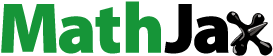
ABSTRACT
The concentrations of fine particles and selected gas pollutants in the flue gas entering the stack were measured under several common operation modes in an operating coal power plant producing electricity. Particle size distributions in a diameter range from 10 nm to 20 μm were measured by a scanning mobility particle sizer (SMPS), and the flue gas temperature and concentrations of CO2 and SO2 were monitored by a continuous emission monitoring system (CEMS). During the test campaign, five plant operating modes were studied: soot blowing, bypass of flue-gas desulfurization (FGD), reheat burner operating at 0% (turned off), 27%, and 42% (normal condition) of its full capacity. For wet and dry aerosols, the measured mode sizes were both around 40 nm, but remarkable differences were observed in the number concentrations (#/cm3, count per square centimeter). A prototype photoionizer enhanced electrostatic precipitator (ESP) showed improved removal efficiency of wet particles at voltages above +11.0 kV. Soot blowing and FGD bypass both increased the total particle number concentration in the flue gas. The temperature was slightly increased by the FGD bypass mode and varied significantly as the rating of reheat burner changed. The variations of CO2 and SO2 emissions showed correlations with the trend of total particle number concentration possibly due to the transitions between gas and particle phases. The results are useful in developing coal-fired power plant operation strategies to control fine particle emissions and developing amine-based CO2 capture technologies without operating and environmental concerns associated with volatile amine emissions.
Implications: The measurement of the fine particle size distributions in the exhaust gas under several common operating conditions of a coal-fired power plant revealed different response relations between aerosol number concentration and the operating condition. A photo-ionizer enhanced ESP was demonstrated to capture fine particles with higher efficiency compared to conventional ESPs, and the removal efficiency increased with the applied voltage. The characteristic information of aerosols and main gaseous pollutants in the exhaust gas is extremely important for developing and deploying CO2 scrubbers, whose amine emissions and operating effectiveness depends greatly on the upstream concentrations of fine particles, SO2, from the power plant.
Introduction
Coal-fired power plants have been regarded as primary contributors to particulate matter (PM) (Guttikunda and Jawahar Citation2014; Xiong, Jiang, and Gao Citation2016; Xu et al. Citation2017; Zhang et al. Citation2013), acidic gas (SO2, NOx) (Guttikunda and Jawahar Citation2014; Xiong, Jiang, and Gao Citation2016; Xu et al. Citation2017), and greenhouse gas (CO2) (Dang, Mba Wright, and Brown Citation2015; Liu et al. Citation2015; Tola et al. Citation2016) emissions. A source apportionment study showed that the contributions of sulfate and nitrate to PM2.5 from coal-fired power plants around the Yangtze River Delta region in China ranged between 37.3 and 72.7% and between 45.0 and 73.8%, respectively (Li et al. Citation2015a). For the same region in China, it was also reported that about 97% and 86% of the total SO2 and NOx emissions could be attributed to coal-fired power plants and other industrial facilities (Huang et al. Citation2011). Other researchers used continuous emission monitoring system (CEMS) data to conclude that U.S. coal-fired power plants were responsible for the largest annual CO2 emissions of all sources of combustion in the United States over the last decade (De Gouw et al., Citation2014). To address the issues of all these types of pollutants, various pollution control technologies are applied in electricity generation units (EGUs). Particle control devices, such as electrostatic precipitators (ESPs) and bag-house filters, have been widely applied downstream of coal boilers in power plants due to their high efficiency in removing fine particles. Huang et al. found that a lab-scale single-stage ESP could achieve >99% collection efficiency of particles with a diameter less than 20 nm (Huang and Chen Citation2002). Also, it has been demonstrated that a bag-house filter in a 220-MW coal-fired power plant could collect 99.57% of PM10 (Yi et al. Citation2008). Flue-gas desulfurization (FGD) (Meij and Te Winkel Citation2004; Zhao et al. Citation2008) technology has proven similarly effective in removing SO2. Meanwhile, the amine-based CO2 scrubbers are widely studied and well-established technologies for CO2 capture because they have great potential to be energy efficient with process and solvent improvements (Rochelle Citation2009).
Nevertheless, the introduction of an amine scrubber for CO2 capture raises another potential issue: The treated exhaust gas from a power plant may contain a large number of fine particles, which can act as condensation sites and carry a large amount of amine in the aerosol phase out of the CO2 scrubber, resulting in increased amine losses and causing environmental concerns. A recent assessment of the NOx control selective catalytic reduction (SCR) (Li et al. Citation2015b) indicated that the SCR treatment tremendously increased both the number and mass concentration of PM2.5 emitted by a coal-fired power plant. The presence of fine particles in the flue gas could be attributed to a “penetration window,” which is the size range of particles that pass through the ESP, around 100 nm for traditional ESPs (Strand et al. Citation2002; Ylätalo and Hautanen Citation1998; Zhuang et al. Citation2000). Consequently, a large number of fine particles provide seed nuclei onto which volatile amine species condense, causing significant amine emissions from CO2 scrubbers (Kamijo et al. Citation2013; Khakharia et al. Citation2015, Citation2013; Mertens et al. Citation2014; Citation2012; Citation2013). A laboratory study on CO2 capture found that when soot particles, which contain mostly carbon and result from incomplete coal combustion, were present in the flue gas, the amine emission loss was 2–4 times higher than that without fine particles present in the inlet gas (Khakharia et al. Citation2013). Mertens (Mertens et al. Citation2014) reported that particles with diameters below 100 nm were more likely to create amine mist emissions downstream of the CO2 scrubber. To reduce the negative effect of fine particles on amine emissions and CO2 capture operation at a full-scale power plant, it is important to know the quantity, size distribution, and composition of fine particle as well as gas impurities in the plant’s flue gas. A common approach to obtaining such information is to perform real-time flue gas measurements in a power plant under its actual operating conditions.
