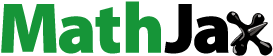
ABSTRACT
This work intended to study differences in oil yield and co-extraction of antioxidant and antibacterial compounds of Nigella sativa L. seed oil as a function of two main extraction processes: mechanical pressing and solvent extraction, including hexane and chloroform. Next, we studied such oils’ chemical composition and antioxidant and antibacterial activities. Notably, the chemical cartelization was conducted by use of HPLC. The antioxidant activity of the oils was assessed using DPPH, TAC, and FRAP assays. Antimicrobial activity was investigated against bacteria and fungi with clinical importanceT. HPLC-DAD analysis identified some bioactive compounds in the oils, including thymoquinone, carvacrol, and gallic acid. Results showed that oils extracted by different methods exhibit varying levels of antioxidant activity, with a higher concentration of antioxidant compounds in chloroform-extracted oils. Antimicrobial tests revealed significant inhibitory effects of the studied oils on the growth of bacteria and fungi. In conclusion, this research has made it possible to characterize the oils extracted from Nigella sativa L. Seeds by determining their chemical composition and biological properties, notably their antioxidant, antibacterial, and antifungal activity. These results contribute to a better understanding of the potential benefits of these oils for human health and open up exciting prospects for their use in various fields, notably as food additives and therapeutic agents.
Introduction
Aromatic and medicinal plants have always been of significant economic interest in various industries such as pharmaceuticals, food, cosmetics, and health. In addition to their aromatic and culinary qualities, they contain a variety of active principles, such as alkaloids, flavonoids, carotenoids, tannins, saponosides, and oils, which also confer medicinal virtues.[Citation1–3] These plants represent an invaluable reservoir of effective traditional remedies and remain the most widely exploited natural source of medicines in the contemporary world.[Citation4,Citation5]
Since ancient times, man has used plants to treat various ailments,[Citation6] although the beneficial effects of these treatments were often overlooked.[Citation7] Today, as the efficacy of conventional medicines shows signs of diminishing over time, herbal therapies will make a strong comeback.[Citation8] These are leading scientific researchers to undertake in-depth studies into the chemical composition of plants, particularly their secondary metabolites, and to explore their potential therapeutic actions.[Citation9,Citation10] Despite this, the potential of plants as sources of new medicines remains under-exploited mainly, with only 22% of plant species studied for their biological activity and 15% assessed for their phytochemical activity.[Citation11]
Due to its privileged biogeographical position, Morocco is home to a rich ecological and floristic diversity, encompassing more than 4,000 species belonging to different botanical families. Of these, 22% are endemic to the region and remain primarily unexplored from a phytochemical and pharmacological point of view.[Citation12,Citation13]
Oxidative stress is one of the most widespread problems in the biological and medical fields.[Citation14] It occurs when cells are exposed to excessive production of toxic free radicals, leading to potentially serious diseases such as cancer.[Citation9,Citation15] Although reactive oxygen species (ROS) are an integral part of aerobic metabolism, their overproduction can harm the organism.[Citation16,Citation17] In this context, plant extracts’ antimicrobial properties are crucial. Several studies have suggested that certain bioactive compounds in these extracts may have significant antimicrobial activity.
This study was focused on black caraway (Nigella sativa L.), an annual plant in the Ranunculaceae family. Native to the Mediterranean basin and the Middle East, black caraway is prized for its unusual-tasting seeds and their many health benefits.[Citation18–20] Black caraway seeds have long been used in roasted, ground, vegetable, and essential oils for thousands of years, notably in medicinal preparations.[Citation10,Citation21]
Oil extracted from black seeds, commonly known as nigella or black cumin, has been recognized for centuries for its medicinal properties. Rich in bioactive phytoconstituents such as flavonoids, thymoquinones, and essential fatty acids, this oil has been used in traditional medicines of the Middle East, Asia, and Africa for its curative virtues. This study aimed to extract, identify, and characterize bioactive molecules present in black caraway. In addition, we will evaluate different extraction methods for bioactive compounds and compare the optimization of extraction parameters for fixed oils. This approach will enable us to explore the biological activities of black caraway seed extracts obtained by various methods, aiming to identify the optimum way in terms of yield and reliability.
Plant material and experimental methods
Plant material
The plant material consists of Nigella sativa L. seeds collected in Morocco’s Souk El Arbaa area (34°3957 N 5°5854 W). Each batch of seeds was ground using an electric grinder. The powder obtained is stored in hermetically sealed bottles, protected from light, until use.
Chemical reagents
Butylated hydroxytoluene (BHT), ammonium molybdate, 2,2-diphenyl-1-picrylhydrazyl (DPPH), ascorbic acid, sodium phosphate, iron III chloride (FeCl3), sodium thiosulfate, potassium ferricyanide (K3Fe(CN)6), acetone, hexane, cyclohexane and chloroform as 99% pure were purchased from Sigma-Aldrich (Munich, Germany).
Extraction of fixed oils from N. Sativa seeds
Pressure extraction only on using FoundGo Automatic oil press Hot/cold oil extraction machine Stainless steel oil press extractor 750 W Oil extractor for seeds, peanuts, 4–7 kg/h. This process produces a very pure oil containing no foreign substances. This type of extraction uses apolar organic solvents hexane (OEHx), and chloroform (OECh). This is the most commonly used method for extracting vegetable oils, as it recovers more oil than the mechanical pressing method (OEPr). The extracted oils are stored in small, dark glass bottles, hermetically sealed to prevent exposure to air and light. They are also kept at a temperature of around 4°C.
HPLC-DAD chromatograms of the three fixed oils from N. sativa seeds
The crude extract is prepared according to the slightly modified method of Gilani et al. (2001), where 5 g of crude extract is macerated in 50 ml boiling distilled water for 72 hours. The crude extract is mixed with ethyl acetate (V/V). After settling, the upper organic phase is recovered. This step is repeated several times, renewing the solvent until it becomes transparent. The ethyl acetate is then evaporated and the extract obtained is freeze-dried and stored at −20°C until used for HPLC analysis.
The oils studied were analyzed using reverse-phase high-performance liquid chromatography with a diode array detector (HPLC-DAD) “Perkin Elmer FLEXAR HPLC System with Diode Array Detector.” The analyses were conducted using a Thermo Scientific HPLC system with a 250 × 4.6 mm MOS-1 HYPERSIL analytical column and a 5 µm Exsil ODS stationary phase. The separation process was carried out in gradient mode using two solvents: A (water) and C (acetonitrile), with an elution gradient of 80% A, 20% C for 1 minute; 60% A, 40% C for 2.5 minutes; and 80% A, 20% C for 4 minutes. The flow rate was maintained at 1 mL/min, and an injection volume of 5 µL was employed. Each compound was identified based on comparing retention times and UV spectra with authentic standards.[Citation22]
Tegument content in fixed oils from N. Sativa seeds
Flavonoid content
Flavonoids in the fixed oils were quantified using the aluminum trichloride (AlCl3) method.[Citation23] For this, 2 mL of 20 g/L AlCl3 solution was added to 2 mL of the sample or standard (prepared in methanol). The mixture was left to incubate for 15 minutes in darkness, and the blank was obtained by replacing the oils with methanol. The absorbance was calculated at 425 nm, and the results were expressed as milligrams of quercetin per gram of seed extract (mg QE/g).
Total carotenoids
The Mackinney method[Citation23] was utilized to determine total carotenoids. 50 mg fresh plant material samples were ground in 10 mL of 80% (v/v) aqueous acetone. The resulting mixture was then filtered and centrifuged, and the extract was analyzed for absorbance readings at three wavelengths: 470, 663 and 647 nm. The concentration of the chemical compound was calculated by applying the following equation:
The Carotenoid concentration was expressed in milligrams per gram of seed oils (mg/g).
Chemical parameters of fixed oils from N. Sativa seeds
Iodine value
The iodine value was determined by introducing the solubilized oil into chloroform. After adding potassium iodide solution, the mixture was titrated with 0.1 M sodium thiosulfate solution until the coloration disappeared. While the titration continued, 5 mL of the starch solution was added until the coloration disappeared completely. The iodine value (I2) is calculated using the following formula[Citation24]:
Where n2 is the number of mL of 0.01 M sodium thiosulfate in the blank test, n1 is the number of mL of 0.1 M sodium thiosulfate in the test sample, and m: Oil mass.
