Abstract
In this study, 10% CuO/Al2O3 catalyst was used in a catalytic wet-air oxidation process to remove chemical oxygen demand (COD) and color from experimentally designed wastewater containing lignin. The catalyst was prepared using an impregnation method and was characterized by X-ray diffraction (XRD), atomic absorption spectroscopy (AAS), and Brunauer-Emmett-Teller method (BET) for surface area before use. A series of Box-Behnken design (BBD) experiments were used to identify the conditions (temperature, pressure, reaction time, and catalysts) necessary for the COD removal process. The predicted model had R2 and R2adj correlation coefficients of 0.98 and 0.97, respectively. Pressure only and the interaction effect between temperature and pressure were found to have a significant effect on COD removal (both confidence interval [CI] 95%). Finally, response surface methodology (RSM)-optimized results suggested that 92% of COD could be removed in 1 L of experimental wastewater with a lignin concentration 350 g/L in 120 min under the following conditions: a reaction temperature of 185 °C, a pressure of 10 bars, and catalyst loading of 1 mg/L. The experiment, performed in triplicate, yielded a COD removal of 90 ± 2%. The results are believed to be of importance to pulp and paper industrial wastewater treatment and other similar applications.
Implications: Catalytic wet-air oxidation (CWAO) has been used as an alternative to overcome problems related to the high temperatures and pressures required by the traditional wet-air oxidation. CWAO has been widely applied to treat various industrial wastewaters. To reduce the overall operational cost, it is necessary to identify the optimal condition required when designing wastewater treatment plant processes. In this work, the authors had successfully demonstrated the application of response surface methodology (RSM) with the Box-Behnken design (BBD) as a means of elucidating the complicated interaction effects between parameters.
Introduction
The wastewater produced by the pulping process contains high levels of biochemical oxygen demand (BOD), chemical oxygen demand (COD), suspended solids (SS), and organic toxins (Pokhrel and Viraraghavan, Citation2004; Catalkaya and Kargi, Citation2007, Citation2008). Although many of these substrates can be removed, discharged water often retains a noticeably black color, which alarms downstream communities who will understandably complain about the pulp processing in paper factories. The black color results from the nontoxic lignin in the black liquor discharged in concentrations ranging from 35% to 40%. Lignin is an organic compound that is not easily degraded by usual biological treatments of wastewater.
Wet-air oxidation (WAO) is the most common oxidative technique used to treat industrial wastewater containing high concentrations of organic pollutants. WAO uses oxygen as an oxidation agent, high temperatures (up to 320 °C), and high pressures (up to 20 bars) to oxidize organic pollutants in water and convert organic compounds to CO2 and H2O and other by-products without producing nitrogen oxides (NOx), sulfur dioxide (SO2, dioxin, and furan (Luan et al., Citationin press). Unfortunately, the high temperatures and high pressures required when applying WAO makes processing cost prohibitive and limits its application.
Catalytic wet-air oxidation (CWAO) uses catalysts to lower the temperature and pressures needed, increases the reaction rate, and shortens the reaction time. Both homogenous and heterogeneous catalysts have been widely used in this process (Levec and Pintar, Citation2007). Although homogeneous catalysts, such as copper, have high catalytic reactivity (Kim and Ihm, Citation2011), once the reaction is complete, it is difficult to separate them from treated wastewater. Thus, heterogeneous metal-oxide catalysts, such as CuO (Kim and Ihm, Citation2007; Hua et al., Citation2013), TiO2 (Cervantes et al., Citation2013; Pintar et al., Citation2008), graphene oxide (Yang et al., Citation2014), and ZrO2 (Wei et al., Citation2013) have recently been used. Of these catalysts, CuO is highly active in the decomposition of phenolic compounds, including phenol (Ayusheev et al., Citation2014; Domínguez et al., Citation2014; Lefèvre et al., Citation2011; Wang et al., Citation2014; Yan et al., Citation2014; Yang et al., Citation2014), dye (Ovejero et al., Citation2013; Fu and Kyzas, Citation2014), and chlorophenol (Wei et al., Citation2013a; Zhou et al., Citation2014; Lee et al., Citation2012). We previously found that 10 wt% CuO/Al2O3 could catalyze the wet oxidation of aniline, making possible high degradation to CO2 at temperatures ranging from 160 to 230 °C under air pressure ranging from 5 to 10 bars for 2 hr (Sriprom et al., Citation2014). CuO should be able to actively degrade lignin, a phenolic polymer, in water. In a preliminary study, 10 wt% CuO/Al2O3 was found to be highly active in the removal of lignin and COD under mild conditions (Sriprom et al., Citation2014). That study found COD removal to be affected by reaction temperature, pressure, time, and catalyst loading, although it did not investigate what optimal conditions would be or study the interaction effects of these variables in the removal process. We explore these concerns in this study.