Numerous studies have focused on measuring the concentration of fine particles before or after a particle control unit, such as an ESP or a bag-house filter, because it is typically the principal unit that causes the most significant variation in particle concentration in the exhaust. Li et al. (Citation2009) used a scanning mobility particle sizer (SMPS) and an electrical low-pressure impactor (ELPI) to measure the particle size distribution in the flue gas upstream and downstream of a pilot-scale ESP, and found that adding photoionizers (such as a soft x-ray [SXR]) could enhance the particle removal efficiency in the submicrometer range. Strand et al. (Citation2002) studied the effect of an ESP and condenser on the particle concentrations from 10 nm to 10 µm in the exhaust gas from a 6-MW biomass combustor. They concluded that the exhaust gas condenser affected the particle mass concentration more significantly than the number concentration, while the ESP exhibited an opposite effect due to the re-entrainment of coarse particles caused by ESP rapping, which is a process of releasing the particles deposited on ESP electrodes by vibration. The removal efficiency of particles from 30 nm to 10 µm for a bag-house filter installed at a 220-MW anthracite coal-fired power plant was studied using an ELPI (Yi et al. Citation2008), and bimodal number concentration size distributions were observed before and after the bag-house filter. These studies indicate that the particle size distribution in the flue gas varies remarkably after different processing stages, and also varies when distinct types of fuel, combustors, or particle control devices are employed. However, the operating parameters of the boiler and flue gas treatment devices were kept constant and thus were not comprehensive to power plant operation in those studies. There are no investigations of which we are aware about how varying operating conditions of the major units in a dynamic power generation plant could affect the particle size and concentration and gas composition in the flue gas. This scientific gap needs to be bridged in terms of developing multipollutant emission mitigation.
In this study, the size distribution and mass concentration of particles and the concentrations of SO2 and CO2 in the flue gas were measured at a bituminous coal-fired power plant. The sampling point was downstream of an FGD scrubber and a reheat burner, and before the inlet of the stack. The aerosol and gas properties were investigated at five operating modes of the power plant: soot blowing in the boiler (releasing soot deposited on boiler wall), flue gas bypass of the FGD, reheat burner operating at 0% (off), and 27%, and 42% (normal steady-state condition) of its full capacity. The effects of these different conditions on the aerosol and gas characteristics are discussed. Also, the particle collection efficiency of a lab-scale ESP with SXR and without SXR were examined to demonstrate the potential improvement of using SXR. Notably, this study was conducted at a nameplate 35-MWe coal-fired power plant that is under a feasibility study to install a novel amine-based CO2 capture unit. Since the aerosol and gas concentrations in the flue gas might have significant influences on the potential amine loss and CO2 capture performance, the research outcome could provide insights for the design of the aerosol pretreatment and CO2 scrubbing devices.
Experimental design
Sampling location
The primary flue gas treatment units within the coal-fired power plant are shown in . Two boilers, boilers 5 and 7, both of which are of a chain-grate Stoker design, were in operation during the test campaign. The two boilers combined are capable of producing up to 350,000 lb/hr of steam, equivalent to 35 MWe electricity. An Illinois #6 high-sulfur coal was burned in the power plant. As shown in the figure, after heat exchange in the economizer and air preheater, the flue gas produced by each boiler passes through the ESP, where most of the combustion-generated fly ash and soot particles are captured. However, particles with diameters between 100 nm and 1 μm tend to penetrate the ESP (Huang and Chen Citation2002; Strand et al. Citation2002; Ylätalo and Hautanen Citation1998; Zhuang et al. Citation2000). The flue gases from both ESPs are mixed together and then pass through an a wet jet bubbling FGD scrubber to remove SOx species. To meet the discharge emission standard for opacity, the exhaust flue gas is generally reheated by a natural gas burner to inhibit condensation of water vapor or formation of droplets before the gases are emitted from the stack. The reheat burner can be operated at different ratings, and a higher reheating percentage is typically used during wintertime. A CEMS measures the concentration of SO2 and CO2 in the flue gas between the reheat burner and the stack. In this study, the aerosol sampling was conducted close to the CEMS sampling port, so that we could study the correlation between the properties of aerosols and exhaust gas species.
Experimental setup
A schematic diagram of the experimental setup for measuring the particle concentration and size distributions is shown in . A stainless-steel tube with an outer diameter (OD) of 0.5 inch and a length of 5 feet was used to take samples from the flue gas duct. The sampling tube was made long enough so that it drew particles from the center of the flue gas duct. The end of the tube in the flue gas duct was also bent at a right angle to achieve isokinetic sampling for a range of particle sizes in the submicrometer and micrometer regimes. The section of the sampling tube outside the flue gas duct was covered with a heating tape to suppress condensation of water vapor. A stream of particle-free air (18 lpm) from a compressed air tank was mixed with the sampled flue gas (1.5 lpm) at the outlet of the sampling tube to dilute the aerosols and reduce water vapor concentration. Before entering the measurement system, the diluted gas sample was split into two streams, of which one (13 lpm) passed to a vacuum pump and the other (6.5 lpm) to a silica-gel diffusion dryer to remove the water content. The desiccated gas stream was further split into two streams going to a scanning mobility particle sizer (SMPS, model 3076, TSI, Inc., Shoreview, MN) and an aerodynamic particle sizer (APS, Model 3321, TSI, Inc., Shoreview, MN) separately. The size distributions of particles from 10 nm to 450 nm were measured continuously in real time with the SMPS. The SMPS uses a differential mobility analyzer (DMA) to classify particles as a function of electrical mobility size, and a condensation particle counter (CPC) to measure particle concentrations. The APS operated by the time-of-flight theory measures the aerodynamic size distributions of particles ranging from 0.5 to 20 μm. In certain tests to determine the influence of water content on particle size distributions and number concentrations, the diffusion dryer was bypassed. In addition, a SidePak AM510 (TSI, Inc., Shoreview, MN) was used to determine the total mass concentration of the particles below 2.5 μm (PM2.5) in the flue gas. During the mass concentration measurement, the compressed air tank and vacuum pump were turned off, and a diffusion dryer and the SidePak were placed at the outlet of the sampling tube directly (shown in the dashed box in ), because the internal pump in the SidePak cannot withstand a pressure difference generated by the vacuum pump. Flow-rate controllers, SMPS, APS, and SidePak were calibrated in laboratory environment before the measurement campaign, and to ensure normal working environment of all instruments, the instruments were placed on the back seat of a vehicle and their surrounding temperature was maintained in appropriate range using vehicle air conditioner. The entire sampling line was ensured with no leakage both in the laboratory and in the field.
