coated with aluminum foil to block out light. The composition of the CP in 100 g of flour included moisture (4.09%), proteins (7.51%), lipids (0.93%), carbohydrates (54.15%), minerals (15.40%), ash (5.24%), fiber (28.90%), total carotene (5.643 μg), β-carotene (590 μg/100 g), and energetic value (340.35kcal). By following this procedure, 1.10 kg of CP was obtained from 15 kg of carrots. This process allows for the production of a powder from carrot pomace, which is a by-product of industrial carrot processing and possesses useful and nutritive characteristics.
Carrot pomace-based emulsion (CPBE) preparation
A water-in-oil emulsion was prepared using a commercial wheat germ oil (Triticum Vulgare), sea buckthorn oil (Hippophae rhamnoides L.), and linseed oil (Linum Usitatissimum L.) as the oil phase. The composition of the emulsion consisted of the following components (%): carrot pomace − 21.01, flower pollen − 4.8, dry buttermilk (moisture 4.2 ± 0.5%, fat 8.0 ± 0.7%, protein 30.0 ± 0.5%) − 28.9, lecithin − 0.8, wheat germ oil − 4.8, sea buckthorn oil − 4.8, linseed oil − 12.0, water − 22.89. The emulsion was prepared by homogenizing an oil phase (45.29 wt.%) with an aqueous phase (54.71 wt.%) using a high-speed a homogenizer (HG-15A-SET-A, Russia). The oil phase consisted of lecithin emulsifier, wheat germ oil, sea buckthorn oil, and linseed oil, while the aqueous phase consisted of carrot pomace powder, buttermilk powder, and flower pollen. Prior to homogenizing process, the aqueous phase was treated at 85°C for 3 min and chilled to 25–30°C. For the final homogenization, the oil phase was injected at a constant speed over the course of three minutes. The emulsification procedure was conducted for 5 min at a speed of 5-sec− 1 and 20 ± 2°C. Each step’s duration was timed and recorded. Rheological characterization was performed after letting the emulsion sit for two hours to allow the internal temperature to drop after processing. Before being used, the CPBE was kept in the fridge at 4°C for no more than 36 h and the color of obtained emulsion was visually determined (Fig. S1).
Manufacture of butter
The butter was manufactured at the Food Technology Laboratory of Shakarim University. The flow chart of butter preparation named “Asylum” is shown in .
Normalization
The fat content in the cream was in the range of 32–37%. If less, then the cream was normalized by adding more fatty cream; if the cream is fattier, then low-fat milk was added.
Pasteurization
Normalized cream (grade I) was pasteurized at 85–90°C without aging (stationary pasteurizer, LLC “Liberty Agro,” Minsk, Belarus). Cream (grade II) was pasteurized at 92–95°C.
Cooling, aging and physical ripening (churning)
The cream was cooled in a fridge cooler (Indesit, Italy) to 12–15°C. During the spring-summer period, the churning process is performed at 7–12°C, and in the autumn-winter period, at 8–14°C. Pasteurized cream with 35–40% fat, properly aged, is pumped into the churn. The cream is filled to 35–40% of the volume of the churner machine (Farmer MB-01, Novosibirsk, Russia). To reduce the time of churning, before the start of the churning process, whey protein concentrate (KSB-60) was introduced into the churn in the amount of 0.4–1% by weight of the cream. The churning process was reduced by 15 min and amounted to 20 min, instead of the traditional 45 min. In the first 5 min, the churning was stopped 1–2 times, and the carbon dioxide produced from the cream during churning was released through the tap. The rotation of the churn was 46-60rpm. The excess of fat in buttermilk is reduced, and the yield of butter is increased.
Washing
After the buttermilk was taken out, the butter grains were washed to stop bacteria from growing while the butter was being stored. The butter was washed twice. The amount of water used to wash grains was two times that of churned cream. The water temperature was 7–12°C in the first wash and 5–10°C in the second wash. The butter grain rotated several times (about 4 turns) in the churn, and then the water was drained off.
Thermomechanical treatment
The goal of the thermomechanical treatment of butter is to reduce the amount of moisture in the product while also compacting the butter granules into a mass that is more manageable for packaging and shipping. For this, butter is passed through the blades of the churn. During initial working, extra moisture and buttermilk are removed. The churn door is then closed, and the machine is put on a low speed once the necessary amount of water has been added. The process is repeated until all the available moisture has been absorbed by the butter and the churn is dry. At this point, the butter tester plug, or a cross-section cut between 25 and 35%, should show no signs of moisture, as specified by the butter product’s formulation. During this process the probiotic culture and vegetable fat emulsion were introduced and mixed for an additional 10 min.
Pre-packaging and packaging
The finished butter was packed in polystyrene cups weighing 200 and 400 g. The butter was placed in freezers set to −18°C for long-term storage.
Storage
Butter was stored at a relative humidity of 70–90%. Storage is carried out under the following modes: non-hermetic polymer packaging at 4°C − 60 days; non-hermetic polymer packaging at −3 to −9°C, for 60 days; and non-hermetic polymer packaging at −14 to −18°C, for a shelf life of 120 days.
Analyses
Analyses were performed in the Testing Regional Engineering Laboratory of the Scientific Center for Radioecological Research at “NutriTest” (Almaty, Kazakhstan).
Proximate analysis
Emulsion and butter were tested for moisture, protein, ash, and fat content following GOST (State Standard) R 3626–73 (oven drying), GOST 23,327–98 (Kjeldahl), GOST 31,727–2012 (muffle furnace), and GOST 58,672–90 (Soxhlet). Using a pH meter (Model 340, Mettler-Toledo GmbH, Switzerland) with an electrode for solid (S7) for butter and an electrode for liquid (LE410) for CPBE, the pH values of both were obtained via direct immersion. The cryoscopy method was used to detect water activity (aw) for highly moisturized materials, while a Hygropalm – AW1 (Rotronic) device was utilized for objects with an intermediate humidity level.
Colour measurement
The instrumental color (L*: lightness; a*: redness; b*: yellowness) of the emulsion and butter using a Konica Minolta CM − 2600 (Japan) spectrophotometer with illuminant D65, 10° observer and 8 mm for measurement; with a pure glass plate between the samples (n = 7) and the equipment. All assays were accomplished in triplicate at 25°C.[Citation15] The color measurement of butter was read at 0, 15, 30 and 45 days during 60 days of refrigerated storage at 4 ± 1°C.
Textural characteristics of carrot pomace-based emulsion and butter
A texture analyzer TA XTPlus (Stable Micro Systems, UK) was utilized to establish the CPBE and butter samples for their textural qualities. A back extrusion rig (A/BE) with a 35 mm compression disc and extension bar equipped with a 5 kg load cell was used to analyze the CPBE’s texture, as described by authors.[Citation16] CPBE sample’s textural properties were measured in terms of firmness, consistency, and adhesiveness. Measurements were taken in triplicate. A TTC spreadability rig (HDP/SR) was used to evaluate the spreadability of butter sample according to authors.[Citation17] The probe moved through the sample at a velocity of 3.0 mm s−1 for the course of the experiment. Firmness (N) and work of shear (N s) were used to describe the maximum penetrating force and mean area, respectively. The samples were analyzed at 23 ± 0.5°C right after being taken out of the refrigerator (+4°C).
Particle size analysis of CPBE and butter
Zetasizer Nano-ZS90 (Malvern Panalytical, UK) was used to analyze the emulsion and butter’s particle size. Samples were diluted 100-fold with Millipore water before being measured for particle size (nm) to achieve a laser obscuration of 10%. The temperature of dispersed phase was set to 4 ± 2°C and dispersing phase to 25 ± 2°C. Depending on the Mie Theory, particle size parameters were stated as follows: span (normalizes the width of the distribution relative to the median value); surface area mean diameter (D[3.2]); and d(0.1), d(0.5), and d(0.9) (i.e., 10%, 50% and 90% of the sample fragments are under a specific diameter, correspondingly).