Response surface methodology (RSM) is a statistically based method widely used to design experiments, estimate the main and the interaction effects of the independent variables, and find optimal condition values with a limited number of experiments (Song et al., Citation2014; Khataee et al., Citation2011). Along with RSM, Box-Behnken design (BBD) has been used to design experiments and limit number of experiments to reduce cost while not sacrificing illustrative conclusions. In advanced oxidation process (AOP) experiments, Box-Behnken design has been used to identify factors to be studied and RSM has been used to determine optimal values of these factors and predict their outcomes of (Mokhtar et al., Citation2014; Parilti and Akten, Citation2011; Jin et al., Citation2014). However, they have not been used in this way to determine the optimum conditions for CWAO removal of COD in pulp and paper wastewater.
In this study, Box-Behnken design was used to identify the conditions (temperature, pressure, reaction time, and catalysts) necessary in a catalytic wet-air oxidation process using 10% CuO/Al2O3 catalyst to remove chemical oxygen demand (COD) and color from experimentally designed wastewater containing lignin, and response surface methodology (RSM) was used to determine their optimal values. Knowing the optimal conditions and understanding the interaction effects should help paper pulp factories treat wastewater more efficiently.
Materials and Methods
Materials and catalyst preparation
Our catalyst support was γ-Al2O3, prepared by a precipitation method (Sriprom et al., Citation2014). Aluminum nitrate Al(NO3)3, which was purchased from Ajax Laboratory Chemicals Ltd. (Sydney, Australia), was dissolved in distilled water to prepare an aluminum nitrate solution. A 30 wt% NH4(OH) solution was obtained from Panreac Ltd. (Barcelona, Spain). The NH4(OH) solution was gradually dropped into aluminum nitrate solution to adjust pH (to keep pH constant at 9) and gently stirred until gel was formed as aluminium hydroxide (Al(OH)3). The gel was washed with distilled water and filtrated. It was dried at 100 °C overnight and calcined under an air temperature of 600 °C for 4 hr.
Copper supported on γ-Al2O3 catalysts was prepared using an impregnation method (Kaewpuang-Ngam et al., Citation2004). We prepared the Cu(NO3)2 solution by dissolving Cu(NO3)2∙2.5H2O (Ajax Laboratory Chemicals, Ltd., Sydney, Australia) with distilled water. The solution was dropped into γ-Al2O3 support. The mixture was stirred and heated at 80 °C for 4 hr, dried at 110 °C overnight, and then calcined at 500 °C for 4 hr.
Catalyst characterization
The Brunauer-Emmett-Teller (BET) surface areas of prepared catalysts were analyzed by nitrogen adsorption at −196 °C using Micrometrics ASAP 2010 (Micromeritics Instrument Corp, Georgia, USA). Phase analyses of the fresh and the used catalysts were performed by X-ray diffraction (XRD; Bruker AXS-D8; Karlsruhe, Germany) using CuKα radiation (λ = 0.15406 nm) with an anode current of 30 mA and an accelerating voltage of 40 kV. The samples were scanned from 20° to 80° (2θ) in scanning step of 0.02° at a rate of 5° min−1.
Experimental design and optimization of COD removal
BBD was used to design the experiment and determine the optimal conditions for COD removal. As recommended by Zhang and Zheng and Wantala et al. (Wantala et al., Citation2012; Z. Zhang and Zheng, Citation2009), we used four main BBD steps to achieve this. These steps involved (1) performing statistically designed experiments tailored to our experimental goals; (2) proposing a statistical model based on the experimental results and analysis of variance (ANOVA); (3) using diagnostic plots to confirm the adequacy of the model; and (4) predicting the response variables and verifying the model.
We measured four variables at three levels (−1, 0, 1) based on minimum to maximum range conditions. These independent variables were temperature (X1), pressure (X2), reaction time (X3), and the catalyst loading (X4) (). The BBD was obtained using MINITAB version 16.0 (Pennsylvania, USA). A set of 28 experimental runs was generated (shown in the first five columns of ).The percentage COD removal (Y) was calculated as in eq 1:
Table 1. The experimental design of CWAO of lignin synthetic wastewater using 10 wt% CuO/Al2O3 catalysts
Table 2. The combinatorial optimization of CWAO removal of COD from lignin synthetic wastewater
where C0 and Ct are the initial COD and COD at a specific time, respectively.
