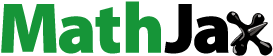
ABSTRACT
The objective of this research was to investigate and compare the effects of various protein or protein-based powders (WPC, SPI, BSA and hemoglobin) addition on transform of texture properties in egg white (EW)/egg yolk (EY) gels. Results showed that the highest hardness value (38.5 N) was detected in BSA-treated EW gels, while the lowest values (46.6 N) were obtained in hemoglobin-treated EY gels. Additionally, significantly increased value of free –SH groups was obtained in SPI/BSA/hemoglobin-treated EW/EY solutions, and increased value of protein solubility was obtained in EY solutions treated by WPC/SPI/BSA/hemoglobin relative to those of the control. The EW solutions treated by BSA/hemoglobin reached significantly higher H0 values than those of other groups. SDS-PAGE analysis showed that the hemoglobin-treated EW solution had slightly intense bands of ovoinhibitor, ovotransferrin and ovalbumin, and the EY solutions treated by WPC/SPI/BSA had higher level of covalent crosslinking. The EW gels treated by WPC/hemoglobin exhibited relatively more ordered protein secondary structures (α-helix and β-sheet) than those of the SPI group. SEM results suggested that WPC/BSA-treated EW gels developed uniform, porous and fiber-like structure, while SPI/BSA-treated EY gels developed rougher structure.
Introduction
Egg white (EW) and yolk (EY) are fundamental ingredients in food processing industry. Egg white, which has excellent foaming, gelling and nutritional properties, is widely used in baked food, meringue, ice cream and souffles, etc.[Citation1] Egg yolk, which combines unquestionably emulsifying, coagulating, gelling and nutritional properties, is also an efficient ingredient in many food products.[Citation2] The quality of egg and egg products is closely related to protein or protein-based gel properties.[Citation3] Protein gelation, coagulation and aggregation are also found responsible for the desirable rheological or textural/shape properties, digestion moderations, 3D network structures, stabilizing foams, dispersions, emulsions[Citation1,Citation4] and particles in food production.[Citation5,Citation6] The formation and textural quality of EW gels, influenced by physical, chemical and biological factors, depend on various pre-treatment methods such as heating, cooling, drying, alkali, enzyme, ion, acid-treating, microwave, magnetic, electric and ultrasonic fields, etc.[Citation6,Citation7] Food gelation/textural attributes, which are also imperative functional characteristics of EY proteins, could be obtained through freezing, heating, pickling and enzyme treatments.[Citation8,Citation9] The textual/gelation behavior of EY could be affected by addition of NaCl, polysaccharides, soy lecithin, alkali and other food ingredients, pH modification, phosphorylation and succinylation.[Citation10]
Even if only egg white or egg yolk proteins could form gels, the textual/gelation properties could be influenced or altered by presence of whey, soybean protein isolate,[Citation11] and blood proteins via protein−protein interactions.[Citation12,Citation13] Whey protein concentrate (WPC) and soybean protein isolate (SPI) are common food gelation proteins, bovine serum albumin (BSA) and hemoglobin, as common and important blood proteins, could add high-by-products values to food and non-food products due to hydration, surface, gelation and sensory properties etc.[Citation13,Citation14]
Thus, this study on textural properties of egg white/yolk gels by application of four methods can further clarify the addition effects of some proteins or protein-based mixture powders on the textural properties of egg white or york, thus shedding lights on the optimization of egg gelation- derived products. Until now, the effects of protein or protein-based powders addition on liquid EW/EY texture properties of gels had not been studied yet. In order to better understand the mechanism in liquid EW/EY texture properties of gels induced by addition of WPC, SPI, BSA and hemoglobin powder, changes in color, texture, water holding capacity (WHC), protein solubility and degradation, surface hydrophobicity, free sulfhydryl (–SH) group, secondary structure and morphology were evaluated.
Materials and methods
Materials and chemicals
Roman pink chicken fresh eggs were purchased from Chongqing Changshou egg market Co., Ltd. (Chongqing, China). The fresh eggs were randomly selected to determine the size (48.93 ± 0.97 g), air cell depth (6.43 ± 0.11 mm), Haugh unit (88.45 ± 1.67), yolk index (0.48 ± 0.01), albumen pH (8.97 ± 0.05), and then carefully hand-broken, separated EW and EY. The samples of liquid EW containing moisture% (86.90 ± 0.07), crude protein% (11.01 ± 0.11) and liquid EY containing moisture% (52.47 ± 0.15), crude fat% (30.97 ± 0.60) and crude protein% (11.01 ± 0.11) were pre-homogenized for 30 s at 11,000×g. WPC and SPI were purchased from Moyihuai Food Co., Ltd (Xiamen, China). BSA and hemoglobin powder, 1-anilino-8-naphthalene-sulfonate (ANS) and 5,’5-dithiobis-(2-nitrobenzoic acid) (DTNB) were obtained from Shanghai Yuanye Biotechnology Co., Ltd. Standard protein marker (15–120 kDa) was purchased from Shanghai Macklin Biochemical Co., Ltd. All other reagents were of analytical grade.