Amino acid (AAC) and the fatty acid (FAC) content of butter
AAC was determined according to Standard 1363–2000, and this technique uses high-performance liquid chromatography (HPLC) to ascertain the relative amounts of 18 amino acids in food products. The amino acid composition was determined on a Shimadzu LC-20 Prominence device using a fluorometric and spectrophotometric detector (Shimadzu, Japan) as described by Okuskhanova et al.[Citation18] FAC of the fatty phase of the butter was determined by GOST 31,663–2012 using gas chromatography (Hewlett Packard HP 6890, Agilent, USA).
Vitamins content (VC) and the mineral composition (MC) determination of butter
The VC was measured by an HPLC system according to the method described by the authors.[Citation19] The MC was carried out using a JEOL 6390 LV low-vacuum scanning electron microscope (JSM-6390LV, 2003) with an INCA ENERGY 250 energy dispersive analysis system (JEOL Japan). All determinations were performed in triplicate.
Antioxidant characteristics of butter incorporated with CPBE
Total phenolic contents (TPC)
The TPC was evaluated using the analytical protocol developed by Nadeem et al.[Citation20] To begin, 250 mg of the test samples were combined with a 10% Folin-Ciocalteu solution (1.5 mL). After five minutes, a 6% Na₂CO₃ solution (1.5 mL) was added to the mixture, which was then incubated in the absence of light for 60 min. The absorbance at 760 nm in the visible region of the spectra was measured using a double-beam spectrophotometer (Shimadzu, Japan) and compared against a blank. The results were expressed as milligrams of gallic acid equivalents per 100 g of sample (mg GAE/g).
Scavenger effect on DPPH free radicals
The DPPH free radical scavenging activity was assessed using the procedure described by Brand-Williams et al.[Citation21] In this method, 60 mg of the sample was combined with 2.44 mL of DPPH solution, and the mixture was incubated at 25°C for 1 h. The absorbance was measured at 515 nm using a double beam spectrophotometer (Shimadzu, Japan).
Oxidative stability during storage
The butter samples were kept at 4 ± 1°C for 60 days, and their quality was evaluated at 0, 15, 30, 45, and 60 days. To assess the butter’s storage capacity and quality, the peroxide value (PV) was determined, according to GOST 216,593–85 and stated as milliequivalents of iodine per kg of lipid (meq/kg) or as millimoles of hydroperoxide per kg of lipid (referred to as “peroxide”). PV is displayed by the following equation:
where V0 - the amount of Na2S2O3 used for the blank (mL), N - the normality of Na2S2O3, and m is the amount of sample (g). Analyses for thiobarbituric acid (TBA) (malonaldehyde equivalent g−1 fat) were performed according to methods reported by Ayar et al.[Citation22]
Microbiological analyses
To conduct the microbiological analysis, a mixture of 10 g of butter and 90 mL of a sterilized 0.85% (w/v) NaCl solution was prepared and heated in a water bath at 45°C until fully melted. The resulting mixture was diluted serially, and coliforms were enumerated by using violet, red bile glucose agar and incubating at 37°C for 24 h. Staphylococcus aureus was quantified using Baird Parker Agar (BPA, Merck) that contains potassium tellurite and egg yolk (Merck), and incubated at 37°C for 48 h. Yeasts and molds were detected by using Sabouraud Dextrose Agar (SDA, Merck, Germany) supplemented with chloramphenicol and incubated at 25°C for 3–5 days. Psychotropic bacteria were counted by using Plate Count Agar (PCA, Merck, Germany) and incubated at 6.5°C for 10 days.
Sensory evaluation
Butter samples for sensory evaluation were aged at 5°C for 48 h and then stored frozen at −18°C before analysis. Sensory investigation of experimental butter was performed using quantitative descriptive analysis. For this purpose, a group of 30 nontrained panelists, chosen among the students and the employees of the Department of Technology of Food Production and Biotechnology of the Shakarim University of Semey, Semey, Kazakhstan (18 women and 12 men, ages 22–54) were enrolled. Butter samples in terms of taste, color, flavor, overall acceptability, texture, and packaging and labeling were determined using five-point hedonic scale,[Citation23] varying from “I extremely disliked” (grade 1) to “I extremely liked” (grade 5). The butter was manufactured according to the same procedure described earlier. Samples were held at 5°C overnight before monadic presentation at 21°C to the panelists; each sample was coded with five-digit random numbers. The sensory analysis procedure (Shakarim University, Semey, Kazakhstan, protocol #9, 15.03.2022) was approved by the Academic Council of the Faculty.
Statistical analysis
Data were analyzed using a one-way or two-way analysis of variance (ANOVA method) to compare differences in mean values between different groups. The significance level was set at p ≤ .05. The Tukey test was then used to assess whether any significant differences observed in mean values between treatment groups were greater than would be expected by chance, for multiple evaluations or statistical rankings (p ≤ .05). All experiments were conducted in triplicate.
Results and discussion
Characterization of carrot pomace-based emulsion
Emulsions are highly dispersed colloidal systems with particle sizes of the dispersed phase of 10−5- 10−9m. One of the phases of the emulsion is water. The second phase is an organic liquid, it is commonly called “oil.” Water and oil form two types of emulsions: direct emulsions – oil in water and reverse emulsions – water in oil. The emulsion developed by us belongs to the second type – water in oil. For their production, energy input, such as through mechanical stirring, is required to stabilize the system kinetically by means of surfactants. The latter serves to lower the interfacial tension between oil and water phase to form droplets with sizes in the micrometer range. The system that our emulsion consisted of is an aqueous phase – buttermilk restored from a dry powder, with the addition of carrot pomace powder, which in this case acts as an emulsion thickener, flower pollen acts as a vitamin and mineral supplement that allows to add nutritional characteristics to the food product. The emulsifying ability of this aqueous phase is in direct proportion to the content of dry residue and protein substances in it. If this system contains less than 5% solids, then when vegetable oils are added, separation will occur 30-40 min after emulsification. Therefore, we aimed for the concentration of solids in this system to be at least 15%. As a dispersion medium, a mixture of vegetable oils was taken, in the ratio of wheat germ oil, sea buckthorn oil, linseed oil as 1:1:2.5. The calculation of the components of the oil mixture makes it possible to obtain a fatty base with the necessary balanced composition of polyunsaturated fatty acids. The process of mixing vegetable oils, reconstituted buttermilk, carrot pomace powder, and flower pollen were carried out at 25–30°C, which allows maintaining the stability of the emulsion and achieving uniformity. Emulsions are metastable systems; their inherent thermodynamic instability eventually causes coalescence and phase separation. To give stability to the emulsion, we used a special stabilizer substance – an emulsifier – soy lecithin. Soy lecithin at the phase boundary exhibits surface-active properties, this is due to the high content of phosphatidylcholines in its composition, it also contains inositol, phosphatidylethanolamine.[Citation24] Features of the emulsifying properties of phospholipids are due to the ability to form and maintain in a homogeneous state both direct and inverse emulsions, which extends their use to all types of food emulsions: from mayonnaises and various salad dressings (direct emulsions) to margarines of various fatty acid composition and different content of the fatty phase (inverse emulsions). Another distinctive feature of phospholipids as food emulsifiers is their ability to form liposomes – lipid vesicles: particles formed by concentric closed lipid bilayers with an inner aqueous layer isolated from the external environment and containing, depending on the purpose of the liposomes, various inclusions, such as carbohydrates, peptides, or proteins. The use of liposomal systems in food products is associated with the functions of isolating and protecting individual food ingredients from external influences (protecting yeast cells from cooling in frozen semi-finished dough products), retaining moisture (ice cream) or flavoring substances. The surface activity of phospholipids at interfacial boundaries (solid/liquid, liquid/gas, etc.) determines the effectiveness of their action in multicomponent disperse systems, including structured ones, in which the purpose of these additives is reduced to a change in rheological properties.[Citation25] Physico-chemical characteristics of carrot pomace-based emulsion are given in .
