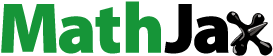
ABSTRACT
In recent years, nanotechnology has emerged as cutting-edge technology with multifarious applications in a wide array of fields. Green synthesis of iron nanoparticles (FeNP) are an upcoming cost effective and eco-friendly technique and recently gained significant importance. In the present study, green FeNPs were prepared using leaf litter which is one of the major seasonal waste contributors in urban built-up areas. Shedding trees during winter months (January – March) were selected. Most abundant trees were Pongamia pinnata (Indian beech), Morus alba (mulberry), Prosopis juliflora (mesquite) and Kigelia africana (sausage tree). Synthesized FeNPs were further used for degrading two commercial dyes, eosin yellow and fuchsin basic, via Fenton’s mechanism. The study showed that the prepared nanoparticles were of iron oxides, but also reported presence of polyphenols as a capping agent. Dye degradation efficiency of nanoparticles synthesized by P. pinnata leaf litter was recorded to be highest, whereas the efficiency of nanoparticles synthesized by K. africana leaf litter was lowest. Chances of iron leaching during dye degradation process was also tested and observed that Fe was present in treated water below the standard guidelines. Thus, FeNPs can serve as a low-cost solution to remediate water pollutants with a green approach. Implications: Nanoparticles prepared in the study were showed as a promising adsorbent and demonstrating high surface area and well-developed porosity. The prepared adsorbent will have a great impact on wastewater treatment technology and possible applications at a large scale. There are several applications of nanoparticles in pollution remediation and at the same time it can solve solid waste issues as it required to prepare nanoparticles. One of the major applications at policy level can be water pollution remediation which is urgently needed.
Introduction
Nanoparticles (NPs) in general refer to particles having internal structural measurement or external dimensions within the size range of a few nanometers, preferably up to 100 nm size as shown in . According to the European Committee for Standardization, nanomaterials are defined as the materials with any external dimension at the nanoscale, or that possess nanoscale internal or surface structures (Subbenaik Citation2016). Over the years, nanoparticles have been exploited in all fields of science and to date have been extracted from various sources through biological, physical, or chemical processes. NPs are made from different elements like iron, gold, silver, copper, zinc, and many more. Most of the synthetic methods are potentially hazardous due to the release of noxious chemicals and require costly and high-energy (pressure and temperature) based instruments. These constraints are encouraging exploration of a new, low-cost and eco-friendly method based on various biological entities like microorganisms (bacteria, yeast, and fungi) or plant species to promote sustainable development. Synthesis of green nanoparticles using leaf extracts has certain advantages over other biological organisms as plants certainly produce more stable metal nanoparticles and have proved to be the best alternative for fast and large-scale biosynthesis compared to microorganisms.
Figure 1. Comparison of plant extracts used for nanoparticles synthesis (source: Saif et al., Citation2016).
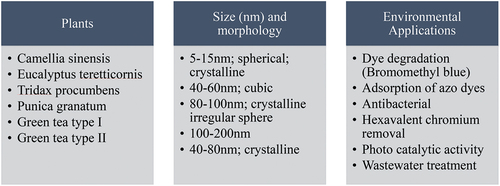
Plant extracts contains abundant chemical constituents such as phenols, reducing sugars, ascorbic acids which are responsible for the bioreduction of metal ions and the stabilization of nanoparticles (Perde-Schrepler et al., Citation2016). These extracts are abundant in antioxidants and polyphenols (e.g. eucalyptus leaves) which act both as a capping and reducing agent (Wang et al., Citation2014). Various studies were also conducted regarding the application of Fe nanoparticles and Fe/Pb bimetallic nanoparticles using green tea extract for the reduction or degradation of chlorinated organic matter (Smuleac et al. Citation2011). Nanoparticles synthesized from green tea extracts were studied for the degradation of malachite green dye (Badmapriya and Asharani Citation2016). Devatha, Thalla, and Katte (Citation2016), reported one of the rare studies where removal of COD, total nitrate, and phosphates occurred simultaneously from wastewater using FeNPs synthesized from various leaf extracts. Iron nanoparticles can be readily prepared against aggregation using plant extracts (polyphenols and caffeine) as intrinsic reductants and dispersants (Ouyang et al. Citation2019; Zhu et al. Citation2018).