Acidity value
The acidity value is a number expressed in mg of Potassium hydroxide (KOH) required to neutralize the free fatty acids in 1 g of oil. To determine the acidity value, 2 g of N. sativa seed oil is solubilized in ethanol neutralized with KOH, 0.1 M, in the presence of phenolphthalein solution. The acidity value (Av) is determined as follows[Citation24]:
Peroxide value
The peroxide value measures the freshness of fatty substances such as oils, fats, or resins. It represents the amount of active oxygen on carbon chains; the more oxidized the fat, the higher the peroxide value. The peroxide value (POV) indicates the quantity of peroxide present in the sample, measured in milliequivalents of active oxygen per kilogram of the product (mEq O2/kg). To determine the peroxide value, 1 g of oil is weighed into a flask to the nearest 0.001 and mixed with 10 mL of chloroform. After that, 15 mL of acetic acid and 1 mL of potassium iodide are added, and the mixture is stirred for 1 minute and left to stand for 5 minutes in the dark at room temperature. Next, 75 mL of distilled water is added, followed by titration of the liberated iodine with 0.01N sodium thiosulfate (Na2S2O3) solution, using starch solution (1 g/100 mL) as an indicator, until the color disappears. A blank test is carried out simultaneously. The peroxide value, expressed in milliequivalents of O2/kg, is calculated according to the following formula[Citation25]:
V is volume of sodium thiosulfate in the sample in mL, V0 is volume required to titrate the blank in mL, m is weight of test sample in g, T is normality of sodium thiosulfate.
Specific extinction (K value)
All natural fats contain linoleic acid in varying amounts. The oxidation of fat leads to the formation of conjugated dienes and trienes. European regulations measure the amount of the latter in oil. The detection of oxidation products of unsaturated fatty acids with a conjugated diene structure (e.g. C18:2 linoleic acid hydroperoxide) and that of oxidation by-products with a triene structure (in the case of fatty acids with three double bonds) is made possible by the determination of absorbance at around 232 nm and 270 nm. For this analysis, 0.25 grams of oil is dissolved in 25 mL of cyclohexane. The absorbance is measured in a quartz cuvette against the solvent on a UV/Visible spectrophotometer. The values are expressed as specific extinction Ecm1% conventionally noted by K and expressed as follows:[Citation26]
Aʎ: absorbance at wavelength ʎ, C: solution concentration in mg/100 mL, S: optical path (1 cm).
Antioxidant activity of the oils studied
DPPH test
To analyze each sample, 2.5 mL of different concentrations are added to 2.5 mL of a methanolic solution of DPPH (2,2-diphenyl-1-picrylhydrazyl) at a concentration of 100 µM. The reaction mixture is stirred immediately and kept at room temperature in the dark for 30 minutes. The reaction mixture containing 2.5 mL of DPPH and 2.5 mL of methanol served as the control. The same test is performed by using ascorbic acid as a reference antioxidant. By measuring the absorbance of DPPH for different antioxidant compounds (extracts and ascorbic acid), it is possible to determine the percentage of inhibition (PI) using the following formula.[Citation27,Citation28] The IC50 represents the substance concentration required to reduce free radicals by 50% in the reaction medium.
Iron reduction test (FRAP)
Iron reduction relies on antioxidants to reduce ferric iron to iron salt, forming a blue solution. Briefly, 200 µL of different concentrations of oils and 500 µL of 0.2 M phosphate buffer (pH 6.6) were added to glass tubes, followed by 500 µL of 1% potassium hexacyanoferrate K3Fe(CN)₆ in distilled water. The mixture was heated to 50°C in a water bath for 20 minutes. A 500 µL volume of trichloroacetic acid (10%) was pipette out and centrifuged at 3000 rpm for 10 minutes. A 500 µL volume of the supernatant was transferred to another tube, adding 500 µL of double distilled water (ddH2O) and 100 µL of 1% FeCl3, freshly prepared in ddH2O.
Similarly, a blank without a sample was also prepared by replacing oils with methanol. The absorbance was detected at 594 nm against the blank, replacing the oils with methanol, allowing calibration of the instrument (UV-VIS spectrophotometer). A solution of standard antioxidants, either BHT (Butylated hydroxytoluene) or quercetin, whose absorbance were read the same way as the samples, served as positive controls. Antioxidant activity linked to the reducing power of extracts is expressed as Reducing Power (RP) using the following formula:
The reducing power of iron is expressed by the EC50, which corresponds to the sample concentration, giving an absorbance of 0.5.
Total antioxidant capacity (TAC)
The oils’ total antioxidant capacity (TAC) was measured using the phosphomolybdenum method. Briefly, 100 µL of different concentrations of oils were added to 1000 µL of reagent mixture of H2SO4, Na2PO4, and ammonium molybdate, with concentrations of 0.6 M, 28 mM, and 4 mM, respectively. The tubes were heated at around 95°C for 90 minutes. After cooling, absorbance was noted at 695 nm. The control consisted of 100 µL of methanol mixed with 1000 µL of the reagent mixture. The samples and controls were incubated under identical conditions. The results are expressed in milligrams of ascorbic acid equivalents per gram (mg EAA/g).
Evaluation of antibacterial activity
Bacterial strains
The bacterial strains used in this study included four: Staphylococcus aureus ATCC 6633 (a pathogenic bacterium commonly associated with various infections in humans), Escherichia coli K12 (a bacterium commonly used as a model organism in microbiology and genetics), Klebsiella pneumoniae CIP A22 (an opportunistic bacterium that can cause hospital-acquired infections, selected for its importance in studies of antibiotic resistance and pathogenicity), and Pseudomonas aeruginosa CIP 82.114 (an opportunistic bacterium implicated in various infections, particularly in immunocompromised individuals, chosen for its potential role in hospital-acquired infections and its resistance to antibiotics). All microbial strains were supplied by the Laboratoire de biotechnologie, environnement, agroalimentaire et santé, Faculté des sciences Dhar El Mahraz, Université Sidi Mohammed Ben Abdellah, Fès, Morocco.
Preparation of bacterial cultures
From fresh cultures of each bacterium in liquid Mueller-Hinton (MH) medium, decimal dilutions were prepared in sterile saline (0.9% NaCl) until a turbidity of 0.5 McFarland (106 to 108 CFU/mL) was attained.
Disc diffusion method
The antibacterial activity of N. sativa fixed oils was tested using the disk diffusion method described by Balouiri et al.. (2016).[Citation29] Petri dishes containing MH medium were seeded separately with the bacterial strains tested using the double-layer method. To prepare the inoculum, 100 µL of each bacterial culture was added to tubes containing 5 mL of soft agar (0.5% agar-agar in MH medium). The inoculated tubes were poured into Petri dishes containing MH culture medium.
Antibacterial activity test
Sterile 6 mm in diameter Whatman paper discs were placed at the center of each seeded Petri dish. These discs were then impregnated with 20 µL of the N. sativa fixed oils studied at 130 mg/mL concentration in DMSO solvent (5% V/V). Following the same procedure, the antibiotic cephalothin (30 µg/disc) was included as a positive control. Notably, the petri dishes were incubated at 37°C in the dark and a humidity-saturated atmosphere for 24 hours. The diameter of the inhibition zones was measured to assess the antibacterial activity of the studied fixed oils.
Assessment of antifungal activity
Fungal strains
In this study, we used four different fungal strains such as Candida albicans ATCC10231 (an opportunistic fungus often responsible for candidiasis in humans. The strain is frequently used as a reference in antifungal studies), Aspergillus niger (is a fungus used in industrial production of certain enzymes. Its presence may be relevant in studies linked to industrial applications and fungal interactions), Aspergillus flavus (produces mycotoxins and is often associated with the contamination of food crops, particularly cereals), and Fusarium proliferatum (is a fungus that can cause plant diseases and produce mycotoxins. Its choice is motivated by agricultural and food safety considerations). All microbial strains were supplied by the Laboratoire de biotechnologie, environnement, agroalimentaire et santé, Faculté des sciences Dhar El Mahraz, Université Sidi Mohammed Ben Abdellah, Fès, Morocco.