Sample preparation
Liquid EW and EY were prepared and mixed with WPC, SPI, BSA and hemoglobin powders at the concentration of 0.5% (w/w), respectively, the untreated ones as the control, then stirred and homogenized using a FSH-2 high-speed homogenizer (Jiangsu Huanyu Instrument Inc., Changzhou, China) for 1 min at 14,000×g. The gels were obtained by transferring all liquid EW and EY samples into cooking cells (2 cm × 2 cm × 2 cm), cooked at 90°C for 40 min in a water bath according to previous studies.[Citation15] The cooked EW and EY gels were cooled and kept overnight at 4°C until further analysis.
Colour measurement
L* (lightness), a* (redness/greenness) and b* (yellowness/blueness) on the surface of all EW and EY gels were analyzed by Digieye Digital Imaging System (UK, Verivide), which was calibrated for internal light (D65) before analysis (Wang & Hartel, 2022),[Citation16] and the whiteness was determined using formula (1).
Texture measurement
The texture profile analysis (TPA) was carried out with a TA.HD Plus Texture Analyzer (Stable Micro Systems, Surrey, England) fitted with a P50 probe according to the method of Dai et al. (2022).[Citation15] All EW and EY gels were compressed to 50% of their original height at a constant speed of 5 mm/s with the trigger point load of 5 g. The results including hardness, adhesiveness, resilience, cohesiveness, springiness, gumminess and chewiness were calculated and recorded for all gels.
Water holding capacity
Water holding capacity (WHC) of all EW and EY gels was determined according to the concentration method of Li et al. (2018),[Citation8] with slight modifications. Gels along with filter paper were placed into 50 mL centrifuge tubes, centrifuged at 10,000 ×g for 20 min. WHC of the gels was calculated as the ratio of mass of gel after centrifugation to the mass of gel before centrifugation using formula (2).
Free sulfhydryl groups
Free sulfhydryl groups (–SH) of all liquid EW and EY solutions were measured according to the procedure of Dai et al. (2022).[Citation15] All solutions were dissolved in Tris-glycine buffer (pH 8.0, 0.086 M Tris, 0.09 M glycine, 4 mM EDTA) containing 0.5% (w/v) SDS to make the final concentration of 7 mg/mL. The Ellman’s reagent (20 μL) was added to each sample (0.1 mL), then incubated in the dark place for 20 min. After that, the absorbance at 412 nm of all solution mixtures (against the blank) was detected in a Puxi T6-UV-Vis spectrophotometer. The content of free SH groups was calculated using formula (3).
where A412 was the absorbance at 412 nm, C was the sample protein concentration in mg/mL, and D was the dilution factor.
Surface hydrophobicity (H0)
Changes in H0 of liquid EW and EY solutions were determined using an 8-anilino-1-naphthalene-sulfonate (ANS) fluorescent probe.[Citation9,Citation15] The liquid EW and EY samples were diluted to protein concentration (0.1–1 mg/mL) with distilled water. 20 μL of ANS solution (8 mM) was added to 4 mL of samples, mixed and kept in the dark for 3 min. The fluorescence intensity was recorded with excitation wavelength (390 nm) and emission wavelength (470 nm) using Shimadzu-F-700098 fluorophotometer (Japan). H0, was defined as 100% and calculated by the initial slope of the curve of fluorescence intensity (FI) against protein concentration using linear regression analysis.
Protein solubility
With minimal adjustments, changes in protein solubility of liquid EW and EY solutions were determined according to the method of Li et al.. (2018).[Citation8] All samples (1 g) were diluted in distilled water (30 mL), then centrifuged at 10,000 × g for 10 min, Both supernatants and the pellets of all samples were collected, and its protein content further analyzed using Biuret method. The protein concentration was measured at 540 nm using a Puxi T6-UV-Vis spectrophotometer (Beijing Puxi Co., Ltd, Beijing). Solubility was expressed as the ratio of protein content in supernatant to the total protein content in samples.
SDS-PAGE electrophoresis
The protein composition of liquid EW and EY solutions was analyzed by sodium dodecylsulfate polyacrylamide gel electrophoresis (SDS-PAGE) according to the method of Li et al.. (2018),[Citation8] with slight modifications. The 12% separation gel and 4% stacking gel were subjected to electrophoresis at a constant current of 30 mA using a DYCZ-24DN system (Beijing Liuyi Biotechnology Co., Ltd). In brief, the liquid EW and EY solutions were directly diluted to protein concentration of 2 mg/mL, mixed with loading buffer at a ratio of 1:1, boiled for 1 min, then stained with Coomassie brilliant blue R-250. Protein molecular weight of all solutions was evaluated using standard protein marker.
Fourier transform infrared spectroscopy (FTIR)
All EW and EY gels were lyophilized by SCIENTZ-10N freeze dryer (Ningbo Scientz Biotechnology Co., Ltd, Ningbo, China) for FTIR test. ATR-FTIR measurements were performed using a TENSOR 27 FTIR (BURUKER, USA) spectrometer in a range 400–4000 cm−1 for 16 scans with a resolution of 4 cm−1 resolutions, and XT-KBr was utilized as the beamsplitter. The FTIR spectra (quantitative estimation of secondary structure components) identification of all gels was performed with PeakFit software.[Citation8,Citation17]
Morphological analysis
The surface morphology of all EW and EY gels was monitored by Thermo Scientific™ Quattro environmental scanning electron microscope (ESEM) (Thermo Fisher Technology Co., Ltd, Shanghai, China). All air-dried gels were sputter-coated with gold, then examined using ESEM at kV accelerating voltages under 500× and 2000× magnification.[Citation9]
Statistical analysis
The entire experiment was replicated in triplicate to calculate the average values and standard errors unless otherwise stated. All the data were statistically compared among groups by one-way ANOVA and Duncan’s multiple range test with p < .05 in SPSS 16.0 software (SPSS Inc., Chicago, IL, USA).