In the winter season, the air quality of Northern India, especially in Delhi (national capital region) begins to deteriorate. A thick blanket of smog engulfs the region, reducing the visibility and causing health hazards especially to the sensitive groups, i.e. elderly and children. The Air Quality Index (AQI) deteriorates very fast and the value ranges between 150 and 500. The major reason behind this problem is stubble burning (which is generally practiced in other northern states of India like Uttar Pradesh, Punjab, and Haryana) and also local burning of leaf litter (Choudhry, Misra, and Tripathi Citation2012). In many places, to manage these dry leaves, biomass burning is most frequently practiced. Residents in many parts of the city have been complaining about the deteriorating air quality resulting from the burning of biomass (especially leaves). Hence, it will be a novel idea to use this waste as a substrate for synthesizing nanoparticles, which in turn can be used for degradation of dyes present in polluted water. Thus, nanoparticles prepared from leaf litter can serve the dual purpose of waste management by treating both solid and liquid waste. To address this issue, the undertaken study aims to prepare iron nanoparticles using leaf litter waste and further monitored the efficiency of these nanoparticles in follow-up dye degradation process.
Material and Method
Site selection and plant samples
Two sites namely, the institutional area of the University of Delhi and the densely vegetated ridge area were selected for the collection of different samples as both the sites have rich diversity of vegetation. Most abundant and easy availability of trees with maximum litter fall during winter months were the major criteria for the sample selection. The North Campus of University of Delhi is rich in vegetation and most of the built-up area has established greenery. Higher vegetation accounts for higher litter fall which is generally dumped within the University nursery.
Most abundant species found in these two sites were Pongamia pinnata (Indian beech), Morus alba (mulberry), and Kigelia africana (sausage tree). Apart from these, leaf litter from Prosopis juliflora (mesquite), a wild growing invasive species, was also considered for the sampling. All the samples were processed and used to synthesize iron nanoparticles.
Waste processing and preparation of leaf litter extract
All the leaves collected were sorted individually and weighed after which they were washed thoroughly in tap water to remove dust particles, followed by sun drying and then placed in microwave oven for a period of 24–48 hr to remove all the moisture. The dried leaves were then crushed to fine powder and sieved through a sieve of size 0.25 mm or 60 BSS. The method used by Shahwan et al. (Citation2011) was adopted to prepare leaf litter extract by heating 60.0 g of the dried, crushed powder in 1.0 L of distilled water until the color changes to pale yellow. The resulting leaf litter extract was cooled for approximately an hour and vacuum filtered.
Preparation of FeNPs using leaf litter extract
A solution of 0.10 M ferrous chloride tetrahydrate (FeCl2.4 H2O) was prepared and added to the leaf litter extract in a ratio of 2:3. An intense black precipitate was observed on mixing. A pH of 6 was maintained by adding a solution of 1.0 M sodium hydroxide (NaOH). Iron nanoparticles thus prepared were separated by evaporating the water from the iron solution on a hot plate followed by drying as shown in (Shahwan et al. Citation2011).
Identification and characterization of FeNPs
The synthesized Fe-NPs were characterized using FTIR, TEM, XRD, and SEM-EDX to analyze the properties of FeNPs.
Fourier Transform Infrared Spectroscopy (FTIR)
On model of Shimadzu, Broker & Perkin Elmer, FeNP sample was exposed to different waves of infrared light ranging from 400 cm−1 to 4000 cm−1 and the instrument quantifies the wavelength to be absorbed. Prior to analysis, the powdered nanoparticles were ground with potassium bromide (KBr) at 1:100 ratio and a thin film of the mixed powder was prepared.
Surface Electron Microscopy– Energy DispersiveX-Ray Analyser (SEM-EDX)
Studies were also conducted using SEM instrument JEOL, USA at fixed electron beam energy of 30 kV and at a working distance of 11 mm. with an acquisition time of 100 sec. Before analysis, FeNPs were washed with ethanol to remove the attached impurities, if any. Samples were dehydrated through series of ethanol concentrations. Further, to minimize buildup charge on the samples from exposure to the SEM electron beam, samples were coated with (10–15 nm) layer of high purity gold prior to analyses. To confirm the elemental content in particles EDX study was carried out.
Transmission Electron Microscopy (TEM)
TEM images were recorded on Delong low voltage electron microscope operated at an accelerating voltage of 5 kV. For sample preparation, a diluted solution of the FeNPs was prepared in ethanol and sonicated for few minutes. Samples were carbon coated and placed on copper grids by dropping the solution of FeNPs.
X-Ray Powder Diffraction (XRD)
XRD was acquired from Bruker D8-Advance Eco powder X-ray diffractometer with Cu-Kα radiation (λ = 1.5418 Å) with 2 θ range of 10–80° at 30 Kv and 40 mA.