Disk diffusion method
The test to assess the antifungal activity of the fixed oils studied was carried out using the disk diffusion method described by Balouiri et al..[Citation29] For Candida albicans, the same principles and protocols as those used for antibacterial activity were followed. For filamentous fungi (Aspergillus niger, Aspergillus flavus, and Fusarium proliferatum), antifungal activity was evaluated on Extract of Malt agar medium. This evaluation involved a confrontation between each harmful fungus and 20 µL of the fixed oils studied at 130 mg/mL concentration in DMSO solvent (5% V/V). The antifungal Fluconazole (15 mg/mL) included positive controls following the same procedure. Noteworthy, the petri dishes were incubated under specific conditions. For Candida albicans, incubation was carried out at 37°C in the dark and a humidity-saturated atmosphere for 48 hours. For filamentous fungi (Aspergillus niger, Aspergillus flavus, and Fusarium proliferatum), incubation was carried out at 30°C under the same conditions for 7 days. Inhibition diameter and inhibition rate were determined according to the methods described by.[Citation30–32]
Statistical analysis
Data was presented as means with standard deviation (SD) calculated from three replicates. GraphPad Prism (version 8.0.1) was used to perform statistical analyses. In addition, The Shapiro-Wilk test was employed to check whether variables followed a normal distribution, as Levene’s test was utilized to assess the homogeneity of variances. Statistical differences between means were determined using analysis of variance (one-way ANOVA), followed by Tukey’s test for multiple comparisons. The significance thresholds were set at a probability level of p ≤ .05.
Results
Fixed oil yield of N. sativa L seeds
The effect of the extraction process on the fixed oil yield of Nigella sativa L seeds is shown in . The fixed oil yields obtained differed non-significantly from the different extraction methods. The chloroform extraction process (OECh) gave the highest fixed oil yield, with a value of (27.850 ± 0.212%) (). The hexane extraction process (OEHx) also showed a high yield of fixed oils, reaching (26.580 ± 0.495%). In comparison, the yield obtained by mechanical pressure (OEPr) was of the order of (25.300 ± 0.424%) ().
These results highlight the significant influence of the choice of extraction solvent on the fixed oil yield of N. sativa seeds. The extraction methods using chloroform and hexane proved more efficient than mechanical pressure extraction.
Organoleptic analysis: color, odor and texture
Vegetable oils extracted from black cumin seeds are generally yellow to brown in color. They have a distinct, fairly strong odor, often described as spicy and aromatic. This characteristic odor is one of the signatures of black cumin oil. In terms of texture, nigella oils are typically oily and fluid. They have a light consistency that makes them easy to apply and absorb by the skin. This fluidity is often appreciated when using black cumin oil in cosmetics or aromatherapy, as it enables gentle application and rapid absorption.
HPLC-DAD of the three fixed oils studied
Four major peaks were identified in the OECh extract obtained by chloroform maceration at retention times of 2.842, 10.686, 17.916 and 19.758 minutes, respectively (). Similarly, the fixed oil (OEPr), obtained by mechanical pressing, showed peaks at retention times of 2.865, 10.619, 17.872, and 19.746 minutes. The “OEHx” extract, resulting from hexane maceration, displayed three major compounds similar to those detected by other extraction methods, located at retention times of 2.866, 18.019, and 19.838 minutes. Interestingly, this OEHx also showed minor products in its nonpolar fraction.
provides a comparison of the three extracts (overlay of their three identical chromatograms presented in the previous figure) based on the expression of detected molecules, including the overlay of chromatograms related to the detected standards, namely thymoquinone, carvacrol, and gallic acid.
Figure 3. Superposition of peaks relating to the standards detected, thymoquinone carvacrol, and gallic acid on the chromatograms of the extracts studied OEHx, OTCh and OEPr, Thermo Scientific HPLC system – Chromera Software.
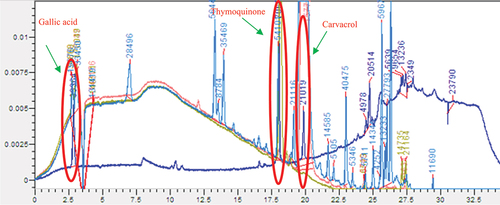
Further insight was gained through standards screening, confirming that the 17.872-minute compound in all three extracts was thymoquinone, a bioactive component with potential health benefits. In addition, the compound at 19.758 minutes was identified as carvacrol, a well-known aromatic compound, and the compound at 2.842 minutes was determined to be Gallic Acid, thus contributing to the complex composition of the extracts.
Comparison between the peaks identified in the three extracts suggests that the most significant molecules are shared between the different extraction methods. However, some may be specific to a particular method. For example, the peak at 17.916 minutes in OECh displays a significant area, while OEPr shows a higher concentration of the same compound at 17.872 minutes. These variations could be due to the inherent efficiency of each extraction method in transferring compounds.
Quantitative determination of flavonoids and carotenoids
Taking into account the selectivity of each extraction method, the results of quantitative flavonoid determination are shown in . Significant differences in flavonoid content were observed between extraction methods. The chloroform extraction process revealed the highest flavonoid content, with a concentration of around 13.49 ± 0.46 mgEQ/g (): hexane and mechanical pressure extraction methods produced flavonoid contents of 10.50 ± 0.5 mgEQ/g and 8.35 ± 0.74 mgEQ/g, respectively.
Figure 4. Flavonoid and carotenoid contents in seed oils. Means (± SD, n = 3) bearing the same letter indicate no significant difference according to Tukey tests, p ≤ .05.
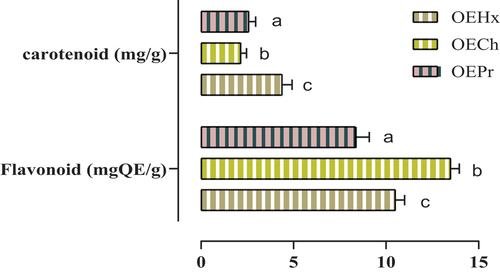
Regarding carotenoid content, OEHx showed the highest carotenoid content, with a concentration of around 4.38 ± 0.53 mg/g. OEPr oil revealed a carotenoid content of 2.58 ± 0.36 mg/g, while OECh showed a lower range of 2.14 ± 0.31 mg/g (). These results highlight the distinct selectivity of each extraction mode and its influence on flavonoid and carotenoid composition in the fixed oils tested.
Physicochemical parameters of N. sativa fixed oils
The results of the iodine index analyses are shown in . A significant difference was observed between the analyzed seed oil’s iodine indices. The oil extracted with chloroform (OECh) had an acidity of 98.28 ± 2.18 while those obtained by hexane (OEHx) and pressing (OEPr) show acidity indices of 48.000 ± 1.00 and 42.70 ± 4.10, respectively. The results of the acid number analyses are shown in . The seed oils analyzed offer a significant difference in acid value. The OEHx has a higher acid number (1.026 ± 0.064 mg KOH/g) than OECh (0.968 ± 0.009 mg KOH/g). The lowest iodine value was found in OEPr oil, with a value of 0.061 ± 0.019 mg KOH/g. These results highlight significant differences in the acidity and iodine characteristics of fixed oils obtained by different extraction methods. Analyses of the peroxide value of fixed oils obtained from N. sativa L. seeds using different extraction methods are presented in . The peroxide value measures the amount of peroxidic species present in the oils tested, which may indicate their oxidation level. The results show subtle differences in peroxide values between oils obtained by different extraction methods. OEHx fixed oil has an average peroxide value of 1.500 ± 0.002 milliequivalent O2/kg of oil. Similarly, OECh fixed oil has a peroxide value of 1.493 ± 0.001 milliequivalent O2/kg of oil. On the other hand, OEPr oil shows a slightly higher peroxide value, with an average value of 1.495 ± 0.003 milliequivalent O2/kg of oil.
Figure 5. Physicochemical parameters of N. sativa fixed oils. Iodine value (a), Acid value (b), Peroxide value (c), and oxidation stability indices (d). Means (± SD, n = 3) bearing the same letter indicate no significant difference according to Tukey tests, p ≤ .05.