Results and discussion
Colour
The color parameters (L*, a*, b*, whiteness) and appearance of all EW/EY gel samples were shown in and . The lowest L* values (62.28, 66.05) were found in hemoglobin-treated EW/EY gel samples partly due to dark red color of hemoglobin itself, and the decline in L* values might suggested that hemoglobin used in the study caused a slightly lower degree of denaturation compared to those of control and other protein powder treatment groups.[Citation18]
Figure 1. Visual images of (a) control, (b) WPC, (c) SPI, (d) BSA and (e) hemoglobin-treated egg white gels and (A) control, (B) WPC, (C) SPI, (D) BSA and (E) hemoglobin-treated egg yolk gels. WPC, whey protein concentrate. SPI, soy protein isolate. BSA, bovine serum albumin.
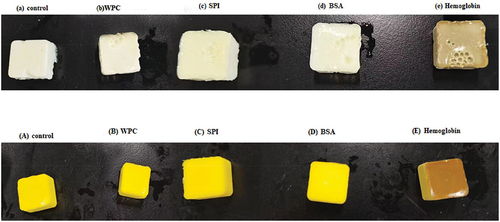
Table 1. Changes in color attributes of egg white and egg yolk gels treated byWPC, SPI, BSA and hemoglobin.
Compared with control EW/EY gels, significant increases (p < .05) of a⁎ values were observed in WPC and hemoglobin-treated gels. The gels treated by hemoglobin showed the highest a⁎ value (7.15) in all EW gels and lowest a⁎ value (7.68) in all EY gels. WPC, SPI and hemoglobin-treated EW gels showed significantly (p < .05) higher b* values, whereas BSA-treated ones showed lower b* values compared with those of the control. Compared to control EY gels, those treated by WPC, SPI, BSA and hemoglobin showed significantly (p < .05) lower b* values. The BSA-treated EW gels exhibited the highest (p < .05) whiteness value (82.25) in all EW gels. The control and BSA-treated EY gels had significantly (p < .05) higher whiteness values than those of other groups. The particular enhancement in whiteness of BSA-treated EW gels could be attributed to the white color of BSA.[Citation19]
Texture
displayed TPA parameters (hardness, adhesiveness, resilience, cohesiveness, springiness, gumminess and chewiness) of all EW/EY gels. No significant differences (p > .05) were found in adhesiveness value of all EW gels, and resilience, cohesion and springiness values of all EY gels were found. The BSA-treated EW gels showed significantly highest values of hardness (38.54 N), gumminess (28.17 N) and chewiness (25.90 N) among all five groups of EW gels, thus maintaining more ordered secondary gel structures due to electrostatic repulsion and covalent bond formations. [.Citation20] [Citation21] The lowest values of hardness (46.59 N) and chewiness (25.65 N) were detected in hemoglobin-treated EY gels, thus indicating suppressed gel formation and unordered secondary gel structures. [20.21]
Table 2. Changes in texture attributes of egg white and egg yolk gels treated by WPC, SPI, BSA and hemoglobin.
Compared with the control, lower springiness value (91.98) was detected in those treated by BSA due to slight incompatibility between BSA and EW proteins. The resilience, cohesion and springiness also serve as indexes to evaluate integrity of the food gel network.[Citation21] The EW gels treated by SPI showed significantly (p < .05) higher values of resilience (44.65%) and cohesion (0.76) than those of other four groups. It was demonstrated that the SPI application improves integrity structure and capability of withstanding deformation of EW gels.[Citation22]
Compared with other hemoglobin-treated EW/EY gels, lower adhesiveness values were obtained especially in WPC, SPI and BSA-treated ones, thus determining that WPC, SPI and BSA-treated EW/EY gels tended to keep food compact during swallow.[Citation22]
WHC
The WHC reflects the water retention ability of gels under external forces and is closely related to variations in protein−water interactions, pore size of gel structures and biopolymer nature.[Citation9,Citation23] displayed WHC of all EW/EY gels. All EY gels had strong WHC higher than 95%, which was in accordance with Li et al.. (2018).[Citation8] However, there were no differences (p > .05) between any of EY gel groups. The WHC values of control, WPC, BSA-treated EW gels were significantly (p < .05) higher compared with those of other groups, partly due to higher gelling properties and more trapped water.[Citation24] It has been revealed that the gels treated with WPC and egg white protein exhibited stronger WHC%,[Citation25] which was also in consistent with our present study. The centrifugation WHC% might have a positive correlation with gel strength attributes,[Citation8] which is consistent with the data of WPC and BSA-treated EW gels. It is also interesting to deliver that the WPC and BSA-treated EW gels might display a stronger WHC against the centrifugation force than that of SPI and hemoglobin-treated ones because of the slight compact building blocks which strongly trapped the water inside the gel network.[Citation9]
Figure 2. Changes in the WHC of (a) liquid egg white gels and (b) liquid egg yolk gels treated by WPC, SPI, BSA and hemoglobin. Values are means ± SE of three replicates measurements. Error bars represent positive standard errors of the mean. Different lowercase letters (a-e) donate significant difference in these treatment group gels (p < .05). Means having no letter do not significantly differ at p < .05. WPC, whey protein concentrate. SPI, soy protein isolate. BSA, bovine serum albumin.
