Abstract
An increasing amount of attention has been devoted to studying the impact of non-native plant and animal species on native species. In this paper, we examined the antipredator response of naïve European brown frog (Rana temporaria Linnaeus, 1758) tadpoles to water-borne chemical cues from invasive red-eared slider turtles (Trachemys scripta elegans Wied, 1838) in the presence and the absence of conspecific tadpoles. The level of swimming activity was expressed as the length of the tadpoles’ trajectory. The tadpoles showed a decrease in swimming activity when predator stimuli were present, which was highly significant when the tadpoles were measured individually and did not have contact (visual and chemical) with other tadpoles. In the absence of chemical cues of slider turtles, the presence of other tadpoles had no effect on the level of swimming activity. Moreover, for the first time in tadpoles, we found that the decrease in swimming activity was also accompanied by changes in swimming trajectory, with tadpoles exposed to predator cues swimming in more zigzagged trajectories. Our experiment shows that invasive slider turtles, as a novel predator, have a measurable influence on the swimming behaviour of European brown frog tadpoles. Consequences of the reduced swimming activity of tadpoles, like its impact on growth, are discussed.
Introduction
Invasive species have a documented or presumed negative impact on native fauna and flora. The amount of literature devoted to the study of non-native plant and animal species is steadily increasing (e.g. Cox Citation2004; DAISIE Citation2009; Rotherham & Lambert Citation2011; Parker et al. Citation2013). Numerous impacts have been described, including the emergence of new diseases, increased predation, hybridization with native species, alteration of biotic communities, and even extirpations or extinctions of native species (Cox Citation2004; Kraus Citation2009; Paolucci et al. Citation2013). In addition to the direct impact on the survival of native species, many invasive species can shape or alter the life history of natives by changing their typical behaviour patterns (e.g. Griffiths et al. Citation1998; Cadi & Joly Citation2004; Almeida et al. Citation2011; Lenda et al. Citation2013). Documented reduction in activity and swimming performance can lead to changes in predation rates and, because activity and feeding are tightly bound, may result in slowed growth leading to a failure to emerge before pond drying or an indirect reduction in adult fitness (Bulen & Distel Citation2011; García-Muñoz et al. Citation2011).
The pond slider (Trachemys scripta) is naturally confined to the United States, from southeastern Virginia to northern Florida (Ernst & Lovich Citation2009). However, this turtle species has been widely introduced, both purposely and incidentally, on every continent except Antarctica (Kraus Citation2009). Most introductions are simply the side effect of pet trade (unwanted overgrown turtles are released into the wild), but T. scripta has been able to reproduce in many of its introduced habitats (Rödder et al. Citation2009). The subspecies T. s. elegans was imported to Europe as pets and other parts of the world in huge numbers; during the period from 1989 to 1997, 52 million turtles were exported from the United States (Scalera Citation2009), and in many countries, breeding populations were founded (France: Cadi & Joly Citation2003; Italy: Ficetola et al. Citation2002; Spain: Ramsay et al. Citation2007). Within the turtle’s non-natural range, the impacts of escaped or introduced individuals on native species have been repeatedly documented. For example, slider turtles were observed to outcompete native turtles for their favoured basking sites (Cadi & Joly Citation2003; Kaltenegger Citation2006; Polo-Cavia et al. Citation2010), which can lead to both weight loss and high mortality of individuals of less competitive species (Cadi & Joly Citation2004). When shared resources become limited, red-eared slider turtles negatively impact the growth of native turtles, and are also expected to cause long-term population declines in native species that are already threatened, such as the red-bellied turtle (Pseudemys rubriventris) (Pearson et al. Citation2013, Citation2015). Even the presence of slider turtle chemical stimuli can lead to the avoidance of such localities by native turtle species (Polo-Cavia et al. Citation2009). While the import of T. s. elegans to the European Union has been prohibited since 1997, new records continue to be reported as pet animals that were imported before 1997 or bred in captivity are discarded in the wild.
Pond sliders are known as opportunistic omnivores, and their diet includes amphibians in any of their life history phases (Ernst & Lovich Citation2009). Many studies have demonstrated that tadpoles can rapidly and strikingly respond to the presence of chemical stimuli from various predators (Kats & Dill Citation1998; Maher et al. Citation2013). Such responses include behaviourally mediated changes in activity, morphological changes (Hossie & Murray Citation2012) and metabolic responses (Barry & Syal Citation2013). However, several studies have confirmed that native larval amphibians are often not able to recognize introduced species as potential predators due to the absence of common evolutionary history (Kiesecker & Blaustein Citation1997; Polo-Cavia et al. Citation2010; but see Chivers & Smith Citation1995; Kiesecker & Blaustein Citation1997; Chivers et al. Citation2001 for documented cases of native prey ability to avoid invasive species). In this study, we experimentally tested the response of European brown frog (Rana temporaria) tadpoles to the presence of chemical cues from a potential novel turtle (T. scripta), which has spread throughout Czech Republic recently (Brejcha Citation2015). We focused on the changes of movement activity as one type of antipredatory behaviour measuring the swimming activity of the tadpoles; however, we also investigated the effect of the predator’s presence on the shape of the swimming path. Based on some recent studies (Alford Citation1999; Relyea Citation2001; Brown et al. Citation2003; Kopack et al. Citation2015), we expect that predation risk in tadpoles can cause: (1) decrease of swimming activity and (2) changes in the swimming trajectory of tadpoles.