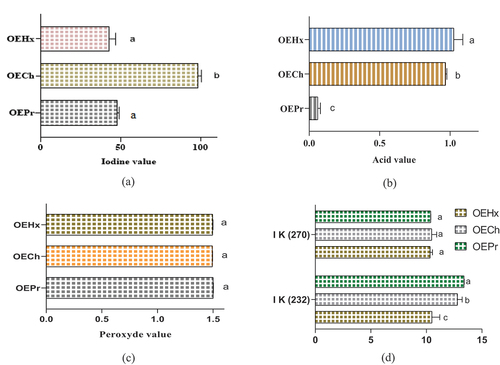
These results highlight subtle but significant variations in the oxidation levels of fixed oils obtained by different extraction methods. The differences observed in peroxide indices may reflect variations in the oxidative stability of the oils and have potential implications for their shelf life and quality. Oxidation stability indices, measured by K232 and K270, provide valuable information on the resistance of fixed oils obtained from N. sativa seeds to oxidation and deterioration. illustrates the K232 and K270 indices of the fixed oils studied. The values obtained for the different fixed oils reveal significant differences in oxidation stability. The fixed oil (OEHx) has a K232 value of 10,500 ± 0.707, while OECh has a higher K232 value of 12.8 ± 0.424. OEPr oil shows a K232 value of 13.390 ± 0.014. The K270 values provide further information on the oxidation stability of fixed oils. OEHx has a K270 value of around 10.336 ± 0.209, while OECh has a slightly higher K270 value of 10.476 ± 0/458. OEPr oil has a K270 value of 10,394 ± 0.008. These results highlight significant differences in oxidation stability between fixed oils obtained by different extraction methods. The variations observed in the K232 and K270 indices reflect the different compositions of oxidizable compounds in the oils and have important implications for their shelf life and use.
Assessment of antioxidant activity
DPPH test
The antioxidant activity of fixed oils obtained from N. sativa seeds by different extraction methods was evaluated using the DPPH test (). The analyses show significant differences in IC50 values between the different fixed oils tested and the standards used. Fixed oil OEHx has an IC50 of around 0.063 ± 0.001 mg/mL, indicating good antioxidant activity. Similarly, OECh oil shows pronounced antioxidant activity, with an IC50 of around 0.047 ± 0.001 mg/mL. In contrast, OEPr oil has a slightly higher IC50 of 0.254 ± 0.006 mg/mL.
Figure 6. Antioxidant activity of N.sativa fixed oils obtained by different extraction methods. DPPH test (A), IC50 values expressed in mg/mL (B), total antioxidant capacity (C), and EC50 of ferric reducing antioxidant power (D). Means (± SD, n = 3) bearing the same letter indicate no significant difference according to Tukey tests, p ≤ .05.
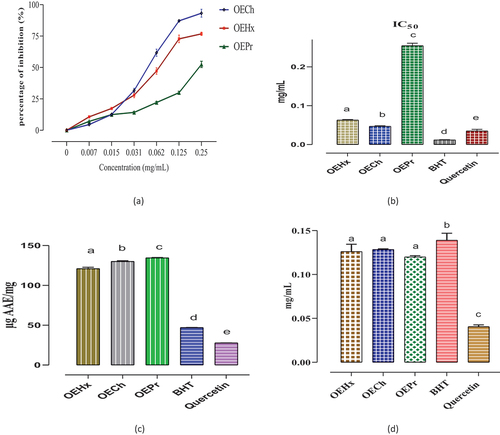
These results were compared with the antioxidant standards used, BHT and quercetin. BHT, a well-known synthetic antioxidant, has a low IC50 of around 0.0 11 ± 0.000 mg/mL, indicating high antioxidant activity. Similarly, quercetin, a flavonoid naturally present in many foods, shows an IC50 of 0.035 ± 0.004 mg/mL. The results of the DPPH test thus reveal that Nigella sativa L fixed oils obtained by different extraction methods display varied antioxidant activity, with IC50 values reflecting their ability to neutralize free radicals.
TAC test
The results of the TAC test are shown in . They reveal significant differences in total antioxidant capacity between the different fixed oils tested and the standards used. The fixed oil OEHx has an average total antioxidant capacity of 121.023 ± 1.7211 µgAAE/mg of oil. Similarly, OECh oil has a total antioxidant capacity of 130.0487 ± 0.950 µgAAE/mg. In contrast, OEPr oil has the highest total antioxidant capacity of the three extraction processes, with an average value of 134.552 ± 0.508 µgAAE/mg.
These results are compared with the antioxidant standards used, BHT and quercetin. BHT has a total antioxidant capacity of 47.026 ± 0.241 µgAAE/mg, reflecting its high antioxidant activity. Similarly, quercetin has a total antioxidant capacity of 27.759 ± 0.090 µgAAE/mg. The results of the TAC test underline the variability in the total antioxidant capacities of the fixed oils studied obtained by different extraction methods, highlighting their potential to prevent oxidation and neutralize free radicals.
FRAP test
The results of the FRAP tests are summarized in , where EC50 values are compared between the essential oils tested and the standards used. Fixed oils obtained by different extraction methods show significant differences in their antioxidant capacity, expressed in EC50.
The fixed oil OEHx has an EC50 of 0.126 ± 0.008 mg/mL, indicating a moderate antioxidant capacity to reduce oxidizing species. Similarly, the chloroform-extracted oil shows a close EC50, with a 0.128 ± 0.000 mg/mL value. In contrast, oil extracted by mechanical pressure stands out as having the lowest EC50 of the three methods, with a value of 0.120 ± 0.001 mg/mL.
Comparatively, the antioxidant standards BHT and quercetin show different EC50 values. BHT, a widely used synthetic antioxidant, displays an EC50 of 0.139 ± 0.011 mg/mL. On the other hand, quercetin, a natural flavonoid, shows the highest antioxidant activity of all the samples tested, with an EC50 of 0.040 ± 0.002 mg/mL. The results of the FRAP test highlight the variability of antioxidant capacities of the oils tested, obtained by different extraction methods, and compare them with commonly used antioxidant standards.
Antibacterial activity
lists the effectiveness of three oils, OEHx, OECh, and OEPr, in inhibiting the growth of different bacterial strains. The larger the diameter of the zone of inhibition, the more effective the substance is at stopping bacterial growth. The three fixed oils tested have similar inhibitory activities against S. aureus and K. pneumoniae. All three show comparable inhibition zone diameters for these two strains. Cephalothin is an antibiotic that also shows inhibitory activity but is more specific, acting primarily against S. aureus and P. aeruginosa. However, these oils show no significant inhibitory activity against E. coli and P. aeruginosa. On the other hand, cephalothin has a specific action against S. aureus and P. aeruginosa, with no notable effect on E. coli and K. pneumoniae. Moreover, cephalothin is more effective against S. aureus, showing larger inhibition zones than those observed with oils. However, the oils appear to have some activity against K. pneumoniae, with zone diameters of inhibition comparable to cephalothin. All the oils tested, along with cephalothin, have no significant inhibitory effect on E. coli and P. aeruginosa, these strains being less sensitive or resistant to these substances.
Table 1. Diameters of Zones of Inhibition.
Results for antibacterial potency in solid media are presented as mean values ± SD of three measurements. Results in the table with the same letter (a, b, c) do not represent a significant difference (p < .05).
OEHx, OECh and OEPr oils appear to have similar antibacterial properties, with moderate activity against S. aureus and K. pneumoniae, but no significant activity against E. coli and P. aeruginosa (). They have low MICs (0.332–0.664 µg/mL) for S. aureus and K. pneumoniae, showing moderate antibacterial activity. Cephalothin, on the other hand, as an antibiotic, has slightly higher MIC (1.5625 µg/mL) for S. aureus and P. aeruginosa, showing higher efficacy against S. aureus and P. aeruginosa, but not affecting E. coli and K. pneumoniae.
Table 2. Minimum Inhibitory Concentrations (MIC) Top of Form.
Results for antibacterial potency in liquid media are presented as mean values of three measurements. Results in the table with the same letter (a, b, c) do not represent a significant difference (p < .05).
Antifungal activity
The three fixed oils tested (OEHx, OECh, and OEPr) showed similar antifungal activity against C. albicans. The inhibition zones are comparable (19–20 mm), indicating moderate inhibition of this fungus’s growth. However, none of the three oils had any noticeable inhibitory effect against A. niger or A. flavus. All oils also showed slight inhibitory activity against F. proliferatum, with similar inhibition percentages of around 32.3%-35.17% for OEHx, 32.14%-34.12% for OECh and 32.43%-35.13% for OEPr ().
Table 3. Diameters of Zones of Inhibition (ZDI.).