Efficiency of Nanoparticles in Dye Degradation (Efficiency and Fenton mechanism)
Dye Selection
Efficiency of prepared nanoparticles was tested on two commercial dyes namely, an eosin yellow which is acidic in nature with a chemical formula C20H6Br4Na2O5 and a fuchsin basic which is basic in nature has a chemical formula C20H19N3·HCl (). These dyes are used intensively in several industries like textile, printing ink, hair dye products and are reported to be carcinogenic in nature, and are a major source of water pollution. Therefore, with an objective to reduce water pollution and to treat waste out of waste, degradation of these dyes using iron nanoparticles was carried out.
Results and discussion
Survey
Based on a random survey conducted in different areas of the university campus majorly in the university nursery and in the vegetated ridge area, the results showed that most of the trees were common in both the places i.e. ridge and within the university campus, which became the substrate of our study. Green waste (mainly litter fall) from all the departments and colleges of north campus of the university was regularly collected and dumped in one corner of the nursery for collection. Northern ridge is a biodiversity rich zone with diverse flora and fauna popularly known as the “Lungs of Delhi”. The ridge is dominated by an invasive species Prosopis Juliflora which alters the growth of other native species. Samples were collected from all the sites and the composition of leaf litter is described in .
Data collected from both sites depicted that Kigelia africana (sausage tree), Morus alba (mulberry), and Pongamia pinnata (Indian beech) were the most abundant species with maximum litter fall during sampling period. It was also observed that Prosopis juliflora (mesquite) is a wild growing invasive species within ridge area and capable of forming an evergreen forest with its persistent foliage when all other plants are devoid of leaves. Due to its aggressive invasion dominance, to find out its alternative role in bioremediation process leaf samples from this tree were also selected for the preparation of FeNPs. Tea plant (Camellia sinensis) was used as reference to compare the efficiency of these substrates as FeNPs. illustrates leaf samples used in the study with the final yield of FeNPs obtained from 100 mL of sample extract.
Table 1. Final yield of nanoparticles from different plant samples.
FTIR
FTIR spectroscopy is applied to find out information about the different functional groups from the peak positions in the spectrum. Information about capping and stabilization of the nanoparticles may also be inferred from this analysis. FTIR spectra of sample revealed peaks in range of 400–600 cm−1. These peaks are attributed to iron oxide bonds present at the surface of iron nanoparticles. Peaks in the region 400–450 cm−1 and around 600 cm−1 comprehend for vibrational transition of Fe2O3 (Bell, Roush, and Morris Citation1995). A very strong peak was observed around 1,065/cm relates to the stretching vibrations of C-O-C bond. (Shahwan et al. Citation2011) The features around 1,400/cm correspond to the in plane bending vibrations of – OH phenols. Moreover, the peak around 1,600 cm−1 can be attributed to stretching in polyphenols. The study confirms the presence of iron oxide and polyphenols in the prepared nanoparticles using different waste materials. depicts the IR spectra for Pongamia pinnata, similar results were also obtained for other substrates as well.
SEM Analysis
SEM analysis was also done to configure the structure of FeNPs formed by different leaf extract. SEM images of FeNPs showed presence of some large particles which can be attributed by overlapping of smaller particles. It was observed all FeNPs showed amorphous structure as shown in .
EDX Analysis
The EDX analysis was also performed on the prepared nanoparticles and confirmed that the nanoparticles were mainly constituted of iron with some amount of carbon, oxygen (originating from the polyphenols of the extract) and chlorine (originating from the iron chloride used to produce the NPs) present in the background. The study confirmed presence of Fe and O within FeNPs as shown in . The K-α line at 6.37 keV indicates the presence of elemental iron (Vasantharaj et al. Citation2019) and the lines at 0.30 keV and 0.80 keV correspond to C and O atoms, respectively. The elemental analysis as confirmed by EDX were : 37.71% C, 39.06% O, 22.12% Fe, and 1.11% Cl.
XRD Analysis
Analysis through X-ray diffraction was carried out to understand and to confirm the crystalline nature of particles. A comparison of the XRD spectrum with the standard Tea plant (Camellia sinensis) confirmed the formation of the types of iron oxides. XRD analysis for Pongamia pinnata showed diffraction peaks at 2 θ at 33.09, 43.48 depicting the formation of Fe2O3 as shown in . Fe3O4 were depicted at 2 θ = 36.82, corresponding to 311 plane. Similar results were found for other substrates as well. Nurmi et al. (Citation2005) already reported formation of different iron oxides in nanoparticles due to aerial oxidation.