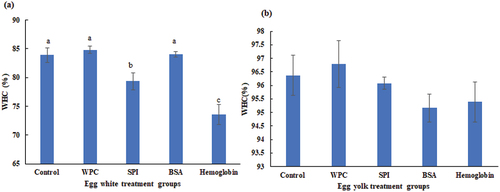
Free sulfhydryl groups, H0 and protein solubility
Changes in free sulfhydryl (–SH) groups, H0 and protein solubility of all solutions of EW/EY are presented in . Sulfhydryl groups, as the most active protein functional groups, could form disulfide bonds through oxidation and influence food gelation properties.[Citation26] As seen in , the EW/EY samples treated by SPI, BSA and hemoglobin displayed significantly (p < .05) higher free –SH groups compared with those of the control. The spatial structure of SPI, BSA and hemoglobin-treated EW/EY solutions might expose part of the embedded sulfhydryl group and hydrophobic core due to increased free sulfhydryl groups.[Citation9,Citation27] The slightly increased free –SH groups treated by those proteins might influence gelation properties.[Citation28]
Figure 3. Changes in the free sulfhydryl group of (a) liquid egg white and (b) liquid egg yolk, surface hydrophobicity of (c) liquid egg white and (d) liquid egg yolk, protein solubility of (e) liquid egg white and (f) liquid egg yolk samples treated by WPC, SPI, BSA and hemoglobin. Values are means ± SE of three replicates measurements. Error bars represent positive standard errors of the mean. Different lowercase letters (a-e) donate significant difference in these treatment group samples (p < .05). Means having no letter do not significantly differ at p < .05. WPC, whey protein concentrate. SPI, soy protein isolate. BSA, bovine serum albumin.
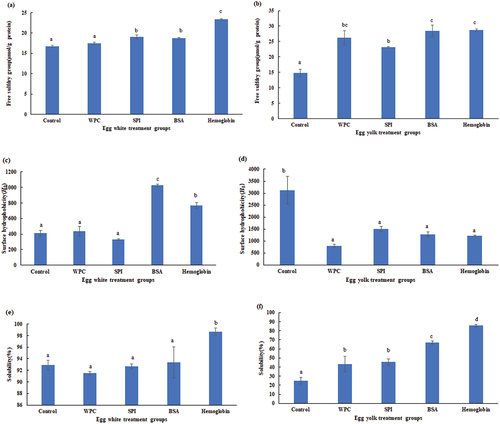
H0, as a structure characteristic to evaluate hydrophobic interactions, conformational changes and tertiary structure of proteins, could contribute to gel network formations.[Citation3] The EW samples treated by BSA/hemoglobin and control EY samples showed significantly (p < .05) higher H0 values than those of other groups, respectively. The increase of H0 might induce more formation of unfolded structures and fluorescent aromatic amino acids transforming from protein gel core to surface.[Citation28] Higher H0 also leads to more protein denaturation, dissociation, expansion of peptide chains, while lower H0 causes protein aggregates.[Citation29] Our finding indicated that all protein-treated EY solutions might have had more protein mixture aggregates, exposing less hydrophobic surface for ANS to bind and wrapping hydrophobic groups in these protein gels.[Citation29]
Protein solubility, as one of the important protein indicators, is also related to food gelation properties.[Citation30] Hemoglobin-treated EW solutions showed significantly (p < .05) the highest protein solubility among those of all groups. Compared to control group, WPC, SPI, BSA and hemoglobin-treated EY solutions showed significantly (p < .05) higher values of protein solubility, thus increasing net charge of final protein gels.[Citation28] According to the research of Kuang et al. (2023)[Citation31] and Ren et al. (2020),[Citation32] higher repulsion forces might also promote protein−solvent interaction and protein unfolding due to better protein solubility.
Electrophoresis
Electrophoresis was performed to observe protein changes in liquid EW/EY solutions treated by various proteins. As shown in , the liquid EW solutions consistingd of ovotransferrin (63 kDa), ovoinhibitor (44–49 kDa), ovoglobulin (48 kDa), ovalbumin (35 kDa), ovomucoid (28 kDa) were identified according to previous studies.[Citation33] The hemoglobin-treated EW samples had slightly intense bands of ovoinhibitor, ovotransferrin and ovalbumin, which might promote textual properties.[Citation34] The disappeared band of ovoglobulin in all EW samples (except WPC) might form soluble aggregates.[Citation35] The intense band of ovomucoid, with a molecular weight of 28 kDa and 55 kDa, identified in WPC and hemoglobin-treated liquid EW samples, could not participate in the early gel formation contributions.[Citation36] As shown in , the granule and plasma proteins were identified in current EY samples by comparison with previous studies.[8,36][8, [Citation37] The slightly intense bands of EY granule proteins (105 apo-HDL, 78 apo-HDL, 53 apo-HDL and 32 apo-HDL), ovalbumin and ovotransferrin were identified in control, WPC, SPI and BSA-treated EY samples. The increased intensity of plasma proteins (γ-livetin and 60–70 apo-LDL, 15 apo-LDL) was found especially in WPC and SPI-treated EY samples. The SDS-PAGE results showed that the addition of WPC, SPI and BSA might slightly cause higher level of covalent crosslinking in EY samples than hemoglobin-treated ones, which was in consistent with texture results.
