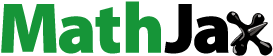
ABSTRACT
Carbon monoxide (CO) emitted from roasted coffee is a potential occupational respiratory exposure hazard to workers within the coffee industry. The current study objective was to estimate CO emission factors from commercially available roasted whole bean and ground coffee measured in loose form, not packaged, and to assess the utility of CO monitoring in nonventilated storage spaces such as within coffee roasting and packaging facilities, transport vessels, and cafés. Determinants affecting CO emissions from coffee were investigated, including form (whole bean vs. ground), roast level (light, medium, medium-dark, dark), and age (time since the package was opened). CO emission factors were estimated for roasted coffee samples from a variety of manufacturers purchased from local grocery stores and online. Emission tests were performed on 36 brands of coffee, some with more than one sample per brand and with various roast levels. Decaying source equations or smoothing functions were fitted to the CO concentration measurements. Maximum observed emission factors at the peak of the predicted concentration curve were adjusted by the time required to reach the maximum CO concentration and reported as emission factors (EFbuildup). Ground coffee had a significantly increased EFbuildup (P < 0.0001) compared with whole bean. Roast level did not significantly affect emissions for whole bean (P = 0.72) but did for ground (P < 0.001) coffee. For ground coffee, medium-dark and dark roasts had significantly higher emissions than medium and light roasts. Worst-case emission factors from commercially available whole bean and ground coffee measured in loose form, not packaged, showed that roasted coffee can rapidly emit CO. CO concentrations should be monitored in storage spaces in service and manufacturing facilities as well as transport vessels to ensure exposures do not exceed occupational exposure limits. Storage spaces may need to be ventilated to control CO concentrations to safe levels.
Implications: Emission rates of carbon monoxide (CO) from roasted coffee showed that unventilated or underventilated storage spaces should be monitored and ventilated, if necessary, to control CO concentrations to safe levels.
Introduction
Carbon monoxide (CO) is produced as a result of the coffee roasting process, and exposures have been associated with fatalities and near-fatalities in the coffee industry (Newton Citation2002; Nishimura, Abe, and Fukunaga Citation2003). Given the prolific consumption of coffee in the United States, it is expected that unventilated or underventilated storage of roasted coffee in nonindustrial sectors, such as service (e.g., coffee shops, conference centers, restaurants) and transport (e.g., cruise lines) could be of concern.
CO is a simple asphyxiant that is colorless and odorless. Health symptoms associated with CO exposures such as headache, nausea, weakness, dizziness, and confusion are nonspecific, making it difficult to create causal links without exposure measurements, carboxyhemoglobin levels, or known sources. Standard test methods use electrochemical sensors to assess the exposure hazard (National Institute for Occupational Safety and Health [NIOSH] Citation1996).
CO is produced during incomplete combustion. Common sources include welding, cooking, vehicle exhaust, cigarettes, and improperly functioning heating systems. CO is also produced during the coffee roasting process when oxygen combines with methane from natural gas–fired roasting. Gases are trapped in the pore structure of roasted coffee, which is influenced by roasting temperature and time (Schenker et al. Citation2000). CO and carbon dioxide are emitted from the roasted coffee beans over time. Degas (i.e., off-gas) rates of carbon dioxide are faster with higher temperatures and faster roasting times, and the emission rate of trapped gases is influenced by the degree of grinding (Wang and Lim Citation2014). Emissions of CO and carbon dioxide evolve more quickly when the beans are ground due to the release of trapped gases and the increased surface area of ground coffee compared with whole beans (Newton Citation2002). CO exposures in the workplace above the Occupational Safety and Health Administration (OSHA) ceiling limit of 200 parts per million (ppm) may occur when entering unventilated, roasted coffee storage spaces and when working with roasted coffee in storage containers in coffee roasting and packaging facilities (Hawley, Cox-Ganser, and Cummings Citation2017). CO air concentration in the workplace will depend on factors such as the amount of coffee stored, how it is stored (sealed vs. unsealed), room volume, air exchange rate, and generation rate.