TEM
In the TEM studies, it was found that the FeNPs prepared from the leaf litter of Pongamia pinnata had attained the size in the range of 50–70 nm, which is even supported by the work done by Rajeshkumar (Citation2016), in which the size of silver nanoparticles prepared from the fresh bark extract of Indian beech was reported to be in the range of 5–55 nm. TEM analysis displayed that Morus alba (mulberry) had particle size around 70–90 nm. Substantiated with the report by Poguberović et al. (Citation2016) where mulberry nanoparticles were found spherical and nonagglomerated nZVIs with size varies between 30 and 50 nm. Machado et al. (Citation2013) also reported synthesis of pomegranate and mulberry leaf extracts with similar size. Kigelia africana (sausage tree) had size in the range of 150–200 nm. It has been reported that particles with smaller size have higher efficiency. Leaf extracts act as both dispersive and capping agents, thus minimizing the nanoparticle’s oxidation agglomeration (Nadagouda et al. Citation2010), indicating that in a synthesized material, nanoparticles of different sizes are present, as shown in .
Mechanism of dye degradation
NPs act as a catalyst for Fenton-like mechanism where Fe-NPs react with H₂O₂ to yield OH∙ radical which cleaves the chromophore of dye. Various studies were conducted regarding the application of Fe nanoparticles using Oolong tea extract further employed to degrade malachite green (MG). OT-Fe NP proved to be efficient in the degradation of MG, resulting in 75.5% of MG (50 mg/L) being removed (Huang et al., Citation2013). Devatha, Thalla, and Katte (Citation2016), reported one of the rare studies where removal of COD, total nitrate and phosphates occurred simultaneously from wastewater using synthesized FeNPs from various leaf extract.
Oxidative degradation mechanism of dye (D) is proposed by Kuang et. al., Citation2013 for green tea synthesized iron nanoparticles which is described in EquationEquations (1)(1)
(1) and (Equation2
(2)
(2) ) below:
(i) Adsorption process:
Fe2+ and Fe3+ leach from Fe0 and iron oxides on the surface of FeNPs, as shown in EquationEquations (3)(3)
(3) and (Equation4
(4)
(4) ), and this process accelerates the decomposition of H2O2 and generates highly oxidative OH· radicals when Fe2+ was oxidized by H2O2 into Fe3+ EquationEquation (5)
(5)
(5)
(ii) The process of generating hydroxyl radicals’ species:
In the meantime, generated Fe2+ and Fe3+ in the solution will react with H2O and yield oxyhydroxide EquationEquation (6)(6)
(6) , which can also adsorb D. Furthermore, Fe3+ on the surface of FeNPs was converted into Fe2+ and HO2· and the generated HO2· possibly further react with Fe3+ and favored the decomposition of H2O2.
(iii) Hydroxyl radicals’ species attack the D on the surface of FeNPs:
The generation of radical species rapidly reacts with the adsorbed D and also attacks D, resulting in mineralization of some part of D on surface of FeNPs into CO2 and H2O, which is also involved in removal of COD (chemical oxygen demand).
The sustainable mechanism behind degradation of dye which is simple and economical depending on the nature of catalyst which is described below (Huang et. al., Citation2013):
Degradation of dye samples was observed by measuring the absorbance in UV spectroscopy at 545 nm and 518 nm for fuchsin basic and eosin yellow, respectively. Change in color from pink of fuchsin basic and orange of eosin yellow to pale yellow on treatment with FeNPs.
Yan et. al., Citation2013 and Wu et al., Citation2021 conducted similar study and found that removal efficiency of MTX by adding H2O2 alone was observed to be poor at just 11.3% and the addition of FeNPs alone increased the removal efficiency to 41.8% due to adsorption.
As per the study by Xiao et al. (Citation2020), the surface of FeNPs made from green tea is negatively charged, so positive cationic dyes are easily attracted to adsorb it. Firstly, these dye molecules adsorb on the reactive sites on surface of FeNPs-GT containing iron oxide and tea extract. It has been reported that the layer of organic compounds that surrounds FeNP has influence on the pollutants. The FeNPs synthesized under different atmosphere (nitrogen or oxygen) have different removal efficiency on dyes (Lin et. al., Citation2020). The formation and properties of iron oxide capped on the surface of iron nanoparticles affect the interaction with dyes. However, in the presence of oxygen, iron nanoparticles can still achieve a good dye removal effect. Secondly, Fe0 reacted with H2O and lost electrons, which were used by H+ to generate active hydrogen with strong reducibility.