In the current study, RSM was used to identify the main and the interaction effects of the operating catalytic wet-air oxidation variables of COD removal from lignin solution under different conditions. The responses were evaluated and analyzed using MINITAB 16.0. Model fit was expressed as coefficient of determination, R2. The final terms to be used in our model were selected based on response P values with 95% confidence levels. The responses were then analyzed by ANOVA. The interaction of operating parameters was evaluated. Optimal conditions were determined using the response optimizing function in MINITAB 16.0. Additional experiments under these optimal conditions were performed in triplicate.
Catalytic wet-air oxidation experiments
Synthetic wastewater containing softwood lignin was used as a substitute for wastewater from a pulp and paper wastewater pre-treatment plant. The softwood lignin solution was prepared by dissolving 0.35 g of softwood lignin (Sigma-Aldrich, St. Louis, MO, USA) in 1000 mL of distilled water to an initial concentration of 350 mg/L, providing 1100 mg/L of COD. The pH of this solution was adjusted by NaOH until it reached a pH of 9. This concentration is equivalent to a typical influent COD concentration of pulp and paper wastewater treatment plant. The CWAO was performed in a 1000-mL high-pressure reactor equipped with a stirrer. A stirring rate of 500 rpm was used to ensure the proper mass transfer of air in the liquid phase. The reactor was heated using a temperature controlled electrical heater. Four hundred milliliters of lignin synthetic wastewater with an initial concentration of 350 mg/L was added into the reactor with different catalyst loadings (0–1 g/L). Before the reaction was performed, air was fed into the reactor to increase the pressure to the level to be evaluated. The reaction was carried out under the various conditions presented in . After each run, the reactor was quenched to room temperature by electric fan. The solution was analyzed for the remaining concentration, and the percentage of COD removal (Y) was calculated using eq 1.
Analytical methods
COD of synthetic and treated wastewater were measured by closed reflux standard method (titration method). The dissolved metal ions in treated solutions were measured by atomic absorption spectrometer (AAS; PerkinElmer Analyze 100; Massachusetts, USA).
Results and Discussion
Characteristic of catalysts
The XRD patterns of support and CuO/Al2O3 catalysts are shown in . CuO/Al2O3 catalyst consisted of CuO crystalline phase and Al2O3 amorphous phases (), suggesting that the CuO was homogenously distributed over the surface of Al2O3 without formation of crystalline phase. As can be seen in , which shows XRD pattern of used versus fresh catalyst, the peak of Al2O3 was found to have disappeared after the reaction was completed, compared with fresh catalyst. According to Kaewpuang-Ngam et al. (Citation2004), the chemical form of the catalyst after reactions catalyzed by catalysts containing gamma alumina (γ-Al2O3) was found as boehmite (AlOOH). This shows that free alumina probably reacts with water and forms boehmite during the reaction (Kaewpuang-Ngam et al., Citation2004). Thus, the result suggests that the Al2O3 peak disappeared due to a chemical form change, as alumina catalysts may react with water during the reaction. Both used and fresh CuO/Al2O3 catalyst were found to have the same peaks (2θ = main peak 35.5°, 38.7°, and 63.3° vs. 2θ = 35.5° and 38.7°) (), indicating that CuO in the used catalyst was the same as the fresh catalyst. This finding was similar to that of a previous study that reported main peaks of 2θ = 35.5° and 38.7°̊ found in −111 and 111 reflections of the CuO crystalline (G. Zhang et al., Citation2011). In addition, the peaks were similar, and peak intensities were decreased slightly. However, the copper remained in CuO form. And using the N2 adsorption-desorption technique, the BET surface area of Al2O3 and 10 wt% CuO/Al2O3 to be 258 and 125 m2/g, respectively. And the associated adsorption of lignin was found to be about 50% on CuO/Al2O3 at room temperature.
Statistical analysis and model fitting
As can be seen in , 53.37–92.86% of the COD could be removed by catalytic wet-air oxidation using 10 wt% CuO/Al2O3 catalysts. We calculated the quadratic regression coefficients for the four variables to predict the outcome or response (Y):
where Y represents predicted COD removal (%) and X1, X2, X3, and X4 are the coded variables corresponding to the temperature, pressure, time, and catalyst loading, respectively.