Materials and methods
Ethics statement
The Biology Centre, Academy of Sciences of the Czech Republic in České Budějovice, received accreditation for experiments on vertebrates according to article No. 15, section 2, act registered under number 9103/2009-17210 from the Czech Ministry of Agriculture, Department of Animal Welfare. The senior author is qualified to perform experiments on vertebrates by the Central Commission for the Protection of Animals (license number ČZU604/03).
Experimental animals
In March 2012, we netted 200 naïve tadpoles of the European brown frog, Rana temporaria, from a small temporary pool near České Budějovice, Czech Republic (48°58′50″N, 14°25′11″E). Naïve tadpoles were those that had had no previous contact with slider turtles or any other turtle species (no occurrence is reported for this locality so far by the national authority – http://portal.nature.cz), which ensured that there was no habituation response through learned recognition of the introduced predator. The tadpoles were housed in a 45 × 27 × 27 cm (length × depth × height) glass tank with aged tap water and a foam filter. An adult female of T. s. elegans (bred in captivity, obtained from a hobby shop) was maintained in a similar-sized terrarium with a water level of 16 cm. Both tanks were placed on a shelf in a climate room that was maintained at 23 ± 1°C, and light was provided by fluorescent tubes for 12 hours per day. The tadpoles were fed a commercial flake food for aquarium fishes ad libitum, and the turtle was fed chicken flesh and food pellets for freshwater turtles. Both species were fed every day after the measurements. Evaporated water was replaced as required in the tadpoles’ tank. Water in the turtle’s tank was changed every day after the experiment. Procedures conformed to the recommended guidelines for the use of live amphibians and reptiles in laboratory research (Beaupre et al. Citation2004).
Experimental design
The tadpoles’ activity was measured by randomly selecting six tadpoles of the same age from the deposit tank and placing them in a set of six plastic rectangular containers (arenas) with slanted opaque sides (10.0 × 5.5 cm and 7.5 × 3.0 cm inner dimensions at the top and bottom, respectively, and 4.0 cm in height) arranged in a 2 × 3 grid. Slanted walls enabled the tracking of a tadpole in the whole arena of all containers (when vertical walls are used some parts of the arenas are not visible) by the camera, and in the “perforated walls experiment” this also facilitated water circulation between containers. Opaque sides prevent any visual cues from being transmitted and minimize any reflections of tadpoles’ bodies, which would interfere with videotracking. One tadpole was placed in each arena of the set. Tadpoles had been acclimatised for 30 minutes before each measurement. During the measurements, the height of the water column in the arenas was 4 cm. For treatments investigating the effect of tadpole presence in the group, the sides of each arena were perforated to allow water passage between arenas. The development stage of the tadpoles that were used in the trials ranged from 34 to 36, according to the stages developed by Gosner (Citation1960). The tadpoles were only used once each in the trials.
The experimental design consisted of four treatments. In treatment 1, each tadpole was exposed to the turtle chemical stimuli. Turtle chemical cues were attained by placing the turtle in 20 L of aged tap water for 20 hours. This water was replaced into the experimental tank. Tadpoles’ activity was measured after 30 minutes of acclimatisation. In treatment 2, each tadpole was exposed to both of the investigated factors: the chemical cues of the turtle and other tadpoles (group effect). In treatment 3, each tadpole was exposed to chemical stimuli from other tadpoles. Treatment 4 was the control, wherein each tadpole’s swimming activity was measured in the absence of exposure to chemical cues from either the turtle or other tadpoles. All measurements were performed between 09:00 and 14:00 in an air-conditioned cabinet at 23 ± 1°C. For each treatment type, 30 tadpoles were measured.
Video tracking, data acquisition and statistical analysis
The swimming patterns of tadpoles were observed over 30 minutes with a colour CCD camera with a zoom lens. The camera was fixed vertically above the centre of the set of six experimental arenas. A composite video signal was fed into a computerized video tracking system that consisted of a video monitor, a personal computer with a frame grabber (Targa Plus, Truevision, Inc., Indianapolis, USA) and EthoVision software (Noldus Information Technology Citation1997). The location of each tadpole was determined automatically by the software using the grey scaling method of object detection. The coordinates of the centre of each animal’s body (centre of gravity) were calculated using a spatial resolution of 256 × 238 pixels in the x- and y directions, respectively. Tracking was recorded at a frequency of two frames per second, which was a compromise of requirements for the highest possible sampling rate (Bell Citation1991) based on the processor speed and storage capacity of the computer.