In summary, the possible removal mechanism for fuchsin basic and eosin yellow can be adsorption and reduction process: adsorbed on iron oxide and masking agent, and reduced by zero-valent iron (Xiao et al. Citation2020).
Efficiency of Nanoparticles
Efficiency of synthesized nanoparticles was studied by plotting standard curve to understand the rate of degradation and decolorization of selected dyes (eosin yellow and fuchsin basic). Efficiency of different nanoparticles showed that Pongamia pinnata nanoparticles had highest efficiency in comparison to other substrates. Initial rate of degradation was comparatively slow in the range of 65–76% reaching more than 90% at the end of 20 min, followed by Morus alba which had initial rate of 70% reaching 86% in 20 min. Prospis juliflora showed good performance with 66% initial degradation reaching 85% at 20 min. This showed it can be used as a bioremediator to treat dyes, an alternative option for its economical use being an aggressive invasive species. Kigelia africana showed the least performance with initial rate of degradation 50% reaching 70% at the end of 20 min.
The difference in performance could be due to presence of different particle size of nanoparticles and may be due to varying antioxidant potential of different extracts of leaves as well. A study conducted by Sajid et al. (Citation2012) showed that Pongamia pinnata contains high levels of total phenolics and total flavonoids. Similarly, Morus alba has also been reported with high levels of antioxidants thereby making it a good degrading agent. Schauermann et al. (Citation2012) has also reported that iron oxides nanoparticles act as a better catalyst with the reduction in the particle size.
Likewise, nanoparticle efficiency trend was also obtained for fuchsin basic dye. The degradation efficiency was more of Pongamia pinnata with initial rate of 75% reaching 96% at the end of 20 min, whereas Morus alba and Prosopis julifera showing comparatively lower efficiency of 70 and 66% in first five minutes to approximately 85% in 20 min. Green production of nZVI has been considered as a promising alternative for chemical and physical methods, due to its advantages of a simple and cost-effective synthesis, biodegradable materials instead of toxic reagents (e.g. borohydride) and less agglomeration of nanoparticles (Ebrahiminezhad et al. Citation2018). For example, green synthesis of FeNPs by Euphorbia cochinchensis leaf as a heterogeneous catalyst in Fenton-like system, has been applied for the removal of 2,4-dichlorophenol (Gan et. al., Citation2018). Throughout the experiment, the pH of eosin yellow dye was around 7 and that of fuchsin basic was around 5.
Out of the selected two dyes, it was observed that the nanoparticles degraded fuchsin basic more than eosin yellow. Efficiency analysis of different nanoparticles for both the dyes was compared on their performance as depicted below, .
Atomic Absorption Spectroscopy
Atomic absorption spectroscopy was done for all the degraded leftover dye samples to detect the leaching of iron, if any, from the nanoparticles. To analyze samples for the same, all 60 samples were taken as shown in . It is reported that at low concentrations, iron plays an important role in metabolic and fermentation processes, as an enzyme activator, stabilizer, and functional component of proteins. Above trace levels, however, iron has other roles. It has been stated that iron, has fairly narrow “concentration window” between the essential and toxic levels. Iron is a moderately toxic element when compared with other transition metals. However, the toxic doses of iron and its compounds can lead to serious problems, including depression, rapid and shallow respiration, coma, convulsions, and cardiac arrest. Thus, appropriate knowledge of the iron content is highly desirable. So, the toxicity of this element demands a fast and accurate method for its determination in water (Steponeniene, Tautkus, and Kazlauskas Citation2003).
It was observed that all the degraded dye solution had iron concentration around 0.5 ppm only. However, the maximum permissible limit of Fe is 100 ppm. Hence it showed that there is no leaching of iron from the nanoparticles into the dye solution. This further proves that iron nanoparticles for degrading the dye solution is an environmentally safe method. We can further conclude that synthesis of iron nanoparticles and its use for cleaning the water pollution created by dye solutions is highly recommended.
Conclusion
Using a green chemical approach, we have developed a fast, eco-friendly and convenient method for the synthesis of iron nanoparticles using leaf litter extract from different locally abundant trees and the nanoparticles were found to be in the diameter range of 40–200 nm. No chemical reagent or surfactant template was required in this method, which consequently enables the bioprocess with the advantage of being environment friendly. Color change occurs due to surface plasmon resonance during the reaction with the ingredients present in the plant leaves extract results in the formation of iron nanoparticles which was confirmed by XRD, EDX, and SEM.