Table 2. Physico-chemical characteristics of carrot pomace-based emulsion.
The emulsion had a pH of 5.5, putting it in the acidic range. It was believed that these acidic emulsions could reduce microbial activity, increasing a product’s shelf life.[Citation26] In addition, the emulsion had a lower moisture content of 29.67%. Ultimately, this may have a beneficial influence on the butter’s shelf life. The content of fiber is 45.02% and it could be positive impact because the rheological and textural properties of a food system could be enhanced with the addition of dietary fiber or ω-3 rich oil.[Citation27] Powdered carrot pomace is the primary source of carbohydrates and dietary fiber in the emulsion.
The size of the particles or droplets in an emulsion is the primary factor in determining the emulsion’s stability. Many characteristics of an emulsion, such as its physical and microbiological stability, rheology, and optical properties were found to be highly dependent on the droplet size distribution.[Citation28] displays the average droplet size (d4,3) of the investigated emulsion. The physical stability of an emulsion can be enhanced by decreasing the droplet size, which, in turn, reduces the number of lipid molecules per droplet and increases the number of surface-active chemicals adsorbed at the interface. The increased number of particles and the enhanced particle-particle interactions caused by the smaller droplet size contributed to the enhanced viscosity of the emulsion. The average particle size of the emulsion created in this study was 23.54 ± 3.05 µm. Guggisberg et al.[Citation28] noticed that the particle size of emulsions destabilized at D[4.3] was more than 33 µm. This formulation produces droplets of a size comparable to commercial mayonnaises, and the results of this study fall within a region where the product is stable. The emulsion quality was directly related to droplet size, and high amounts of energy are needed to generate emulsion droplets; the size of the droplets produced by homogenization is a direct function of the rotational speed, which stands in for the energy of homogenization. When the droplet size is reduced, the amount of energy required to produce it also increases. However, completing droplet size reduction, which takes more energy, is necessary for achieving droplet size distribution uniformity. Emulsion preparation at 9500rpm incorporated mild heat (52°C) into the system and demonstrated greater emulsion homogeneity. The results show that the pH (5.5) and size of the ingredients had a significant effect (p < .05) on the emulsion droplet size.
Specifically, the carotenoids in carrot pomace powder are responsible for the emulsion’s vibrant orange hue (). However, color values can be drastically altered by flower pollen and oils. The lightness-darkness is displayed by the L* value, with 0 indicating complete darkness and 100 displaying full brightness. The brightness of an emulsion (L*) was found to be around 95, and average a* and b* values are significantly affected (p < .05) by the presence of flower pollen, buttermilk, and an emulsion of oils. The a* (redness) value (6.42 ± 0.05) and the b* (yellowness) value (26.77 ± 0.44) of the emulsion are possibly due to the high carotenoid content of the carrot pomace powder. The positive b* value signifies a rise in the yellow shade of the emulsion. From aforesaid, it is clear that the emulsion’s ingredient combinations yielded a b* value higher than the a* value, suggesting that the emulsion might impart a yellow hue to food products and be used as a natural source of colorant in a wide variety of foods. The hue angle of 81.24 confirmed this as well since a hue angle of 90 indicates yellow, and hues of 0, 360, 180, and 270 represent red, green, and blue hues, respectively.[Citation29] As a result, we can conclude that the emulsion is in the red-yellow quadrant, leaning more toward the yellow side. Furthermore, the chroma value of 75.62 represents the strength and purity of the color. Our findings are consistent with those of Tiwari et al.[Citation30] and Baria et al.,[Citation31] who found that carotenoids-rich extracts of mango pulp and carrot pomace led to a higher percentage rise in b* value than a*.
A negative zeta potential value (−35.05 ± 3.04 mV) was observed in lecithin-stabilized emulsions (p < .05) as shown in . Important elements for the stabilization of the emulsions are the zeta potential of the emulsions and the repulsive force between the droplets.[Citation32] The stability of emulsions depends on the features of the interfacial film formed of lecithin and therefore, inducing a negative surface charge on the droplets of the emulsion through ionization of the lipids is one way in which they aid in the emulsion’s stability and lead to lower zeta potential values. Moreover, the concentration of lecithin and particle size of emulsion impact on zeta potential and stability of emulsion.[Citation33]
Textural parameters like firmness, adhesiveness, and cohesiveness of CPBE sample are summarized in . The obtained textural parameters results showed that oils used for the formulation of emulsion decide the textural characteristics of the emulsion, and the present work clearly emphasized the suitability of selected oils.
Physicochemical, texture, and particle size characteristics evaluation of butter
shows the physicochemical analysis of butter formulated with CPBE. As a control, the commercially produced butter “Buterbrodnoe” was chosen. The moisture content of the experimental butter was lower than the control sample (p < .05). It might be due to the water-holding capacity of carrot pomace[Citation34] and buttermilk powder used to improve low-fat yogurt’s rheological and storage properties.[Citation35] The introduction of whey protein concentrates into cream, which contains water-soluble proteins in its composition, helps to accelerate the formation of stable foam, reduces the process of oil formation, and contributes to less fat discharge into buttermilk.[Citation36] The use of methods of enhanced foaming due to the introduction of whey protein concentrate and foam destruction in their sequential combination provides complete degreasing of the entire volume of cream, destruction of the shells of fat globules, the release of their constituent components of solid and low-melting glycerides, coagulation of these components, and the formation of butter grains. Therefore, churning time decreased during butter production. Too much fat in the cream does not allow efficient use of the equipment, and substantial losses of fat with buttermilk can occur, so the use of the KSB-60 preparation is effective in the butter formation process. It could be due to the particle size of butter grains formed during the pasteurization, aging, and churning of cream processes. Similar to our work, the authors[Citation37] noted that dry buttermilk powder improves the sensory properties of cheese and promotes the release of fatty acids, which makes the cheese softer.
Table 3. Physicochemical characteristics of butter formulated with the CPBE.
In comparison to the control sample, “Asylum” experimental butter is a more abundant source of vitamins (B1, E, PP, C, and β-carotene) and minerals. Increasing the fortification of butter products with vitamins and minerals offered by CPBE during production could be potentially beneficial to public health. The results indicate that CPBE increased the quantity of carbohydrates and proteins while reducing the quantity of fat and had a noticeable impact on its energy value (p > .05).
The particle size values of the experimental butter and the control butter were almost the same (p > .05) (). The span value of butter samples was 0.968 (control sample) and 1.132 for the butter “Asylum.” The mean particle size (D [3.2]) of the experimental butter was higher than the control sample. The d(0.1), d(0.5), and d(0.9) values all followed the same pattern. This might be due to the proper separation of buttermilk from butter grains during the processes of churning, butter washing, and thermomechanical treatment of the butter. The addition of CPBE might increase the particle size of the butter grains. Butter’s structure is not set by a single variable but rather by the interrelationship of many. Crystal polymorphism, as well as the quantity and size of fat crystals, are affected by factors such the cream’s water content, chemical profile, and temperature during the buttermaking process.[Citation38] shows the particle size distribution of butter samples. For the butter samples, the particle size distribution was broad, ranging from 50 to 300 μm, with a peak at around 100 μm. Those insignificant peaks at 2 and 20 μm were disregarded. As a result, both samples of butter had a very similar (monomodial) distribution.