The regression coefficients, standard error, and P values for eq 2 were calculated (). The quadratic model had an R2 of 0.98. An R2 of nearly 1.0 indicates a very close fit (Khataee and Dehghan, Citation2011; Mokhtar et al., Citation2014). We also tested our model using ANOVA (). The linear-model term of pressure (X2) and the quadratic-model terms of temperature (X12), pressure (X22), and catalyst loading (X42) were found to have a significant effect on removal of COD (all 95% confidence levels having a P value <0.05). In the model analyzing the interaction effect, only temperature and pressure (X1X2) was found to have a significant interaction effect. After removing variables not found to be significant from the regression, we were left with the following new COD removal regression:
Table 3. The estimated regression coefficients for % COD removal
This new regression eq 3 had an R2 of 0.98, indicating an excellent fit. , which summarizes ANOVA results, shows that all remaining terms were significant (P ≤ 0.05) except for X1 (P = 0.0550), which had a slightly a little higher 95% confidence level. The new COD removal regression was found to have a highly significant 95% confidence level (P value = 0.0000). The P values of linear, square, and interaction regressions were 0.000, 0.000, and 0.001, respectively. Together, these findings indicate that the new model (eq 3) adequately fit the experimental data (Salman, Citation2014).
Table 4. The estimated regression coefficients for new-model equation
In , which displays the plot for the predicted values versus the observed values for % COD removal, the observed points were distributed in a nearly straight line, indicating a highly satisfactory fit (R2 = 0.9830), further indicating that the model predicted the COD removal efficiencies very well.
Figure 2. The observed values (%) plotted against the predicted values (%) derived from model of COD removal of lignin from synthetic wastewater.
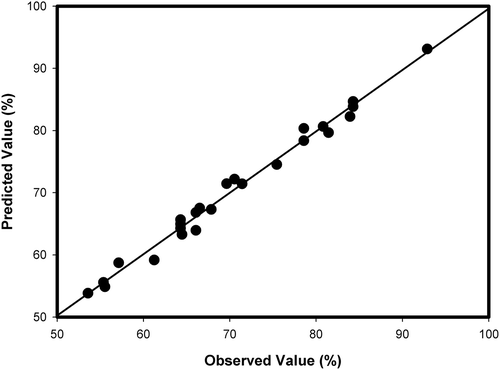
The predicted response values for catalytic wet-air oxidation over 10 wt% CuO/Al2O3 were obtained using eq 3 and experimental data in . The response model was checked using graphical analysis of residuals, which were calculated from the differences in the experimental and the predicted values. The residual plots for the quadratic regression model are shown in . The normal-probability plot of residuals is shown in , which shows a straight line pattern, indicating satisfaction of the normality assumption. The plot’s slight bend down on the left and its slight bend upward on the right suggest that the tails of error distribution were partly thinner than the normal distribution. This plot was not grossly nonabnormal. , which depicts the standardized residual and the fitted value (predicted response), shows random scattering, which suggests that the data seemed to exhibit the relatively constant variance across the predicted values and had no outliers or influential observations. The histogram chart, shown in , shows a normal curve. , a plot of the standardized residual versus the run order of COD removal by catalytic wet-air oxidation, shows fluctuation around the center line (in the range of ±2), indicating the data were distributed well. Together these plots show that the data were accurate and reliable. None of these plots show any serious problems with the model’s assumptions. No abnormality was found.
Main effects of variables
The main effects of the four independent variables on the removal of COD by catalytic wet-air oxidation are shown in . Increases in pressure and time were associated with a rapid increase the removal of COD from lignin synthetic wastewater. More oxygen dissolved into the aqueous system when pressure increased, and subsequently more hydroxyl radicals were generated, enhancing COD removal efficiency (Chang et al., Citation2005). In a study of the oxidation rate in phenol synthetic wastewater, Lin et al. (Citation2003) reported that the intermediates could not be further degraded when there was too much oxygen in the wastewater. That study attributed the inability to degrade intermediates on the saturation of oxygen on the surface of the catalyst; therefore, further increasing in oxygen partial pressure did not enrich the hydroxyl free radical (Lin et al., Citation2003).
Figure 4. The main effect plots for COD removal of lignin from synthetic wastewater by CWAO over 10 wt% CuO/Al2O3 catalysts.
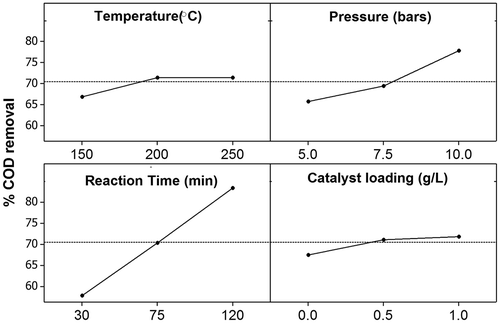
Moreover, COD removal rapidly increased between temperatures of 150–200 °C and between catalysts loading of 0 and 0.5 g/L and remained constant once these ranges were exceeded (). Theoretically, a raise in temperature increases the number of collisions between the reacting species, increasing the reaction rate. It also has been found that increases in catalyst loading, caused by the reaction between the organic compounds in the wastewater and hydroxyl radicals on the surface of the catalyst, increase pollutant degradation (Garg et al., Citation2007; Lin et al., Citation2003).