Acknowledgment
The authors deeply acknowledge the University Grant Commission (UGC) and Department of Environmental Studies for providing financial support for the study. Also, for the advance instrumentation analysis the authors show deep gratitude to the University Science Instrumentation Centre, University of Delhi.
Disclosure statement
No potential conflict of interest was reported by the authors.
Data availability statement
The authors confirm that the data supporting the findings of this study are available within the article and its supplementary materials.
Additional information
Funding
Notes on contributors
Reena Jain
Reena Jain, an associate professor in the Department of Chemistry Hindu College, University of Delhi has taught Inorganic Chemistry for over 24 years and environmental chemistry to master’s degree students at the Department of Environmental Studies, University of Delhi for the past 10 years. She has a Ph.D. from the Chemistry Department, Delhi University and the Prof. K.N. Johri Memorial Gold Medal from the University of Delhi. Her research interest is primarily focused on organometallic chemistry, green chemistry, nanochemistry and environmental chemistry. She has almost a dozen of papers to her credit in renowned national and international journals, has authored five books in chemistry for the senior secondary students, edited a book for Indian adaptation, and developed a lot of e-material. Her contributions in several reputed projects include, National Science Digital Library – a project of NISCAIR, CSIR, SAKSHAT, i-CBSE, and UDAAN. She has given talks in her field of inorganic chemistry and environment chemistry at more than 30 workshops, conferences, and faculty development programs, presented papers in several iational and international conferences, and has organized more than 15 workshops/conferences.
Guncha Sharma
Guncha Sharma, Ph.D. research scholar in the Department of Environmental Studies, University of Delhi graduated from Kirori mal College, University of Delhi and postgrad. from Gautam Buddha University. During her master's she was conferred with the Vice Chancellor Gold medal by Gautam Buddha University.
Shailender Kumar
Shailender Kumar is assistant professor and co-founder of Centre for Environmental Studies and Disaster Management, Miranda House, University of Delhi and associated with the National Institute of Disaster Management, Govt of India for research on Solid waste management in postdisaster scenarios. He has worked in the field of environmental pollution monitoring and assessment for the last seven years as junior research fellow, senior research fellow at University of Delhi, and also worked at the Department of Environmental Sciences at Banaras Hindu University, Varanasi. He taught environmental science as a compulsory subject in different prestigious colleges of Delhi University His Industrial training was at the coal-based power plants of North Delhi Power Corporation, Delhi. He was a member of the organizing team in environmental pollution related seminars and workshops. His research focused on qualitative and quantitative water, soil and air analysis along with geospatial assessment and mapping of potentially toxic elements (PTEs) distribution with special emphasis on mercury exposure. His research outlined PTEs including suspected carcinogens present in threatening concentrations in NCT, Delhi. He has published in several national and international reputed journals.
Anita Dubey
Anita Dubey holds a B.Sc. and M.Sc. from DDU Gorakhpur University, Gorakhpur, India and is currently pursuing a Ph.D. program at Department of Environmental Studies, University of Delhi, New Delhi, India. Her research area includes the removal of hazardous heavy metals by nanomaterials.
Nikita Gakhar
Nikita Gakhar holds a B.Sc. in Zoology (Hons) from Ramjas College, University of Delhi and an M.Sc. in Environmental Studies from the Department of Environmental Studies, University of Delhi. Her area of interest is use of green chemistry in tackling water pollution related problems.
Chirashree Ghosh
Chirashree Ghosh holds a Ph.D. in Ecotoxicology and followed this with postdoctoral research at the University of Wageningen, the Netherlands, in the area of Wastewater Management using nonconventional method. In 2000, she received a prestigious DAAD fellowship (Germany) and attended a graduate program on water technology at the University of Applied Sciences, Suderburg, Germany. Returning home she initiated major research in the area of Environment Pollution & Health and in 2003, joined the faculty in the Department of Environmental Studies, University of Delhi. She wasproject coordinator of a number of sponsored research projects, one of her major contributions was preparation of a carbon footprint map of Delhi, which was presented at the Copenhagen summit, 2009 by state Chief Secretary. She collaborated in the national air quality monitoring program “SAFAR” since the Commonwealth Games, 2010 and is presently sharing data with the Pollution Control Board and National Green tribunal as a national database. In 2011 her Water footprint map was presented as a case study from Asia Pacific region by the international climate change work group at Johannesburg organized by IDRC, Canada. She was selected as a panel speaker by the SEG women’s network committee in 2019 at San Antonio, Texas. A teacher and researcher, she trys to mobilize the community on environmental issues and sensitizing how simple changes can help attain better quality of health.