Experimental plan
Aerosol size distributions and concentrations were measured at five different operating modes of the power plant. During the test campaign, the two boilers were operated with a total coal feed rate of 10,614 kg/hr, generating a total flue gas flow rate of 204,116 kg/hr, equivalent to an actual scale of approximately 20 MWe. provides a brief summary of the experiments conducted in chronological order. In the Results and Discussion section, we present the test results in the order of reheat burner operating at 42% of its full capacity (normal steady-state condition), reheat burner operating at 0% and 27% of its full capacity, flue gas bypass of the FGD (FGD bypass), and soot blowing in the boilers. The reported particle size distribution corresponding to a certain condition is the averaged result of at least three sampling runs in the stable phase of that condition. For each condition, the temperature and gas concentrations are time-averaged values over the whole stable phase of that condition.
Table 1. Summary of performed experiments.
Results and discussion
This section first presents the particle characteristics during the normal steady state operation of the reheat burner. The effects of adding a diffusion dryer and a photo-ionizer (SXR)-enhanced ESP on the steady-state particle size distributions are elaborated. The next part of this section focuses on understanding how the modes of derated reheat burner, FGD bypass, and soot blowing affect the particle size distributions. The last subsection discusses variation of the temperature, CO2 concentration, and SO2 concentration along with the changing operating modes during the measurement.
Reheat burner operated at 42% of full capacity (normal steady-state condition)
and show the size distributions of aerosols downstream of the reheat burner during the normal operation of the power plant measured by SMPS and APS, respectively. The size distributions measured with and without diffusion dryers are both presented. A diffusion dryer ensured that only desiccated particles were measured. The standard deviation of the measurement was negligible, indicating a relatively constant aerosol property at the sampling location. For the results measured with a dryer (represented by white connected symbols), the particles had a mode diameter of 37.2 nm, a value similar to those found in previous studies on particle measurement in flue gas (Li et al. Citation2009; Ylätalo and Hautanen Citation1998). The concentration of particles at 37.2 nm was 3.3 × 108 #/cm3. The total particle number concentration (over the size range of 15.7 to 399.5 nm throughout this report) was calculated by integrating the size distribution, yielding a value of 9.0 × 109 #/cm3. It should be noted that the scales of the vertical axes in the SMPS and APS figures are different. Hence, it can be concluded that very few particles were larger than 0.5 μm, and most particles were in the range of 10 to 100 nm, consistent with the “penetration window” through a conventional ESP (Li et al. Citation2009; Ylätalo and Hautanen Citation1998; Zhuang et al. Citation2000). Ylatulo et al. found that the size of the particles with the highest number concentration at the outlet of an ESP was approximately 70 nm (Ylätalo and Hautanen Citation1998), which was higher than the observed peak size in . This slight distinction may be attributed to evaporation of volatile contents induced by the reheat burner. The penetration of fine particles could result in aerosol growth in the proposed amine-based CO2 scrubber downstream of the reheat burner, and eventually could cause amine loss, primarily due to a large total surface area of submicrometer particles available for volatile amine condensational growth (Schaber Citation1995).
Figure 3. Size distributions of particles with diameters between 10 nm and 20 μm when the reheat burner rating was 42% of full capacity (normal steady-state condition) (both with and without dryer): (a) SMPS and (b) APS.
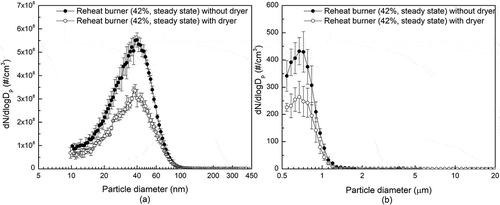
The black connected symbols in and display the size distributions of particles from the reheat burner without desiccation by a diffusion dryer. The highest number concentration was reached at around 40 nm, which was similar to the result for dry particles. However, the total number concentration of wet particles was around 1.50 × 1010 #/cm3, which was significantly higher than that of dry particles. The highest concentration was around 5.5 × 108 #/cm3, nearly double the result obtained when a diffusion dryer was used, which emphasizes the effect of water droplets in the original flue gas. Given the fact that the test campaign was conducted during winter and the flue gas duct was exposed to ambient air, the observed water vapor condensation could have occurred due to reduced temperature during transport in the duct. By implication, the reheat burner should operate at a higher rating when the outdoor temperature is remarkably low.