The rate of the tadpoles’ locomotor activity was expressed as the length of the tadpoles’ swimming trajectory. Data from the digitized paths of individual tadpoles were used to calculate the following parameters: (1) total path length (TPL), (2) absolute turn angle (ATA), (3) absolute angular velocity (AAV) and (4) absolute meander (AM). The TPL (or total distance moved) is defined as the sum of the distances measured in a straight line that a tadpole moved in a sample interval () and as such measures total swimming distance. The other three variables describe the swimming path. The ATA is defined as the unsigned angle between the movement vectors of two consecutive sample intervals. The AAV (or turning rate) is the absolute change in the direction of movement of a tadpole per unit time and ranges from 0 to 180 degrees/sec. The AM is defined as the absolute change in the direction of movement of a tadpole relative to the distance moved. It is calculated according to the equation AM = |RTA/DM| where RTA is relative turn angle and DM is distance moved. The AM indicated the tortuosity of the swimming path and ranges from 0 to 180 degrees/cm. For detailed algorithms of parameter calculation, see Bell (Citation1991) and Noldus Information Technology (Citation1997). To prevent the scoring of slight movements that were caused by noise in the system (pivoting on the spot) as genuine displacement, a threshold value of 2 mm was used in the analyses. Thus, a parameter per sample was calculated once the tadpole moved at least 2 mm away from the point at which the parameter was last calculated. The data were normalized by exponentiation with an exponent of 2/3, and the data were statistically analysed by two-way analysis of variance (ANOVA) followed by Tukey honest significant difference (HSD) tests to determine the individual effects using the Statistica v. 9 software package (StatSoft Inc. Citation2010).
Results
Tadpoles of the European common brown frog were active when they were released into the arenas and spent the majority of the 30-minute trials swimming. The motion analysis showed that they modified their activity when they came into contact with chemical cues of their potential predator, the turtle T. s. elegans (; ). The distance covered during the trials with and without turtle cues differed significantly. The highest TPL was observed in the control treatments when the individual tadpoles were not exposed to tadpole or turtle chemical stimuli (single treatment; mean ± SD: 9.16 ± 6.39 m); the next highest average activity values were tadpoles exposed to tadpole chemical stimuli alone (group treatment; 6.71 ± 5.06 m) and tadpoles exposed to both tadpole and turtle chemical stimuli (6.69 ± 4.11 m). The lowest activity was observed in the treatment with turtle chemical stimuli alone, without any chemical contact with other tadpoles (5.05 ± 3.86 m; ). While the influence of the presence or absence of tadpole chemical contact (single or group treatment) was not significant, the ANOVA test showed a significant interaction between this factor and the presence or absence of turtle chemical stimuli (). The TPL of single tadpoles was significantly lower in the presence of chemical cues from predatory sliders (Tukey HSD test for pairwise comparisons: p < 0.05), but predator cues did not affect TPL of tadpoles when they were in contact with conspecific cues (Tukey HSD test for pairwise comparisons: p = 0.99; ).
Table I. Results of the two-way analyses of variance (ANOVAs) analysing the crossed effects of predator cues from Trachemys scripta and conspecific cues on tadpoles’ activity. df = 1,116 in all cases.
Figure 2. Graphical summary of tadpole parameters in all experimental conditions (mean values and 95% confidential intervals): A, total path length (TPL), B, absolute turn angle (ATA), C, absolute meander (AM), and D, absolute angular velocity (AAV).
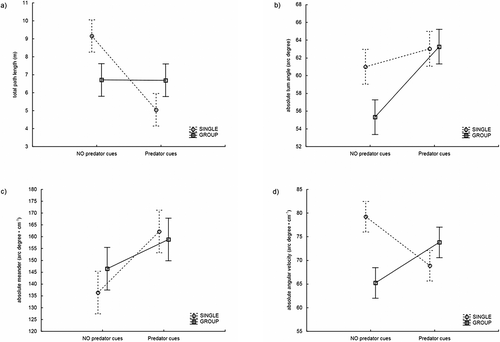
Of the three parameters describing the shape of the swimming path, both ATA and AM were higher when tadpoles were in contact with turtle chemical cues (63.15 ± 12.59° for ATA; 160.55 ± 61.06° cm−1 for AM) than in the non-predator treatment (58.16 ± 8.45° for ATA; 141.42 ± 31.3° cm−1 for AM), with independence of conspecifics cues (; ). Neither the presence of predator cues nor tadpole exposition to conspecifics impacted on AAV. However, their interaction was highly significant for AAV (). In the non-predator treatment, the AAV of single tadpoles was higher than that of tadpoles exposed to conspecific cues (Tukey HSD test for pairwise comparisons: p < 0.05).
Discussion
The responses of tadpoles to the presence of predators are numerous and varied (see Ferrari et al. Citation2010 and references therein). Because amphibian larvae can detect chemical cues that are emitted by specific predators, a precise combination of responses may be favoured when they are confronted by such predators (Van Buskirk Citation2001). Potential invasive predators represent a serious threat to many naïve native species (Cox Citation2004). Considering the independent evolution of the predator and the prey, the introduction of alien species into a new community is unpredictable and often results in destructive outcomes (Paolucci et al. Citation2013).