References
- Badmapriya, D., and I.V. Asharani. 2016. Dye degradation studies catalysed by green synthesized Iron oxide nanoparticles. Int. J. ChemTech Res. 9 (6):409–16.
- Bell, J.F., T.L. Roush, and R.V. Morris. 1995. Mid‐infrared transmission spectra of crystalline and nanophase iron oxides/oxy hydroxides and implications for remote sensing of Mars. J. Geophys. Res.: Planets 100 (E3):5297–307. doi:10.1029/94JE01389.
- Choudhry, P., A. Misra, and S.N. Tripathi. 2012. Study of MODIS derived AOD at three different locations in the Indo Gangetic Plain: Kanpur, Gandhi College and Nainital. AnnalesGeophysicae 30 (10):1479–93. doi:10.5194/angeo-30-1479-2012.
- Devatha, C.P., A.K. Thalla, and S.Y. Katte. 2016. Green synthesis of iron nanoparticles using different leaf extracts for treatment of domestic waste water. J. Clean. Prod. 139:1425–35. doi:10.1016/j.jclepro.2016.09.019.
- Ebrahiminezhad, A., S. Taghizadeh, Y. Ghasemi, and A. Berenjian. 2018. Green synthesized nanoclusters of ultra-small zero valent iron nanoparticles as a novel dye removing material. Sci. Total Environ. 621:1527–32. doi:10.1016/j.scitotenv.2017.10.076.
- Gan, L., B. Li, M. Guo, X. Weng, T. Wang, and Z. Chen. 2018. Mechanism for removing 2, 4-dichlorophenol via adsorption and Fenton-like oxidation using iron-based nanoparticles. Chemosphere 206:168–74.
- Huang, L., X. Weng, Z. Chen, M. Megharaj, and R. Naidu. 2013. Synthesis of iron-based nanoparticles using Oolong tea extract for the degradation of malachite green. Spectrochim. Acta A. 117:801–804. doi:10.1016/j.saa.2013.09.054.
- Kuang, Y., Q. Wang, Z. Chen, M. Megharaj, and R. Naidu. 2013. Heterogeneous fenton-like oxidation of monochlorobenzene using green synthesis of iron nanoparticles. J Colloid Interface Sci. 410:67–73. doi:10.1016/j.jcis.2013.08.020.
- Machado, S., S.L. Pinto, J.P. Grosso, H.P.A. Nouws, J.T. Albergaria, and C. Delerue-Matos. 2013. Green production of zero-valent iron nanoparticles using tree leaf extracts. Sci. Total Environ. 445–446:1–8. doi:10.1016/j.scitotenv.2012.12.033.
- Nadagouda, M.N., A.B. Casle, R.C. Murdock, S.M. Hussain, and R.S. Varma. 2010. In vitro biocompatibility of nanoscale zero valentiron particles (NZVI) synthesized using tea polyphenols. Green Chem 12 (1):114–22. doi:10.1039/B921203P.
- Nurmi, J.T., P.G. Tratnyek, V. Sarathy, D.R. Baer, J.E. Amonette, K. Pecher, C. Wang, J.C. Linehan, D.W. Matson, R.L. Penn, et al. 2005. Characterization and properties of metallic iron nanoparticles: Spectroscopy, electrochemistry, and kinetics. Environ Sci Technol 39 (5):1221–30. doi:10.1021/es049190u.
- Ouyang, Q., F. Kou, P.E. Tsang, J. Lian, J. Xian, J. Fang, and Z. Fang. 2019. Green synthesis of Fe-based material using tea polyphenols and its application as a heterogeneous Fenton-like catalyst for the degradation of lincomycin. J. Clean. Prod. 232:1492–98. doi:10.1016/j.jclepro.2019.06.043.
- Pan, Z., Y. Lin, B. Sarkar, G. Owens, and Z. Chen. 2020. Green synthesis of iron nanoparticles using red peanut skin extract: Synthesis mechanism, characterization and effect of conditions on chromium removal. J. Colloid Interface Sci. 558:106–14.
- Perde-Schrepler, M., L. David, M. Potara, E. Fischer-Fodor, P. Virag, F. Imre-Lucaci, I. Brie, A. Florea, and L. Olenic. 2016. Gold Nanoparticles Synthesized with a Polyphenols- Rich Extract from Cornelian Cherry (Cornus mas) Fruits: Effects on Human Skin Cells. J. Nanomater. 1:Article ID 6986370.