It has been demonstrated that SXR-enhanced ESP can boost the capture efficiency for ultrafine particles because SXR can increase ion concentrations by enhancing photoionization (Hogan, Lee, and Biswas Citation2004; Kulkarni et al. Citation2002). Kulkarni et al. found that the capture efficiency for particles ranging in diameter from 20 to 600 nm could increase remarkably when SXR was used with the ESP, and the SXR enhancement was augmented as the applied voltage was elevated from 5 kV to 10 kV (Kulkarni et al. Citation2002). However, there is no study on applying SXR to capture fine particles in coal-fired power plants. In our work, the capture efficiency of a lab-scale SXR-enhanced ESP, for which the configuration details can be found in Figure S1 in the supplemental information and in previous studies (Jing et al. Citation2015), was tested with the undesiccated wet flue gas. Figure S2 shows the current–voltage (I-V) characteristics of the ESP with SXR. For both positive and negative polarity, the onset voltage of the ESP with SXR turned on was slightly lower than the ESP without SXR, which is consistent with the results obtained by Hogan et al. (Hogan, Lee, and Biswas Citation2004). Also, for both positive and negative polarity, the current was higher with SXR at low voltages. Interestingly, with voltages higher than 12 kV, the currents were similar regardless of the presence of SXR, which was not reported in previous studies (Hogan, Lee, and Biswas Citation2004; Jing et al. Citation2015; Kulkarni et al. Citation2002).
, , and show the particle penetration size distributions with SXR off and on using ESP voltages of 8.0 kV, 11.0 kV, and 13.5 kV, respectively. As illustrated, at each ESP voltage, the penetrations of most of the particles with diameters between 20 nm and 100 nm were lower with SXR turned on. As the voltage increased, the particle penetration was reduced for all the particles in that size range. It should be noted that the particle penetration () and capture efficiency (
) of particles with a given diameter were calculated using eq 1,
Figure 4. Particle penetration in the ESP with SXR (on and off) at different voltages: (a) +8.0 kV; (b) +11.0 kV; (c) +13.5 kV.
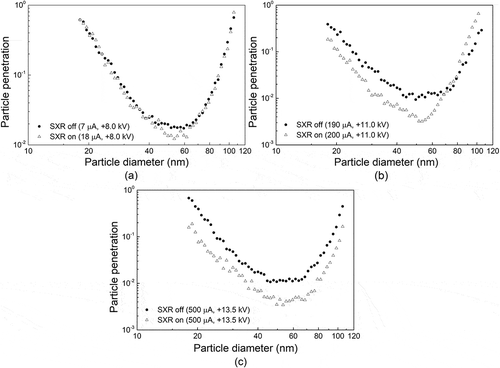
where Non(dp) and Noff(dp) are the particle number concentrations at the outlet of the ESP when ESP is turned on and off, respectively. shows that ESP with SXR on still achieved higher capture efficiency than a bare ESP, although the current was not increased at the same voltage. It was reported by Kulkarni et al. (Citation2002) that the SXR affected the ESP performance in two primary ways: ion concentration enhancement due to bipolar ions created by photoionization, and photoionization of particles. It can be speculated that when the applied voltage is high, the ion concentration increase is dominantly contributed by electron–molecule bombardment driven by the high electric field, while the ions yielded by photoionization due to SXR could be negligible. Therefore, the current might be governed by the voltage magnitude when the voltage is over a certain threshold, which is indicated by the I-V characteristics in Figure S2. Nonetheless, when particles are present, the presence of SXR could still facilitate particle charging by direct particle photoionization, which is probably the mechanism behind the increasing capture efficiency illustrated in . It also needs to be emphasized that this study used a positive corona, which allows more effective particles charging when coupled with SXR than a negative corona (Kulkarni et al. Citation2002). Based on the SXR experimental results and an approximate estimate of energy requirement of applying SXR as shown in the supplemental material, it is advised for air quality management professionals in coal-fired power plants that SXR can be utilized with ESP with careful design and operation strategy. The trade-off between energy investment and emission control efficiency should be addressed appropriately during special operation periods such as peak electricity consumption season with high emissions.
Effect of derating reheat burner on the aerosol size distribution
Flue gas processed by FGD scrubbing is typically cooled and saturated with water vapor and commonly needs to be reheated by a gas burner to avoid duct corrosion by suppressing condensation of water or other vapors and improve plume buoyancy from the stack (Rubin and Nguyen Citation1978; Sijbesma et al. Citation2008). As a side benefit, the number concentration of fine particles can be also reduced at an elevated temperature. Therefore, the operation of the reheat burner is crucial to controlling of particles in the flue gas. The effect of flue gas reheating was investigated when the reheat burner was operated at two lower ratings, that is, 0% (shutoff) and 27% of its full capacity. compares the particle size distributions when the ratings of the reheat burner were maintained at 0% and 42% (steady-state condition described before). For each rating, the size distributions measured with and without the diffusion dryer are also shown. Figure S3 in the supplemental information shows the size distributions when the rating of the reheat burner was 27%. When the reheat burner was in steady state, the mode diameters of the size distributions with and without the dryer were 38.5 and 40.0 nm, respectively, as shown in . However, when the reheat burner was turned off, the mode diameters of the size distributions with and without the dryer were both greater than the values obtained under the normal condition. These observations indicate that reducing the reheat burner rating might allow new particles to form and grow by condensation of more water vapor. In addition, the number concentrations of particles in most sizes shown in and were significantly higher when the reheat burner was turned off, which can be attributed to the possibility that more droplets were formed at lower temperature and carried over by the flue gas. A field study at a 450-MW coal-fired power plant (Sijbesma et al. Citation2008) showed that the flue gas after passing through a scrubber and before entering a reheat burner contained 11.2 vol.% H2O, 150–300 vppm NOx, and 50–100 vppm SO2. It has been reported that if the temperature of flue gas is lower than the sulfuric acid dew point, H2SO4 aerosols can be generated and can corrode the reheat burner tubes (Rubin and Nguyen Citation1978). Therefore, to extend the service life of the reheat burner and control the particle emissions, it is essential to maintain an elevated temperature in the burner to inhibit water vapor and SO2 condensation. A comparison of the size distributions of dry aerosols suggests that a higher reheat burner rating could even lead to a lower number concentration of dry aerosols and a lower mode diameter. This result also implies that there might be some volatile components in dry aerosols than can be released by the reheating.