Fluconazole, a medicated antifungal agent, shows significantly more vigorous antifungal activity than the oils. It exhibited larger inhibition zones (up to 41.33 mm) against C. albicans than oils. It strongly inhibited the growth of A. niger (47.67 ± 1.53%) and A. flavus (43.67 ± 1.53%), showing high efficacy against these strains. Fluconazole also showed increased activity against F. proliferatum (59.17 ± 0.76%), outperforming oils in inhibiting this strain.
Antifungal potency results in solid media are presented as mean values ± SD of three measurements. Results in the table with the same letter (a, b, c) do not represent a significant difference (p < .05). The values in represent the Minimum Inhibitory Concentrations (MIC) for the antifungal activity of different substances against different fungal strains. The MIC is the lowest concentration of a substance (in µg/mL) required to inhibit the visible growth of the fungus. The three oils tested have almost similar MIC against C. albicans, ranging from 1.319 to 1.328 µg/mL. Fluconazole had a higher MIC of 11.71 µg/mL. However, oils obtained by OEHx, OECh, and OEPr have MIC of 10.820 µg/mL, 10.425 µg/mL and 9.625 µg/mL, respectively, against F. proliferatum. Fluconazole has a MIC of 5.86 µg/mL against F. proliferatum.
Table 4. MIC for antifungal activity.
Results for antifungal potency in liquid media are presented as mean values ± SD of three measurements. Results in the table with the same letter (a, b, c) do not represent a significant difference (p < .05).
Discussion
The results obtained from the study of fixed oil extraction from N. sativa seeds have significant implications for the effect of the extraction process on oil yields and the composition of the extracts obtained. Firstly, concerning oil yields, it is interesting to note that the mechanical pressure extraction process leads to lower yield as compared to solvent extraction processes, particularly chloroform) and hexane.[Citation33] This disparity in yield is consistent with the literature, where it is generally accepted that solvent extraction tends to extract more oil from seeds than mechanical pressure extraction.[Citation34–36] It can be attributed to organic solvents’ more efficient solvent properties, which can solubilize a broader range of lipid compounds.
In addition, volatile organic solvent extraction remains the most common method. The most commonly used solvents currently are hexane, cyclohexane, and chloroform.[Citation37–40] Hexane is presently the only solvent used industrially to extract vegetable oils. It has been employed for many years for its apolar properties, which give it an excellent affinity for lipids.[Citation34,Citation41,Citation42] However, hexane is likely to be questioned because of its toxicity and flammability to the nervous system. It is a dangerous substance with several classifications: highly flammable, category 3 reprotoxic, harmful, irritant, and hazardous to the environment. New avenues are being explored, particularly in the search for substitute solvents.[Citation43–45]
However, it is essential to consider the extracted oil’s purity, particularly in mechanical pressure extraction. Unlike solvent extraction processes, mechanical extraction generates oil of very high purity, with no foreign substances present.[Citation33] This observation aligns with other studies highlighting the purity of oils obtained by mechanical pressing.[Citation33] This increased purity could have a positive impact on the use of oil in food and cosmetic applications by avoiding the presence of potentially undesirable solvent residues.
In similar studies, our results are consistent with previous research, which explored the composition of N.sativa extracts obtained by different extraction methods. For example, identifying thymoquinone in our extracts aligns with the work of Habib N. et al.. (2021).[Citation46] In addition, several studies have conducted a comparative analysis of extraction methods, including maceration and pressing, to assess the chemical composition of N. sativa extracts. Their results showed similar trends, indicating that certain compounds, such as thymoquinone, were consistently present in different extraction techniques.[Citation47,Citation48] This consistency across various studies underlines the robustness of these conclusions and reinforces understanding of the composition of N. sativa extracts.
Concerning oil seed composition, it is remarkable to note that the choice of extraction method also influences the chemical profiles of the oils obtained. Results show that acidity, iodine, and peroxide values vary according to extraction mode. This variability in the quality parameters of extracted oils can be associated with the specific conditions of each extraction method. For example, solvent extraction, using apolar organic solvents such as chloroform and hexane, can result in higher lipid oxidation compared to mechanical pressure extraction, where the oil is extracted mechanically without introducing solvents.
Regarding the flavonoid content of N. sativa fixed oil, analysis of our results shows a low flavonoid content by the mechanical pressure extraction process compared to solvent extractions. It is thus suggested that the method of oil extraction (pressing or solvent extraction) may affect the flavonoid and carotenoid content of the oils,[Citation49,Citation50] with the chloroform and hexane extracts of N. sativa seeds being the most flavonoid-rich. About the carotenoid content of our oil, our results show a considerably low range compared to the flavonoid content.
In general, the indices vary significantly according to the extraction method. However, it was found that the extraction process did not alter the quality of the oils analyzed. The acid values obtained from N. Sativa seeds are within the limit the Codex Alimentarius (1999) set, i.e., less than or equal to 4 mg KOH/kg of oil.[Citation43] In addition, extraction by mechanical pressing gives oils containing less free fatty acids than those extracted with a solvent.[Citation51,Citation52] This difference would be because the temperature reached during hexane extraction would cause the hydrolysis of one or two of the three ester bonds of the triglycerides, thus favoring the formation of free fatty acids. The extraction conditions by pressing are not severe, as they do not decrease the degree of establishment observed for hexane-extracted oil. In addition, hexane extraction would break certain double bonds of unsaturated fatty acids, thus lowering the diode value of the oils analyzed.[Citation53,Citation54]
The results of this study revealed that the fixed oil of N. sativa has a high level of carotenoids, and these impregnating flavonoid compounds could scavenge free radicals. The antioxidant activity obtained can be explained by the qualitative and quantitative nature of the compounds in the oil.[Citation49,Citation55] In addition, results on the antioxidant activity of oils obtained by different extraction processes provide significant information. Using tests such as the DPPH and FRAP assays to assess antioxidant activity has revealed significant differences between oils obtained by different extraction methods. The extract obtained by mechanical pressure showed a lower antioxidant activity than organic solvents. These results align with previous studies showing that organic solvents such as chloroform and hexane can enhance the extraction of antioxidant compounds. This is directly related to its higher content of phenolic antioxidants, particularly flavonoids, and carotenoids, and confirms the existence of a frequent correlation between antioxidant capacity and the richness of plant extracts in these natural antioxidants. Indeed, these phenolic antioxidants can reduce reactive oxygen species, including free radicals, through a biomolecule protection mechanism.[Citation56–58]
In this study, we investigated the antioxidant properties of N. sativa fixed oil, based on experimental data. Our results showed a significant increase in antioxidant activity, suggesting the potential of this oil as a protective agent against oxidative damage. The results reveal important information concerning the antimicrobial activity of the various substances tested against multiple bacterial and fungal strains. The results suggest that the substances tested have selective antimicrobial activity depending on the target strains. Some substances have significant inhibitory effects against certain strains but do not affect others. This specificity can be attributed to differences in the chemical composition of the substances tested and the biological and genetic characteristics of the target strains. MIC provides a quantitative estimate of the concentration required to inhibit microbial growth. The oils show comparable MIC for specific strains, indicating a certain consistency in their efficacy at specific concentrations.
OEHx, OECh, and OEPr oils show moderate antimicrobial activity against certain bacterial and fungal strains, particularly C. Albicans and F. proliferatum. These results suggest that the extraction processes used to obtain these oils appear to preserve certain components with inhibitory properties against certain microorganisms. However, it is essential to note that several factors, such as the chemical composition of the oils, the presence of volatile compounds, and other environmental factors, could influence the antimicrobial activity of the oils obtained by these processes. Fluconazole, a commonly used medicinal antifungal, showed significantly higher antimicrobial activity than the oils tested. This observation is consistent with expectations, as antifungal drugs are specifically designed to target pathogenic fungi. In contrast, natural oils may contain a broader range of compounds and may not have such strong specificity.