- Poguberović, S.S., D.M. Krčmar, S.P. Maletić, Z. Kónya, D.D.T. Pilipović, D.V. Kerkez, and S.D. Rončević. 2016. Removal of As(III) and Cr(VI) from aqueous solutions using “green” zero-valent iron nanoparticles produced by oak, mulberry and cherry leaf extracts. Ecol Eng 90:42–49. doi:10.1016/j.ecoleng.2016.01.083.
- Rajeshkumar, S. 2016. Synthesis of silver nanoparticles using fresh bark of Pongamia piñata and characterization of its antibacterial activity against gram positive and gram negative pathogens. Resour. Effic. Technol 2 (1):30–35. doi:10.1016/j.reffit.2016.06.003.
- Saif, S., A. Tahir, and Y. Chen. 2016. Green synthesis of iron nanoparticles and their environmental applications and implications. Nanomaterials 6:209.
- Sajid, Z.I., F. Anwar, G. Shabir, G. Rasul, K.M. Alkharfy, and A.-H. Gilani. 2012. Antioxidant, Antimicrobial Properties and Phenolics of Different Solvent Extracts from Bark, Leaves and Seeds of Pongamia pinnata (L.) Pierre. (L.) Pierre. Molecules 17 (4):3917–32. doi:10.3390/molecules17043917.
- Schauermann, S., N. Nillius, S. Shaikhutodnov, and H.J. Freund. 2012. Nanoparticles for heterogenous catalysis: New mechanistic insights. Acc. Chem. Res. 4 (68):1673–81. doi:10.1021/ar300225s.
- Shahwan, T., S. Abu Sirriah, M. Nairat, E. Boyacı, A.E. Eroğlu, T.B. Scott, and K.R. Hallam. 2011. Green synthesis of iron nanoparticles and their application as a fenton-like catalyst for the degradation of aqueous cationic and anionic dyes. Chem. Eng. J. 172 (1):258–66. doi:10.1016/j.cej.2011.05.103.
- Smuleac, V., R. Varma, S. Sikdar, and D. Bhattacharyya. 2011. Green synthesis of Fe and Fe/Pd bimetallic nanoparticles in membranes for reductive degradation of chlorinated organics. J Memb Sci 379 (1):131–37. doi:10.1016/j.memsci.2011.05.054.
- Steponeniene, L., S. Tautkus, and R. Kazlauskas. 2003. Determination of zinc in plants and grains by atomic absorption spectrometry. Chemija 14 (2):99–102.
- Subbenaik, S.C. 2016. Physical and Chemical Nature of Nanoparticles. In Plant Nanotechnology, ed. C. Kole, D. Kumar, and M. Khodakovskaya, 15–27. Cham: Springer.
- Vasantharaj, S., S. Sathiyavimal, P. Senthilkumar, F. Lewis Oscar, and A. Pugazhendhi. 2019. Biosynthesis of iron oxide nanoparticles using leaf extract of Ruellia tuberosa: Antimicrobial properties and their applications in photocatalytic degradation. Photochem. Photobiol. B Biol 192:74–82. doi:10.1016/j.jphotobiol.2018.12.025.
- Wang, Z., C. Fang, and M. Megharaj. 2014. Characterization of iron–polyphenol nanoparticles synthesized by three plant extracts and their fenton oxidation of azo dye. ACS Sustain. Chem. Eng. 2:1022–25.
- Wu, J., M. Lin, X. Weng, G. Owens, and Z. Chen. 2021. Pre-adsorption and Fenton-like oxidation of mitoxantrone using hybrid green synthesized rGO/Fe nanoparticles. Chem. Eng. J. 408:127273. doi:10.1016/j.cej.2020.127273.
- Xiao, C., H. Li, Y. Zhao, X. Zhang, and X. Wang. 2020. Green synthesis of iron nanoparticle by tea extract (polyphenols) and its selective removal of cationic dyes. J. Environ. Manage. 275:111262. doi:10.1016/j.jenvman.2020.111262.
- Yan, L., W. Chen, X. Zhu, L. Huang, Z. Wang, G. Zhu, and X. Chen. 2013. Folic acid conjugated self-assembled layered double hydroxide nanoparticles for high-efficacy-targeted drug delivery. Chemical Communications 49 (93):10938–40.
- Zhu, F., S. Ma, T. Liu, and X. Deng. 2018. Green synthesis of nano zero-valent iron/Cu by green tea to remove hexavalent chromium from groundwater. J. Clean. Prod. 174:184–90. doi:10.1016/j.jclepro.2017.10.302.