Figure 5. Size distributions at different operating ratings of the reheat burner (both with and without dryer): (a) SMPS and (b) APS.
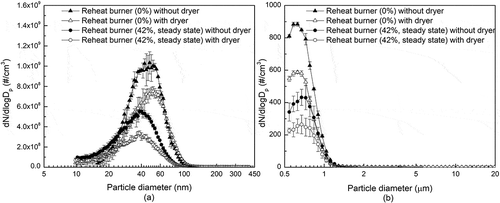
lists the total number concentrations of dry aerosols in different size ranges and mass concentrations of PM2.5 obtained by the SidePak downstream of the diffusion dryer when the reheat burner was operated at 0% and 27% ratings. The total number concentration in the range of 15.7–399.5 nm when the reheat burner was turned off was 2.5 times as high as the value when the reheat burner rating was 27%. This conclusion is consistent with a comparison of results between the 0% and 42% ratings. Nonetheless, the mass concentrations of PM2.5 were similar for both ratings. Meanwhile, the total number concentration in the range of 0.54–19.81 µm, as measured by the APS, for the 0% rating was close to that for the 27% rating as well. It appears that the mass concentration was governed by the number concentration of larger particles, because mass is proportional to the cube of particle size. Comparing the size distributions of dry aerosols for the 27% rating and 42% rating in Figure S3 and , it is interesting to find that the 27% rating can achieve size distributions of submicrometer particles as low as the 42% rating, although the 42% rating results in a much lower size distribution of particles larger than 500 nm. It can be implied that the rating increase from 27% to 42% might not be necessary to suppress submicrometer dry aerosols. Nonetheless, the chemical compositions of dry aerosols collected at different ratings of reheat burner are important for further studies.
Table 2. Comparison of number and mass concentrations of dry aerosols at different operating conditions of reheat burner.
Effect of FGD bypass on the size distribution of dry aerosols
FGD bypass is an operating mode that may temporarily occur when the boiler is in the starting phase, when FGD is malfunctioning, or when the flue gas flowing into the FGD exceeds the desulfurization capacity. Partially bypassing the FGD as long as the SO2 emission standard is achieved can minimize the FGD energy requirement (Rubin and Nguyen Citation1978). Nevertheless, while FGD removes the SOx species in the flue gas, particles may also be collected by the scrubbing liquid. It has been reported that wet FGD can remove more than 50% of fly ash particles with sizes larger than 2.5 µm (Meij and Te Winkel Citation2004). Hence, it is reasonable to expect a greater particle number concentration in the flue gas bypassing the FGD than that treated with the FGD. shows the comparison of particle size distributions from 10 nm to 20 µm between FGD bypass mode and steady-state operation mode, both of which were measured with the diffusion dryer installed in the sampling line. Compared with the steady-state result, the number concentrations of particles in diameter of from 10 nm to 120 nm and from 500 nm to 1 µm were higher when the FGD was bypassed. For the results measured by the SMPS as shown in , the mean diameter of size distribution shifted from 40.9 nm to 55.3 nm when the mode changed from steady-state operation into FGD bypass (explicit values of mean diameters are summarized in Table S1 in the supplemental material). The total number concentration of particle in diameter of from 15.7 to 399.5 nm was 1.96 × 1010 #/cm3 in FGD bypass mode, 2.2 times as high as that of steady-state operation mode. The highest concentration in FGD bypass mode measured by the APS shown in was 2.5 times as high as that of steady-state operation mode. All the findings imply that many dry particles were captured in the FGD scrubber. Furthermore, more than 50% of the particles in the size range from 50 nm to 100 nm and from 500 nm to 700 nm are trapped in the FGD during steady-state operation, as indicated by and , respectively. Two studies (Wang et al. Citation2008; Yang et al. Citation2010) showed that the particle removal efficiency of wet FGD was enhanced as particle size grew, which is consistent with the observations in . Therefore, it can be inferred that many more fine particles could exist in the flue gas when the FGD bypass is implemented, requiring careful design of targeted particle control strategy.
Effect of soot blowing on the size distribution of dry aerosols
Soot blowing is widely employed by coal-fired power plants to avoid deterioration of thermal efficiency due to soot accumulation in the coal boiler (Shi, Wang, and Liu Citation2015). Soot blowers using compressed air or steam are triggered periodically to blow away the ash attached on boiler tubes, which inevitably increases the amount of fly ash leaving the boiler. shows the size distributions measured by the SMPS and APS during soot blowing in boilers 5 and 7 compared with those obtained under the normal operating condition. During soot blowing in either boiler 5 or boiler 7, the measured size distributions showed no significant difference. Like the result of FGD bypass mode, most submicrometer particles displayed higher number concentrations at soot blowing mode. The total particle number concentrations reached 1.95 × 1010 and 1.88 × 1010 #/cm3 when conducting soot blowing in boiler 5 and boiler 7, respectively. These observations verified that soot blowing could increase the particle number concentration in the flue gas even though the gas passed through the ESP and FGD. Valmari et al. demonstrated in a field study that around 70% by weight of the total generated fly ash could be deposited on the surface of a heat exchanger between two consecutive soot blowing operations, and significant emissions would be expected during soot blowing (Valmari et al. Citation1999). This statement is consistent with the results shown in . Their study also showed that nearly all particles with diameters larger than 10 µm were deposited, but only a small portion of particles smaller than 3 µm was deposited. Nonetheless, our study indicates that the smaller particles re-entrained in the flue gas by soot blowing could double the particle load emitted to the atmosphere. On the contrary, the large particles generated by soot blowing can probably be recaptured by the ESP and FGD, which both have high removal efficiency for larger particles (Li et al. Citation2009; Wang et al. Citation2008; Yang et al. Citation2010; Zhuang et al. Citation2000).