Interaction effects of variables
The results of our regression analysis (eq 3) of the interaction effects of the independent variables on the removal of COD are shown in . The line-plot in shows that the interaction effects between increases in temperature (150–250 °C) and pressure (5–10 bars) significantly enhanced the removal of COD. However, in the two-dimensional (2-D) contour plot (), at the left side of the saddle points, it was seen that COD removal increased as the temperature increased at a constant pressure. Surprisingly, at the right side of the saddle points, it was seen that COD removal decreased (or stayed constant) as the temperature increased. For example, when the system pressure was set at 10 bars, COD removal increased as the temperature increased from 150 to 250 °C. It is important to note that when the system pressure was set at 6 bars, COD removal increased from 150 to 200 °C, and decreased from 200 to 250 °C. These complications further suggest interaction effects among variables. While applying wet-air oxidation to treat textile dye wastewater, Demirel and Kayan (Citation2012) reported that the free radical easily occurred at high temperatures and oxygen-rich conditions. Their findings are in agreement with our observation at the 10 bars case. However, they did not report the complication that we observed in the case of 6 bars (or below 8 bars). This complication can be further explained by the effects of temperature. Increasing temperature played two roles in the reaction: (1) they increased the chance of oxygen molecule to collide onto the catalyst surface to produce free radical, considered as positive effect; and (2) they decreased the solubility of oxygen in the solution according to Henry’s law, which is considered a negative effect. In the case of 10 bars, the higher oxygen partial pressure (positive effect) over shadowed the negative effect; thus, COD removal rate continued increasing as temperatures increased. However, in cases of 6 bars or lower, when temperatures exceeded the saddle point (200–250 °C), negative effect overrode the positive effect, resulting in a drop in COD removal during temperature increases. Such a finding is very important, because without proper design, a treatment may consume too much energy (to heat up the temperature) and also become less efficient. This is more clearly seen in the 2-D contour plot, which provides further insight not shown on the line-plot. Similar results were observed in the 3-D response surface plot (), in which at the left side of the saddle points, COD removal increased as the temperature increased, and it decreased (or stayed constant) at the right side as the temperature further increased. Moreover, the response surface plot shows that COD removal always increased as the pressure increased in all the tested temperature ranges (150–250°C). Up to this point, one can see the advantages of using RSM because it provides more insight into the interaction effects of a reaction system. In this case, the interaction effects between temperature and pressure are well depicted. To the best of our knowledge, there is no study reporting a decrease in COD removal when temperature keeps increasing beyond the saddle point. Further research is needed to better understand this phenomenon.
Figure 5. The interaction effects between temperature and pressure on COD removal of lignin from synthetic wastewater by CWAO over 10 wt% CuO/Al2O3 catalysts: (a) interaction plot at the mean value of temperature, (b) contour plots, and (c) surface plot.
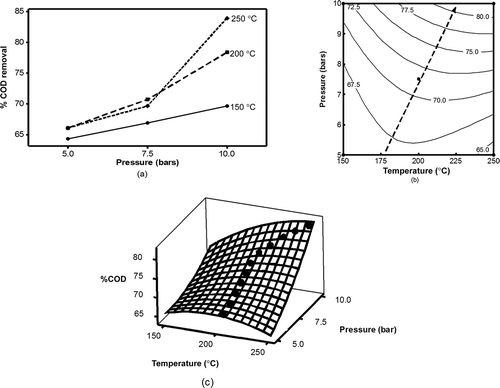
Nevertheless, it is clear that the COD removal (Y) was significantly affected by interaction effect of temperature and pressure (X1X2). When the pressure is increased, rises in oxygen concentration in the solution increased the COD removal rate. As the temperature rose at the left side of the saddle points (), the lignin molecules moved more quickly and increased collision and adsorption probability between lignin and the catalytic active site, resulting in a high rate of COD removal. This explanation is supported by Wei et al. (Citation2013) in a study using CWAO to treat pentachlorophenol in aqueous system.