Table 4. Texture, particle size, and sensory characteristics of butters.
Properties of spreadability (firmness and work of shear) were determined in butter samples after storage at 4°C for 24 h (). The addition of CPBE noticeably altered the spreadability of butter (p < .01). Firmness and work of shear value were 226.14 N and 243.94N s for control butter and 240.85 N and 322.32 N s for butter “Asylum,” respectively. The butter “Asylum” showed lower spreadability than the control sample. According to Rohm et al.[Citation39] research, butter color has a significant impact on spreadability. Customers preferred and referred to the more yellow butter as being “easier to spread.” According to research by Eissa et al.,[Citation40] cheeses scored higher in sensory evaluation when a* and b* were higher and L* was lower. Moreover, the lower moisture content could affect the spreadability of the butter “Asylum.” As described by the authors[Citation41] butter with a small fat globule size has softer and higher spreadable characteristics. However, the texture of butter depends on many interrelated factors, and there is no single parameter that can explain firmness.[Citation38] The lower spreadability of the butter “Asylum” may be due to the presence of unsaturated fatty acids from CPBE and the crystal lattice formation with CPBE, which resulted in strong cross-linkages that resulted in lower spreadability than the control sample.
Effect of CPBE incorporation on butter “Asylum” color during refrigerated storage
Color parameters for control butter and butter “Asylum” are shown in and the visual determination of fresh butter after 2 days of refrigerated storage presented in . It can be perceived that L* (lightness), a* (redness), and b* (yellowness) were changed in all treatments during 60 days of storage. As expected, the CPBE-added butter showed more redness and yellowness, consistent with the green and yellowish tones related with the presence of chlorophylls and carotenoids in the ingredients of CPBE.[Citation42] The carrot pomace-based emulsion has a yellow-orange color, homogeneous, creamy consistency like mayonnaise with visible well distributed particles of carrot pomace (Fig. S1). In addition, the L*, a*, and b* values were largely affected by CPBE incorporation. Compared to L* and a*, the b* color parameter of butter incorporated with CPBE showed a greater value, indicating that the butter color is yellower due to CPBE addition (p < .05), and this is due to its richness in yellow pigments coming from carotenoids. Furthermore, L* values were almost the same at the beginning of the storage period, remained practically constant and then decreased. These color changes could be due to the isomerization of carotenoids and degradation of compounds due to oxidation.[Citation43] For butter production, the butter color, b*, is a key parameter, and O’Callaghan et al.[Citation23] reported that the yellowness (b*) was directly correlated with the trans--carotene content. Similarly, carrots have been found to have α-carotene levels ranging from 22 to 106.5 µg/g. Only through diet can carotenoids be consumed, making carotenoid fortification a subject of study, especially given the possible health benefits of carotenoids. The color changes in CPBE incorporated butter could be also due to the partial precipitation of unstable suspended particles and oxidation reactions.[Citation44] The results regarding the changes in color parameters are in good agreement with the results of Vukoja et al.,[Citation43] where they documented a decrease in color attributes of regular, extra, and light tart cherry jams stored at room temperature for 8 months. Authors[Citation5] also noted a decrease in color attributes in traditional Tunisian butter production incorporated with tomato processing by-products (seed and peel). Šeregelj et al.[Citation45] reported that carotenoids from carrot waste encapsulated in alginate beads and used in a yogurt product at concentrations of 2.5 and 5 g per 100 g maintained its microbiological and physiochemical properties while showing increased antioxidant activity after 28 days of storage at 4°C.
Table 5. Effect of CPBE addition and storage on color, TPC, DPPH, and oxidative stability parameters of the functional butter “Asylum”.
Effect of CPBE incorporation on TPC, DPPH, and oxidative stability of butter during refrigerated storage
shows changes in TPC and DPPH of CPBE-incorporated functional butter from 0 to 60 days of storage. Total polyphenol content (TPC) and free radical scavenging activity (DPPH) were significantly (p < .05) increased in CPBE-enriched butter compared to control after formulation. This is consistent with previous studies on carrot pomace powder and liquid oils, which also showed higher bioactive compound contents. Kamiliglu et al.[Citation46] reported the phenolic range of 54 to 202 mg GAE/100 g dw. Haq et al.[Citation47] reported the phenolics range of 39.136 to 44.675 mg gallic acid equivalent/100 g FW and Olawuyi & Lee[Citation48] demonstrated 34.21 mg GAE/100 g in 15% carrot pomace-enriched muffin. Possible causes for the discrepancy between reported values include variations in the carrot cultivars used for the research, the extraction procedures, and the reporting formats. However, the mean values of TPC and DPPH after 60 days of storage at 4°C significantly (p < .05) decreased in control sample but not in the CPBE-enriched butter, indicating that the inclusion of CPBE was more effective in preserving the polyphenolic content and antioxidants in the butter during storage. The decline in TPC and DPPH values during storage could be attributed to the degradation, oxidation, and polymerization of polyphenolic compounds, which are responsible for the antioxidant potential in food.[Citation44,Citation46] The treatment with CPBE also showed less reduction in DPPH values during storage, likely due to the higher amounts of polyphenolic and antioxidant compounds present in carrot powder, pollen, and liquid oils. These compounds have an inhibitory effect on the enzymes responsible for oxidative reactions and help maintain the higher retention of antioxidant potential in the samples.[Citation49] Overall, the use of CPBE was found to be effective in preserving the polyphenolic content and antioxidants in the CPBE-incorporated butter during storage. This is consistent with a similar study on yogurt supplemented with carrot waste extract, which showed higher stability of phenolic compounds and antioxidant activities during storage.[Citation45]
Fat oxidation causes rancid odors, discoloration, nutritional loss, and harmful compounds synthesis. Hydroperoxides are the primary oxidation byproducts; they can further deteriorate into secondary oxidation byproducts. The effect of CPBE incorporation on the oxidative stability of butter was evaluated using peroxide index. The highest peroxide level measured in this investigation was below the permissible limit (5meq/kg) specified in the Codex Alimentarius Commission.[Citation50] Shelf-life values were based on this limit. As shown in , PV of the butter added with CPBE increased with the storage time, and higher values (p < .05) were observed in control. The lower peroxide values (p < .05) registered in butter incorporated with CPBE compared to control can be attributed to the role of carotenoids in preventing fat oxidation. The oxidative stability of food lipids was improved in the presence of these phytochemicals. Thus, studies have shown that carotenoids are very potent antioxidants which interrupt the chain of free radicals involved in self-oxidation. These phytochemicals can scavenge singlet molecular oxygen and peroxide radicals.[Citation51] Lipid oxidation is a significant factor that affects the quality of oils and fats during storage. The use of natural antioxidants has been shown to reduce oxidation in margarine, as reported in previous studies.[Citation4,Citation20] The present research involved the development of a carrot pomace-based emulsion that could enhance the nutritional value of butter by providing it with ω-3 fatty acids. This innovative approach could pave the way for a new range of functional foods.
The pH values of the butter samples noticeably differed, probably due to the added probiotic EM “Kurunga.” Similar data were described by Erkaya et al.[Citation52] when the pH of butter using different probiotic strains changed during storage. It may indicate the inhibitory effect of carrot pomace and herbal oils on the lactic acid bacteria growth, which was also reported by Ayar et al.[Citation53] The level of this parameter remained almost unchanged during the whole experimental period.
Malonaldehyde and TBA reactive substances are produced as a result of oxidation of polyunsaturated fatty acids. TBA values of CPBE treated and control butter samples are shown in . According to analysis after 60 days, TBA values of CPBE-added butter sample were generally lower than that of the control butter. In this study, oxidative stability of butter was improved through the phenolic compounds of carrot pomace and herbal oils, without any addition of antioxidants. Bule et al.[Citation54] noted that butter incorporated with leaf extract of Koseret was more effective in retardation of oxidation, lowering microbial load and has better sensory acceptance than other samples.