Considering the determined zones of inhibition, the high activity of the fixed oils obtained by chloroform and hexane could be due to a difference in concentration of the different chemical groups present in these extracts.[Citation20,Citation59] Indeed, researchers have proved that phytochemical studies eliminate some chemical groups. In contrast, others are more concentrated, with a high proportion of alkaloids, flavonoids, sterols, and tannins whose antibacterial activities have been reported by several studies.[Citation60,Citation61]
The current state of the art in antimicrobial research is characterized by in-depth exploration of novel peptides, plant extracts, and their bioactive compounds.[Citation62] Identifying these compounds offers considerable opportunities for their application in key sectors such as medicine, where antiproliferative properties and enzyme inhibition activities may pave the way for innovative treatments.[Citation63] These investigations open up promising prospects in the fields of medicine, food, and health-related industries. Hybrid peptides, such as LENART01, are designed to enhance efficacy against various bacterial pathogens, representing a potential avenue for developing new therapeutic agents.[Citation64]
Nigella sativa seeds have been used for centuries in various medical traditions for their healing properties. They contain a range of bioactive compounds, such as thymoquinones, flavonoids and essential fatty acids, giving them anti-inflammatory, antioxidant, antimicrobial properties and more.[Citation65,Citation66] What’s more, the oil extracted from these seeds is widely used in phytotherapy, aromatherapy and the cosmetics industry for its skin and hair health benefits.
Black cumin honey is also recognized for its high biological value. Honey is naturally rich in nutrients, enzymes and antioxidants, and black cumin honey offers additional benefits due to the properties of Nigella sativa seeds. It is often used as a natural remedy to boost the immune system, relieve digestive disorders, promote wound healing and even as an adjuvant treatment for certain respiratory ailments.
This in-depth study of N. sativa seed oil extraction methods and their impact on chemical composition and antioxidant and antimicrobial properties has shed significant light on the potential benefits of this plant. Although the health benefits of N. sativa were highlighted, there are still areas for improvement, including exploring mechanisms of action, optimizing extraction methods for food applications, in vivo studies to validate in vitro results, and assessing the stability of bioactive compounds. These avenues of research offer significant potential for broadening our understanding of N. sativa and maximizing its beneficial applications in various fields, from nutrition to industry. Current results provide a solid basis for future studies aimed at exploiting the full potential of this plant in the context of health and food safety.
Conclusion
In conclusion, the study of different oil extraction processes from Nigella sativa L. seeds reveals significant variations in oil yield and the composition and antioxidant properties of the extracts obtained. The experimental results demonstrate that the choice of extraction mode significantly impacts the parameters studied and underline the importance of judiciously selecting the extraction process according to the desired applications for the extracted oils. These findings contribute to a better understanding of the extraction process’s effects on the oils’ characteristics and open up prospects for the potential use of these extracts in various fields, from health to the food and cosmetics industries.
Author’s contributions
Conceptualization, writing the original draft, reviewing and editing: Otmane Zouirech, Amira Metouekel, Azeddin El Barnossi, Farhan Siddique, Sumaira Nadeem. Formal analysis, investigations, funding acquisition, reviewing, and editing: Karima Mikou, Mohammed Bourhia, Hiba-Allah Nafidi, Turki M. Dawoud. Resources, data validation, data curation, and supervision: Badiaa Lyoussi, Elhoussine Derwich, Musaab Dauelbait.
Acknowledgments
The authors extend their appreciation to the Researchers Supporting Project number (RSP2024R197), King Saud University, Riyadh, Saudi Arabia.
Disclosure statement
No potential conflict of interest was reported by the author(s).
Additional information
Funding
References
- Proestos, C.; Boziaris, I. S.; Nychas, G. E.; Komaitis, M. Analysis of Flavonoids and Phenolic Acids in Greek Aromatic Plants: Investigation of Their Antioxidant Capacity and Antimicrobial Activity. Food Chem. 2006, 95(4), 664–671. DOI: 10.1016/j.foodchem.2005.01.049.
- Benmakhlouf, Z.; Benserradj, O.; Kellab, R. Short Communication: Identification of Phytochemical Constituents of Syzygium Aromaticum L. Using Gas Chromatography Coupled with Mass Spectrometry and Evaluation of Antimicrobial Activity. Biodiversitas J. Biol. Diversity 2022, 23(5), 2586–2593. DOI: 10.13057/biodiv/d230540.
- Arasu, A.; Pingley, V.; Prabha, N.; Ravikumar, O. V.; Annathurai, K.; Kasirajan, S.; Govindasamy, A.; Alwahibi, M. S.; Elshikh, M. S.; Abdel Gawwad, M. R., et al. Impact and Fungitoxic Spectrum of Trachyspermum Ammi Against Candida albicans, an Opportunistic Pathogenic Fungus Commonly Found in Human Gut That Causes Candidiasis Infection. J. Infect Public Health 2021, 14(12), 1854–1863. DOI: 10.1016/j.jiph.2021.09.027.
- Elujoba, A.; Odeleye, O. M.; Ogunyemi, C. M. Traditional Medicine Development for Medical and Dental Primary Health Care Delivery System in Africa. Afr. J. Trad. Compl. Alt. Med 2005, 2(1), 46–61. DOI: 10.4314/ajtcam.v2i1.31103.
- Salhi, S.; Fadli, M.; Zidane, L.; Douira, A. Etudes floristique et ethnobotanique des plantes médicinales de la ville de Kénitra (Maroc). LAZAROA 2011, 31, 133–146. DOI: 10.5209/rev_LAZA.2010.v31.9.
- Edris, A. E. Evaluation of the Volatile Oils from Different Local Cultivars of Nigella Sativa L. Grown in Egypt with Emphasis on the Effect of Extraction Method on Thymoquinone. J. Essent. Oil Bear. Plants 2010, 13(2), 154–164. DOI: 10.1080/0972060X.2010.10643805.
- Kandouli, C.; Cassien, M.; Mercier, A.; Delehedde, C.; Ricquebourg, E.; Stocker, P.; Mekaouche, M.; Leulmi, Z.; Mechakra, A.; Thétiot-Laurent, S., et al. Antidiabetic, Antioxidant and Anti in Fl Ammatory Properties of Water and N - Butanol Soluble Extracts from Saharian Anvillea Radiata in High-Fat-Diet Fed Mice. J. Ethnopharmacol. 2017, 207, 251–267. DOI: 10.1016/j.jep.2017.06.042.
- Zouirech, O.; El Moussaoui, A.; Saghrouchni, H.; Gaafar, A. R. Z.; Nafidi, H. A.; Bourhia, M.; Khallouki, F.; Lyoussi, B.; Derwich, E. Prefatory in silico Studies and in vitro Insecticidal Effect of Nigella Sativa (L.) Essential Oil and Its Active Compound (Carvacrol) Against the Callosobruchus Maculatus Adults (Fab), a Major Pest of Chickpea. Open Chem. 2023, 21(1), 1–12. DOI: 10.1515/chem-2023-0133.
- Favier, A. Stress oxydant et pathologies humaines. Annales Pharmaceutiques Françaises 2006, 64(6), 390–396. DOI: 10.1016/s0003-4509(06)75334-2.
- Karaçil Ermumucu, M. Ş.; Şanlıer, N. Black cumin (Nigella sativa) and its active component of thymoquinone: effects on health. J. Food Heal. Sci 2017, 3, 170–183. DOI: 10.3153/jfhs17020.
- Verpoorte, R. Pharmacognosy in the New Millennium: Leadfinding and Biotechnology Pharmacognosy in the New Millenium: Leadfinding and Biotechnology. 2014. DOI: 10.1211/0022357001773931.
- Hseini, S.; Kahouadji, A. Étude ethnobotanique de la flore médicinale dans la région de Rabat (Maroc occidental). Lazaroa 2007, 28, 79–93.
- Fadil, M.; Farah, A.; Haloui, T.; Rachiq, S. Étude ethnobotanique des plantes exploitées par les coopératives et les associations de la région Meknès-Tafilalet au Maroc. 2014. DOI: 10.1007/s10298-014-0902-2.
- Ouattar, H.; Zouirech, O.; Kara, M.; Assouguem, A.; Almutairi, S. M.; Al-Hemaid, F. M.; Rasheed, R. A.; Ullah, R.; Abbasi, A. M.; Aouane, M., et al. In vitro Study of the Phytochemical Composition and Antioxidant, Immunostimulant, and Hemolytic Activities of Nigella Sativa (Ranunculaceae) and Lepidium Sativum Seeds. Mol. Artic 2022, 27(18), 5946. DOI: 10.3390/molecules27185946.