Diffusion of gases out of roasted whole bean or ground coffee decreases as the mass trapped in the coffee decreases and the concentration gradient between the material and its surroundings decreases. The effect of packaging or containment on the emission of CO from coffee was not investigated here, but it should be considered when coffee is stored as a final packaged product or in temporary storage or transport containers in service or manufacturing facilities. When coffee is stored in a sealed or partially sealed container (e.g., bag with or without one-way valve, bins with or without lids, etc.), gases accumulate in the container void volume until a concentration equilibrium is established between the material and the void volume. Pressure inside a storage container with a tight-sealing lid may increase over time due to the release of carbon dioxide (mainly) and other gases and gaseous chemicals (e.g., CO and aroma compounds), which will create a driving force for gas emissions through leakage. When the container is opened, the concentration gradient increases by dilution of the void volume with fresh air, which reinvigorates diffusion until most of the trapped gas has escaped. Gases may also be forced out of containers because of volume displacement when roasted coffee is added to a container.
The current study objective was to estimate emission factors from commercially available whole bean and ground coffee measured in unpackaged, loose form. This study was conducted to assess CO emissions from roasted coffee as an occupational exposure agent and to highlight the need for CO monitoring in storage spaces and the coffee industry. The emission estimates produced here are worst-case estimates, as the samples were tested in loose form and at high air exchange rates, which allowed for rapid screening of samples. Determinants affecting CO emissions from coffee were investigated, including form (whole bean vs. ground), roast level (light, medium, dark), and age (time since the package was opened).
Methods
Experimental methods
Roasted coffee samples from a variety of manufacturers purchased from local grocery stores and online from Amazon.com were tested. CO emission data from these samples were acquired by placing ground, freshly ground (within 10 min of grinding), or whole bean coffee into a microchamber (M-CTE 250; Markes International, Sacramento, CA). CO concentration measurements were recorded every 10 sec for approximately 1.5 hr using a CO monitor (982 IAQ probe/Velocicalc 9555X; TSI, Shoreview, MN). The CO monitor uses an electrochemical sensor housed in a probe that was attached to the chamber exhaust using the probe calibration sleeve and a 1-inch piece of Teflon tubing. The sensor had a measurement range from 0.1 to 500 ppm and was calibrated daily using zero-grade air (NorLab, Boise, ID) and a 35-ppm CO calibration standard (NorLab) to adjust the span. Prior to commencing the emission tests, the microchamber background CO was measured and was generally below 0.1 ppm. Measurements began immediately upon placing the sample in the chamber contrary to available standard practices (American Society for Testing and Materials [ASTM] Citation2017). ASTM D7706 recommends a 30-min equilibration time prior to sampling volatile organic compound (VOC) emissions from products, but in this study the collection of the buildup portion of the decay curve was desired. The emission studies were performed using humidified air at 36.9 ± 5.6% relative humidity (RH; mean± standard deviation) and 24.3 ± 0.8 °C, measured with a Control Company 4085 hygrometer/thermometer monitor (Webster, TX).
Control of flow rate was achieved using pressure along with an in-line rotameter containing manual flow rate adjustment. Flow rates were calibrated prior to testing using a digital flowmeter (Bios DryCal Defender 530; Mesa Laboratories, Butler, NJ). The microchamber system used laboratory compressed air at 19 pounds per square inch gauge, which was humidified with a glass bottle containing 500 mL water (18.2 MOhm-cm). The test chamber had a volume of 114 mL, translating to approximately 52.6 air changes per hour (hr−1) at 100 mL/min. To achieve the desired flow rate and temperature, the Markes M-CTE 250 microchamber was operated in high-flow mode with the cooling fans on and chamber heaters set to 25 °C.
Preliminary emission testing at 40 mL/min with 2.5 and 7.5 g coffee demonstrated that results were more reliable with increased mass due to increased CO concentration achieved in the emission chamber. Preliminary emission testing was performed at several flow rates (40, 60, and 100 mL/min, using 7.5 g coffee) to determine the flow rate that provided the highest or worst-case estimate of emissions. For emissions, higher air exchange rates produce higher emissions, since the concentration gradient between the material and the chamber is maintained. Subsequent tests were performed using 7.5 ± 0.01 g coffee and 100.4 ± 1.9 mL/min air on 36 brands of coffee, some with more than one sample per brand and with various roast levels; 33 samples were originally packaged as whole bean and 31 samples were originally packaged as ground coffee (). Grind level for the “packaged as ground coffee” was assumed to be a medium grind because these coffees were intended for drip coffee makers. One coffee sample was packaged in a tin with valve on the top with the rest packaged in bags with valves. None of the whole bean samples were flavored, and six of the ground samples were flavored. The roast level recorded was based on manufacturer’s descriptions on the product packaging. Whole bean coffees were tested both as whole beans and as freshly ground beans (Cuisinart DBM-8AMZ Supreme Grind Automatic Burr Mill, Stainless Steel; Stamford, CT) using a medium grind setting. Coffees were subjected to testing immediately after opening the manufacturer’s package and resealed; sell by/best by date, serial number, and package open date were recorded. The median number of days to sell by/best by date was 196 days with a range of 4–667 days. It appeared that most coffees had sell by/best by dates of 1 yr from packaging.