A specific and frequently documented reaction of the prey to the presence of a predator is the distinctive reduction of locomotor activity (Alford Citation1999; Relyea Citation2001), although an increase in locomotor activity has also been reported (Škaloudová et al. Citation2007). Here, we present additional evidence of a reduction of swimming activity in tadpoles of the European brown frog when they are faced with the presence of water-borne chemical cues of an invasive slider turtle. Contrary to other studies that used the proportion of active tadpoles (Griffiths et al. Citation1998; Awan & Smith Citation2007; McClure et al. Citation2009; Almeida et al. Citation2011; Nunes et al. Citation2012; Pujol-Buxó et al. Citation2013), spatial avoidance (Almeida et al. Citation2011; Nunes et al. Citation2012) or the number of movements (Polo-Cavia et al. Citation2010) as a proxy of swimming activity, we used a more accurate approach by videotracking and analysing exact swimming trajectories and lengths, including path-shape parameters. We observed that tadpoles significantly reduced their swimming activity when chemical stimuli from the slider turtle were present in the water. In tanks with turtle chemical stimuli and without contact with other tadpoles, the average tadpole swimming length was 55% of the length that was covered by tadpoles without turtle chemical cues. Tadpoles in chemical contact with other tadpoles and tadpoles without it behaved consistently.
The time unstressed tadpoles spend swimming is tightly correlated with the time spent feeding (Egea-Serrano et al. Citation2011), and thus reduction in swimming activity may slow down growth. However, due to the complex life cycle of frogs, no studies on this exist so far. Many studies (reviewed in Bolker et al. Citation2003; Werner & Peacor Citation2003; Schmitz et al. Citation2004) demonstrated that antipredator behaviour caused reduced feeding. This could result in a failure to emerge before pond drying or indirectly in a reduction in adult fitness (García-Muñoz et al. Citation2011; Çakıcı Citation2013). Many tadpoles escape predators by being too large to capture or by emerging from ponds as early as possible. Tadpoles must reach a minimum size before metamorphosis, and faster growth shortens the larval period, thus ultimately decreasing exposure to predators. Therefore, reduced growth can lead to indirect mortality by prolonging the susceptibility to predators, either by tadpoles remaining small enough to capture or by lengthening the larval period (Bulen & Distel Citation2011). On the other hand, tadpoles can proximately reduce the probability of being the victims of a predatory event by diminishing their actual swimming activity. The tactic used can therefore depend on the sensory capability of syntopic predators (visually or tactically versus chemically orienting predators). In natural systems, tadpoles spend their lives in a cocktail of chemical cues from potential predators and food sources. Previous research has reported examples of (1) frog species that only respond to predators that they would naturally experience in the wild (Kiesecker et al. Citation1996; Griffiths et al. Citation1998; Polo-Cavia et al. Citation2010; Almeida et al. Citation2011; Nunes et al. Citation2012) and (2) species that shape their behaviour based on the presence of either native or alien predators (Almeida et al. Citation2011; Nunes et al. Citation2012). As we are aware, there is no report about amphibian species which respond to novel predators only. The European brown frog tadpoles in this study had never been exposed to either slider turtles or any other turtle or tortoise species; therefore, our measurements show that they belong to the second group of species, which shape their behaviour based on the presence of both native (Laurila & Kujasalo Citation1999) and alien predators (this study). Reduced activity in the presence of a potential predator may decrease the likelihood of a tadpole being consumed (Anholt et al. Citation2005). The larvae of two other European frogs, Pelophylax perezi and Pelobates cultripes, have been shown to innately reduce their activity levels when they are in contact with the native turtle Mauremys leprosa; however, neither species’ larvae reduced their activity when they were presented with chemical cues from the non-native slider turtle Trachemys scripta (Polo-Cavia et al. Citation2010). According to Sih et al. (Citation2010), the ability of naïve prey to recognize non-native predators depends on the similarity of cues between the introduced and native predators, and the generality of cues that are used by the prey to assess the risk of predation. In our study, the first possibility does not apply because our tadpoles were non-sympatric with all turtle species. Furthermore, in central European conditions, the predator of brown frog tadpoles that is most closely related to the slider turtle (in terms of the evolutionary relationship) is the grass snake (Natrix natrix). We can only speculate about whether slider turtles produce universal predator cues or water-borne stimuli that tadpoles are able to evaluate as a threat. Studies that were designed to test the generalization of predator recognition in aquatic vertebrates have not obtained clear results (Chivers & Smith Citation1994; Darwish et al. Citation2005), which has led certain authors to hypothesize that generalization behaviour generally fails when presented with distantly related predators (Polo-Cavia et al. Citation2010). Our results may contradict this conclusion.
Factors that influence tadpole activity may include a diverse range of cues, including lighting conditions, predator presence and group size. While the group effect was not significant in our experiment, the presence of conspecific cues interacted significantly with the predator treatment in the case of TPL and AAV (see ). The majority of published results demonstrate no effect (Golden et al. Citation2000; Awan & Smith Citation2007) or the opposite effect: an increase of swimming activity with increasing group size (Relyea Citation2002; Spieler Citation2005; Rot-Nikcevic et al. Citation2006; McClure et al. Citation2009). In another study using European brown frog tadpoles, individuals who were raised in groups were more active than those that were raised alone (Nicieza Citation1999).