Essential amino acid and fatty acid content of butter
The profile of amino acids (AAs) is an important factor in evaluating the value of any animal product. CPBE was able to add to the formulation’s enrichment of soluble and insoluble dietary fiber, essential amino acids, and unsaturated fatty acids, all of which increased the final product’s biological value. reviews the effect of the incorporation of the CPBE on the amino acid profile of the butter. It can be observed that the butter “Asylum” content of eight essential amino acids exceeded (p < .05) the control sample of the butter “Buterbrodnoe.” The content of valine exceeds 1.7 times, isoleucine exceeds 1.63 times, leucine 2.58 times, lysine 1.3 times, methionine 2.5 times, threonine 1.25 times, tryptophan 2.1 times, phenylalanine by 1.95 times. The obtained data show that the butter “Asylum” is more beneficial to health than the control sample (butter “Buterbrodnoe”).
Figure 4. Comparative analysis of the content of essential amino acids in the butter “Asylum” and the control sample.
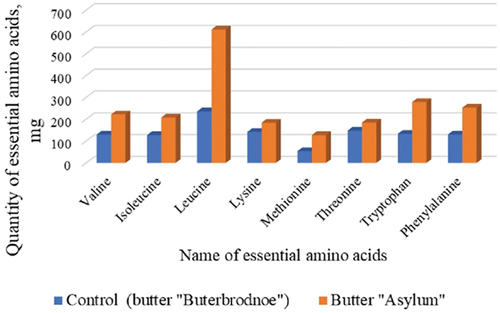
This higher concentration of AAs in the butter “Asylum” is most likely due to the protein content in CPBE (14.05%). The increase in the amount of essential amino acids in butter “Asylum” in comparison with the control sample (butter “Buterbrodnoe”) is associated with the addition of dry buttermilk, plant oils, and pollen to the formulation and pollen also has antioxidant properties.[Citation55] It is known that pollen is a source of biologically active substances, free amino acids and it is often used as a biologically active additive in functional nutrition. Thus, this explains the increase in essential amino acids in the obtained butter.
To maintain normal health, each person needs to get about 2.5 g of fatty acids (FA) every day. At the same time, for their optimal ratio in the body, there should be more fatty acids of plant origin. They are of the greatest importance for the human cardiovascular system because they lower blood cholesterol levels and prevent the development of atherosclerosis.[Citation56] Linoleic and linolenic acids, two types of polyunsaturated fatty acids (PUFA), are crucial to human health because they serve as membrane structural elements and promote healthy growth and adaptation.[Citation57] Linoleic acid is unique among polyunsaturated fatty acids since it is the precursor to arachidonic acid, the most physiologically active acid in this family. The recommended daily allowance (RDA) for ω-6 fatty acids in adults is 8-10 g, while the RDA for ω-3 fatty acids is 0.8–1.6 g.[Citation58] The ideal ratio of ω-6 to ω-3 fatty acids in the diet is 5–10:1. shows the findings of the fatty acid composition of “Asylum” butter and a control sample.
Table 6. Fatty acid compositions of butter prepared with CPBE.
The results of the fatty acids content analysis revealed that the addition of carrot pomace emulsion had a great impact on its final product. Therefore, the saturated fatty acids (SFA) found in the butter “Asylum” were 16.343% palmitic, 20.085% stearic, 2.631% myristic acids, 39.418% was composed of monounsaturated fatty acids (
MUFA), and 15.611% was composed of polyunsaturated fatty acids (
PUFA). The results indicated that the addition of vegetable oils had a significant effect on the total content of fatty acids (p < .05), and increased the content of
PUFA by 8.67 times, including linoleic by 10.34 times, and linolenic by 6.33. Similarly, a study by Złoch et al.[Citation8] reported an increase in monounsaturated fatty acids in butter can extend the shelf life of butter in the future. The number of ω-3 fatty acids in butter “Asylum” has increased 105.66 times due to the addition of flaxseed oil into the formulation of the vegetable fat emulsion. The content of ω-3 fatty acids, dietary fiber, and lignan has attracted food technologists to explore their potential in the food industry.[Citation59] The manufacture of butter with CPBE resulted in butter with lower amounts of saturated fat and higher amounts of unsaturated fat, reducing the ratio of saturated and unsaturated fat, which increases the nutritional and biological value of butter “Asylum.”
Microbiological analysis
The safety of butter can be compromised by the entry of microorganisms from cream and equipment surfaces, leading to the accumulation of mycotoxins. Proteolytic microorganisms like lactobacillus, yeasts, and Pseudomonas spp. can cause deterioration in butter quality.[Citation53] The study’s microbiological results () showed that bacterial counts increased with extended storage time. In this investigation, the addition of CPBE was effective in controlling Coliforms, Staphylococcus aureus, and Psychotropic bacteria (PTC). When dairy products are produced under poor hygiene conditions, the increase in total psychotropic bacteria is more significant. At day 0, the control butter had a PTC level of 2.16log CFU/g, while CPBE treated butter had none detected. After 60 days of storage, PTC levels reached 8.78 and 5.45log/CFU in control and CPBE-treated butter, respectively, indicating that CPBE addition inhibited the growth of PTC in butter. The CPBE ingredients’ inhibitory effect on Gram-negative bacteria populations, which may be due to the emulsion’s phenols and flavonoids,[Citation60] could be attributed to the outcomes. Furthermore, the antibacterial activity of carrot pomace powder contributed to the product’s microbiological stability. The CPBE treatment also inhibited S. aureus, which was not detected until day 45. According to Bello et al.,[Citation61] the aqueous extract of carrot peel (concentrations 25-200 mg/mL) has bacteriostatic effects against S. aureus and E. coli.
The addition of CPBE to butter resulted in significantly lower yeast and mold counts compared to the control during storage (p < .01), according to species known to cause spoilage of butter. This finding is consistent with a study by Erkaya et al.[Citation52] which showed that mold-yeast counts in butter varied depending on storage time. Although the yeast/mold count increased over time in both samples, there were no statistically significant differences (p > .05) between the two butters during storage. Other studies have also investigated the antimicrobial effects of plant extracts on butter. Ayar et al.[Citation53] and Vidanagamage et al.[Citation62] found that oregano and sage extracts, as well as cinnamon extracts, had the greatest inhibitory effects on yeast and molds and extended the shelf life of butter.
Table 7. Microbiological changes of butter during refrigerated storage (4°C).
Sensory evaluation
presents the sensory evaluation results of the butter “Asylum.” The incorporation of the carrot pomace-based emulsion did not have a significant effect on the structure or overall acceptability of the butter; however, it was observed that the color scores were significantly different at p < .05 level. The color of the butter was slightly darker due to the presence of carrot pomace and pollen in the emulsion. The butter treated with CPBE exhibited a slightly firmer texture. The flavor of the butter was preferred by the panelists and was improved by adding CPBE compared to the control. Similar findings were reported by Erkaya et al.[Citation52] who found that the color scores were affected by the addition of probiotic strains. Additionally, da Silva et al.[Citation63] showed that the sensory properties of probiotic butter containing encapsulated L. acidophilus were acceptable and had potential for commercialization.
presents the sensory evaluation results of the butter “Asylum.” The incorporation of the carrot pomace-based emulsion did not have a significant effect on the structure or overall acceptability of the butter; however, it was observed that the color scores were significantly different at p < .05 level. The color of the butter was slightly darker due to the presence of carrot pomace and pollen in the emulsion. The butter treated with CPBE exhibited a slightly firmer texture. The flavor of the butter was preferred by the panelists and was improved by adding CPBE compared to the control. Similar findings were reported by Erkaya et al.[Citation52] who found that the color scores were affected by the addition of probiotic strains. Additionally, da Silva et al.[Citation63] showed that the sensory properties of probiotic butter containing encapsulated L. acidophilus were acceptable and had potential for commercialization.