- Favier, A. Le stress oxydant Intérêt conceptuel et expérimental dans la compréhension des mécanismes des maladies et potentiel thérapeutique. L’actualité Chimique. 2003, 108(10):863–832.
- Nath, K. A.; Norby, S. M. Reactive Oxygen Species and Acute Renal Failure. Am. J. Med. 2000, 109(8), 665–678. DOI: 10.1016/S0002-9343(00)00612-4.
- Bandyopadhyay, U.; Das, D.; Banerjee, R. K. Reactive Oxygen Species: Oxidative da and Pathogenesis. Curr. Sci. 1999, 77, 658–666.
- Mmbengwa, V.; Samie, A.; Gundidza, M.; Matikiti, V.; Ramalivhana, N. J.; Magwa, M. L. Biological Activity and Phytoconstituents of Essential Oil from Fresh Leaves of Eriosema Englerianum. Afr. J. Biotechnol. 2009, 8, 361–364. DOI: 10.4314/ajb.v8i3.59812.
- Atta, M. B. Some Characteristics of Nigella (Nigella Sativa L.) Seed Cultivated in Egypt and Its Lipid Profile. Food Chem. 2003, 83, 63–68. DOI: 10.1016/S0308-8146(03)00038-4.
- Zouirech, O.; Alyousef, A. A.; Barnossi, A. E.; Moussaoui, A. E.; Bourhia, M.; Salamatullah, A. M.; Ouahmane, L.; Giesy, J. P.; Aboul-Soud, M. A. M.; Lyoussi, B., et al. Phytochemical Analysis and Antioxidant, Antibacterial, and Antifungal Effects of Essential Oil of Black Caraway (Nigella Sativa L.) Seeds Against Drug-Resistant Clinically Pathogenic Microorganisms. Biomed Res. Int. 2022, 2022(11), 1–11. DOI: 10.1155/2022/5218950.
- Singh, G.; Marimuthu, P.; De Heluani, C. S.; Catalan, C. Chemical Constituents and Antimicrobial and Antioxidant Potentials of Essential Oil and Acetone Extract of Nigella Sativa Seeds. J. Sci. Food Agric. 2005, 85(13), 2297–2306. DOI: 10.1002/jsfa.2255.
- Bouslamti, M.; Metouekel, A.; Chelouati, T.; El Moussaoui, A.; Barnossi, A. E.; Chebaibi, M.; Nafidi, H. A.; Salamatullah, A. M.; Alzahrani, A.; Aboul-Soud, M. A. M., et al. Solanum elaeagnifolium Var. Obtusifolium (Dunal) Dunal: Antioxidant, Antibacterial, and Antifungal Activities of Polyphenol-Rich Extracts Chemically Characterized by Use of in vitro and in silico Approaches. Molecules 2022, 27(24), 8688. DOI: 10.3390/molecules27248688.
- Mackinney, G. Absorption of Light by Chlorophyll Solutions. J. Biol. Chem. 1941, 140, 315–322. DOI: 10.1016/s0021-9258(18)51320-x.
- Innocent, I. O.; Igwilo, I. Physico-Chemical and Anti-Oxidant Properties of Moringa Oleifera Seed Oil. Pak. J. Nutr. 2011, 10(5), 409–414. DOI: 10.3923/pjn.2011.409.414.
- Lafka, T. I.; Sinanoglou, V.; Lazos, E. S. On the Extraction and Antioxidant Activity of Phenolic Compounds from Winery Wastes. Food Chem. 2007, 104, 1206–1214. DOI: 10.1016/j.foodchem.2007.01.068.
- Karoui, I. J.; Ayari, J.; Ghazouani, N.; Abderrabba, M. Physicochemical and Biochemical Characterizations of Some Tunisian Seed Oils. Lipids - Oilseeds Fats, Crop. OCL 2020, 27(9), 29. DOI: 10.1051/ocl/2019035.
- Puntia, D. E. B. A. O.; Indica, F. Activites anti-radicalaires d’extraits de jus de fruits du figuier de barbarie (opuntia ficus indica) b. Leban. Sci. J 2006, 7, 3–8.
- Lopes-Lutz, D.; Alviano, D. S.; Alviano, C. S.; Kolodziejczyk, P. P. Screening of Chemical Composition, Antimicrobial and Antioxidant Activities of Artemisia Essential Oils. Phytochemistry 2008, 69(8), 1732–1738. DOI: 10.1016/j.phytochem.2008.02.014.
- Balouiri, M.; Sadiki, M.; Ibnsouda, S. K. Methods for in vitro Evaluating Antimicrobial Activity: A Review. J. Pharm. Anal. 2016, 6(2), 71–79. DOI: 10.1016/j.jpha.2015.11.005.
- Lafraxo, S.; El Barnossi, A.; El Moussaoui, A.; Bourhia, M.; Salamatullah, A. M.; Alzahrani, A.; Akka, A. A.; Choubbane, A.; Akhazzane, M.; Aboul-Soud, M. A. M., et al. Essential Oils from Leaves of Juniperus Thurifera L. Exhibiting Antioxidant, Antifungal and Antibacterial Activities Against Antibiotic-Resistant Microbes. Horticulturae 2022, 8(4), 321. DOI: 10.3390/horticulturae8040321.
- Elegbede, J. A.; Lateef, A.; Azeez, M. A.; Asafa, T. B.; Yekeen, T. A.; Oladipo, I. C.; Hakeem, A. S.; Beukes, L. S.; Gueguim-Kana, E. B. Silver-Gold Alloy Nanoparticles Biofabricated by Fungal Xylanases Exhibited Potent Biomedical and Catalytic Activities. Biotechnol. Prog. 2019, 35(5). DOI: 10.1002/btpr.2829.
- El Barnossi, A.; Moussaid, F.; Iraqi, H. A. Antifungal Activity of Bacillus sp. Gn-A11-18 Isolated from Decomposing Solid Green Household Waste in Water and Soil Against Candida albicans and Aspergillus Niger. E3S Web Conf. 2020, 150, 02003. DOI: 10.1051/e3sconf/202015002003.
- Khan, L. M.; Hanna, M. A. Expression of Oil from Oilseeds—A Review. J. Agric. Eng. 1983, 28(6), 495–503. DOI: 10.1016/0021-8634(83)90113-0.
- Santori, G.; Di, G.; Moglie, M.; Polonara, F. A Review Analyzing the Industrial Biodiesel Production Practice Starting from Vegetable Oil Refining. Appl. Energy 2012, 92, 109–132. DOI: 10.1016/j.apenergy.2011.10.031.
- Kumar, S. P. J.; Prasad, S. R.; Banerjee, R.; Agarwal, D. K.; Kulkarni, K. S.; Ramesh, K. V. Green Solvents and Technologies for Oil Extraction from Oilseeds. Chem. Cent. J 2017, 11(1), 1–7. DOI: 10.1186/s13065-017-0238-8.
- Saranya, B.; Sulfikarali, T.; Chindhu, S.; Muneeb, A. M.; Leela, N. K.; Zachariah, T. J. Turmeric and Cinnamon Dominate in Antioxidant Potential Among Four Major Spices. J. Spices Aromat. Crop 2017, 26, 27. DOI: 10.25081/josac.2017.v26.i1.803.
- Grodowska, K.; Parczewski, A. Organic Solvents in the Pharmaceutical Industry. Acta. Pol. Pharm. 2010, 67, 3–12.
- Abrams, K.; Harvell, J. D.; Shriner, D.; Wertz, P.; Maibach, H.; Maibach, H. I.; Rehfeld, S. Effect of Organic Solvents on in vitro Human Skin Water Barrier Function. J. Invest. Dermatol. 1993, 101(4), 609–613. DOI: 10.1111/1523-1747.ep12366068.
- Mubarak, M.; Shaija, A.; Suchithra, T. V. Review Article a Review on the Extraction of Lipid from Microalgae for Biodiesel Production. Algal Res. 2015, 7, 117–123. DOI: 10.1016/j.algal.2014.10.008.
- Geow, C. H.; Tan, M. C.; Yeap, S. P.; Chin, N. L. A Review on Extraction Techniques and Its Future Applications in Industry. 2021, 2000302, 1–10. DOI: 10.1002/ejlt.202000302.
- Johnson, L. A.; Lusas, E. W. Comparison of Alternative Solvents for Oils Extraction. J. Am. Oil Chem. Soc. 1983, 60(2Part1), 229–242. DOI: 10.1007/BF02543490.