Figure 4. SDS-PAGE profiles of (a) liquid egg white and (b) liquid egg yolk samples treated by WPC, SPI, BSA and hemoglobin. 1, control; 2, WPC; 3. SPI; 4. BSA; 5. hemoglobin; HDL, high density lipoprotein, LDL, low density lipoprotein. WPC, whey protein concentrate. SPI, soy protein isolate. BSA, bovine serum albumin.
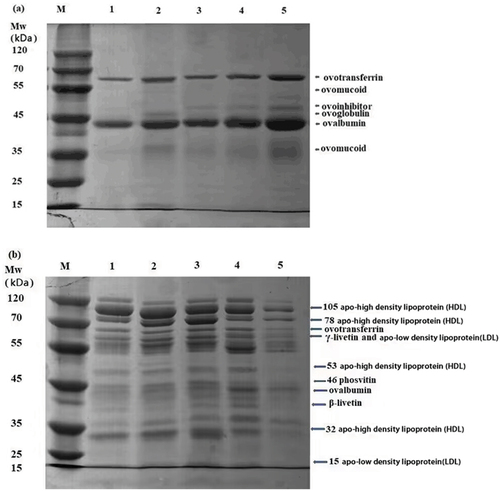
FTIR analysis
showed secondary structure changes in all EW/EY gel groups, and the curve-fitting analysis applied to amide I (1700–1610 cm−1) region.[Citation8] Compared with those of SPI group, the EW gels treated by WPC and hemoglobin exhibited relatively ordered protein secondary structure due to higher α-helix and β-sheet contents, which might be positively correlated with EW gel hardness.[Citation38] Both EW and EY gels treated by SPI powder displayed unordered secondary structure due to lower α-helix and β-sheet contents, which might originate from protein denaturation.[Citation8] Compared with BSA-treated EY gels, significant (p < .05) higher percentage of α-helix was obtained in those of WPC group. Lower percentage of α-helix and higher percentage of β-sheet indicated that BSA-treated EY gels might form more flexible and regular secondary structure due to weakness of intermolecular hydrogen bonds, protein upfolding,[Citation38,Citation39] polymerization, aggregation and protein−lipid interactions.[Citation40,Citation41] The relatively higher percentage of β-turn and irregular curl was identified in EW/EY gels treated by BSA, while lower percentage in especially WPC-treated EY gels. The SPI-treated EW/EY gels might promote conversion of α-helix and β-sheet into β-turn and irregular curl, thus stretching protein molecular chains in a more disordered state.[Citation42] Higher percentage of β-turn structure in control, SPI and hemoglobin-treated EW gels indicated slightly stronger hydrogen bonds perpendicular to the main chain of proteins, thus resulting in better gel formations.[Citation39]
Table 3. Changes in secondary structure percentage of egg white and egg yolk gels treated by WPC, SPI, BSA and hemoglobin.
SEM analysis
The microstructure of all EW/EY gels could be observed by ESEM and is depicted in . Control EW gels exhibited a relatively fine, uniform and complete structure. The WPC and BSA-treated EW gels developed uniform, porous and fiber-like structure, which was in consistent with the WHC results. A denser and tighter gel structure was formed in particularly BSA-treated EW gels. The microstructure of hemoglobin-treated EW gels displayed some granular materials as a result of the combination between EW proteins and hemoglobin. As expected, the formation of dense, relatively fine networks was responsible for higher springiness and resilience of BSA-treated EW gels. The combination/crosslinking between BSA and EW proteins might make the compact gel network resistant to moisture loss.[Citation23]
Figure 5. SEM micrographs of (a) control, (b) WPC, (c) SPI, (d) BSA and (e) hemoglobin-treated liquid egg white gels, and (A) control, (B) WPC, (C) SPI, (D) BSA and (E) hemoglobin-treated liquid egg yolk gels. 500× (scale bars, 200 μm) and 2000× (scale bars, 50 μm). WPC, whey protein concentrate. SPI, soy protein isolate. BSA, bovine serum albumin.
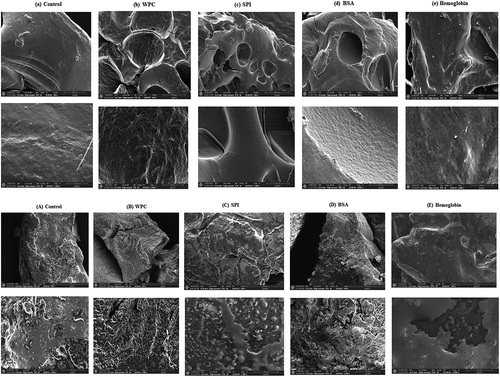
The EY gels treated by BSA showed relatively regular, stable and compact network structure due to upfolding and crosslinks in proteins. A few voids were observed on the surface of the control and SPI-treated EY gels. Rougher structure was detected in SPI and BSA-treated EY gels, while smoother structure in control and hemoglobin-treated ones. The WPC-treated EY gels had regular, loose network structure. The higher hardness or gumminess might be reflected in coarse and compact network structure, while lower values with smooth and loose network structure in gels. The liberated lipids may also maintain gel network structure through recombination, interaction and polymerization with proteins.[Citation43]
Conclusion
To sum up, various protein or protein-based powders (WPC, SPI, BSA and hemoglobin)-treated liquid egg white and yolk samples with the addition concentration of 0.5% (w/w) exhibited slightly various texture properties of gels. The present study highlights the potential application of BSA addition on the improved texture properties of egg white and yolk gels, respectively, which could be beneficial for future food industry. Our results expressed that BSA-treated egg white and yolk slightly enhanced whiteness values, texture properties and network microstructure in gels. Also, higher values of free −SH groups and H0 were detected in liquid egg white solutions treated by BSA/hemoglobin than those of the control. The higher values of a⁎, b* and protein solubility, slightly intense band of ovoinhibitor could be expected in hemoglobin-treated liquid egg white samples. The addition of SPI/BSA led to higher free –SH groups, protein solubility and covalent crosslinking level in egg yolk samples. The egg yolk gels treated by SPI developed a more disordered state of protein secondary structure and rougher microstructure. Future research might optimize and concentrate on the enhancement of gel taste and flavor of the mixture between egg white/yolk and other proteins to broaden the possibilities of these productions.