Optimization of reaction condition and verification test
Finally, MINITAB 16.0 was used to optimize the model (eq 3) for the maximum response (% COD removal). Maximum COD removal (92%) was predicted by a reaction temperature of 185 °C, an initial pressure of 10 bars, a reaction time of 120 min, and a catalyst concentration of 1 g/L. The prediction of the model was verified by an additional three runs under the optimal conditions, resulting in a removal of 90 ± 2%. This experimental value closely agreed with theoretical values of 92% predicted by RSM, showing that the RSM was an effective and reliable method for determining the optimum conditions for the removal of COD from lignin synthetic wastewater. Additionally, it is worth mentioning that we performed AAS analysis of the wastewater after CWAO to investigate whether the catalyst CuO/Al2O3 remained stable after reaction and found that only minor amount (less than 0.1%) of copper in the catalyst had leached into the aqueous phase. Together, these finding further support the advantage of choosing the heterogeneous (rather than homogeneous) catalysts for the CWAO.
Conclusions
The main effects of variables and the interaction effects between temperature and pressure correlated significantly with the removal of COD from lignin synthetic wastewater by CWAO. The optimal conditions for a removal rate of 90 ± 2% was a temperature of 185 °C, a pressure of 10 bars, a reaction time of 120 min, and a catalyst loading of 1 g/L. In addition, it was found that by combining RSM and BBD, the optimum conditions for COD removal from lignin synthetic wastewater by CWAO using 10 wt% CuO/Al2O3 catalysts would be determined. And we found that COD removal may drop or stop increasing when temperature exceeds the saddle point. Such finding is very important when designing the CWAO system; otherwise, too much energy will be consumed and removal efficiency decreased. Finally, the proposed CuO/Al2O3 catalysts were found to be very stable after treatment. Therefore, CWAO-treated wastewater containing lignin can be discharged into the environment safely. CWAO should be able to reduce energy consumption and operational cost in the treatment of pulp wastewater.
Funding
This work was supported by the Higher Education Research Promotion and National Research University Project of Thailand, Office of the Higher Education Commission.
Orcid
Kitirote Wantala
http://orcid.org/0000-0001-6768-1996Arthit Neramittagapong
http://orcid.org/0000-0001-6768-1996Additional information
Funding
Notes on contributors
Pongsert Sriprom
Pongsert Sriprom is a Ph.D. candidate, Sutasinee Neramittagapong, Kitirote Wantala, and Arthit Neramittagapong are assistant professors at the Department of Chemical Engineering, Khon Kaen University, Khon Kaen, Thailand.
Chitsan Lin
Chitsan Lin is a professor at the Department of Marine Environmental Engineering, National Kaohsiung Marine University (NKMU), Kaohsiung, Taiwan.
Nurak Grisdanurak
Nurak Grisdanurak is an associate professor at the Department of Chemical Engineering, Thammasat University, Pathumthani, Thailand.
References
- Ayusheev, A.B., O.P. Taran, I.A. Seryak, O. Yu. Podyacheva, C. Descorme, M. Besson, L.S. Kibis, A.I. Boronin, A.I. Romanenko, Z.R. Ismagilov, V. Parmon 2014. Ruthenium nanoparticles supported on nitrogen-doped carbon nanofibers for the catalytic wet air oxidation of phenol. 7th International Conference on Environmental Catalysis (ICEC2012), Lyon, France. Appl. Catal. B Environ. 146:177–185. doi:10.1016/j.apcatb.2013.03.017
- Catalkaya, E.C., and F. Kargi. 2007. Color, TOC and AOX removals from pulp mill effluent by advanced oxidation processes: A comparative study. J. Hazard. Mater. 139:244–253. doi:10.1016/j.jhazmat.2006.06.023
- Catalkaya, E.C., and F. Kargi. 2008. Advanced oxidation treatment of pulp mill effluent for TOC and toxicity removals. J. Environ. Manage. 87:396–404. doi:10.1016/j.jenvman.2007.01.016
- Cervantes, A., G. Del Angel, G. Torres, G. Lafaye, J. Barbier Jr., J.N. Beltramini, J.G. Cabañas-Moreno, and A. Espinosa de los Monteros. 2013. Degradation of methyl tert-butyl ether by catalytic wet air oxidation over Rh/TiO2–CeO2 Catalysts. In Special Issue on Selected Contributions of the 4th International Symposium on New Catalytic Materials, Cancun (México), August 2011. Catal. Today 212:2–9. doi:10.1016/j.cattod.2012.11.021