Table 1. Distribution of 64 test samples packaged as whole bean or ground coffee at various roast levels.
Data analysis
CO concentration emission data were processed in SAS 9.4 and JMP 12.1 (SAS Institute, Cary, NC). Statistical significance was defined as P < 0.05. CO concentration in ppm was converted to mg/m3 using a molecular weight of 28.01 g/mol and a molar volume adjusted based on the average temperature of the trial. CO mass concentration was plotted against elapsed time to investigate the emission curve.
Emission factors were estimated based on concentration curves using ASTM D5116: Standard Guide for Small-Scale Environmental Chamber Determinations of Organic Emissions from Indoor Materials/Products (ASTM Citation2010). A nonlinear regression technique, PROC NLIN, in SAS was used to fit the CO data to the following first-order decaying source equation:
where C(t) is the CO mass concentration, mg m−3, at time t; L is the loading factor, which is the mass of material divided by the chamber volume, kg m−3; EF0 is the initial emission factor, mg kg−1 hr−1; EFss is the steady-state emission factor, mg kg−1 hr−1; k is the decay rate constant, hr−1; N is the air exchange rate, hr−1; and t is the time, hr.
The known parameters in eq 1 are CO mass concentration (C(t)), loading factor (L), and time (t). The fitted parameters of initial emission factor (EF0), decay rate constant (k), and steady-state emission factor (EFss) were all given ranges to iteratively identify solutions based on minimization of the sum of squares error. EFss was added to the decaying source equation in ASTM D5116 to account for an asymptotic approach to a nonzero steady-state value within the limits of the tested time. The ranges and step increments supplied in PROC NLIN were 0.1–150 by 10 for EF0, 0.00005–5 by 0.3 for k, and 0.2–20 by 0.5 for EFss. The first-order decaying source fit was usually best suited for whole bean coffee.
For ground coffee, the first-order decaying source solution failed to adequately predict the CO concentration data most of the time, as indicated by poor model parameter estimates and/or a poorly fitted function when plotted against actual CO concentration data. An alternative technique was chosen that allowed for smoothing of the data and estimation of an emission factor. A nonparametric smoothing function, Proc LOESS, in SAS was used with smoothing parameters ranging from 0.01 to 0.2 depending on the data.
The maximum observed concentration of CO occurred at the peak of the curve, referred to as the steady-state maximum, where the emission of CO was equal to the removal. Regardless of fitting function, maximum observed emission factors at steady-state maximum were calculated as follows:
where EFssmax is the steady-state maximum emission factor, mg kg−1 hr−1; Cmax is the maximum predicted CO concentration, mg m−3, from the fitted curve; Q is the volumetric flow rate, m3 hr−1; and m is the mass of coffee, kg.
It should be noted that this is a reduced form of the equation that uses volumetric flow rate and mass instead of air exchange rate and loading factors due to cancellation of chamber volume.
The maximum adjusted EF (EFbuildup) may be slightly higher than EFssmax depending on how fast CO accumulates in the emission chamber during the buildup portion of the concentration curve, where emission of CO is greater than removal. EFbuildup was used for all data analysis, with mean ± standard deviation presented unless otherwise noted. The time required to reach the maximum concentration enables an adjustment of the EFssmax to obtain EFbuildup using the following expression:
where EFbuildup is the adjusted steady-state maximum emission factor, mg kg−1 hr−1; EFssmax is the steady-state maximum emission factor, mg kg−1 hr−1; N is the air exchange rate, hr−1; and tmax is the time required to reach maximum predicted CO concentration, hr.