- Li, Y.; Fine, F.; Fabiano-Tixier, A.-S.; Abert-Vian, M.; Carre, P.; Pages, X.; Chemat, F. Evaluation of Alternative Solvents for Improvement of Oil Extraction from Rapeseeds. Comptes Rendus. Chimie 2014, 17(3), 242–251. DOI: 10.1016/j.crci.2013.09.002.
- CODEX STAN, 210: Toxicological Profile for N-HEXANE. 1999.
- Yara-Varón, E.; Li, Y.; Balcells, M.; Canela-Garayoa, R.; Fabiano-Tixier, A.-S.; Chemat, F. Vegetable Oils As Alternative Solvents for Green Oleo-Extraction, Purification and Formulation of Food and Natural Products. Molecules 2017, 22(9), 1–24. DOI: 10.3390/molecules22091474.
- Bruckner, J.V. Organic Solvents. In Mammalian Toxicology, Abou-Donia, M. B., Ed. Wiley, 2015; pp. 188–209.
- Habib, N.; Choudhry, S. HPLC Quantification of Thymoquinone Extracted from Nigella Sativa L. (Ranunculaceae) Seeds and Antibacterial Activity of Its Extracts Against Bacillus Species. Evidence-Based Complement. Altern. Med. 2021, 2021, 1–11. DOI: 10.1155/2021/6645680.
- Ramadan, M. F. Nutritional Value, Functional Properties and Nutraceutical Applications of Black Cumin (Nigella Sativa L.): An Overview. Int. J. Food Sci. Technol 2007, 42(10), 1208–1218. DOI: 10.1111/j.1365-2621.2006.01417.x.
- Bm, M.; Sk, O.; Gl, A. Biological and Chemical Evaluations of the Seeds of Nigella Sativa Linn (Ranunculaceae). J. Pharmacogn. Phytochem. 2020, 9, 3452–3457.
- Boudieb, K.; Kaki, S. A.; Amellal-Chibane, H. Effect of Maturation Degree on the Fixed Oil Chemical Composition, Phenolic Compounds, Mineral Nutrients and Antioxidant Properties of Pistacia Lentiscus L. Fruits. Not. Bot. Horti Agrobot. Cluj-Napoca Eff 2019, 47(3), 836–847. DOI: 10.15835/nbha47311534.
- Spotorno, V.; Mateo, C. M.; Ixtaina, V. Y.; Martı, M. L.; Diehl, B. W. K.; Nolasco, S. M.; Toma, M. C.; Tomás, M. C. Characterization of Chia Seed Oils Obtained by Pressing and Solvent Extraction. J. Food Compost. Anal. 2011, 24(2), 166–174. DOI: 10.1016/j.jfca.2010.08.006.
- Ward, J. A. Processing High Oil Content Seeds in Continuous Screw Presses. J. Am. Oil Chem. Soc. 1976, 53(6Part1), 261–264. DOI: 10.1007/BF02605697.
- Mrsa, M. S. A.; Mdr, O. M.; Douglas, H.; Coutinho, M.; Martins, G. Modulation of the Antibiotic Activity by the Mauritia Flexuosa (Buriti) Fixed Oil Against. 2018, 1–8. DOI: 10.3390/pathogens7040098.
- Kiralan, M.; Özkan, G.; Bayrak, A.; Fawzy, M. Physicochemical Properties and Stability of Black Cumin (Nigella Sativa) Seed Oil As Affected by Different Extraction Methods. Ind. Crop Prod. 2014, 57, 52–58. DOI: 10.1016/j.indcrop.2014.03.026.
- De Azevedo, A. B. A.; Kieckbush, T. G.; Tashima, A. K.; Mohamed, R. S.; Mazzafera, P.; Melo, S. A. B. V. D. Extraction of Green Coffee Oil Using Supercritical Carbon Dioxide. J. Supercrit. Fluids 2008, 44(2), 186–192. DOI: 10.1016/j.supflu.2007.11.004.
- Biegelmeyer, R.; Maria, J.; Andrade, M.; Ana, L.; Apel, M. A.; Dresch, R. R.; Marin, R. Comparative Analysis of the Chemical Composition and Antioxidant Activity of Red (Psidium Cattleianum) and Yellow (Psidium Cattleianum Var. Lucidum) Strawberry Guava Fruit. J. Food Sci. 2011, 76(7), C991–6. DOI: 10.1111/j.1750-3841.2011.02319.x.
- Asif, M. Chemistry and Antioxidant Activity of Plants Containing Some Phenolic Compounds. Chem. Int. 2015, 1, 35–52.
- Pietta, P.-G. Flavonoids As Antioxidants. J. Nat. Prod 2000, 63(7), 1035–1042. DOI: 10.1021/np9904509.
- Tlili, N.; Elfalleh, W.; Hannachi, H.; Yahia, Y. Screening of Natural Antioxidants from Selected Medicinal Plants. Int. J. Food Prop. 2013, 37–41. DOI: 10.1080/10942912.2011.576360.
- Tchuenkam, T.; Ornella, J.; Flore, T. N.; Tiencheu, B.; Tenyang, N.; Oben, A.; Ashu, E.; Marie, M. E.; Ufuan, A. A. Chemical and Antibacterial Properties of Lipids Extracted from Some Plant Seeds and Fruits Commonly Used in Cosmetics. Am. J. Food Sci. Technol. 2022, 10, 10–19. DOI: 10.12691/ajfst-10-1-2.
- Ndam, L. M.; Mih, A. M.; Fongod, A. G. N.; Tening, A. S. Original Research Article Phytochemical Screening of the Bioactive Compounds in Twenty (20) Cameroonian Medicinal Plants. Int. J. Curr. Microbiol. App. Sci. 2014, 3, 768–778.
- Abdali, Y. E.; Allali, A.; Lahkimi, A. Phytochemical Screening, and in vitro Antiradical and Phytochemical Screening, and in vitro Antiradical and Immunostimulant Potential of Linum Usitatissimum L. Pharmacologieonline 2021, 3, 602–604.
- Sinan, K. I.; Yagi, S.; Llorent-Martínez, E. J.; Ruiz-Medina, A.; Gordo-Moreno, A. I.; Stefanucci, A.; Mollica, A.; Bene, K.; Zengin, G. Understanding the Chemical Composition and Biological Activities of Different Extracts of Secamone Afzelii Leaves: A Potential Source of Bioactive Compounds for the Food Industry. Molecules 2023, 28(9), 1–18. DOI: 10.3390/molecules28093678.
- Mohammed, A. B. A.; Yagi, S.; Tzanova, T.; Schohn, H.; Abdelgadir, H.; Stefanucci, A.; Mollica, A.; Mahomoodally, M. F.; Adlan, T. A.; Zengin, G. Chemical Profile, Antiproliferative, Antioxidant and Enzyme Inhibition Activities of Ocimum Basilicum L. and Pulicaria Undulata (L.) C.A. Mey. Grown in Sudan. South African J. Bot. 2020, 132, 403–409. DOI: 10.1016/j.sajb.2020.06.006.
- Serafin, P.; Kowalczyk, P.; Mollica, A.; Stefanucci, A.; Laskowska, A. K.; Zawadzka, M.; Kramkowski, K.; Kleczkowska, P. Evaluation of Antimicrobial Activities Against Various E. Coli Strains of a Novel Hybrid Peptide—LENART01. Molecules 2023, 28(13), 4955. DOI: 10.3390/molecules28134955.
- Kemal, M.; Esertaş, Ü. Z. Ü.; Kanbur, E. D.; Kara, Y.; Özçelik, A. E.; Can, Z.; Kolaylı, S. Characterization of the Black Cumin (Nigella Sativa L.) Honey from Türkiye. Food Biosci. 2023, 53, 102760. DOI: 10.1016/j.fbio.2023.102760.
- Kolayli, S.; Kazaz, G.; Özkök, A.; Keskin, M.; Kara, Y.; Demir Kanbur, E.; Ertürk, Ö. The Phenolic Composition, Aroma Compounds, Physicochemical and Antimicrobial Properties of Nigella Sativa L. (Black Cumin) Honey. Eur. Food Res. Technol. 2023, 249(3), 653–664. DOI: 10.1007/s00217-022-04160-2.