- Chang, L., I.-P. Chen, and S.-S. Lin. 2005. An assessment of the suitable operating conditions for the CeO2/γ-Al2O3 catalyzed wet air oxidation of phenol. Chemosphere 58:485–492. doi:10.1016/j.chemosphere.2004.09.011
- Demirel, M., and B. Kayan. 2012. Application of response surface methodology and central composite design for the optimization of textile dye degradation by wet air oxidation. Int. J. Ind. Chem. 3:1–10. doi:10.1186/2228-5547-3-24
- Domínguez, C.M., A. Quintanilla, J.A. Casas, and J.J. Rodriguez. 2014. Kinetics of wet peroxide oxidation of phenol with a gold/activated carbon catalyst. Chem. Eng. J. 253:486–492. doi:10.1016/j.cej.2014.05.063
- Fu, J., and G.Z. Kyzas. 2014. Wet air oxidation for the decolorization of dye wastewater: An overview of the last two decades. Chin. J. Catal. 35:1–7. doi:10.1016/S1872-2067(12)60724-4
- Garg, A., I.M. Mishra, and S. Chand. 2007. Catalytic wet oxidation of the pretreated synthetic pulp and paper mill effluent under moderate conditions. Chemosphere 66:1799–1805. doi:10.1016/j.chemosphere.2006.07.038
- Hua, L., H. Ma, and L. Zhang. 2013. Degradation process analysis of the azo dyes by catalytic wet air oxidation with catalyst CuO/γ-Al2O3. Chemosphere 90:143–149. doi:10.1016/j.chemosphere.2012.06.018
- Jin, Y., Y. Wu, J. Cao, and Y. Wu. 2014. Optimizing decolorization of methylene blue and methyl orange dye by pulsed discharged plasma in water using response surface methodology. J. Taiwan Inst. Chem. Eng. 45:589–595. doi:10.1016/j.jtice.2013.06.012
- Kaewpuang-Ngam, S., K. Inazu, T. Kobayashi, and K.-I. Aika. 2004. Selective wet-air oxidation of diluted aqueous ammonia solutions over supported Ni catalysts. Water Res. 38:778–782. doi:10.1016/j.watres.2003.10.025
- Khataee, A.R., and G. Dehghan. 2011. Optimization of biological treatment of a dye solution by Macroalgae cladophora sp. using response surface methodology. J. Taiwan Inst. Chem. Eng. 42:26–33. doi:10.1016/j.jtice.2010.03.007
- Kim, K.-H., and S.-K. Ihm. 2007. Characteristics of titania supported copper oxide catalysts for wet air oxidation of phenol. Environ. Appl. Adv. Oxid. Process. 146:610–616. doi:10.1016/j.jhazmat.2007.04.063
- Kim, K.-H., and S.-K. Ihm. 2011. Heterogeneous catalytic wet air oxidation of refractory organic pollutants in industrial wastewaters: A review. J. Hazard. Mater. 186:16–34. doi:10.1016/j.jhazmat.2010.11.011
- Lee, C.-Li, C. Lin, and C.-J.G. Jou. 2012. Microwave-induced nanoscale zero-valent iron degradation of perchloroethylene and pentachlorophenol. J. Air Waste Manage. Assoc. 62:1443–1448. doi:10.1080/10962247.2012.719579
- Lefèvre, S., O. Boutin, J.-H. Ferrasse, L. Malleret, R. Faucherand, and A. Viand. 2011. Thermodynamic and kinetic study of phenol degradation by a non-catalytic wet air oxidation process. Chemosphere 84:1208–1215. doi:10.1016/j.chemosphere.2011.05.049
- Levec, J., and A. Pintar. 2007. Catalytic wet-air oxidation processes: A review. Adv. Catal. Oxid. Process. 124:172–184. doi:10.1016/j.cattod.2007.03.035
- Lin, S.S., D.J. Chang, C.-H. Wang, and C.C. Chen. 2003. Catalytic wet air oxidation of phenol by CeO2 catalyst—Effect of reaction conditions. Water Res. 37:793–800. doi:10.1016/S0043-1354(02)00422-0
- Luan, M., G. Jing, Y. Piao, D. Liu, and L. Jin. In press. Treatment of refractory organic pollutants in industrial wastewater by wet air oxidation. Arabian J. Chem. doi:10.1016/j.arabjc.2012.12.003
- Mokhtar, W.N.A.W., W.A.W.A. Bakar, R. Ali, and A.A.A. Kadir. 2014. Deep desulfurization of model diesel by extraction with N, N-dimethylformamide: Optimization by Box–Behnken design. J. Taiwan Inst. Chem. Eng. 45:1542–1548. doi:10.1016/j.jtice.2014.03.017
- Ovejero, G., A. Rodríguez, A. Vallet, and J. García. 2013. Catalytic wet air oxidation of a non-azo dye with Ni/MgAlO catalyst. Chem. Eng. J. 215–216:168–173. doi:10.1016/j.cej.2012.11.028
- Parilti, N.B., and D. Akten. 2011. Response surface methodological approach and the kinetic study for the assessment of the photodegradation of a pulp mill effluent with H2O2/Fe(III)/solar UV. Fresenius Environ. Bull. 20:1390–1400.