Conclusion
In conclusion, this study revealed that adding carrot pomace-based emulsion to butter production can be an effective approach to improve its nutritional value, microbiological safety, and oxidative stability. The physicochemical properties of the butter were also enhanced, with improved pH, fat content, and moisture levels. The study found that CPBE addition resulted in a slightly firmer body and texture of the butter and improved its flavor, making it more preferable to panelists compared to the control. The thermal stability of the butter was also higher in the CPBE-treated samples, indicating good quality. Although the spreadability behavior of the butter decreased after adding CPBE, the addition of vegetable fat emulsion in butter production may have potential health benefits due to the additional vitamins, minerals, and fiber from the emulsion’s other ingredients such as liquid oils, pollen, and culture. Furthermore, the use of CPBE in butter production could also have potential applications in the functional food industry. The high nutrient content of carrot pomace-based emulsion could make it an attractive ingredient for developing food formulations, such as baked goods or spreads, and studying the effect of CPBE addition on the sensory and nutritional properties of these products. Additionally, more research is needed to investigate the effects of long-term storage on the nutritional and sensory properties of CPBE-enriched butter, as well as the efficacy of CPBE addition in different butter-making processes. Figure S1, Tables S1-S3; Figure S1: Color of CPBE; Table S1: Physical and chemical parameters of milk for butter production; Table S2: Organoleptic characteristics of milk for butter production; Table S3: Microbiological indicators of milk for butter production.
Institutional review board statement
The study was conducted in accordance with the Declaration of Helsinki and approved by the Ethics Committee of Shakarim State University, Semey, Kazakhstan – protocol #96. The participants provided their written agreement to participate in this study. Informed consent was obtained from all subjects involved in the study.
Supplemental Material
Download ()Acknowledgments
Authors would like to thank the Testing Regional Engineering Laboratory of the Scientific Center for Radioecological Research at “NutriTest” (Almaty, Kazakhstan) for helping to perform analyses.
Disclosure statement
No potential conflict of interest was reported by the author(s).
Data availability statement
The authors confirm that the data supporting the findings of this work are available within the article and its supplementary materials.
Supplementary material
Supplemental data for this article can be accessed online at https://doi.org/10.1080/10942912.2023.2251718
References
- Guiné, R. P. F.; Florença, S. G.; Barroca, M. J.; Anjos, O. The Link Between the Consumer and the Innovations in Food Product Development. Foods. 2020, 9(9), 1317. DOI: 10.3390/foods9091317.
- Román, S.; Sánchez-Siles, L. M.; Siegrist, M. The Importance of Food Naturalness for Consumers: Results of a Systematic Review. Trends Food Sci. Tech. 2017, 67, 44–57. DOI: 10.1016/j.tifs.2017.06.010.
- Bhardwaj, K.; Najda, A.; Sharma, R.; Nurzyńska-Wierdak, R.; Dhanjal, D. S.; Sharma, R.; Manickam, S.; Kabra, A.; Kuča, K.; Bhardwaj, P., et al. Fruit and Vegetable Peel-Enriched Functional Foods: Potential Avenues and Health Perspectives. Evid. Based Complement Alternat. Med. 2022, 2022, 1–14. DOI: 10.1155/2022/8543881.
- Ullah, R.; Nadeem, M.; Imran, M.; Khan, M. K.; Mushtaq, Z.; Asif, M.; Din, A. Effect of Microcapsules of Chia Oil on Ω-3 Fatty Acids, Antioxidant Characteristics and Oxidative Stability of Butter. Lipids. Health Dis. 2020, 19(1), 10. DOI: 10.1186/s12944-020-1190-5.
- Abid, Y.; Azabou, S.; Jridi, M.; Khemakhem, I.; Bouaziz, M.; Attia, H. Storage Stability of Traditional Tunisian Butter Enriched with Antioxidant Extract from Tomato Processing By-Products. Food Chem. 2017, 233, 476–482. DOI: 10.1016/j.foodchem.2017.04.125.
- Denisov, S. V. inventor. 2020. Functional Butter. RU Patent No. 2733292 C2.
- Ewe, J. A.; Loo, S. Y. Effect of Cream Fermentation on Microbiological, Physicochemical, and Rheological Properties of L.Helveticus-Butter. Food Chem. 2016, 201, 29–36. DOI: 10.1016/j.foodchem.2016.01.049.
- Złoch, M.; Rafińska, K.; Sugajski, M.; Buszewska-Forajta, M.; Walczak-Skierska, J.; Railean, V.; Pomastowski, P.; Białczak, D.; Buszewski, B. Lacticaseibacillus paracasei as a Modulator of Fatty Acid Compositions and Vitamin D3 in Cream. Foods. 2022, 11(11), 1659. DOI: 10.3390/foods11111659.
- Gundogdu, E.; Cakmakci, S.; Hayaloglu, A. A. Effects of Starter Culture and Storage on Volatile Profiles and Sensory Characteristics of Yogurt or Cream Butter. Mljekarstvo. 2020, 70(3), 184–200. DOI: 10.15567/mljekarstvo.2020.0305.
- Menezes, C. A.; Magalhães, L. B.; da Silva, J. T.; da Silva Lago, R. M. R.; Gomes, A. N.; Ladeia, A. M. T.; Vianna, N. A.; Oliveira, R. R. Ultra-Processed Food Consumption is Related to Higher Trans Fatty Acids, Sugar Intake, and Micronutrient-Impaired Status in Schoolchildren of Bahia, Brazil. Nutri. 2023, 15(2), 381. DOI: 10.3390/nu15020381.
- Nasirpour-Tabrizi, P.; Azadmard-Damirchi, S.; Hesari, J.; Khakbaz Heshmati, M.; Savage, G. P. Production of a Spreadable Emulsion Gel Using Flaxseed Oil in a Matrix of Hydrocolloids. J. Food Process Preserv. 2020, 44(8), e14588. DOI: 10.1111/jfpp.14588.
- Firdaus, M. A.; Mishra, S. Industrial Trans-Fatty Acid Intake Associated with Coronary Heart Disease Risk: A Review. J. Nutr. Food Sci. 2021, 11, 806. DOI: 10.35248/2155-9600.21.11.806.
- Bascuas, S.; Salvador, A.; Hernando, I.; Quiles, A. Designing Hydrocolloid-Based Oleogels with High Physical, Chemical, and Structural Stability. Front. Sustain. Food Syst. 2020, 4, 111. DOI: 10.3389/fsufs.2020.00111.
- Zhao, W.; Wei, Z.; Xue, C. Recent Advances on Food-Grade Oleogels: Fabrication, Application and Research Trends. Crit. Rev. Food Sci. Nutr. 2022, 62(27), 7659–7676. DOI: 10.1080/10408398.2021.1922354.
- Samet-Bali, O.; Ayadi, M. A.; Attia, H. Traditional Tunisian Butter: Physicochemical and Microbial Characteristics and Storage Stability of the Oil Fraction. LWT-Food Sci. Tech. 2009, 42(4), 899–905. DOI: 10.1016/j.lwt.2008.11.007.
- Sert, D.; Mercan, E.; Dertli, E. Characterization of Lactic Acid Bacteria from Yogurt-Like Product Fermented with Pinecone and Determination of Their Role on Physicochemical, Textural, and Microbiological Properties of Product. LWT - Food Sci. Tech. 2017, 78, 70–76. DOI: 10.1016/j.lwt.2016.12.023.