Author contribution
Yan Dai: Conceptualization (Equal), Data curation (Equal), Formal analysis (Equal), Investigation (Equal), Writing-original draft (Lead). Guo-xing Cai: Conceptualization (Supporting), Data curation (Equal), Funding acquisition (Equal), Investigation (Equal), Project administration (Equal), Writing-review & editing (Equal). Yu Liang: Conceptualization (Equal), Writing-review & editing (Equal). Zi-ying He: Writing-review & editing (Supporting). Ying Zhou: Project administration (Equal). Chun-hong Li: Resources (Supporting), Supervision (Equal); Software (equal). Haijun Chang: Conceptualization (Equal), Investigation (Equal), Supervision (Equal).
Disclosure statement
No potential conflict of interest was reported by the author(s).
Additional information
Funding
References
- Li, Z.; Huang, X.; Tang, Q.; Ma, M.; Jin, Y.; Sheng, L. Functional Properties and Extraction Techniques of Chicken Egg White Proteins. Foods. 2022, 11(16), 2434. DOI: 10.3390/foods11162434.
- Anton, M. Egg Yolk: Structures, Functionalities and Processes. Journal of Science Food Agriculture 2013, 93(12), 2871–2880. DOI: 10.1002/jsfa.6247.
- Lv, X.; Huang, X.; Ma, B.; Chen, Y.; Batool, Z.; Fu, X. Modification Methods and Applications of Egg Protein Gel Properties: A Review. Comprehensive Reviews in Food Science and Food Safety. 2022, 21(3), 2233–2252. DOI: 10.1111/1541-4337.12907.
- Liu, Y.; Wang, K.; Ma, J.; Wang, Z.; Zhu, Q.; Jin, Y. Effect of Yolk Spheres as a Key Histological Structure on the Morphology, Character, and Oral Sensation of Boiled Egg Yolk Gel. Food Chemistry. 2023, 424, 136380. DOI: 10.1016/j.foodchem.2023.136380.
- Noguerol, A. T.; Igual, M. M.; Pagan, M. J. Developing Psyllium Fibre Gel-Based Foods: Physicochemical, Nutritional, Optical and Mechanical Properties. Food Hydrocolloids. 2022, 122, 107108. DOI: 10.1016/j.foodhyd.2021.107108.
- Zang, J.; Zhang, Y.; Pan, X.; Peng, D.; Tu, Y.; Chen, J.; Zhang, Q.; Tang, D.; Yin, Z. Advances in the Formation Mechanism, Influencing Factors and Applications of Egg White Gels: A Review. Trends Food Science and Technology 2023, 138, 417–432. DOI: 10.1016/j.tifs.2023.06.025.
- Xue, H.; Liu, H.; Wu, N.; Zhang, G.; Tu, Y.; Zhao, Y. Improving the Gel Properties of Duck Egg White by Synergetic Phosphorylation/Ultrasound: Gel Properties, Crystalline Structures, and Protein Structure. Ultrason Sonochem. 2022, 89, 106149. DOI: 10.1016/j.ultsonch.2022.106149.
- Li, J.; Li, X.; Wang, C.; Zhang, M.; Xu, Y.; Zhou, B.; Su, Y.; Yang, Y. Characteristics of Gelling and Water Holding Properties of Hen Egg White/Yolk Gel with NaCl Addition. Food Hydrocolloids. 2018, 77, 887–893. DOI: 10.1016/j.foodhyd.2017.11.034.
- Liu, J.; Chai, J.; Yuan, Y.; Zhang, T.; Saini, R. K.; Yang, M.; Shang, X. Dextran Sulfate Facilitates Egg White Protein to Form Transparent Hydrogel at Neutral pH: Structural, Functional, and Degradation Properties. Food Hydrocolloids 2022, 122, 107094. DOI: 10.1016/j.foodhyd.2021.107094.
- Patil, S.; Rao, B.; Matondkar, M.; Bhushette, P.; Sonawane, S. K. A Review on Understanding of Egg Yolk As Functional Ingredients. Journal of Microbiology Biotechnology and Food Science. 2022, 11(4), e4627–e4627. DOI: 10.55251/jmbfs.4627.
- Alrosan, M.; Tan, T. C.; Easa, A. M.; Gammoh, S.; Alu’datt, M. H. Molecular Forces Governing Protein-Protein Interaction: Structure-Function Relationship of Complexes Protein in the Food Industry. Critical Review Food Science and Nutrition. 2022, 62(15), 4036–4052. DOI: 10.1080/10408398.2021.1871589.