Variations of temperature, total particle number concentration, and gas concentrations
The temperature of the flue gas duct at the sampling location was monitored by an electronic thermometer as aerosol measurements were conducted. The normalized temperatures and total number concentrations (from 15.7 to 399.5 nm) measured in every test scenario are plotted in . As shown, after transitioning from SootBlow7 to FGDbypass mode, the temperature increased because the FGD typically reduces the flue gas temperature from 150°C to 50°C (Rubin and Nguyen Citation1978; Sijbesma et al. Citation2008) due to possible condensation of sulfuric acid on passing aerosols, and the total particle number concentration increased slightly, indicating the capability of FGD to remove submicrometer particles to some small extent. When the reheat burner was turned off, the total particle number concentration remained nearly the same, but the flue gas temperature began to decline. Nonetheless, the total particle number concentration grew after the dryer was offline. The same phenomenon was also observed when comparing Reheat42 and Reheat42_NoDryer mode. These observations imply that derating the reheat burner barely affected the quantity of dry aerosols, but had a strong influence on wet particles, probably due to the enhancement of particle nucleation and condensation at lower temperature. Furthermore, as the reheat burner rating was increased to 27% of full capacity, the temperature increased as expected, but the total number concentration of dry aerosols plummeted, and stayed low after the reheat burner operated at 42% of full capacity. This variation implies that there were dry aerosols composed of highly volatile matter that probably vaporized at elevated temperature. It is proposed that the chemical composition of those volatile dry aerosols would be of great interest for further study of fly ash behavior in coal-fired power plants.
Figure 8. (a) Temperature and total number concentration for different scenarios in the order tested (normalized by the value of SootBlow5). (b) CO2 and SO2 average concentration obtained from CEMS measurements for different operating modes (normalized by the value of SootBlow5). Annotations: SootBlow5: soot blowing of boiler 5 (with dryer). SootBlow7: soot blowing of boiler 7 (with dryer). FGDbypass: bypass of FGD (with dryer). Reheat_0: reheat burner 0% (with dryer). Reheat_0_NoDryer: reheat burner 0% (without dryer). Reheat_27: reheat burner 27% (with dryer). Reheat_42: normal operation (reheat burner 42%; with dryer). Reheat_42_NoDryer: normal operation (reheat burner 42%; without dryer).
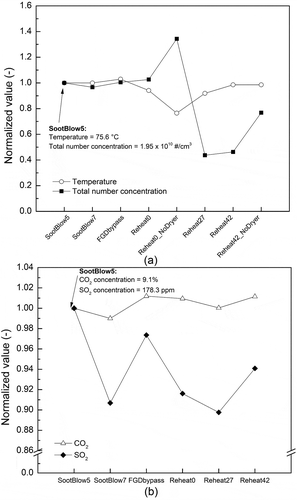
As illustrated in , between SootBlow7 and FGDbypass mode, the concentrations of both CO2 and SO2 grew considerably. The SO2 concentration increase is intuitively attributed to the bypass of the FGD, but it seems more interesting that the FGD could also capture much CO2. It has been found that suspensions of lime (Juvekar and Sharma Citation1973; Ryu, Grace, and Lim Citation2006) can absorb CO2 efficiently through carbonation. Thereafter, when the FGD bypass mode was disabled and the reheat burner was turned off, the concentrations of CO2 and SO2 both decreased, which can be attributed to the FGD capture effect and possibly to gas-to-particle conversion via nucleation and condensation at lower temperature due to the derated reheat burner. The total particle number concentration increase from FGDbypass to Reheat0 can be observed in . Thereafter, as the reheat burner returned to its normal operating condition, the CO2 and SO2 concentrations increased, possibly due to particle-to-gas conversion caused by desorption and vaporization of volatile aerosols. This speculation can also be evidenced by the decrease in total number concentration after transitioning from Reheat0 to Reheat42 as shown in .
Conclusion
The size distribution of particles from 10 nm to 20 µm in the flue gas before entering the stack was investigated under various operating modes at a 35-MW full-scale coal-fired power plant. Particles with diameters of around 40 nm showed the highest number concentration at a normal steady-state operating condition. A substantial fraction of particles was found as wet droplets. SXR was demonstrated to allow the ESP to capture more particles, and the removal efficiency increased as the applied voltage was elevated. Derating the reheat burner and bypassing the FGD resulted in more wet droplets and more larger particles, respectively. Soot blowing increased the number of submicrometer particles significantly, even after partial capture in the ESP and FGD. The flue gas temperature was slightly increased by the FGD bypass mode, which could be attributed to the absence of condensation of sulfuric acid in the FGD, and varied significantly as the rating of reheat burner changed. The variations of CO2 and SO2 emissions showed correlations with the trend of total particle number concentration, possibly due to the transitions between gas and particle phases.
This study focused on examining the effect of several common operating modes incurred in the power plant on the fine particle size distribution and main gases in flue gas. The outcomes provided robust evidence of the presence of a large number of fine particles in the flue gas. It is suggested for air quality control management professionals in coal-fired power plants that appropriate operation changes such as increasing the operation voltage of ESP, installing an SXR photoionizer on the ESP, or suppressing the inlet flow rate to amine CO2 scrubber need to be implemented during soot blowing and FGD bypass modes. The characteristic information of aerosols and main gas pollutants in the flue gas is extremely important for developing and deploying CO2 scrubbers, for which the amine emissions and operating effectiveness depend greatly on the upstream concentrations of fine particles, SO2, and so on from the power plant. Further investigations on chemical compositions of wet and dry aerosols before entering the scrubber are highly proposed.
Supplemental Material
Download PDF (354.5 KB)Supplemental data
Supplemental data for this paper can be accessed on the publisher’s website.
Additional information
Funding
Notes on contributors
Zhichao Li
Zhichao Li and Yang Wang are research assistants at the Aerosol and Air Quality Research Laboratory in the Department of Energy, Environmental & Chemical Engineering, at Washington University in St. Louis, in St. Louis, MO.