- Glibowski, P.; Zarzycki, P.; Krzepkowska, M. The Rheological and Instrumental Textural Properties of Selected Table Fats. Int. J. Food. Prop. 2008, 11(3), 678–686. DOI: 10.1080/10942910701622599.
- Okuskhanova, E.; Assenova, B.; Rebezov, M.; Amirkhanov, K.; Yessimbekov, Z.; Smolnikova, F.; Nurgazezova, A.; Nurymkhan, G.; Stuart, M. Study of Morphology, Chemical, and Amino Acid Composition of Red Deer Meat. Vet. World. 2017, 6(6), 623–629. D O I: 10.14202%2Fvetworld.2017.623-629.
- Ndaw, S.; Bergaentzlé, M.; Aoudé-Werner, D.; Hasselmann, C. Extraction Procedures for the Liquid Chromatographic Determination of Thiamin, Riboflavin, and Vitamin B6 in Foodstuffs. Food Chem. 2000, 71(1), 129–138. DOI: 10.1016/S0308-8146(00)00135-7.
- Nadeem, M.; Imran, M.; Taj, I.; Ajmal, M.; Junaid, M. Omega-3 Fatty Acids, Phenolic Compounds and Antioxidant Characteristics of Chia Oil Supplemented Margarine. Lipids. Health Dis. 2017, 16(1), 102. DOI: 10.1186/s12944-017-0490-x.
- Brand-Williams, W.; Cuvelier, M. E.; Berset, C. Use of a Free Radical Method to Evaluate Antioxidant Activity. Food Sci. Technol. 1995, 28(1), 25–30. DOI: 10.1016/S0023-6438(95)80008-5.
- Ayar, A.; Özcan, M.; Akgül, A.; Akin, N. Butter Stability as Affected by Extracts of Sage, Rosemary and Oregano. J. Food Lipids. 2001, 8(1), 15–25. DOI: 10.1111/J.1745-4522.2001.Tb00180.X.
- O’Callaghan, T. F.; Faulkner, H.; McAuliffe, S.; O’Sullivan, M. G.; Hennessy, D.; Dillon, P.; Kilcawley, K. N.; Stanton, C.; Ross, R. P. Quality Characteristics, Chemical Composition, and Sensory Properties of Butter from Cows on Pasture versus Indoor Feeding Systems. J. Dairy. Sci. 2016, 99(12), 9441–9460. DOI: 10.3168/jds.2016-11271.
- McClements, D. J.; Jafari, S. M. Improving Emulsion Formation, Stability and Performance Using Mixed Emulsifiers: A Review. Adv. Colloid Interface Sci. 2018, 251, 55–79. DOI: 10.1016/j.cis.2017.12.001.
- Pamunuwa, G. K.; Karunaratne, D. N. Liposomal Delivery of Plant Bioactives Enhances Potency in Food Systems: A Review. J. Food Qual. 2022, 2022, 1–11. DOI: 10.1155/2022/5272592.
- Anjali, C. H.; Dash, M.; Chandrasekaran, N.; Mukherjee, A. Anti Bacterial Activity of Sunflower Oil Microemulsion. Int. J. Pharm. Pharm. Sci. 2010, 2, 123–128.
- Debusca, A.; Tahergorabi, R.; Beamer, S. K.; Partington, S.; Jaczynski, J. Interactions of Dietary Fibre and Omega-3-Rich Oil with Protein in Surimi Gels Developed with Salt Substitute. Food Chem. 2013, 141(1), 201–208. DOI: 10.1016/j.foodchem.2013.02.111.
- Guggisberg, D.; Chollet, M.; Schreier, K.; Portmann, R.; Egger, L. Effects of Heat Treatment of Cream on the Physical–Chemical Properties of Model Oil-In-Buttermilk Emulsions. Int. Dairy. J. 2012, 26(1), 88–93. DOI: 10.1016/j.idairyj.2012.01.008.
- Malekjani, N.; Emam-Djomeh, Z.; Hashemabadi, S. H.; Askari, G. R. Internal and External Color Development Kinetics During Microwave Assisted Fluidized Bed Drying of Hazelnut. JAST 2017, 19, 613–626. http://jast.modares.ac.ir/article-23-3620-en.html
- Tiwari, S.; Upadhyay, N.; Malhotra, R. Three Way ANOVA for Emulsion of Carotenoids Extracted in Flaxseed Oil from Carrot Bio-Waste. Waste Manag. 2021, 121, 67–76. DOI: https://doi.org/10.1016/j.wasman.2020.11.037.
- Baria, B.; Upadhyay, N.; Singh, A. K.; Malhotra, R. K. Optimization of ‘Green’ Extraction of Carotenoids from Mango Pulp Using Split Plot Design and Its Characterization. LWT-Food Sci. Tech. 2019, 104, 186–194. DOI: 10.1016/j.lwt.2019.01.044.
- Schuh, R. S.; Bruxel, F.; Teixeira, H. F. Physicochemical Properties of Lecithin-Based Nanoemulsions Obtained by Spontaneous Emulsification or High-Pressure Homogenization. Quím. nova. 2014, 37, 1193–1198. DOI: 10.5935/0100-4042.20140186.
- Ponphaiboon, J.; Limmatvapirat, S.; Limmatvapirat, C. Influence of Emulsifiers on Physical Properties of Oil/Water Emulsions Containing Ostrich Oil. Key Eng. Mater. 2018, 777, 592–596. DOI: 10.4028/www.scientific.net/KEM.777.592.
- Ma, S.; Ren, B.; Diao, Z.; Chen, Y.; Qiao, Q.; Liu, X. Physicochemical Properties and Intestinal Protective Effect of Ultra-Micro Ground Insoluble Dietary Fibre from Carrot Pomace. Food Funct. 2016, 7(9), 3902–3909. DOI: https://doi.org/10.1039/C6FO00665E.
- Zhao, L.; Feng, R.; Mao, X. Addition of Buttermilk Powder Improved the Rheological and Storage Properties of Low-Fat Yogurt. Food Sci. Nutr. 2020, 8(7), 3061–3069. DOI: 10.1002/fsn3.1373.
- Bacenetti, J.; Bava, L.; Schievano, A.; Zucali, M. Whey Protein Concentrate (WPC) Production: Environmental Impact Assessment. J. Food Eng. 2018, 224, 139–147. DOI: 10.1016/j.jfoodeng.2017.12.018.
- Hickey, C. D.; O’Sullivan, M. G.; Davis, J.; Scholz, D.; Kilcawley, K. N.; Wilkinson, M. G.; Sheehan, J. J. The Effect of Buttermilk or Buttermilk Powder Addition on Functionality, Textural, Sensory and Volatile Characteristics of Cheddar-Style Cheese. Food. Res. Int. 2018, 103, 468–477. DOI: https://doi.org/10.1016/j.foodres.2017.09.081.
- Rønholt, S.; Mortensen, K.; Knudsen, J. C. The Effective Factors on the Structure of Butter and Other Milk Fat-Based Products. Compr. Rev. Food Sci. Food Saf. 2013, 12(5), 468–482. DOI: 10.1111/1541-4337.12022.
- Rohm, H.; Strobl, M.; Jaros, D. Butter Colour Affects Sensory Perception of Spreadability. Z. Lebensm. Unters. Forsch. 1997, 205(2), 108–110. DOI: 10.1007/s002170050134.
- Eissa, H. A.; Abbas, H. M.; Bayoumi, H. M. Water Activity, Color Characteristics and Sensory Properties of Egyptian Gouda Cheese During Ripening. J. Am Sci. 2010, 6(10), 447–453.
- Panchal, B.; Truong, T.; Prakash, S.; Bansal, N.; Bhandari, B. Influence of Fat Globule Size, Emulsifiers, and Cream-Aging on Microstructure and Physical Properties of Butter. Int. Dairy. J. 2021, 117, 105003. DOI: 10.1016/j.idairyj.2021.105003.