Group size also interacted with the presence or absence of predator chemical cues to influence the activity of the tadpoles. This result reflects the observation that tadpoles in large groups of conspecifics engage in riskier behaviours because of the diluted or reduced risk of predation (e.g. Spieler Citation2005). Similarly, Relyea (Citation2004) found that in the presence of odonate predators, the activity of wood frog (Rana sylvatica) tadpoles increased with increasing densities of conspecific tadpoles. However, in similar experiments with wood frog tadpoles and the predatory fish Lepomis macrochirus, Awan and Smith (Citation2007) found no evidence of this type of interaction. In the same paper, the authors discussed their own unpublished results of experiments on the behavioural response of different group sizes of tadpoles of the American toad (Bufo americanus) and bullfrog (Rana catesbeiana) to the presence of fish (Lepomis macrochirus) and invertebrate (dytiscid beetle larvae) predators; their results suggested that these tadpole species demonstrate the expected interaction between group size and predator cues.
The reduction of swimming activity is not the only tadpole response to signs of the presence of predators; changes in the shape of the swimming path may have importance as a part of antipredatory behaviour as well. In our study, we found that tadpoles in contact with the turtle chemical stimuli swam in different trajectories than tadpoles without such contact. Specifically, their ATA and AM were higher, which means that their swimming path shape was more tortuous or zigzagged. We are aware of only a handful papers that measured the influence of predator cues on the total path trajectory of potential prey; however, all three studies were performed on mites, so a comparison with their results would yield limited value. In the study by Škaloudová et al. (Citation2007), no significant effect of predatory cues on the two-spotted spider mite path shape was found. However, experiments by Walzer and Schausberger (Citation2013a) showed that high-risk predators triggered more rapid changes of direction than did low-risk predators. Moreover, Walzer and Schausberger (Citation2013b) documented the effect of the type of predator chemical cue on the prey path shape and revealed that traces of the females of a high-risk predator species reduced the turning angles of prey compared to other types of predator cues. However, Van Buskirk and McCollum (Citation2000) did not find a difference in swimming performance (specifically angle of escape) between the predator-induced deep-tailed phenotype and tadpoles from predator-free ponds of tadpoles of Hyla chrysoscelis, and concluded that swimming performance is not the factor responsible for the lower vulnerability of predator-induced tadpoles. The more tortuous path found in the present study would indicate that tadpoles either try to avoid possible predator attacks or try to escape and hide somewhere. We are aware of the fact that under real conditions when tadpoles are not constrained by a container’s walls the values of, for example, AM might be lower. Moreover, in a large volume of water, the chemical cues of a single turtle will probably create some gradient while in small containers (as in this study) they are evenly dispersed. The gradient would probably increase the dispersal rate of tadpoles, but to verify this hypothesis much larger arenas should be used (cf. Socha & Zemek Citation2003).
We do not know the exact substances responsible for the behavioural changes of tadpoles. Tadpole behavioural responses can be mediated by two not mutually exclusive types of chemical cues. First, tadpoles only react to chemical cues that are produced by their conspecific individuals as prey during predation events (McClure et al. Citation2009). Second, chemical cues that are inadvertently emitted by the potential predator are sufficient stimuli for behavioural change (Griffiths et al. Citation1998). Because our tadpoles do change their behaviour in contact with pond slider cues, our tadpoles match the second group of behavioural responses.
In conclusion, our experiments show for the first time that invasive slider turtles, as a novel predator, have a measurable influence on the swimming activity of European brown frog tadpoles. Moreover, our results show that changes in swimming activity are not the only response to the presence of predators; variation in swimming path shape is another possible coping strategy that should be considered in future studies. Additional experiments are required to determine the impact of these behavioural changes to cope with predators.
Acknowledgements
This research was conducted with institutional support RVO: 60077344. We would like to thank Vlastimil Křivan, Zdeněk Faltýnek Fric and three anonymous reviewers for insightful comments on an earlier draft of the manuscript.
References
- Alford RA. 1999. Ecology: Resource use, competition, and predation. In: McDiarmid RW, Altig R, editors. Tadpoles: The biology of anuran larvae. Chicago, USA: University of Chicago Press. pp. 240–278.
- Almeida E, Nunes A, Andrade P, Alves S, Guerreiro C, Rebelo R. 2011. Antipredator responses of two anurans towards native and exotic predators. Amphibia-Reptilia 32:341–350. doi:10.1163/017353711X579849.
- Anholt BR, Negovetic S, Rauter C, Som C. 2005. Predator complement determines the relative success of tadpoles of the Rana esculenta complex. Evolutionary Ecology Research 7:733–741.
- Awan AR, Smith GR. 2007. The effect of group size on the responses of wood frog tadpoles to fish. The American Midland Naturalist 158:79–84. doi:10.1674/0003-0031(2007)158[79:TEOGSO]2.0.CO;2.