Yongqi Lu
Yongqi Lu is the head of the Applied Research Laboratory at Illinois State Geological Survey in Prairie Research Institute at the University of Illinois at Urbana–Champaign, in Champaign, IL.
Pratim Biswas
Pratim Biswas is the Lucy & Stanley Lopata Professor and Chair of the Department of Energy, Environmental and Chemical Engineering, at Washington University in St. Louis, in St. Louis, MO.
References
- Dang, Q., M. Mba Wright, and R. C. Brown. 2015. Ultra-low carbon emissions from coal-fired power plants through bio-oil co-firing and biochar sequestration. Environ. Sci. Technol. 49 (24):14688–14695. doi:10.1021/acs.est.5b03548.
- De Gouw, J., D. Parrish, G. Frost, and M. Trainer. 2014. Reduced emissions of CO2, NOx, and SO2 from US power plants owing to switch from coal to natural gas with combined cycle technology. Earth’s Future 2 (2):75–82. doi:10.1002/2013EF000196.
- Guttikunda, S. K., and P. Jawahar. 2014. Atmospheric emissions and pollution from the coal-fired thermal power plants in India. Atmos. Environ. 92:449–460. doi:10.1016/j.atmosenv.2014.04.057.
- Hogan, C. J., M.-H. Lee, and P. Biswas. 2004. Capture of viral particles in soft X-ray–Enhanced corona systems: Charge distribution and transport characteristics. Aerosol Sci. Technol. 38 (5):475–486. doi:10.1080/02786820490462183.
- Huang, C., C. Chen, L. Li, Z. Cheng, H. Wang, H. Huang, D. Streets, Y. Wang, G. Zhang, and Y. Chen. 2011. Emission inventory of anthropogenic air pollutants and VOC species in the Yangtze River Delta region, China. Atmos. Chem. Phys. 11 (9):4105–4120. doi:10.5194/acp-11-4105-2011.
- Huang, S.-H., and -C.-C. Chen. 2002. Ultrafine aerosol penetration through electrostatic precipitators. Environ. Sci. Technol. 36 (21):4625–4632. doi:10.1021/es011157+.
- Jing, H., X. Wang, W.-N. Wang, and P. Biswas. 2015. Elemental mercury oxidation in an electrostatic precipitator enhanced with in situ soft X-ray irradiation. J. Air Waste Manage. Assoc. 65 (4):455–465. doi:10.1080/10962247.2014.998352.
- Juvekar, V., and M. Sharma. 1973. Absorption of CO2 in a suspension of lime. Chem. Eng. Technol. 28 (3):825–837. doi:10.1016/0009-2509(77)80017-1.
- Kamijo, T., Y. Kajiya, T. Endo, H. Nagayasu, H. Tanaka, T. Hirata, T. Yonekawa, and T. Tsujiuchi. 2013. SO 3 impact on amine emission and emission reduction technology. Energy Procedia 37:1793–1796. doi:10.1016/j.egypro.2013.06.056.
- Khakharia, P., L. Brachert, J. Mertens, C. Anderlohr, A. Huizinga, E. S. Fernandez, B. Schallert, K. Schaber, T. J. Vlugt, and E. Goetheer. 2015. Understanding aerosol based emissions in a post combustion CO 2 capture process: Parameter testing and mechanisms. Int. J. Greenhouse Gas Control 34:63–74. doi:10.1016/j.ijggc.2015.01.001.
- Khakharia, P., L. Brachert, J. Mertens, A. Huizinga, B. Schallert, K. Schaber, T. J. Vlugt, and E. Goetheer. 2013. Investigation of aerosol based emission of MEA due to sulphuric acid aerosol and soot in a post combustion CO 2 capture process. Int. J. Greenhouse Gas Control 19:138–144. doi:10.1016/j.ijggc.2013.08.014.
- Kulkarni, P., N. Namiki, Y. Otani, and P. Biswas. 2002. Charging of particles in unipolar coronas irradiated by in-situ soft X-rays: Enhancement of capture efficiency of ultrafine particles. J. Aerosol. Sci. 33 (9):1279–1296. doi:10.1016/S0021-8502(02)00067-8.
- Li, L., J. An, M. Zhou, R. Yan, C. Huang, Q. Lu, L. Lin, Y. Wang, S. Tao, and L. Qiao. 2015a. Source apportionment of fine particles and its chemical components over the Yangtze River Delta, China during a heavy haze pollution episode. Atmos. Environ. 123:415–429. doi:10.1016/j.atmosenv.2015.06.051.
- Li, Y., A. Suriyawong, M. Daukoru, Y. Zhuang, and P. Biswas. 2009. Measurement and capture of fine and ultrafine particles from a pilot-scale pulverized coal combustor with an electrostatic precipitator. J. Air Waste Manage. Assoc. 59 (5):553. doi:10.3155/1047-3289.59.5.553.
- Li, Z., J. Jiang, Z. Ma, S. Wang, and L. Duan. 2015b. Effect of selective catalytic reduction (SCR) on fine particle emission from two coal-fired power plants in China. Atmos. Environ. 120:227–233. doi:10.1016/j.atmosenv.2015.08.046.
- Liu, F., Q. Zhang, D. Tong, B. Zheng, M. Li, H. Huo, and K. He. 2015. High-resolution inventory of technologies, activities, and emissions of coal-fired power plants in China from 1990 to 2010. Fuel Process. Technol. 15 (23):13299–13317. doi:10.5194/acp-15-13299-2015.
- Meij, R., and B. Te Winkel. 2004. The emissions and environmental impact of PM 10 and trace elements from a modern coal-fired power plant equipped with ESP and wet FGD. Fuel Process. Technol. 85 (6):641–656. doi:10.1016/j.fuproc.2003.11.012.