- Meléndez-Martínez, A. J.; Böhm, V.; Borge, G. I. A.; Cano, M. P.; Fikselová, M.; Gruskiene, R.; Lavelli, V.; Loizzo, M. R.; Mandić, A. I.; Brahm, P. M. Carotenoids: Considerations for Their Use in Functional Foods, Nutraceuticals, Nutricosmetics, Supplements, Botanicals, and Novel Foods in the Context of Sustainability, Circular Economy, and Climate Change. Annu. Rev. Food Sci. Technol. 2021, 12(1), 433–460. DOI: 10.1146/annurev-food-062220-0132.
- Vukoja, J.; Pichler, A.; Kopjar, M. Stability of Anthocyanins, Phenolics and Color of Tart Cherry Jams. Foods. 2019, 8(7), 255. DOI: 10.3390/foods8070255.
- Younis, K.; Islam, R. U.; Jahan, K.; Yousuf, B.; Ray, A.; Yildiz, F. Effect of Addition of Mosambi (Citrus limetta) Peel Powder on Textural and Sensory Properties of Papaya Jam. Cogent. Food Agric. 2015, 1(1), 1023675. DOI: 10.1080/23311932.2015.1023675.
- Šeregelj, V.; Pezo, L.; Šovljanski, O.; Lević, S.; Nedović, V.; Markov, S.; Tomić, A.; Čanadanović-Brunet, J.; Vulić, J.; Šaponjac, V. T., et al. New Concept of Fortified Yogurt Formulation with Encapsulated Carrot Waste Extract. LWT - Food Sci. Tech. 2021, 138, 110732. DOI: 10.1016/j.lwt.2020.110732.
- Kamiloglu, S.; Ozkan, G.; Isik, H.; Horoz, O.; Van Camp, J.; Capanoglu, E. Black Carrot Pomace as a Source of Polyphenols for Enhancing the Nutritional Value of Cake: An in vitro Digestion Study with a Standardized Static Model. LWT - Food Sci. Tech. 2017, 77, 475–481. DOI: 10.1016/j.lwt.2016.12.002.
- Haq, R.; Kumar, P.; Prasad, K. Physico-Chemical, Antioxidant and Bioactive Changes in Cortex Core Sections of Carrot (Daucus carota Var. Pusa rudhira). J. Food Meas. Charact. 2016, 10(3), 701–708. DOI: 10.1007/s11694-016-9354-8.
- Olawuyi, I. F.; Lee, W. Y. Quality and Antioxidant Properties of Functional Rice Muffins Enriched with Shiitake Mushroom and Carrot Pomace. Int. J. Food Sci. Technol. 2019, 54(7), 2321–2328. DOI: 10.1111/ijfs.14155.
- Khan, M. R.; Syed, A.; Zia, S.; Ahmed, W.; Aadil, R. M.; Manzoor, M. F.; Inam-Ur-Raheem, M.; Abid, M.; Shabbir, M. A.; Qureshi, S., et al. Stabilization and Attributive Amelioration of Sugarcane Juice by Naturally Derived Preservatives Using Aonla and Moringa Extract. Food Sci. Nutr. 2021, 9(6), 3048–3058. DOI: 10.1002/fsn3.2262.
- Codex Alimentarius Commission. Report Of The Sixteenth Session Of The Codex Committee On Fats And Oils. 1999; http://www.codexalimentarius.org/codexhome/en/Last (accessed 12 4, 2020).
- Bhat, T.; Patel, K. Carotenoids: Potent to Prevent Diseases Review. Nat. Prod. Bioprospect. 2020, 10(3), 109–117. DOI: https://doi.org/10.1007/s13659-020-00244-2.
- Erkaya, T.; Ürkek, B.; Doğru, Ü.; Çetin, B.; Şengül, M. Probiotic Butter: Stability, Free Fatty Acid Composition and Some Quality Parameters During Refrigerated Storage. Int. Dairy. J. 2015, 49, 102–110. DOI: 10.1016/j.idairyj.2015.04.011.
- Ayar, A.; Özcan, M.; Akgül, A. Effect of Oregano and Sage Extracts on Microbiological Quality of Molten Butter. Food Sci. Technol. Res. 2007, 10(2), 111–113. DOI: 10.3136/fstr.10.111.
- Bule, G. S.; Awegachew, E. D.; Hassen, Y. Evaluation of Selected Spices and Herb Extracts on Oxidative Stability, Microbial and Sensory Quality of Fermented Cow Milk Butter. J. Food Sci. Nutr. 2022, 5(7), 1–7. DOI: 10.35841/AAJFSN-5.7.131.
- Zuluaga, C.; Martínez, A.; Fernández, J.; López-Baldó, J.; Quiles, A.; Rodrigo, D. Effect of High-Pressure Processing on Carotenoid and Phenolic Compounds, Antioxidant Capacity, and Microbial Counts of Bee-Pollen Paste and Bee-Pollen-Based Beverage. IFSET 2016, 37, 10–17. Part A. DOI: 10.1016/j.ifset.2016.07.023.
- Wei, T.; Liu, J.; Zhang, D.; Wang, X.; Li, G.; Ma, R.; Chen, G.; Lin, X.; Guo, X. The Relationship Between Nutrition and Atherosclerosis. Front. Bioeng. Biotech. 2021, 9, 635504. DOI: 10.3389/fbioe.2021.635504.
- Mariamenatu, A. H.; Abdu, E. M. Overconsumption of Omega-6 Polyunsaturated Fatty Acids (PUFAs) versus Deficiency of Omega-3 PUFAs in Modern-Day Diets: The Disturbing Factor for Their “Balanced Antagonistic Metabolic Functions” in the Human Body. J. Lipids. 2021, 2021, 1–15. DOI: 10.1155/2021/8848161.
- Vannice, G.; Rasmussen, H. Position of the Academy of Nutrition and Dietetics: Dietary Fatty Acids for Healthy Adults. J. Acad. Nutr. Diet. 2014, 114(1), 136–153. DOI: 10.1016/j.jand.2013.11.001.
- Popoola-Akinola, O. O.; Raji, T. J.; Olawoye, B. Lignocellulose, Dietary Fibre, Inulin and Their Potential Application in Food. Heliyon. 2022, 8(8), e10459. DOI: 10.1016/j.heliyon.2022.e10459.
- Nakamura, K.; Ishiyama, K.; Sheng, H.; Ikai, H.; Kanno, T.; Niwano, Y. Bactericidal Activity and Mechanism of Photoirradiated Polyphenols Against Gram-Positive and -Negative Bacteria. J. Agric. Food. Chem. 2015, 63(35), 7707–7713. DOI: 10.1021/jf5058588.
- Bello, M.; Bukuyum, Y. M.; Danraka, R. B. Antibacterial Activity of Daucus Carota (Carrot) Peel Extract on Some Selected Clinical Isolates from Maryam Abacha Women and Children Hospital, Sokoto. Eur. J. Pharm. Med. Res. 2019, 6(4), 45–51.
- Vidanagamage, S. A.; Pathirajea, P. M. H. D.; Pereraa, O. D. A. N. Effects of Cinnamon (Cinnamomum Verum) Extract on Functional Properties of Butter. Procedia. Food Sci. 2016, 6, 136–142. DOI: 10.1016/j.profoo.2016.02.033.
- da Silva, M. N.; Tagliapietra, B. L.; Pivetta, F. P.; dos Santos Richards, N. S. P. Nutritional, Functional and Sensory Profile of Added Butter from Lactobacillus acidophilus Encapsulated and Hyposodium Salt. LWT - Food Sci. Tech. 2022, 161, 113385. DOI: 10.1016/j.lwt.2022.113385.