- Barry MJ, Syal S. 2013. Metabolic responses of tadpoles to chemical predation cues. Hydrobiologia 700:267–276. doi:10.1007/s10750-012-1236-4.
- Beaupre SB, Jacobson ER, Lillywhite HB, Zamudio K. 2004. Guidelines for use of live amphibians and reptiles in field and laboratory research. Lawrence, KS: American Society of Ichthyologists and Herpetologists, approved by board of Governors.
- Bell WJ. 1991. Searching behaviour: The behavioural ecology of finding resources. 1st ed. London, UK: Chapman and Hall.
- Bolker B, Holyoak M, Krivan V, Rowe L, Schmitz OJ. 2003. Connecting theoretical and empirical studies of trait-mediated interactions. Ecology 84:1101e1114. doi:10.1890/0012-9658(2003)084[1101:CTAESO]2.0.CO;2.
- Brejcha J. 2015. Želva nádherná - aktuální status druhu v České republice. In: Moravec J, editor. Plazi (Reptilia) - Fauna ČR. Praha, Česká republika: Academia. pp. 531.
- Brown C, Davidson T, Laland K. 2003. Environmental enrichment and prior experience of live prey improve foraging behaviour in hatchery-reared Atlantic salmon. Journal of Fish Biology 63:187–196. doi:10.1111/jfb.2003.63.issue-s1.
- Bulen BJ, Distel CA. 2011. Carbaryl concentration gradients in realistic environments and their influence on our understanding of the tadpole food web. Archives of Environmental Contamination and Toxicology 60:343–350. doi:10.1007/s00244-010-9630-2.
- Cadi A, Joly P. 2003. Competition for basking places between the endangered European pond turtle (Emys orbicularis galloitalica) and the introduced red-eared slider (Trachemys scripta elegans). Canadian Journal of Zoology-Revue Canadienne De Zoologie 81:1392–1398. doi:10.1139/z03-108.
- Cadi A, Joly P. 2004. Impact of the introduction of the red-eared slider (Trachemys scripta elegans) on survival rates of the European pond turtle (Emys orbicularis). Biodiversity and Conservation 13:2511–2518. doi:10.1023/B:BIOC.0000048451.07820.9c.
- Çakıcı Ö. 2013. Carbaryl-induced histopathologic alterations on testes of Levantine frog, Pelophylax bedriagae (Anura: Ranidae). Bulletin of Environmental Contamination and Toxicology 91:96–101. doi:10.1007/s00128-013-1010-y.
- Chivers DP, Smith RJF. 1994. Fathead minnows, Pimephales promelas, acquire predator recognition when alarm substance is associated with the sight of unfamiliar fish. Animal Behaviour 48:597–605. doi:10.1006/anbe.1994.1279.
- Chivers DP, Smith RJF. 1995. Free—Living fathead minnows rapidly learn to recognize pike as predators. Journal of Fish Biology 46:949–954. doi:10.1111/jfb.1995.46.issue-6.
- Chivers DP, Wildy EL, Kiesecker JM, Blaustein AR. 2001. Avoidance response of juvenile pacific treefrogs to chemical cues of introduced predatory bullfrogs. Journal of Chemical Ecology 27:1667–1676. doi:10.1023/A:1010418526991.
- Cox GW. 2004. Alien species and evolution: The evolutionary ecology of exotic plants, animals, microbes, and interacting native species. 1st ed. Washington, USA: Island Press. pp. 377.
- DAISIE (ed.). 2009. Handbook of alien species in Europe. Dordrecht, Netherlands: Springer.
- Darwish TL, Mirza RS, Leduc A, Brown GE. 2005. Acquired recognition of novel predator odour cocktails by juvenile glowlight tetras. Animal Behaviour 70:83–89. doi:10.1016/j.anbehav.2004.09.017.
- Egea-Serrano A, Tejedo M, Torralva M. 2011. Behavioral responses of the Iberian waterfrog, Pelophylax perezi (Seoane, 1885), to three nitrogenous compounds in laboratory conditions. Ecotoxicology 20:1246–1257. doi:10.1007/s10646-011-0673-6.
- Ernst CH, Lovich JE. 2009. Turtles of the United States and Canada. 2nd ed. Baltimore, USA: Johns Hopkins University Press. pp. 827.
- Ferrari MC, Wisenden BD, Chivers DP. 2010. Chemical ecology of predator-prey interactions in aquatic ecosystems: A review and prospectus. Canadian Journal of Zoology 88:698–724. doi:10.1139/Z10-029.
- Ficetola GF, Monti A, Padoe-Schippoa E. 2002. Prima segnalazione di riproduzione di Trachemys scripta elegans nel Delta del Po. Annali del Museo civico di Storia naturale di Ferrara 5:125–128.
- García-Muñoz E, Guerrero F, Parra G. 2011. Larval escape behavior in anuran amphibians as a wetland rapid pollution biomarker. Marine and Freshwater Behaviour and Physiology 44:109–123. doi:10.1080/10236244.2011.557855.