The effects of determinants of emission factors were visually represented using box plots, statistically compared using an analysis of variance (ANOVA) with post hoc testing (Student’s t for groups of two and Tukey’s test for groups of three or more), and generally summarized by effect groups. Repeatability of EFbuildup was evaluated using replicate measurements of the same sample over the course of a day. The effect of age was evaluated by comparing decay rate constants from fitting these replicate measurements conducted on the same day with a decay curve as well as testing three individual coffees up to 19 days after the bag was first opened. The effect of form (ground or whole bean) was assessed by comparing freshly ground coffee and whole bean emission factors. The effect of roast level was assessed by comparing emission factors for various roast levels by form. The effect of packaged ground or freshly ground coffee was assessed by comparing coffee that had been packaged in ground form with whole bean coffee that was freshly ground.
Results
Representative CO concentration emission curves
Ground coffee CO concentration curves () generally show higher concentrations and a smoother fit to predicted concentrations than whole bean coffee (). In , the asterisks are measured CO concentration values and the circles are predicted from the fitting function.
Repeatability and age
Whole bean EFbuildup from a single dark roast coffee was repeatable across six consecutive trials conducted on the same day, with a precision of 8%. Examining age effect, whole bean EFbuildup showed a slight decrease over time, with a decay rate constant of 0.019 hr−1 (data not shown). Packaged ground coffee EFbuildup from a single medium roast decreased over the day, with an emission decay rate of 0.224 hr−1 (). Three individual whole bean coffees were tested 7, 14, or 19 days after the bag was opened. EFbuildup decreased 49% over 7 days (from 10.8 to 5.56 mg kg−1 hr−1), 67% over 14 days (from 1.32 to 0.44 mg kg−1 hr−1), and 37% over 19 days (from 5.68 to 3.58 mg kg−1 hr−1).
Freshly ground versus whole bean
Box plots of coffee form show a large variability (tall box) among freshly ground coffees but relatively low variability (short box) among whole bean coffees (). Freshly ground coffee had a significantly increased EFbuildup (P < 0.0001; n = 31) compared with whole bean (). The median difference between freshly ground and whole bean EFbuildup was 52.5 mg kg−1 hr−1 (mean: 61.6 mg kg−1 hr−1; range: 5.2–137 mg kg−1 hr−1).
Roast level
Box plots of roast level by form show a large variability among roast levels for ground coffee but relatively low variability among roast levels for whole bean coffee (). Roast level significantly affected emissions for ground coffee (P < 0.001; ), and emissions were significantly higher for medium-dark and dark roasts than medium and light roasts. Roast level did not significantly affect emissions for whole bean coffee (P = 0.72; ).
Packaged ground versus freshly ground
Box plots for coffee originally packaged in ground form (n = 34) and freshly ground coffee (n = 33) show a large variability between samples within a given group, with three outliers (represented as dots) observed for packaged ground (). Freshly ground coffee mean EFbuildup (69.5 ± 33.5 mg kg−1 hr−1) was more than double that of coffee that was packaged in ground form (30.9 ± 53.6 mg kg−1 hr−1); the difference between the means of these groups was statistically significant (P < 0.001).
Discussion
In this study, CO emission factors for whole bean and ground coffee were estimated to highlight the importance of monitoring CO in roasted coffee storage spaces in service and manufacturing facilities. The emission curves generally took three forms, with two of them being the most common. The first curve form was smooth, increased quickly, peaked, and decayed to an asymptote different from zero (see eq 1 steady-state emission factor, EFss) (); this curve was common and generally observed for ground coffee. The second curve form was lower in magnitude than the first form, generally decayed to zero, and had multiple peaks (); this curve was common and generally observed for whole bean coffee. The third curve form was relatively smooth and increased to an asymptotic value with no observable decay period during the time tested (not shown); this curve was not common and occurred due to the equilibrium established between emission and removal of CO within the chamber. Ground coffee produced smoother concentration curves () and higher CO emissions () than whole bean coffee, which often showed spikes in the concentration profile (). These spikes may have been due to an opening of the surface pore space allowing for the occluded CO to be released.