- Nicolai, T. Gelation of Food Protein-Protein Mixtures. Advanced Colloid Interface Science. 2019, 270, 147–164. DOI: 10.1016/j.cis.2019.06.006.
- Csurka, T.; Varga-Tóth, A.; Kühn, D.; Hitka, G.; Badak-Kerti, K.; Alpár, B.; Surányi, J.; Friedrich, L. F.; Pásztor-Huszár, K. Comparison of Techno-Functional and Sensory Properties of Sponge Cakes Made with Egg Powder and Different Quality of Powdered Blood Products for Substituting Egg Allergen and Developing Functional Food. Front Nutrition 2022, 9, 979594. DOI: 10.3389/fnut.2022.979594.
- Lynch, S. A.; Mullen, A. M.; O’Neill, E. E.; García, C. Á. Harnessing the Potential of Blood Proteins as Functional Ingredients: A Review of the State of the Art in Blood Processing. Comprehensive Review Food Science Food. 2017, 16(2), 330–344. DOI: 10.1111/1541-4337.12254.
- Dai, Y.; Xia, Z. Q.; Huang, Z.; Cheng, X.; Zhang, J.; Chang, H. J. Effect of Refrigeration Storage on Functional Properties and Structure of Egg White After Defrosting Treatments. International Journal of Food Science and Technology. 2022, 57(11), 7175–7183. DOI: 10.1111/ijfs.16060.
- Wang, R.; Hartel, R. W. Citric Acid and Heating on Gelatin Hydrolysis and Gelation in Confectionery Gels. Food Hydrocolloids. 2022, 129, 107642. DOI: 10.1016/j.foodhyd.2022.107642.
- Uysal, R. S.; Boyaci, I. H. Authentication of Liquid Egg Composition Using ATR‐FTIR and NIR Spectroscopy in Combination with PCA. Journal of Science and Food Agriculture 2020, 100(2), 855–862. DOI: 10.1002/jsfa.10097.
- Ngamlerst, C.; Vatthanakul, S.; Leelawat, B.; Supawong, S.; Prinyawiwatkul, W. The Impact of Inulin Addition and High‐Pressure Processing on Physical Characteristics of Strawberry‐Flavoured Egg White Pudding. Int J. Food Science and Technology. 2023, 58(3), 1230–1240. DOI: 10.1111/ijfs.16272.
- Ullah, I.; Hu, Y.; You, J.; Yin, T.; Xiong, S.; Din, Z. U.; Huang, Q.; Liu, R. Influence of Okara Dietary Fiber with Varying Particle Sizes on Gelling Properties, Water State and Microstructure of Tofu Gel. Food Hydrocolloids 2019, 89, 512–522. DOI: 10.1016/j.foodhyd.2018.11.006.
- Ma, Z.; Ma, Y.; Wang, R.; Chi, Y. Influence of Antigelation Agents on Frozen Egg Yolk Gelation. Journal Food Engineering. 2021, 302, 110585. DOI: 10.1016/j.jfoodeng.2021.110585.
- Yu, L.; Xiong, C.; Li, J.; Luo, W.; Xue, H.; Li, R.; Tu, Y.; Zhao, Y. Ethanol Induced the Gelation Behavior of Duck Egg Whites. Food Hydrocolloids 2020, 105, 105765. DOI: 10.1016/j.foodhyd.2020.105765.
- Wee, M. S. M.; Goh, A. T.; Stieger, M.; Forde, C. G. Correlation of Instrumental Texture Properties from Textural Profile Analysis (TPA) with Eating Behaviours and Macronutrient Composition for a Wide Range of Solid Foods. Food Function 2018, 9(10), 5301–5312. DOI: 10.1039/C8FO00791H.
- Yu, X.; Wang, Y.; Xie, Y.; Wei, S.; Ding, H.; Yu, C.; Dong, X. Gelation Properties and Protein Conformation of Grass Carp Fish Ball As Influenced by Egg White Protein. Journal of Texture Studies 2022, 53(2), 277–286. DOI: 10.1111/jtxs.12668.
- Liu, J.; Zhu, K.; Ye, T.; Wan, S.; Wang, Y.; Wang, D.; Li, B.; Wang, C. Influence of Konjac Glucomannan on Gelling Properties and Water State in Egg White Protein Gel. Food Research International 2013, 51(2), 437–443. DOI: 10.1016/j.foodres.2013.01.002.
- Pu, X.; Zhang, M.; Lin, X.; Tuo, Y.; Murad, M. S.; Mu, G.; Jiang, S. Development and Characterization of Acid-Induced Whey Protein Concentrate and Egg White Protein Composite Gel. Lebensmittel Wissenschaft Technologie 2022, 164, 113624. DOI: 10.1016/j.lwt.2022.113624.
- Wang, R.; Ma, Y.; Ma, Z.; Du, Q.; Zhao, Y.; Chi, Y. Changes in Gelation, Aggregation and Intermolecular Forces in Frozen-Thawed Egg Yolks During Freezing. Food Hydrocolloids. 2020, 108, 105947. DOI: 10.1016/j.foodhyd.2020.105947.
- Ai, M.; Xiao, N.; Jiang, A. Molecular Structural Modification of Duck Egg White Protein Conjugates with Monosaccharides for Improving Emulsifying Capacity. Food Hydrocolloids. 2021, 111, 106271. DOI: 10.1016/j.foodhyd.2020.106271.