- Golden D, Smith G, Rettig J. 2000. Effects of age and group size on habitat selection and activity level in Xenopus laevis tadpoles. Transactions of the Nebraska Academy of Sciences and Affiliated Societies 26:23–27.
- Gosner KL. 1960. A simplified table for staging anuran embryos and larvae with notes on identification. Herpetologica 16:183–190.
- Griffiths RA, Schley L, Sharp PE, Dennis JL, Roman A. 1998. Behavioural responses of Mallorcan midwife toad tadpoles to natural and unnatural snake predators. Animal Behaviour 55:207–214. doi:10.1006/anbe.1997.0596.
- Hossie TJ, Murray DL. 2012. Assessing behavioural and morphological responses of frog tadpoles to temporal variability in predation risk. Journal of Zoology 288:275–282. doi:10.1111/j.1469-7998.2012.00955.x.
- Kaltenegger D. 2006. Die heimische Europäische Sumpfschildkröte (Emys orbicularis) und die zunehmende Problematik durch illegal ausgesetzte Rotwangen-Schmuckschildkröten (Trachemys scripta elegans). Österreichs Fischerei 59:93–97.
- Kats LB, Dill LM. 1998. The scent of death: Chemosensory assessment of predation risk by prey animals. Ecoscience 5:361–394.
- Kiesecker JM, Blaustein AR. 1997. Population differences in responses of red-legged frogs (Rana aurora) to introduced bullfrogs. Ecology 78:1752–1760. doi:10.1890/0012-9658(1997)078[1752:PDIROR]2.0.CO;2.
- Kiesecker JM, Chivers DP, Blaustein AR. 1996. The use of chemical cues in predator recognition by western toad tadpoles. Animal Behaviour 52:1237–1245. doi:10.1006/anbe.1996.0271.
- Kopack CJ, Broder ED, Lepak JM, Fetherman ER, Angeloni LM. 2015. Behavioral responses of a highly domesticated, predator naïve rainbow trout to chemical cues of predation. Fisheries Research 169:1–7. doi:10.1016/j.fishres.2015.04.005.
- Kraus F. 2009. Alien reptiles and amphibians: A scientific compendium and analysis. 1st ed. Dordrecht, Netherlands: Springer.
- Laurila A, Kujasalo J. 1999. Habitat duration, predation risk and phenotypic plasticity in common frog (Rana temporaria) tadpoles. Journal of Animal Ecology 68:1123–1132. doi:10.1046/j.1365-2656.1999.00354.x.
- Lenda M, Witek M, Skorka P, Moron D, Woyciechowski M. 2013. Invasive alien plants affect grassland ant communities, colony size and foraging behaviour. Biological Invasions 15:2403–2414. doi:10.1007/s10530-013-0461-8.
- Maher JM, Werner EE, Denver RJ. 2013. Stress hormones mediate predator-induced phenotypic plasticity in amphibian tadpoles. Proceedings of the Royal Society B-Biological Sciences 280:2012–3075.
- McClure KV, Mora JW, Smith GR. 2009. Effects of light and group size on the activity of wood frog tadpoles (Rana sylvatica) and their response to a shadow stimulus. Acta Herpetologica 4:103–107.
- Nicieza AG. 1999. Context-dependent aggregation in Common Frog Rana temporaria tadpoles: Influence of developmental stage, predation risk and social environment. Functional Ecology 13:852–858. doi:10.1046/j.1365-2435.1999.00375.x.
- Noldus Information Technology. 1997. Ethovision: Video tracking, motion analysis and behavior recognition system [Reference Manual]. Version 1.90. Wagenigen, Netherlands: Noldus Information Technology.
- Nunes AL, Richter-Boix A, Laurila A, Rebelo R. 2012. Do anuran larvae respond behaviourally to chemical cues from an invasive crayfish predator? A community-wide study. Oecologia 171:115–127. doi:10.1007/s00442-012-2389-6.
- Paolucci EM, MacIsaac HJ, Ricciardi A. 2013. Origin matters: Alien consumers inflict greater damage on prey populations than do native consumers. Diversity and Distributions 19:988–995. doi:10.1111/ddi.12073.
- Parker JD, Torchin ME, Hufbauer RA, Lemoine NP, Alba C, Blumenthal DM, Bossdorf O, Byers JE, Dunn AM, Heckman RW. 2013. Do invasive species perform better in their new ranges? Ecology 94:985–994. doi:10.1890/12-1810.1.
- Pearson SH, Avery HW, Kilham SS, Velinsky DJ, Spotila JR. 2013. Stable isotopes of C and N reveal habitat dependent dietary overlap between native and introduced turtles Pseudemys rubriventris and Trachemys scripta. Plos One 8:e62891. doi:10.1371/journal.pone.0062891.
- Pearson SH, Avery HW, Spotila JR. 2015. Juvenile invasive red-eared slider turtles negatively impact the growth of native turtles: Implications for global freshwater turtle populations. Biological Conservation 186:115–121. doi:10.1016/j.biocon.2015.03.001.