Higher CO emissions from ground coffee are a result of increased surface area available for diffusion and the release of trapped gas from the pore structure of the whole bean. The surface area of a whole bean, based on ellipsoid geometry and available length, width, and depth radii, is estimated to be 204 mm2 (Severa, Buchar, and Nedomová Citation2012). In a study of carbon dioxide degassing from roasted coffee, medium ground coffee was found to have an average particle radius of 467 µm (Wang and Lim Citation2014). An approximate surface area of a medium ground coffee particle at 0.467 mm, assuming a sphere, is 2.74 mm2. Assuming an average mass of 0.14 g per roasted bean (Severa, Buchar, and Nedomová Citation2012) and bulk density of coffee of 0.5 g/mL (Jokanović et al. Citation2012), approximately 700 particles per bean would be produced during a medium grind, with a total surface area available for diffusion of 1900 mm2. This equates to a 9-fold increase in available surface area for ground compared with whole bean coffee.
Ground coffee CO emission factors generally increased with increasing roast level (). The same relationship did not hold for whole bean coffee, presumably due to trapped gases in the pore structure limiting emissions (). Freshly ground coffee had higher emission factors than packaged ground coffee (). Wang and Lim (Citation2014) found that roasting at higher temperatures and for shorter times increased the whole bean coffee degassing rates for CO2; however, their tests were conducted over hours to days, allowing for slow emissions to occur (Wang and Lim Citation2014). Their tests were also conducted with no air flow (i.e., static) changing the evolution dynamics of emissions; as the concentration in the vessel increases, the concentration gradient decreases, limiting diffusion into the vessel headspace. In a dynamic test atmosphere, such as that used in the current study, the vessel is purged with clean air, removing the emitted gas and maintaining a sufficient concentration gradient to enable uninhibited or accelerated diffusion.
Average CO emissions measured from roaster exhaust stacks have been reported at 49 mg CO/kg roasted coffee from continuous roasters controlled with thermal oxidizers, and at 740 mg/kg from uncontrolled continuous roasters (U.S. Environmental Protection Agency [EPA] Citation1995). Although these emissions are not directly comparable to the emission factors produced in this study, it demonstrates that a large amount of CO is produced as a result of combustion of natural gas.
Occupational CO exposures may occur when entering unventilated or underventilated roasted coffee storage spaces and when working with roasted coffee in storage containers in coffee roasting and packaging facilities (Hawley, Cox-Ganser, and Cummings Citation2017). Area CO concentrations at the face of, or in storage bins of, roasted whole bean coffee have been measured up to 223 ppm (NIOSH Citation2017a), 410 ppm (NIOSH Citation2017b), and 473 ppm (NIOSH Citation2017c). Assuming 10 kg of roasted whole bean coffee with an average emission factor of 7.8 mg−1 kg−1 hr−1 are stored in a 2-m3 container with a relatively low air exchange rate of 0.1 hr−1, an estimated average CO air concentration would be 340 ppm, which is above the NIOSH ceiling limit of 200 ppm and compares well with the range measured in the field. Assuming a facility with a volume of 235 m3 stored 7000 kg of roasted whole bean coffee, an average CO air concentration would be 203 ppm at an air exchange rate of 1.0 hr−1. This assumes a well-mixed room volume, which is typically not the case. The probability of overexposure to CO will depend on a number of factors, including amount of roasted coffee stored, how it is stored, whether it is ground or whole bean, the room or container volume, mixing effects, air exchange rate, proximity of the employee to the source, and duration spent next to the source. Although hypothetical scenarios are useful for gauging the extent of a potential risk, monitoring CO concentrations in places where roasted coffee beans are stored is recommended to ensure safe levels exist.
Conclusion
Worst-case emission factors from commercially available roasted whole bean and ground coffee measured in unpackaged, loose form showed that roasted coffee can rapidly emit CO. Emission factors were higher for ground than whole bean coffee. Darker roasts emitted more CO than lighter roasts when the coffee was ground. CO concentrations should be monitored in storage spaces in service and coffee manufacturing facilities as well as transport vessels to ensure exposures do not exceed occupational exposure limits. Storage spaces may need to be ventilated to control CO concentrations to safe levels.
Acknowledgment
The authors would like to thank Leonard Zwack and Lee Greenawald for reviewing this paper. This publication is sponsored by the Sampling and Laboratory Analysis Committee of the American Industrial Hygiene Association.