- Xue, H.; Xu, M.; Zhang, G.; Wang, P.; Yu, L.; Zhao, Y.; Tu, Y.; Zhao, Y. Study on the Mechanism of Enhanced Gel Strength of Heat-Induced Egg White by Shikimic Acid Braising. Poultry Science 2022, 101(5), 101774. DOI: 10.1016/j.psj.2022.101774.
- Primacella, M.; Fei, T.; Acevedo, N.; Wang, T. Effect of Food Additives on Egg Yolk Gelation Induced by Freezing. Food Chemistry. 2018, 263, 142–150. DOI: 10.1016/j.foodchem.2018.04.071.
- Zhang, R.; Yao, F.; Ning, Z. Characterization of Four Thermogelled Egg Yolk Varieties Based on Moisture and Protein Content. Poultry Science. 2023, 102(4), 102499. DOI: 10.1016/j.psj.2023.102499.
- Kuang, J.; Hamon, P.; Lechevalier, V.; Saurel, R. Thermal Behavior of Pea and Egg White Protein Mixtures. Foods. 2023, 12(13), 2528. DOI: 10.3390/foods12132528.
- Ren, L.; Liu, J.; Zhang, X.; Zhao, S.; Lv, Y.; Guo, H. Emulsion, Gelation, Physicochemical Properties and Microstructure of Phosphorylated and Succinylated Egg Yolk. Lebensmittel Wissenschaft Technologie. 2020, 131, 109675. DOI: 10.1016/j.lwt.2020.109675.
- Han, T.; Xue, H.; Hu, X.; Li, R.; Liu, H.; Tu, Y.; Zhao, Y. Combined Effects of NaOH, NaCl, and Heat on the Gel Characteristics of Duck Egg White. Lebensmittel Wissenschaft Technologie 2022, 159, 113178. DOI: 10.1016/j.lwt.2022.113178.
- Hunt, A.; Park, J. W.; Handa, A. Effect of Various Types of Egg White on Characteristics and Gelation of Fish Myofibrillar Proteins. Journal of Food Science. 2009, 74(9), C683–C692. DOI: 10.1111/j.1750-3841.2009.01354.x.
- Koyama, S.; Kodama, D.; Tsujii, Y.; Handa, A. Soluble-Protein-Aggregate-Assisted Improvements in Heat-Induced Gel Properties: Effect of Genipin-Mediated Crosslinks on Egg White Protein. Lebensmittel Wissenschaft Technologie. 2023, 184, 115079. DOI: 10.1016/j.lwt.2023.115079.
- Wang, J.; Liu, X.; Li, S.; Ye, H.; Luo, W.; Huang, Q.; Geng, F. Ovomucin May Be the Key Protein Involved in the Early Formation of Egg-White Thermal Gel. Food Chemistry 2022, 366, 130596. DOI: 10.1016/j.foodchem.2021.130596.
- Le Denmat, M.; Anton, M.; Beaumal, V. Characterisation of Emulsion Properties and of Interface Composition in O/W Emulsions Prepared with Hen Egg Yolk, Plasma and Granules. Food Hydrocolloids. 2000, 14(6), 539–549. DOI: 10.1016/S0268-005X(00)00034-5.
- Liu, L.; Shi, S.; Zhang, Y.; Li, Y.; Guo, J.; Zhang, M. Effect of Microwave Freeze Drying on Moisture Migration and Gel Characteristics of Egg White Protein. Food Processing Preservation 2022, 46(9), e16899. DOI: 10.1111/jfpp.16899.
- Guo, W.; Zhao, Y.; Yao, Y.; Wu, N.; Xu, M.; Du, H.; Tu, Y. Relationship Between Protein Structure Changes and in vitro Digestion of Preserved Egg White During Pickling. International Journal of Biological Macromolecular 2019, 138, 116–124. DOI: 10.1016/j.ijbiomac.2019.07.057.
- Xiang, X. L.; Liu, Y. Y.; Liu, Y.; Wang, X. Y.; Jin, Y. G. Changes in Structure and Flavor of Egg Yolk Gel Induced by Lipid Migration Under Heating. Food Hydrocolloids 2020, 98, 105257. DOI: 10.1016/j.foodhyd.2019.105257.
- Li, L.; Ren, S.; Yang, H.; Liu, J.; Zhang, J. Study of the Molecular Structure of Proteins in Eggs Under Different Storage Conditions. Journal of Food Process Preservation 2023, 2023, 1–7. DOI: 10.1155/2023/4754074.
- Zhang, J.; Liu, L.; Jiang, Y.; Shah, F.; Xu, Y.; Wang, Q. High-Moisture Extrusion of Peanut Protein-/carrageenan/sodium Alginate/Wheat Starch Mixtures: Effect of Different Exogenous Polysaccharides on the Process Forming a Fibrous Structure. Food Hydrocolloids. 2020, 99, 105311. DOI: 10.1016/j.foodhyd.2019.105311.
- Yang, Y.; Zhao, Y.; Xu, M.; Wu, N.; Yao, Y.; Du, H.; Liu, H.; Tu, Y. Changes in Physico-Chemical Properties, Microstructure and Intermolecular Force of Preserved Egg Yolk Gels During Pickling. Food Hydrocolloids 2019, 89, 131–142. DOI: 10.1016/j.foodhyd.2018.10.016.