- Polo-Cavia N, Gonzalo A, López P, Martín J. 2010. Predator recognition of native but not invasive turtle predators by naïve anuran tadpoles. Animal Behaviour 80:461–466. doi:10.1016/j.anbehav.2010.06.004.
- Polo-Cavia N, Lopez P, Martin J. 2009. Interspecific differences in chemosensory responses of freshwater turtles: Consequences for competition between native and invasive species. Biological Invasions 11:431–440. doi:10.1007/s10530-008-9260-z.
- Pujol-Buxó E, San Sebastián O, Garriga N, Llorente GA. 2013. How does the invasive/native nature of species influence tadpoles’ plastic responses to predators? Oikos 122:19–29. doi:10.1111/more.2013.122.issue-1.
- Ramsay NF, Ng PKA, O’Riordan RM, Chou LM. 2007. The red-eared slider (Trachemys scripta elegans) in Asia: A review. In: Gherardi F, editor. Biological invaders in inland waters: Profiles, distribution and threats. Dordrecht: Springer. pp. 161–174.
- Relyea RA. 2001. Morphological and behavioral plasticity of larval anurans in response to different predators. Ecology 82: 523–540. doi:10.1890/0012-9658(2001)082[0523:MABPOL]2.0.CO;2.
- Relyea RA. 2002. Competitor-induced plasticity in tadpoles: Consequences, cues, and connections to predator-induced plasticity. Ecological Monographs 72: 523–540. doi:10.1890/0012-9615(2002)072[0523:CIPITC]2.0.CO;2.
- Relyea RA. 2004. Fine-tuned phenotypes: Tadpole plasticity under 16 combinations of predators and competitors. Ecology 85: 172–179. doi:10.1890/03-0169.
- Rödder D, Schmidtlein S, Veith M, Loetters S. 2009. Alien invasive slider turtle in unpredicted habitat: A matter of niche shift or of predictors studied? Plos One 4: e7843. doi:10.1371/journal.pone.0007843.
- Rotherham ID, Lambert RA. 2011. Invasive and introduced plants and animals: Human perceptions, attitudes, and approaches to management. 1st ed. Washington, DC, USA: Earthscan. pp. 352.
- Rot-Nikcevic I, Taylor CN, Wassersug RJ. 2006. The role of images of conspecifics as visual cues in the development and behavior of larval anurans. Behavioral Ecology and Sociobiology 60: 19–25. doi:10.1007/s00265-005-0133-5.
- Scalera R. 2009. Trachemys scripta (Schoepff), common slider (Emydidae, Reptilia). In: DAISIE, editor. Handbook of alien species in Europe. Dordrecht: Springer. pp. 374.
- Schmitz OJ, Krivan V, Ovadia O. 2004. Trophic cascades: The primacy of trait‐mediated indirect interactions. Ecology Letters 7: 153–163. doi:10.1111/ele.2004.7.issue-2.
- Sih A, Bolnick DI, Luttbeg B, Orrock JL, Peacor SD, Pintor LM, Preisser E, Rehege JS, Vonesh JR. 2010. Predator-prey naïveté, antipredator behavior, and the ecology of predator invasions. Oikos 119: 610–621. doi:10.1111/j.1600-0706.2009.18039.x.
- Škaloudová B, Zemek R, Křivan V. 2007. The effect of predation risk on an acarine system. Animal Behaviour 74: 813–821. doi:10.1016/j.anbehav.2007.02.005.
- Socha R, Zemek R. 2003. Wing morph-related differences in the walking pattern and dispersal in a flightless bug, Pyrrhocoris apterus (Heteroptera: Pyrrhocoridae). Oikos 100: 35–42. doi:10.1034/j.1600-0706.2003.12100.x.
- Spieler M. 2005. Can aggregation behaviour of Phrynomantis microps tadpoles reduce predation risk? The Herpetological Journal 15: 153–157.
- StatSoft Inc. 2010. STATISTICA (data analysis software system), version 9.1. Available: www.statistica.com. Accessed Jul 2014 14.
- Van Buskirk J. 2001. Specific induced responses to different predator species in anuran larvae. Journal of Evolutionary Biology 14: 482–489. doi:10.1046/j.1420-9101.2001.00282.x.
- Van Buskirk J, McCollum SA. 2000. Influence of tail shape on tadpole swimming performance. Journal of Experimental Biology 203: 2149–2158.
- Walzer A, Schausberger P. 2013a. Phenotypic plasticity in anti-intraguild predator strategies: Mite larvae adjust their behaviours according to vulnerability and predation risk. Experimental and Applied Acarology 60: 95–115. doi:10.1007/s10493-012-9624-z.
- Walzer A, Schausberger P. 2013b. Integration of multiple cues allows threat-sensitive anti-intraguild predator responses in predatory mites. Behaviour 150: 115–132. doi:10.1163/1568539X-00003040.
- Werner EE, Peacor SD. 2003. A review of trait-mediated indirect interactions in ecological communities. Ecology 84:1083e1100.