The findings and conclusions in this report are those of the authors and do not necessarily represent the views of the National Institute for Occupational Safety and Health (NIOSH). Mention of any company or product does not constitute endorsement by NIOSH. In addition, citations to Web sites external to NIOSH do not constitute NIOSH endorsement of the sponsoring organizations or their programs or products. Furthermore, NIOSH is not responsible for the content of these Web sites.
Additional information
Notes on contributors
Ryan F. LeBouf
Ryan F. LeBouf and Michael Aldridge are researchers in the Field Studies Branch, Respiratory Health Division at the National Institute for Occupational Safety and Health, Morgantown, WV.
References
- ASTM. 2010. D5116: Standard Guide for Small-Scale Environmental Chamber Determinations of Organic Emissions from Indoor Materials/Products. West Conshohocken, PA: ASTM International.
- ASTM. 2017. D7706: Standard pracdtice for rapid screening of VOC emissions from products using micro-scale chambers. West Conshohocken, PA: ASTM International.
- EPA. 1995. Emission Factor Documentation for AP-42, Section 9.13.2: Coffee Roasting, Final Report; EPA Contract 68-D2-0159. https://www3.epa.gov/ttnchie1/ap42/ch09/bgdocs/b9s13-2.pdf (accessed June 20, 2018).
- Hawley, B., J. Cox-Ganser, and K. Cummings. 2017. Carbon Monoxide Exposure in Workplaces, Including Coffee Processing Facilities. Am. J Respir. Crit. Care Med. 196 (8):1080–1081. doi:10.1164/rccm.201703-0513LE.
- Jokanović, M. R., N. R. Džinić, B. R. Cvetković, S. Grujić, and B. Odžaković. 2012. Changes of physical properties of coffee beans during roasting. APTEFF 43:21–31. doi:10.2298/APT1243021J.
- Newton, J. 2002. Carbon Monoxide Exposure from Coffee Roasting. Appl. Occup. Environ. Hyg. 17 (9):600–602. doi:10.1080/10473220290095899.
- NIOSH. 1996. NMAM 6604: Carbon Monoxide. https://www.cdc.gov/niosh/docs/2014-151/pdfs/methods/6604.pdf (June 20, 2018).
- NIOSH. 2017a. Health hazard evaluation report: Evaluation of exposures and respiratory health at a coffee processing facility. By Fechter-Leggett ED, Boylstein RJ, Stanton ML. Morgantown, WV: US Department of Health and Human Services, Centers for Disease Control and Prevention, National Institute for Occupational Safety and Health, NIOSH HHE Report No. 2015-0147-3266.
- NIOSH. 2017b. Health hazard evaluation report: Evaluation of exposures and respiratory health at a coffee roasting and packaging facility; By LeBouf RF, Martin SBJ, Mugford C, Stanton ML, Bailey RL. Morgantown, WV: US Department of Health and Human Services, Centers for Disease Control and Prevention, National Institute for Occupational Safety and Health, NIOSH HHE Report No. 2015-0082-3287.
- NIOSH. 2017c. Health hazard evaluation report: Evaluation of exposures and respiratory health at a coffee roasting and packaging facility and attached retail café; By LeBouf RF, Martin SBJ, Stanton ML, Mugford C, Bailey RL. Morgantown, WV: US Department of Health and Human Services, Centers for Disease Control and Prevention, National Institute for Occupational Safety and Health, NIOSH HHE Report No. 2016-0003-3299.
- Nishimura, F., S. Abe, and T. Fukunaga. 2003. Carbon monoxide poisoning from industrial coffee extraction. JAMA 290 (3):334. doi:10.1001/jama.290.3.334.
- Schenker, S., S. Handschin, B. Frey, R. Perren, and F. Escher. 2000. Pore Structure of Coffee Beans Affected by Roasting Conditions. J. Food Sci. 65 (3):452–457. doi:10.1111/jfds.2000.65.issue-3.
- Severa, L., J. Buchar, and Š. Nedomová. 2012. Shape and Size Variability of Roasted Arabica Coffee Beans. Int. J. Food Pro. 15 (2):426–437. doi:10.1080/10942912.2010.487967.
- Wang, X., and L.-T. Lim. 2014. Effect of roasting conditions on carbon dioxide degassing behavior in coffee. Food Res. Int. 61:144–151. doi:10.1016/j.foodres.2014.01.027.