- Mertens, J., L. Brachert, D. Desagher, M. Thielens, P. Khakharia, E. Goetheer, and K. Schaber. 2014. ELPI+ measurements of aerosol growth in an amine absorption column. Int. J. Greenhouse Gas Control 23:44–50. doi:10.1016/j.ijggc.2014.02.002.
- Mertens, J., J. Knudsen, M.-L. Thielens, and J. Andersen. 2012. On-line monitoring and controlling emissions in amine post combustion carbon capture: A field test. Int. J. Greenhouse Gas Control 6:2–11. doi:10.1016/j.ijggc.2011.11.015.
- Mertens, J., H. Lepaumier, D. Desagher, and M.-L. Thielens. 2013. Understanding ethanolamine (MEA) and ammonia emissions from amine based post combustion carbon capture: Lessons learned from field tests. Int. J. Greenhouse Gas Control 13:72–77. doi:10.1016/j.ijggc.2012.12.013.
- Rochelle, G. T. 2009. Amine scrubbing for CO2 capture. Science 325 (5948):1652–1654. doi:10.1126/science.1176731.
- Rubin, E. S., and D. G. Nguyen. 1978. Energy requirements of a limestone FGD system. J. Air. Pollut. Control Assoc. 28 (12):1207–1212. doi:10.1080/00022470.1978.10470728.
- Ryu, H.-J., J. R. Grace, and C. J. Lim. 2006. Simultaneous CO2/SO2 capture characteristics of three limestones in a fluidized-bed reactor. Energy Fuels 20 (4):1621–1628. doi:10.1021/ef050277q.
- Schaber, K. 1995. Aerosol formation in absorption processes. Chem. Eng. Technol. 50 (8):1347–1360. doi:10.1016/0009-2509(95)98846-7.
- Shi, Y., J. Wang, and Z. Liu. 2015. On-line monitoring of ash fouling and soot-blowing optimization for convective heat exchanger in coal-fired power plant boiler. Appl. Therm. Eng. 78:39–50. doi:10.1016/j.applthermaleng.2014.12.002.
- Sijbesma, H., K. Nymeijer, R. Van Marwijk, R. Heijboer, J. Potreck, and M. Wessling. 2008. Flue gas dehydration using polymer membranes. J. Membr. Sci. 313 (1):263–276. doi:10.1016/j.memsci.2008.01.024.
- Strand, M., J. Pagels, A. Szpila, A. Gudmundsson, E. Swietlicki, M. Bohgard, and M. Sanati. 2002. Fly ash penetration through electrostatic precipitator and flue gas condenser in a 6 MW biomass fired boiler. Energy Fuels 16 (6):1499–1506. doi:10.1021/ef020076b.
- Tola, V., G. Cau, F. Ferrara, and A. Pettinau. 2016. CO2 emissions reduction from coal-fired power generation: A techno-economic comparison. J. Energy Res. Technol. 138 (6):061602. doi:10.1115/1.4034547.
- Valmari, T., T. Lind, E. Kauppinen, G. Sfiris, K. Nilsson, and W. Maenhaut. 1999. Field study on ash behavior during circulating fluidized-bed combustion of biomass. 2. Ash deposition and alkali vapor condensation. Energy Fuels 13 (2):390–395. doi:10.1021/ef9800866.
- Wang, H., Q. Song, Q. Yao, and C.-H. Chen. 2008. Experimental study on removal effect of wet flue gas desulfurization system on fine particles from a coal-fired power plant. Proc. Chin. Soc. Electr. Eng. 28 (5):1.
- Xiong, T., W. Jiang, and W. Gao. 2016. Current status and prediction of major atmospheric emissions from coal-fired power plants in Shandong Province, China. Atmos. Environ. 124 (Part A):46–52. doi:10.1016/j.atmosenv.2015.11.002.
- Xu, Y., J. Hu, Q. Ying, H. Hao, D. Wang, and H. Zhang. 2017. Current and future emissions of primary pollutants from coal-fired power plants in Shaanxi, China. Sci. Total Environ. 595:505–514. doi:10.1016/j.scitotenv.2017.03.267.
- Yang, L., J. Bao, J. Yan, J. Liu, S. Song, and F. Fan. 2010. Removal of fine particles in wet flue gas desulfurization system by heterogeneous condensation. Chem. Eng. J. 156 (1):25–32. doi:10.1016/j.cej.2009.09.026.
- Yi, H., J. Hao, L. Duan, X. Tang, P. Ning, and X. Li. 2008. Fine particle and trace element emissions from an anthracite coal-fired power plant equipped with a bag-house in China. Fuel 87 (10):2050–2057. doi:10.1016/j.fuel.2007.10.009.
- Ylätalo, S. I., and J. Hautanen. 1998. Electrostatic precipitator penetration function for pulverized coal combustion. Aerosol Sci. Technol. 29 (1):17–30. doi:10.1080/02786829808965547.
- Zhang, R., J. Jing, J. Tao, S.-C. Hsu, G. Wang, J. Cao, C. S. L. Lee, L. Zhu, Z. Chen, and Y. Zhao. 2013. Chemical characterization and source apportionment of PM 2.5 in Beijing: Seasonal perspective. Atmos. Chem. Phys. 13 (14):7053–7074. doi:10.5194/acp-13-7053-2013.
- Zhao, Y., S. Wang, L. Duan, Y. Lei, P. Cao, and J. Hao. 2008. Primary air pollutant emissions of coal-fired power plants in China: Current status and future prediction. Atmos. Environ. 42 (36):8442–8452. doi:10.1016/j.atmosenv.2008.08.021.
- Zhuang, Y., Y. J. Kim, T. G. Lee, and P. Biswas. 2000. Experimental and theoretical studies of ultra-fine particle behavior in electrostatic precipitators. J. Electrostat. 48 (3–4):245–260. doi:10.1016/s0304-3886(99)00072-8.