- Pintar, A., J. Batista, and T. Tišler. 2008. Catalytic wet-air oxidation of aqueous solutions of formic acid, acetic acid and phenol in a continuous-flow trickle-bed reactor over Ru/TiO2 catalysts. Appl. Catal. B Environ. 84:30–41. doi:10.1016/j.apcatb.2008.03.001
- Pokhrel, D., and T. Viraraghavan. 2004. Treatment of pulp and paper mill wastewater—A review. Sci. Total Environ. 333:37–58. doi:10.1016/j.scitotenv.2004.05.017
- Salman, J.M. 2014. Optimization of preparation conditions for activated carbon from palm oil fronds using response surface methodology on removal of pesticides from aqueous solution. Arabian J. Chem. Special Issue: Environmental Chemistry 7:101–108. doi:10.1016/j.arabjc.2013.05.033
- Song, C., Y. Kitamura, and S. Li. 2014. Optimization of a novel cryogenic CO2 capture process by response surface methodology (RSM). J. Taiwan Inst. Chem. Eng. 45:1666–1676. doi:10.1016/j.jtice.2013.12.009
- Sriprom, P., S. Neramittagapong, A. Neramittagapong. 2011. Optimization for lignin removal from synthetic wastewater by catalytic wet air oxidation. Paper presented at the TIChE International Conference, Hatyai, Songkla, Thailand, November 10–11, 2011.
- Sriprom, P., P. Assawasaengrat, A. Neramittagapong, and S. Neramittagapong. 2014. Catalytic wet-air oxidation of aniline removal from synthetic wastewater. Adv. Mater. Res. 931–932:32–36. doi:10.4028/www.scientific.net/AMR.931-932.32
- Wang, J., W. Fu, X. He, S. Yang, and W. Zhu. 2014. Catalytic wet air oxidation of phenol with functionalized carbon materials as catalysts: Reaction mechanism and pathway. J. Environ. Sci. 26:1741–1749. doi:10.1016/j.jes.2014.06.015
- Wantala, K., E. Khongkasem, N. Khlongkarnpanich, S. Sthiannopkao, and K.-W. Kim. 2012. Optimization of As(V) adsorption on Fe-RH-MCM-41-immobilized GAC using Box–Behnken design: Effects of pH, loadings, and initial concentrations. Recent Progress Environ. Geochem. 27: 1027–1034. doi:10.1016/j.apgeochem.2011.11.014
- Wei, H., X. Yan, S. He, and C. Sun. 2013. Catalytic wet air oxidation of pentachlorophenol over Ru/ZrO2 and Ru/ZrSiO2 catalysts. Catal. Low Carbon Energy Dev. Environ. Qual. Control 201:49–56. doi:10.1016/j.cattod.2012.07.004
- Yang, S., Y. Cui, Y. Sun, and H. Yang. 2014. Graphene oxide as an effective catalyst for wet air oxidation of phenol. J. Hazard. Mater. 280:55–62. doi:10.1016/j.jhazmat.2014.07.051
- Yan, Y., S. Jiang, and H. Zhang. 2014. Efficient catalytic wet peroxide oxidation of phenol over Fe-ZSM-5 catalyst in a fixed bed reactor. Sep. Purif. Technol. 133:365–374. doi:10.1016/j.seppur.2014.07.014
- Zhang, G., S. Wang, S. Zhao, L. Fu, G. Chen, and F. Yang. 2011. Oxidative degradation of azo dye by hydrogen peroxide electrogenerated in situ on anthraquinonemonosulphonate/polypyrrole composite cathode with heterogeneous CuO/γ-Al2O3 catalyst. Appl. Catal. B Environ. 106:370–378. doi:10.1016/j.apcatb.2011.05.042
- Zhang, Z., and H. Zheng. 2009. Optimization for decolorization of azo dye Acid Green 20 by ultrasound and H2O2 using response surface methodology. J. Hazard. Mater. 172:1388–1393. doi:10.1016/j.jhazmat.2009.07.146
- Zhou, S., C. Zhang, X. Hu, Y. Wang, R. Xu, C. Xia, H. Zhang, and Z. Song. 2014. Catalytic wet peroxide oxidation of 4-chlorophenol over Al-Fe-, Al-Cu-, and Al-Fe-Cu-pillared clays: Sensitivity, kinetics and mechanism. Appl. Clay Sci. 95:275–283. doi:10.1016/j.clay.2014.04.024